Abstract
Objectives
To investigate the surface chemical changes in dynamic interactions of delmopinol on salivary films on oral mucosa in healthy participants after rinsing with an unbuffered water solution of delmopinol, and to examine the oral tissue disposition of delmopinol in rats after local administration.
Material and Methods
The contact angle technique was used to monitor the interaction of delmopinol with the salivary film coating the upper labial mucosa of 10 healthy participants through a 4 h period. The tissue disposition of 14C-labelled delmopinol was examined in rats by autoradiography.
Results
Rinsing with delmopinol increased the polarity of the saliva coated mucosa during the time of observation. The binding of delmopinol was verified in the autoradiograms showing that radioactivity remained in the rat oral mucosa after 24 h. Delmopinol was however not irreversibly bound.
Conclusions
The findings indicate that delmopinol interacts with the salivary film of the upper labial mucosa and affects its polarity. It appears that delmopinol assists in the maintenance of the hydrophilicity of the mucosal pellicle and thereby also reinforcing hydration of the mucosa. The rat autoradiograms, showed that radioactivity remains in the oral mucosa after 24 h, but diffuses through the mucosal membranes into the systemic circulation.
Introduction
Delmopinol is a tertiary amine surfactant, developed as an anti-plaque agent to reduce plaque and gingivitis as an adjunct to oral mechanical cleaning. In contrast to other products on the market delmopinol has comparably low antibacterial properties that should not disrupt the natural balance of the oral microbiota. Instead preclinical studies have suggested that one important aspect for the efficacy of delmopinol is that the compound counteracts new plaque formation by interfering with enzymes responsible for the synthesis of polysaccharides important for plaque matrix formation and stability [Citation1–5].
A large number of phase I–II clinical studies have been performed to study the pharmacodynamics and pharmacokinetics of a mouthwash formulation of delmopinol hydrochloride [Citation6–12]. These studies ended up with presenting a mouthwash formulation containing 2 mg/mL of delmopinol hydrochloride intended for oral rinse during 1 min and then to be expectorated. Eight double-blind, parallel-group designed phase III studies were thereafter carried out, showing that rinsing with delmopinol hydrochloride 0.2% (2 mg/mL) fulfilled the ADA effectiveness criteria for controlling plaque and gingivitis [Citation13–18].
The focus of interest in the above-mentioned studies was to show the effect of delmopinol on tooth surfaces in relation to plaque accumulation. Thus, the influence of delmopinol on the wettability of tooth surfaces in vivo was studied with contact angle measurements in humans rinsing with 10 mL of a water solution of delmopinol hydrochloride, 2 mg/mL [Citation19]. It was suggested that delmopinol exposes polar parts of the molecule after adsorption, but this effect was short-lived on the tooth surface (15–30 min). However, the clinical actions of delmopinol may also exert an influence on the oral mucosa, that together with salivary associated films constitute a protective barrier together with the desquamation process. Saliva covers, protects and interacts with all surfaces present in the oral cavity. However, there are groups of patients with for example decreased salivary flow, decreased immune response, oral mucosal conditions due to systemic diseases or medical treatments, who experience extreme discomfort and pain during tooth brushing. This may lead to reluctance in coherence to fulfil daily oral hygiene routines. For these individuals, it is imperative to be able to offer an effective, easy-to-use and affordable comfortable alternative cleaning product, to maintain oral health, often for long term use.
As described by Eriksson et al. [Citation12], the interaction between delmopinol and mucosal surfaces is of great importance for the pharmacokinetics of delmopinol. Secondly, a transient numbing sensation, especially of the tongue, has been noted as a common side effect of delmopinol, along with a low number of reported taste disturbances [Citation18]. For the third, a possible positive side effect, is a reduction of the frequency in occurrence of aphthous stomatitis, spontaneously reported by patients in the phase III studies.
