Figures & data
Figure 1. The AH130 experimental model. (A and B) Phase-contrast (A) and electron microscopy (B) images of AH130 cell population as soon as recovered from the host animal at day 11 after transplantation. Bar = 100 µm and 10 µm, respectively. (C) An electron microscopy image of a normal rat adult liver. Bar = 10 µm. (D and E). The immunocytochemical technique revealed the negativity to Albumin (D) and α fetoprotein (E) of AH130 hepatoma cells. Bars = 50 µm. (F) The loss of p53 in ascites cells. Lysates of AH130 cells were obtained and processed in parallel with those of a positive and a negative control (Kasumi acute myeloid leukemia and K562 chronic myeloid leukemia cells, respectively). Vinculine expression was tested as immunoblot control. (G) Flow chart of experimental procedures. Six days after transplantation (mild hypoxia), aliquots of the tumor were transplantated intraperitoneally in new hosts or utilized for immunophenotypical characterization. Eleven days after transplantation (severe hypoxia), the tumor was harvested and utilized for the in vitro experiments.
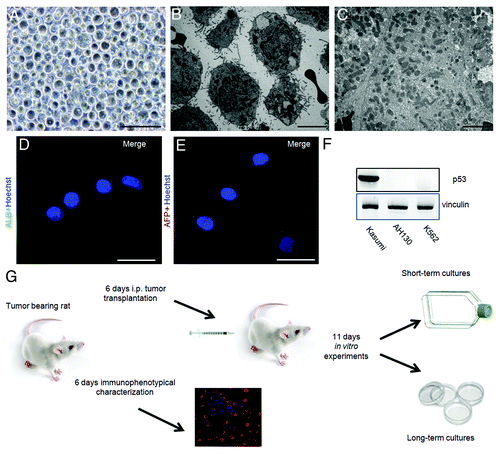
Figure 2. The role of the mitochondrial respiration on the G1/S transition. (A) Time-course of pO2 (open circles) and AH130 tumor development in vivo (closed circles) at various time after transplantation. The pO2 measurements were obtained by a Clark-type microelectrode (Beckman, 315780) mounted on the Beckman Physiological Gas Analyzer (Mod.160) connected to a recorder, according to Del Monte (1967). (B) Cell cycle distribution of AH130 cells at day 11 after transplantation as measured by cytofluorimetric technique. (C). Linear correlation between the number of cells recruited into S as measured by the R = 14C-Thymidine pulse (DPM/90 min/106 viable cells) and by cytofluorimetric technique at the corresponding times. The latter parameter was estimated by multiplying the cytofluorimetric percentage of cells in S for the total number of cells present in the sample at the corresponding time. Note the synchronization of the cells in the S phase at t = 18 as compared with t = 0.
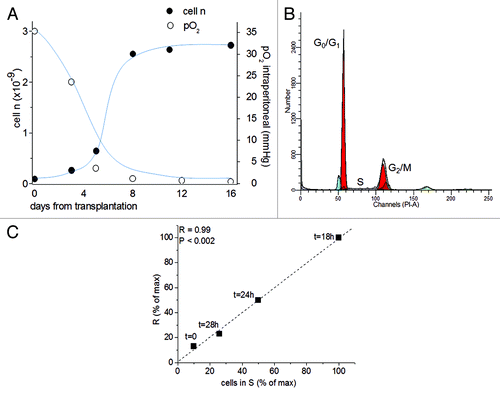
Figure 3. The metabolic check point (MCP) at the G1/S transition. The recruitment into S of ascites cells as measured by R at 18 h as compared with t = 0 and the effects of nitrogen incubation or antimycin A (6 × 10−6M) or pyruvate (10 mM) on this recruitment. Values are expressed as mean ± SEM of three experiments.
