Figures & data
Figure 1. Key role of glucosamine-6-phosphate synthase GlmS for bacterial cell envelope synthesis. (A) The hexosamine pathway in Enterobacteriaceae. This pathway generates UDP–GlcNAc from Fru6P and glutamine (Gln) in four sequential reactions catalyzed by enzymes GlmS, GlmM, and GlmU. UDP–GlcNAc is the dedicated precursor for biosynthesis of peptidoglycan and lipopolysaccharides. GlmS catalyzes synthesis of GlcN6P, which is the key reaction. If available, various amino sugars can be taken up and converted to GlcN6P, bypassing the need for GlmS. GlcN6P can also be recycled from degradation of peptidoglycan. Degradation by enzyme NagB allows utilization of GlcN6P as nitrogen and carbon source. (B) Origin and fate of the glmUS transcript in E. coli. Genes glmU and glmS are co-transcribed from two promoters. In the absence of external amino sugars, promoter P1 is activated by transcriptional regulator NagC increasing transcription rates 3-fold. The glmUS co-transcript is processed by RNase E generating monocistronic mRNAs that are usually rapidly degraded. Upon GlcN6P depletion, the glmS mRNA can be stabilized by base-pairing with sRNA GlmZ enhancing GlmS synthesis.
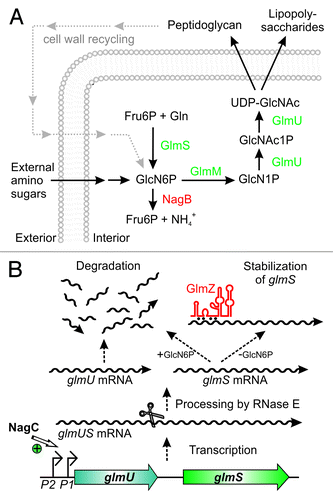
Figure 2. Activation of glmS by base-pairing with sRNA GlmZ. (A) Secondary structure of the glmUS intercistronic region and mechanism of activation of glmS translation by GlmZ. The glmUS co-transcript is processed by RNase E at the glmU stop codon (top). The two adjacent stem-loops might contribute to recognition by RNase E. A stem loop masks the SD in the glmS mRNA, thereby limiting translation initiation (top). Assisted by Hfq, full-length GlmZ base-pairs with the left half-site of this stem loop, opening the structure and providing access to ribosomes (bottom). The 5′ UTR of glmS contains two (ARN)3 motifs, providing binding sites for Hfq. Base-pairing nucleotides in glmS and GlmZ are shown in purple and red, respectively. (B and C) Secondary structures of homologous sRNAs GlmZ and GlmY. N.B., the glmS base-pairing site in GlmZ (marked red) is absent in GlmY. Processing sites are labeled by scissors.
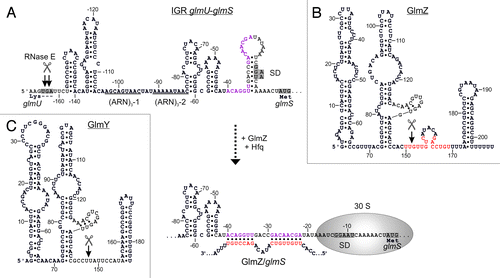
Figure 4. Control of GlmY and GlmZ expression in E. coli. (A) Genomic context of genes glmY and glmZ and role of the TCS GlrK/GlrR (QseE/QseF) for glmY transcription. GlmY can be transcribed from overlapping σ70/σ54 promoters. Transcription of glmY by σ54 RNA-polymerase relies on activator protein GlrR and integration host factor (IHF). GlrR is a response regulator and requires phosphorylation by histidine kinase GlrK for increased DNA binding activity. The TCS GlrK/GlrR is encoded downstream of glmY within the glrK-yfhG-glrR locus, suggesting a functional connection with outer membrane protein YfhG. In E. coli, glmZ is transcribed from a constitutively active σ70 promoter. (B) The glmY promoter in E. coli K12. The sequences of the overlapping σ70/σ54 promoters are boxed in purple and yellow, respectively. The GlrR and IHF binding sites are depicted in green and orange. A putative binding site for response regulator QseB is marked in purple. Formation of the open complex by σ54 RNA-polymerase requires interaction of σ54 with a GlrR hexamer. This process is facilitated by the DNA bending activity of IHF (bottom).
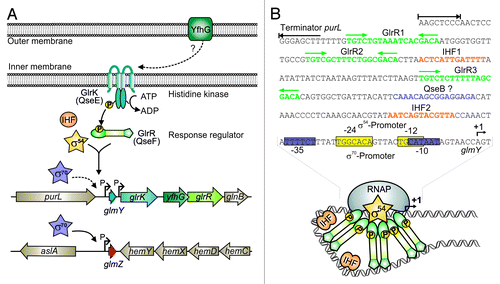
Figure 3. Maintenance of GlcN6P homeostasis by the regulatory GlmY/GlmZ/RapZ circuit. Under ample GlcN6P supply, sRNA GlmY is present in low amounts. Therefore, adaptor protein RapZ recruits the homologous sRNA GlmZ for cleavage by RNase E in a process that involves physical interaction of both proteins. Processed GlmZ lacks complementarity to glmS and is unable to activate glmS expression. Consequently, the glmS SD is not accessible to ribosomes, leading to low translation rates and rapid degradation of the mRNA. In addition, high GlcN6P concentrations trigger conversion of preexisting GlmS dimers to enzymatically inactive hexamers, providing feedback regulation at the protein level.Citation82 Upon GlcN6P limitation, the processed variant of GlmY accumulates and sequesters RapZ by an RNA mimicry mechanism. As a result, GlmZ cannot be cleaved by RNase E. Consequently, unprocessed GlmZ accumulates and base-pairs with the glmS mRNA in an Hfq-dependent manner. Base-pairing disrupts the inhibitory stem loop occluding the SD, thereby allowing translation of glmS, which concomitantly stabilizes the transcript. The newly synthesized GlmS replenishes GlcN6P.
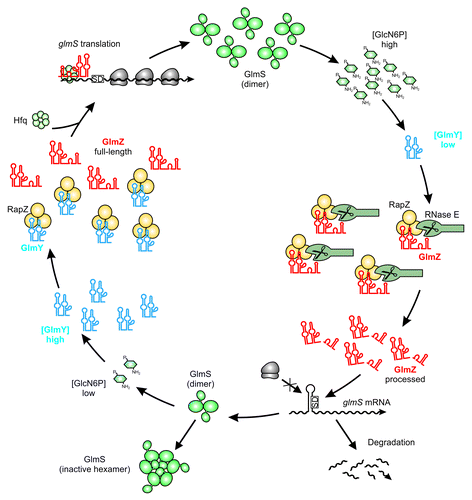