It is plausible that delmopinol will have a longer adsorption rate on the hydrated soft mucus covered oral surfaces, than what has been demonstrated on hard enamel tooth surfaces (20 min), since the surface area of the mucosa is much larger and together with saliva form a complex layered structure. This study was thus designed to assess for how long time a water solution of delmopinol will reside on soft tissue surfaces in the oral cavity, which can be demonstrated in humans by recording contact angles of droplets of polar and non-polar test liquids applied on the saliva coated oral mucosa at certain time intervals after rinsing with delmopinol, and by examination of the oral tissue disposition of 14C-labelled delmopinol in rats by autoradiography after local administration (painting of the oral cavity).
Materials and methods
Investigated compounds
The delmopinol hydrochloride, (±)-3-(4-propylheptyl)-4-morpholineethanol hydrochloride, was synthesized at the Chemical Department at Biosurface AB, Malmö, Sweden, or at DuPont ChemoSwed, Malmö, Sweden. The chemical purity of the compound was 99–100%.
Labelled delmopinol (base), used in the autoradiographic study, was synthesized by Amersham International plc, England. The 14C-labels were positioned at carbon 5 and 6 in the morpholine ring. The specific radioactivity was 3.85 MBq mg−1 (104 μCi/mg). 500 μCi of the labelled compound was dissolved in 1 mL of ethanol. The radiochemical purity of the compound was 98%. For preparation of the test solution the ethanolic solution of 14C-delmopinol (base) was evaporated under nitrogen gas, and an equimolar amount of HCl was added to bring the 14C-delmopinol-base into its hydrochloride form. Unlabelled delmopinol HCl, dissolved in water, was added to give a final concentration of delmopinol HCl (labelled and unlabelled) of 0.2% (2.0 mg/mL) and a radioactivity of 3.1 MBq/mL (85 μCi/mL).
The test liquids used in the contact angle determinations were obtained from Fisher Scientific, US (glycerol, methylene iodide, s-tetrabromoethane, propylene carbonate) and Eastman Chemical Company, US (thiodiglycol, n-hexane). All liquids were of high purity grade. The surface tension of the liquids was determined in our laboratory prior to start of the study.
Study participants
Ten healthy participants, five males and five females, were matched for age and gender among the staff at the Centre for Oral Health Sciences, Malmö University, Sweden. Initially an oral clinical examination was performed to screen individuals in order to include participants with the following inclusion criteria: healthy gingival and upper lip mucosa, perceived good oral and general health, anatomical prerequisite to perform measurements on the upper lip mucosa, no history of drug allergies, and in the age span of 20–65 years. Exclusion criteria were defined as ongoing or within three weeks ended antibiotic treatment, any medication affecting saliva secretion, smokers and use of smokeless tobacco products, fixed or removable prosthetic reconstructions involving the central incisors. Oral and written information was given by the Investigators and Informed consent was obtained from all participants.
At the day of rinsing with delmopinol, the participants were instructed to refrain from eating, drinking, using any form of tobacco, and performing any oral hygiene practise for 2 h prior to measurements.
Assessment of oral mucosal wettability using contact angle determinations
The test substance, delmopinol hydrochloride, was prepared as a 0.2% solution (2 mg/mL) in distilled water and adjusted to pH 5.7 by the addition of NaOH. The solution was prepared fresh in the morning prior to the experiment. The subjects rinsed with 10 mL of the delmopinol solution for 1 min, and thereafter the solution was expectorated.
The clinical adhesiveness of the oral surfaces [Citation20] before and after rinsing was assessed by an indirect technique measuring contact angles from photographs taken when clean liquids with defined surface tension values were allowed to interact with the saliva coated upper lip oral mucosal surface [Citation21]. The test liquids used for contact angle measurements are listed in .
Table 1. Surface tension data for the test liquids used for the contact angle measurements.
The contribution of polar forces in the liquids is declining with the highest value for glycerol, followed by thiodiglycol and methylene iodide. s-Tetrabromoethane, propylene carbonate and hexane are categorized as non-polar.
One drop of each liquid, was delivered by a 16-gauge platinum wire, and applied on the inside of the upper lip mucosa, in an area corresponding to the 4 incisors, after the participant had been positioned horizontally in the dental chair between the light and the optical source. The equipment used was a digital single lens reflex camera (Finepix S5 Pro, Fujifilm, Japan) with Nikon AF-S Micro Nikkor (105 mm, 1:2,8 G ED) lenses. Prior to this procedure the participant was asked to wet the inside of the upper lip with the tongue. The reason for choosing the upper labial mucosa for the contact angle measurements, is that there are a less number of small mucosal salivary glands that may interfere/affect the contact angle measurements, than for example in the lower labial mucosa.
