Figures & data
Figure 1. Regulatory cascade controlling expression of Y. pseudotuberculosis early-stage virulence genes. Expression of the virulence genes invasin (invA) and psaA is activated by the thermosensing virulence regulator RovA. Synthesis of RovA is controlled in a nutrient-dependent manner by a regulatory cascade, including the cAMP receptor protein (Crp) and the two-component regulatory proteins UvrY/BarA. They regulate components of the carbon storage regulator (Csr) system, i.e., the regulatory RNAs CsrB and CsrC and the RNA-binding protein CsrA. Upregulation of CsrB and/or CsrC leads to the sequestration of CsrA, whereby synthesis of the LysR-type repressor RovM is inhibited, which allows production of RovA. Furthermore, RovM synthesis is repressed by Crp downstream of CsrA via an unknown mechanism. The new finding that csrC expression is activated by the PhoP/PhoQ two component system is highlighted in red. Solid lines illustrate direct control, and dashed lines indirect regulation by the indicated regulator.
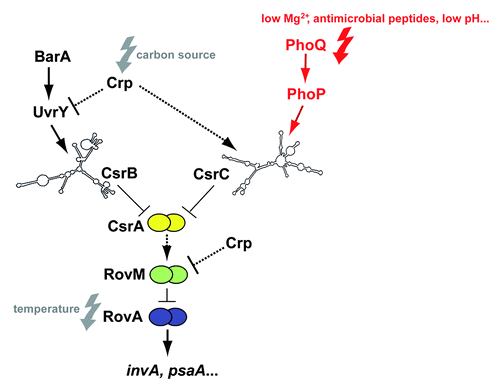
Figure 2. PhoP-dependent RovA expression is mediated via RovM. Whole cell extracts of YPIII (phoP-), YP149 (YPIII phoP+), IP32953 (phoP+), and YPIP06 (IP32953 phoP-) grown to exponential phase at 25 °C, 32 °C, and 37 °C were separated by SDS-PAGE prior to western blotting using polyclonal RovA- and RovM-specific antibodies. As negative controls rovA and rovM deletion strains YP107 and YP72 were included. Relative protein amounts were determined densiometrically using the software ImageJ for three independent experiments and normalized to the respective unspecific protein band (c). Statistical analysis was performed by student’s t test with *, P ≤ 0.05; **, P ≤ 0.005; ***, P ≤ 0.001; n.s., not significant; and n.d., not detectable.
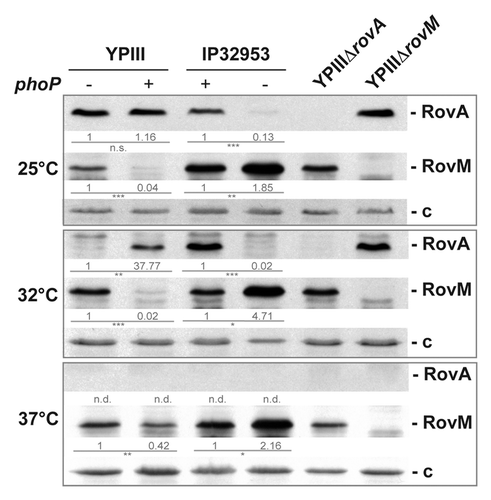
Figure 3. Analysis of crp and csrA expression in phoP+ and phoP- strains. The vector pAKH139, harboring a crp-lacZ fusion (A) and plasmid pKB63, harboring a csrA-lacZ fusion (B) were transformed into Y. pseudotuberculosis strains YPIII (phoP-), YP149 (YPIII phoP+), IP32953 (phoP+), and YPIP06 (IP32953 phoP-). The bacteria were grown to exponential growth phase in LB medium at 25 °C. The data represent the mean ± SEM from three independent experiments each performed in triplicates. Whole cell extracts of YPIII (phoP-), YP149 (YPIII phoP+), IP32953 (phoP+), and YPIP06 (IP32953 phoP-) grown to exponential growth phase at 25 °C were separated by SDS-PAGE prior to western blotting using polyclonal Crp- (C) and CsrA (D)-specific antibodies. As negative controls crp and csrA deletion strains YP89 and YP53 were included. Relative protein amounts were determined densiometrically using the software ImageJ for three independent experiments and normalized to the respective unspecific protein band (c). Statistical analysis was performed by student’s t test with *, P ≤ 0.05; **, P ≤ 0.005; ***, P ≤ 0.001; n.s., not significant; and n.d., not detectable.