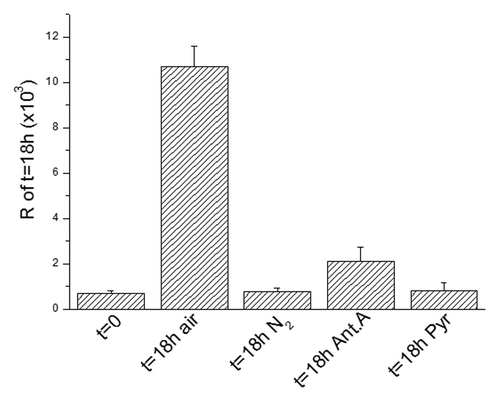
Figure 4. Immunocytochemical profile of AH130 cells at 6 and 11 d after transplantation or after 18 h of incubation in air. (A‒F) The marker signature of the cell population at day 6. Note the co-expression of Nanog and Klf4 revealed by the simultaneous exposure to the corresponding antibodies (E). Bars = 50 µm. (G‒H) The marker signature at day 11. Note the expression of Nanog alone, despite simultaneous exposure to anti-Nanog and anti-Klf4 antibodies (panel (G). Bars = 50 µm. (I‒K) The marker signature after 18 h of incubation in air. Note the expression of Klf4 alone, despite simultaneous exposure to anti-Nanog and anti-Klf4 antibodies (I) Bars = 50 µm. Cell nuclei were stained with Hoechst 33258. (L) Summary of AH130 cells immunocytochemical profiles under the various above conditions. Note the 100% expression of Nanog in N2 atmosphere (black column). Values are expressed as mean ± SEM of three experiments.
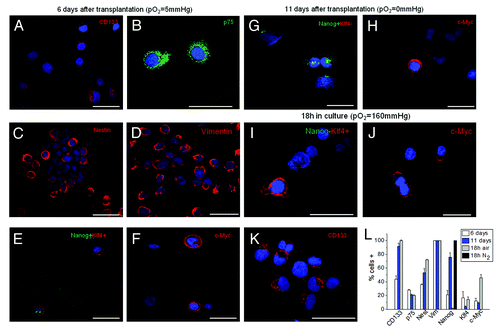
Figure 5. The intracellular modulation of ETFs quantified by RT-PCR experiments. (A‒C). Relative mRNA levels of Nanog, Klf4 and c-Myc in AH130 ascites cells at t = 0 and t = 18 h in fetal and adult liver. Values are expressed as mean ± SEM of three experiments.
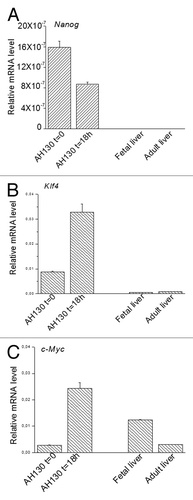
Figure 6. Time course of viability and immunophenotipycal analysis of non-adherent population. (A‒C) Cellular incorporation of propidium iodide at 24 h, 72 h and 5 d after seeding. The percentage of necrotic cells increased up to 95% throughout 5 d in culture. (D‒F1) The immunocytochemical analysis performed in the first 5 d of aerobic culture demonstrated the AH130 population is negative to CD34, CD68, calretinin, platelet/endothelial cell adhesion molecule-1, fibroblast activation protein 1. The overlay of anti-mouse Cy3-, anti-rabbit fluorescein- or anti-goat fluorescein-conjugated antibody staining and Hoechst 33258 signals is shown in the panels labeled “Merge.”