At the time when the liquid reached a mechanical equilibrium the drop was registered by a camera technique described by Glantz et al. [Citation21] First, contact angles were recorded at the baseline, e.g. immediately before the rinsing procedure. Within one minute after the rinse a new set of contact angle recordings were performed. The recordings were then repeated after 0.5, 1, 2 and 4 h.
The contact angles were measured directly on the developed film strips viewed through an enlarger/viewer screen, by the use of a protractor graduated in whole degrees. Two opposing contact angles were measured per drop.
Statistical methods
Mean and standard deviation were determined for the contact angles for each of the test liquids at the different time points in each individual. Test for changes over time was performed according to Fisher’s non-parametric permutation test for matched pairs [Citation22].
Autoradiographic studies of rats
In total 25 rats were included in the autoradiographic investigations. Delmopinol was administrated by intravenous injections, by gastric intubation or by painting in the mouth. In this paper, the presentation will be limited to the rats painted in the oral cavity.
Male albino Sprague-Dawley rats were obtained from Möllegaards Breeding Centre Ltd, Skensved, Denmark. Prior to the study the rats were examined in order to ensure that they were in good state of health. The animals were allowed to get acclimatized for 12 days in the animal facilities before start of the experiments. They were housed in air-conditioned rooms at 20–24 °C and a relative humidity of 20–40%. The animals had free access to water and a pellet diet (R3, Ewos, Södertälje, Sweden). The rats weighed 167 ± 10 g at the days of experiments. The animals were fasting overnight before administration of the test solution, which was administered by painting intra orally with a marten hair brush. The painting was performed during 2 min on conscious animals, immobilized by being held in a hand. The teeth, the gingiva, the palate, the cheeks and the tongue were painted. The amount of painted test solution was calculated by weighing the test tubes with the solution before and after the paintings. The solution applied varied between 92 and 119 μL, corresponding to 0.3–0.4 MBq (8–10 μCi).
Two animals were included per survival time. At the stated intervals (1, 4 and 24 h) the rats were killed by CO2-asphyxiation, embedded in carboxymethyl cellulose and mounted on microtome stages by immersing and rapidly freezing them in hexane cooled with CO2-ice (−78 °C) according to the method of Ullberg et al. [Citation23] One of the animals was sectioned sagittaly through the whole body, whereas the other was sectioned transversally through the head. Fifteen freeze-dried sections from each animal were without further treatment exposed to X-ray films. Five additional sections from each animal were extracted with 5% trichloroacetic acid, distilled water, methanol and heptane before they were exposed to X-ray films. The washing procedure removed material, which was not firmly bound to the tissues.
The clinical study was approved by the Ethics Committee of the Faculty of Medicine, University of Lund, Sweden. The autoradiographic study in rats followed GLP guidelines.
Results
Clinical study
The contact angles were read off from developed film strips. Optimally 72 contact angles were to be registered for each participant (6 test liquids, 2 angles recorded for each liquid at six recording occasions). Including all participants, a total of 720 contact angels could be registered. However, all contact angles could not be measured due to the quality of the developed film strips. For example, the point of contact between the liquid and the lip mucosa in the developed film strips could be out of focus and therefore not measurable. Participant number 5 had to terminate the sequence of measurements at 2 h before the completion of the experiment due to the need of attending family business. These non-registrable contact angles constituted 14% of all contact angle registered. The number of contact angles of the liquids measured per individual was meeting the criteria to follow changes in wettability. The precision of the method is ±2 degrees (SD). However, due to the difficulty in doing precise contact angle measurements on the developed film strips with the protractor, angles between 2 and 10 degrees were arbitrarily set to 5.
and show changes over time for the contact angles of the different liquids. For the two polar liquids, glycerol and thiodiglycol, a decrease from their baseline values was noted at the first registration but recovered close to the baseline value at the end of the period, i.e. at 4 h after the rinsing. The contact angles for the non-polar liquids, liquids number 4 to 6 seem to be comparatively stable throughout the 4 h observation period.