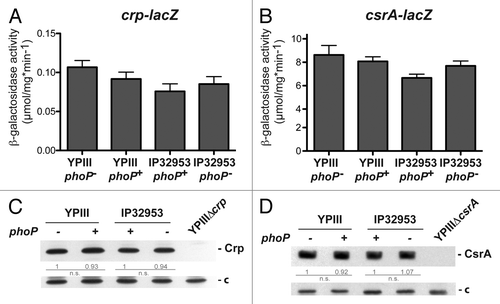
Figure 4. Influence of PhoP on CsrB and CsrC synthesis. The vector pAKH101, harboring a csrB-lacZ fusion (A), and the vector pAKH103, harboring a csrC-lacZ fusion (D), were transformed into Y. pseudotuberculosis strains YPIII (phoP-), YP149 (YPIII phoP+), IP32953 (phoP+), and YPIP06 (IP32953 phoP-). The cells were grown to exponential growth phase in LB medium at 25 °C. The data represent the mean ± SEM from at least two different experiments each done in triplicate and were analyzed with Student’s t test. ***P < 0,001. Total RNA from Y. pseudotuberculosis strains YPIII (phoP-), YP149 (YPIII phoP+), IP32953 (phoP+), and YPIP06 (IP32953 phoP-) grown to exponential growth phase at 25 °C (B and E) or 37 °C (C and F) was prepared and analyzed by northern blotting with CsrB- (B and C) and CsrC (E and F)-specific probes. YPIII ΔcsrB and YPIII ΔcsrC served as negative control, respectively.
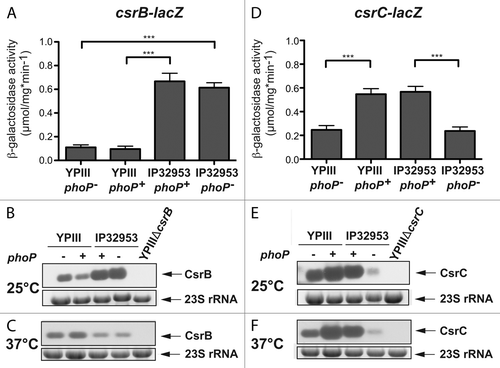
Figure 5. Interaction of PhoP with the csrC promoter region. Three different DNA promoter fragments of csrC were incubated with increasing concentrations of PhoP. A gyrA fragment was used as negative control (c). The PhoP–DNA complexes were separated on 5% polyacrylamide gels. The position of the specific higher molecular weight DNA–protein complexes is marked with an asterisk. The analyzed fragments were the following: csrC 1 (-297 to +93) (A), csrC 2 (-297 to -55) (B), csrC 3 (-76 to +93) (C), and csrC 2 (-297–-55) competitive with csrC 3 (-76–+93) (D). The relative amount of unbound DNA was determined densiometrically using the software ImageJ and the dissociation constant (Kd) was calculated. The Kd values represent the mean ± SEM of three independent experiments.
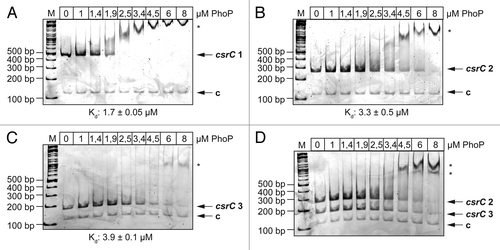
Figure 6. DNase I footprinting of PhoP with the csrC regulatory region. Sense (A) and antisense (B) csrC-probes were DIG-labeled and incubated with increasing amounts of purified His-PhoP or His-PhoP preincubated with acetyl phosphate. DNA or PhoP–DNA complexes were digested with DNase I and separated on a 6% polyacrylamide gel. Lanes T, C, A, and G represent the Sanger sequencing reactions. The DNase I protected regions are indicated with vertical bars, hypersensitive regions are marked with an asterisk. The numbers indicate the nucleotide positions upstream of csrC.