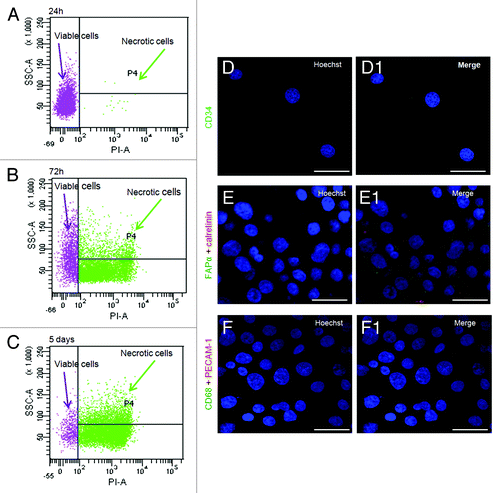
Table 1. Propidium iodide (PI) incorporation in AH130 cells
Figure 7. Morphological and immunocytochemical profile of the tumor during a long-term aerobic incubation. (A) Phase-contrast image of AH130 cell population after 5 d in aerobic culture. Bar = 50 µm. (B‒D) Representative signature of ascites cells after 5 d in aerobic culture. Bar = 50 µm. (E) Phase-contrast image of AH130 cell population after 15 d in aerobic culture. Bar = 50 µm. (F‒H) Representative signature of ascites cells after 15 d in aerobic culture. Bar = 50 µm. (I) Phase-contrast image of AH130 cell population after 30 d in aerobic culture. Bar = 50 µm. (J‒L) Representative signature of ascites cells after 30 d in aerobic culture. Bar = 50 µm. (M) Summary of AH130 cells’ immunocytochemical profiles during a long-term aerobic incubation. Values are expressed as mean ± SEM of three experiments.
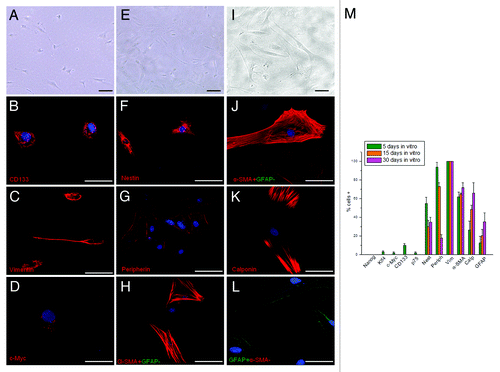
Figure 8. The role of the ETF triad operating in the AH130 reprogrammed cells to regulate cell cycle activation. (A) The pO2 modulation of the Nanog, Klf4, c-Myc transcriptional circuitry. (A1) Hypoxia (t18hyp) enhances Nanog, while it represses Klf4 and c-Myc. (A2) After transfer to aerobic culture (t18ctr), the increase of pO2 represses Nanog and enhances Klf4 and c-Myc. The fold change of Nanog and Klf4 (A1 vs A2) are equivalent in quantity but opposite in sign, so that N + K = C. (B). Diagrammatic representation of the check-points regulating the G1/S transition: the role of ETFs. (B1) The hypoxic ratio N/K = 3, 6/0, 77 is inadequate to generate the mutual interaction necessary to activate c-Myc. This produces a cell cycle check point that restricts the G1/S transition upstream to the pyruvate-sensitive MCP. (B2) The aerobic ratio N/K = 0, 54/3, 7 is adequate to activate c-Myc providing the consensus to the G1/S transition upstream to MCP. (A3) The aerobic incubation in the presence of pyruvate produces the equivalence N = K (1, 8/1, 8), again inadequate to generate the c-Myc activation. (A4) The equivalence N = K and the consequent inhibition of c-Myc activation was also produced by pyruvate in A375 human melanoma cells. (B3) In the presence of pyruvate, the inhibition of c-Myc activation brought about by the N/K equivalence reinforces upstream the block of the G1/S transition, brought about by pyruvate saturation of the respiratory chain (MCP).
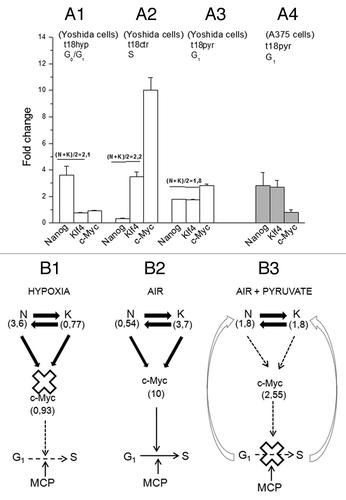