Figure 1. Mean for all subjects for changes over time from baseline for the contact angles obtained for each test liquid. Note that the time-line is not linear. p<.05 and p<.01 are denoted as * and **, respectively.
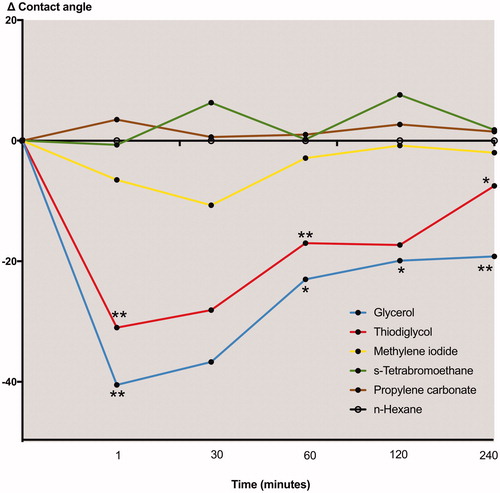
Table 2. Mean, standard deviation (SD) and range for changes over time from baseline for the contact angles obtained for the test liquids applied.
Autoradiographic study of rats
The whole-body autoradiograms of the rats painted with solutions of 14C-delmopinol in the mouth and killed after 1 h (A), 4 h (B) and 24 h (C) are shown in . As can be seen, there is a labelling of the oral and oesophageal mucosa. The distribution pattern in pictures (A) and (B) are similar to those obtained in rats killed at the same intervals after oral administration. However, no labelling of the oral or oesophageal mucosa was observed in the intravenously administered rats (data not included in this paper). In (C) there is still a labelling of the oral mucosa, but in other tissues the radioactivity is very low.
Figure 2. Whole-body autoradiograms of male Sprague-Dawley rats painted with solutions of 14C-delmopinol in the mouth and killed after (A) 1 h, (B) 4 h and (C) 24 h.
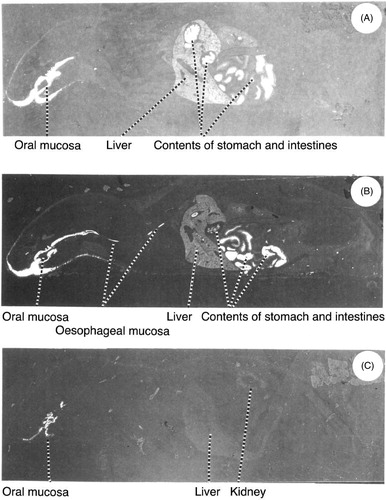
shows an autoradiogram (A) and the corresponding tissue (B) of a transversal section through the head of a rat killed 1 h after administration. The labelling of the mouth included the mucosa of the cheeks, the tongue, the gingiva and the palate. A labelling of the surface of the teeth can also be seen. The washing of the sections removed most of the radioactivity in the oral cavity.
Discussion
Saliva continuously provides all surfaces exposed in the oral cavity with a thin salivary film containing primarily a number of surface active glycoproteins. The functions of this saliva film are to protect oral surfaces from mechanical, physical and chemical influence [Citation24]. The salivary film constitutes the primary layer in the defence barrier, which also includes the underlying oral mucosa/tooth surfaces. The adhesion of microorganisms is effectively prolonged in the presence of a salivary film, since the saliva proteins lower the surface energy of clean polished surface enamel. Depending on the surface chemistry of the underlying surface, the film presents varying molecular packing arrangements, which may affect the diffusion rate for certain components as well as the microbial retention capacity of the oral surfaces [Citation25,Citation26].
In a previous study the influence of delmopinol on the wettability of tooth surfaces in vivo was studied with contact angle measurements in humans rinsing with 10 mL of delmopinol, 2 mg/mL, in water [Citation19]. The experiment was performed in the same way as in the present study, but included only glycerol and methylene iodide as pure liquids for contact angle measurements. It was found that rinsing with delmopinol reduced the contact angle of glycerol, while no such effect was observed for methylene iodide. It was suggested that delmopinol exposes the polar part of the molecule outwards from the tooth surface after adsorption. However, this effect was short-lived and after 15–30 min the contact angles approached the control level.