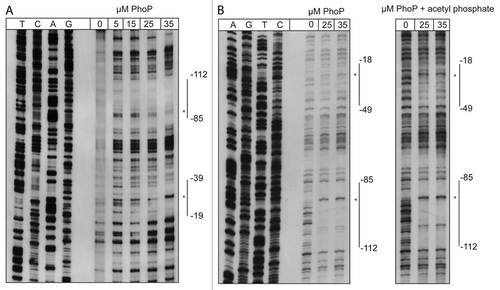
Figure 7. PhoP-dependent transcription of csrC occurs from two distinct start sites. (A) Mapping of the csrC transcriptional start site. For primer extension of the CsrC transcript total RNA isolated from YPIII and YP149 (YPIII phoP+) grown at 25 °C in LB, and a Dig-labeled csrC-specific primer was used. The sequencing reaction (left site) was performed with the same primer used for the extension reaction. The transcriptional start sites (TSSs) are indicated by arrows. (B) Regulatory region of csrC, illustrating the two putative promoters, the identified transcriptional start sites, the DNase I protected areas (highlighted in gray), and putative PhoP boxes (bold letters). (C) High-resolution northern blot of CsrC revealing two CsrC isoforms. Total RNA was isolated from YPIII (phoP-) and YP149 (YPIII phoP+) as well as IP32953 (phoP+) and YPIP06 (IP32953 phoP-) grown to exponential growth phase at 25 °C in LB. Ten µg of RNA were separated using urea-acrylamide gels and analyzed by northern blotting with CsrC-specific probes. YPIII ΔcsrC served as negative control.
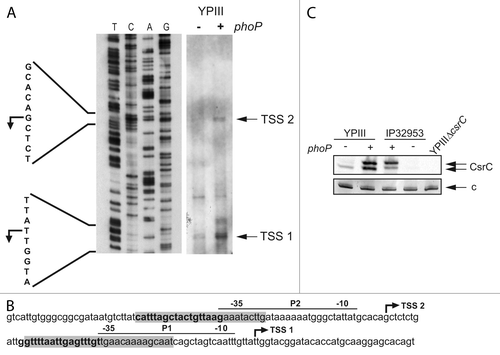
Figure 8. RNA stability assay of CsrC. (A) A RNA stability assay of CsrC in the three different strains YP308 (CsrCYPIII), YP307 (CsrCIP32953), and YP306 (CsrCYPIII+20nt IP32953) was performed. RNA synthesis was stopped by adding 2 mg/ml rifampicin and samples were taken after 0, 15, 30, 45, 60, 90, and 120 min. (B) The half-life of CsrC was measured by a least squares analysis of semi-logarithmic plots of RNA concentration vs. time and represent the mean ± SEM from three independent experiments.
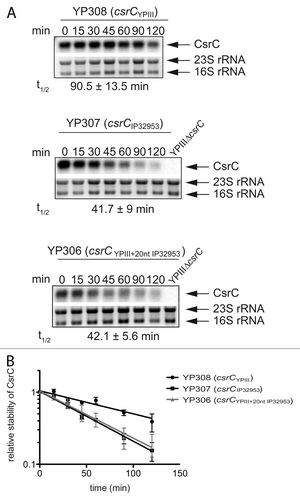
Figure 9. Model of PhoP-dependent regulation of csrC transcription. The current model displays PhoP-dependent regulation of csrC expression and derivative-specific differences in CsrC transcript stability in Y. pseudotuberculosis YPIII and IP32953. Expression of csrC is positively regulated by PhoP via direct DNA-interaction. PhoP stimulates csrC expression from two distinct promoters resulting in two CsrC isoforms. IP32953 CsrC carries an additional 20 nucleotides stretch (black box) leading to decreased CsrC stability.
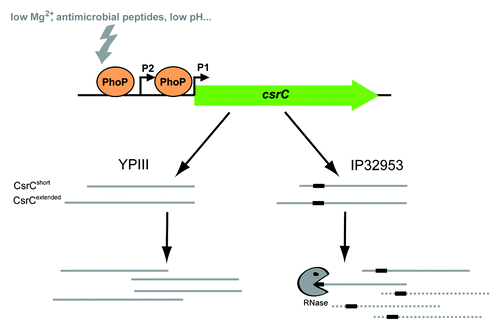