Figures & data
Figure 1 Rho-dependent termination of transcription. (A) The various crystal structures of the Rho hexamerCitation6,Citation10,Citation12 with bound RNA segments in red and nucleotide cofactors in green, filled balls. Hexamers are shown from their top, N-terminal faces. In the first structure (PDB #1PVO; left),Citation10 the hexamer forms a splint-open, “lock-washer” ring which may mimic the transient state required for RNA entry into the ring channel during formation of a catalytically-competent Rho-RNA complex (see C). The two other structures represent distinct, conflicting models of the translocating Rho enzyme. In one model (PDB #2HT1, middle),Citation12 the Rho ring is a trimer of dimers and contains RNA bound to the composite, crown-like primary binding site (PBS). Electron density was insufficient to position RNA precisely in the central channel of the trimer of dimers.Citation12 The other model (PDB #3ICE, right)Citation6 shows an asymmetric Rho hexamer without RNA in the PBS but with an r(U)6 segment bound into the ring channel in a staircase-like arrangement reminiscent of that observed for single-stranded DNA in the crystal structure of the ring-shaped papillomavirus E1 helicase.Citation29 (B) Schematic depiction of Rho-dependent termination of transcription in the context of bacterial gene expression. Note that, contrary to what is shown here, Rho and RNA polymerase may form a binary complex throughout the transcription cycleCitation21 and that RNA may also remain bound to the PBS during translocation.Citation1 (C) The putative Rho activation pathway. Rho bears two separate RNA binding sites that have different but coordinated functions. On top (5′-end of RNA) of the hexameric ring, the PBS is made of six identical subunit clefts (white ovals), each capable of specifically holding the base residues of a 5′-YC dimer (PBS-bound dimers are shown in red on (A), left and center). PBS clefts are responsible for tight and preferential Rho binding to C-rich Rut sites (in red).Citation1 Once bound to the PBS, the substrate presumably awaits transient opening of the Rho ringCitation10,Citation11 to enter its central channel and bind SBS components (SBS-bound RNA is shown in red on A, right). Only upon completion of this multi-step process, which is slow and rate-limiting in vitro does Rho become enzymatically active.Citation1
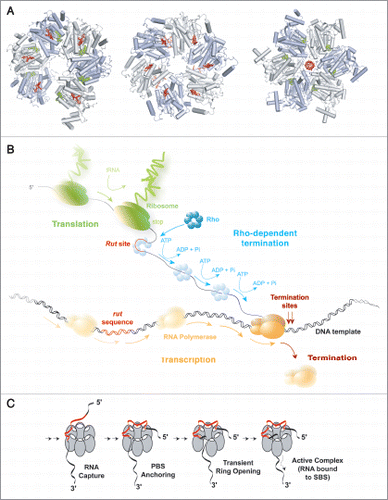
Figure 2 The various models proposed for the mechanochemical cycle of Rho. (A) The escort modelCitation6,Citation29 posits that each subunit binds and translocates a single RNA contact sequentially through the ATP cycle. Lower-case letters indicate the conformational states that each subunit successively adopts to escort a nucleotide through the ring. Green and red circles represent, respectively, the two extreme “distal” and “proximal” subunit conformations. (B) The open-ring modelCitation14 proposes that ATP binding leads to uniform, out-of-plane conformational changes at the subunit interfaces that ultimately lead to large (∼40 A) distance movements between the latch and the lever subunits. Release of product leads to a reset in the hexamer that triggers a burst of RNA translocation. (C) The large-lever modelCitation13 suggests that an internal lever binds and translocates RNA. (D) the RNA scrunching model13 indicates that the RNA is internally compacted during the ATP binding phase (RNA is translocated while at the same time fixed to a latch subunit) and released in a rapid burst in the product release phase.
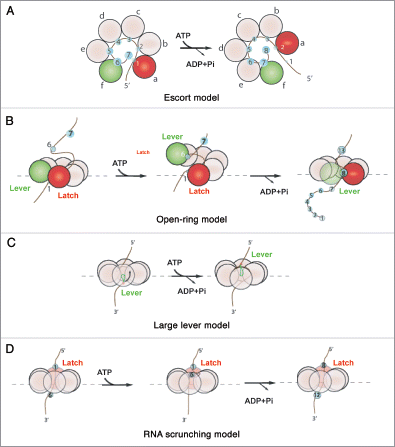