The present study also showed a significant decrease in the contact angles of the polar liquids in humans rinsing with a solution of delmopinol, 2 mg/mL, as seen in . These differences are statistically significant for most of the observations. This effect was not short-lived but persisted over the entire 4 h study period. No such differences from baseline were found for the apolar liquids. These results indicate that delmopinol interacts with salivary films on the oral mucosa, gradually exposing hydrophilic molecules outwards of the saliva coated labial mucosa. Due to the increased polarity of the saliva coated mucosa, delmopinol will probably act as an enabler of saliva association to the upper lip mucosa, which may be beneficial for hydration and lower friction. This may be one effect that would specifically be suited for utilizing delmopinol as an effective mouth rinse for patients who are extremely sensitive to mechanical cleansing regiments. However, whether the increased accessibility of polar units is due to e.g. desorption of salivary components, rearrangements/conformational changes of proteins within the salivary film, formation of layers of delmopinol molecules exposing their polar part outwards or combinations thereof, cannot be concluded from this study. Due to the complexity of the salivary film adsorbed to oral tissues, data obtained from contact angle measurements with polar and non-polar liquids are quite difficult to interpret. To our knowledge, however, this technique is the only method available to measure surface chemical interactions in vivo and in situ.
The rat autoradiograms seem to support the human data. As seen in and , there was a marked radioactivity in the mucosa of the mouth and oesophagus. This is most probably related to the administration procedure (painting), since a corresponding picture was not observed in the intravenously administered rats. The radioactivity in the oral mucosa should therefore represent presence of parent compound (delmopinol) and not e.g. metabolites circulating from blood, even if a substantial part of the locally administered dose of delmopinol can be assumed to be swallowed. As can be seen in , some radioactivity remained in the oral mucosa after 24 h, but washing of these tissue sections removed most of the radioactivity, indicating that delmopinol was not irreversibly bound to any tissue in the oral cavity.
However, one has to consider both biological species differences with respect to the gingiva and palatal mucosa as well as the difference in the administration procedures. An obvious difference is thus the fact that humans are not swallowing the solution, which is expectorated after 1 min of rinsing. The labelling of the oesophageal mucosa should therefore not be present in humans. However, the adsorption picture of delmopinol to the keratinized oral tissues in the painted rats is probably similar to what is seen in humans rinsing with delmopinol, since other studies have shown that delmopinol adsorbs to surfaces with quite different surface properties [Citation27–29]. The absorption through the keratinized tissues in terms of rate, however, can be expected to differ from the human situation.
These findings seem to agree with data from a pharmacokinetic study in humans, where twelve male volunteers either immediately swallowed (no rinsing) 10 mL of a water solution of 14C-delmopinol HCl, 2 mg/mL, or rinsed their mouth with 10 mL of the same solution [Citation12]. The subjects who rinsed, discarded the solution after 1 min and these expectorated mouthwash solutions were collected. It was found that in average 72% of the dose was expectorated after the 1 min rinsing. Thus, about 28% of the dose was retained in the oral cavity. This fraction was clearly absorbed via the buccal mucosa, as demonstrated by the high recovery of radioactivity in urine and faeces, and entered the blood stream as parent (non-metabolized) molecule. It should be added that radioactivity recovered in the saliva samples collected 0–12 h after administration contained only about 1% of the radioactive dose [Citation12].
Similar figures of buccal mucosa retention were obtained in a study with 14C-chlorhexidine [Citation30]. Thus, after rinsing with 10 mL of an aqueous solution of 0.2% chlorhexidine digluconate for 1 min, the mean total retention was found to be about 30% after reduction of the amount swallowed (n = 12). However, the systemic absorption of chlorhexidine via the buccal cavity seems to be very low as shown in a study, where ten subjects rinsed twice daily for 1 min with 10 mL of a formulated 0.2% chlorhexidine digluconate oral rinse during 6 weeks [Citation31]. Venous samples collected at the end of the 6 weeks treatment were not able to detect chlorhexidine in any blood sample.
The difference in systemic absorption between delmopinol and chlorhexidine is probably due to their physico-chemical properties. The pKa-values for delmopinol and chlorhexidine are 7.1 and 10.8, respectively. The pH of the oral cavity is regulated by a complex buffer capacity mediated by saliva electrolytes and a number of small proteins. The pH in the mouth differs in different locations depending on the ecological niche, why the pH is influenced by the amount and buffer capacity of the saliva which reaches a particular location. There are few reports on the pH values of saliva coated mucosa but they all conclude that the variation is large and therefore a close range value cannot be confident. The average pH range for unstimulated whole saliva is 6.2–7.6, and the pH of buccal mucosa has been estimated with a mean pH value of 6.5–7.1 [Citation32]. Over these pH-ranges, chlorhexidine will only exist in its protonated cationic form due to its high pKa-value, and its binding to the oral mucosa can be explained by electrostatic forces to negatively charged glycoproteins. The situation is different for delmopinol having a pKa-value of 7.1. Since the water rinse solution of delmopinol HCl used in the pharmacokinetic study was unbuffered, the pH of the mixture of the administered rinse solution and the saliva will be close to neutral due to the strong buffering effect of the saliva. Both the protonated and non-protonated form of delmopinol will therefore be present. The two forms show quite different water solubility, where ionic delmopinol is very soluble (>400 mg/mL) and the non-ionic base form is just slightly soluble (∼0.2 mg/mL). In connection with rinsing, a considerable fraction of delmopinol will be converted to the base-form and deposited on the mucosa, since the solubility limit will initially probably be exceeded. The adsorption of delmopinol to surfaces has been studied in detail and been shown to be strongly dependent on pH and concentration [Citation27]. The importance of pH and concentration for delmopinol was also found to be true with respect to its action on the removal of salivary components from surfaces [Citation28]. When studying the elution of salivary films by SDS and delmopinol on silica and hydroxyapatite surfaces, it was noticed that at low delmopinol concentrations (0.005 mg/mL and 0.1 mg/mL, where the uncharged form is still soluble), lead to some removal of the saliva layer, while around 2 mg/mL (which was the concentration used by the volunteers in our study) the solubility limit seemed to be reached and delmopinol deposited at the surfaces [Citation28].
In the human pharmacokinetic study peak plasma levels of 14C-labelled compounds were attained at 1.5 h after rinsing and 0.5 h after oral administration. For parent (non-metabolized) delmopinol, peak plasma levels occurred after 1.5 and 1.3 h, respectively [Citation12]. The authors suggested that the slower absorption after buccal compared to oral administration could be due to retention of the compound in the oral cavity. This suggestion is in line with our contact angle and autoradiography data. It also corroborates with a model in which a drug partitions into the mucosal membranes, where it is stored for extended periods, and then released out of lipid tissue into the systemic circulation [Citation33].
Furthermore, it has also been suggested that for drugs given at doses above the saturation solubility, the bioavailability becomes more dependent not only on the distribution equilibrium, but also on contact time in the mouth because additional variables, e.g. dissolution of excess drug and re-establishing the distribution equilibrium, need to be accounted for [Citation33]. The importance of contact time is supported by the pharmacokinetic results obtained in the rinse time study, where it was found that increased rinse time gave an increase in AUC and Cmax [Citation10].
Conclusions
The combined results from the contact angle determinations in humans, the rat autoradiographic pictures as well as human pharmacokinetic data suggest that delmopinol hydrochloride, administered at a concentration of 2 mg/mL in an unbuffered water solution, will be rapidly adsorbed and retained in the oral mucosa and then slowly partitioned out of this tissue into the systemic circulation. It is proposed that the major part of adsorbed delmopinol consists of its non-ionized form, which can be explained by the pKa-value for delmopinol, the great difference in water solubility between its cationic and non-ionized forms, the pH of the system as well as the concentration of delmopinol in the administered water solution.
Acknowledgements
The authors thank Professor Hans Tjälve at Uppsala University for the performance and interpretation of the rat radiograms.
Disclosure statement
The authors report no conflicts of interest related to this study.
References
- Simonsson T, Hvid EB, Rundegren J, et al. Effect of delmopinol on in vitro dental plaque formation, bacterial acid production and the number of microorganisms in human saliva. Oral Microbiol Immunol. 1991;6:305–309.
- Steinberg D, Beeman D, Bowen WH. The effect of delmopinol on glucosyltransferase adsorbed on to saliva-coated hydroxyapatite. Arch Oral Biol. 1992;37:33–38.
- Rundegren J, Simonsson T, Petersson L, et al. Effect of delmopinol on the cohesion of glucan-containing plaque formed by Streptococcus mutans in a flow cell system. J Dent Res. 1992;71:1792–1796.
- Rundegren J, Arnebrant T. Effect of delmopinol on the viscosity of extracellular glucans produced by Streptococcus mutans. Caries Res. 1992;26:281–285.
- Elworthy AJ, Wade WG. Antimicrobial properties of delmopinol against oral bacteria. Lett Appl Microbiol. 1995;20:191–194.
- Collaert B, Attstrom R, Bruyn H, et al. The effect of delmopinol rinsing on dental plaque formation and gingivitis healing. J Clin Periodontol. 1992;19:274–280.
- Collaert B, Edwardsson S, Attström R, et al. Rinsing with delmopinol 0.2% and chlorhexidine 0.2%: short-term effect on salivary microbiology, plaque, and gingivitis. J Periodontol. 1992;63:618–625.
- Moran J, Addy M, Wade WG, et al. A comparison of delmopinol and chlorhexidine on plaque regrowth over a 4-day period and salivary bacterial counts. J Clin Periodontol. 1992;19:749–753.
- Rundegren J, Bondesson Hvid E, Johansson M, et al. Effect of 4 days mouth rinsing with delmopinol or chlorhexidine on the vitality of plaque bacteria. J Clin Periodontol. 1992;19:322–325.
- Sjödin T, Håkansson J, Sparre B, et al. Pharmacokinetics and clinical efficacy of delmopinol in an open rinse time study in healthy volunteers. Am J Dent. 2011;24:383–388.
- Eriksson B, Hallström G, Ottersgård Brorsson AK, et al. Metabolic fate of delmopinol in man after mouth rinsing and after oral administration. Xenobiotica. 2000;30:179–192.
- Eriksson B, Ottersgård Brorsson AK, Hallström G, et al. Pharmacokinetics of 14C-delmopinol in the healthy male volunteer. Xenobiotica. 1998;28:1075–1081.
- Claydon N, Hunter L, Moran J, et al. A 6-month home-usage trial of 0.1% and 0.2% delmopinol mouthwashes (I). Effects on plaque, gingivitis, supragingival calculus and tooth staining. J Clin Periodontol. 1996;23:220–228.
- EIworthy AJ, Edgar R, Moran J, et al. A 6-month home-usage trial of 0.1% and 0.2% delmopinol mouthwashes (II). Effects on the plaque microflora. J Clin Periodontol. 1995;22:527–532.
- Hase J, Attström R, Edwardsson S, et al. 6-Month use of 0.2% delmopinol hydrochloride in comparison with chlorhexidine digluconate and placebo. (I). Effect on plaque formation and gingivitis. J Clin Periodontol. 1998;25:746–753.
- Hase J, Edwardsson S, Rundegren J, et al. 6-Month use of 0.2% delmopinol hydrochloride in comparison with 0.2% chlorhexidine digluconate and placebo (II). Effect on plaque and salivary microflora. J Clin Periodontol. 1998;25:841–849.
- Lang N, Hase J, Grassi M, et al. Plaque formation and gingivitis after supervised mouthrinsing with 0.2% delmopinol hydrochloride, 0.2% chlorhexidine digluconate and placebo for 6 months. Oral Dis. 1998;4:105–113.
- Addy M, Moran J, Newcombe R. Meta-analyses of studies of 0.2% delmopinol mouth rinse as an adjunct to gingival health and plaque control measures. J Clin Periodontol. 2007;34:58–65.
- Simonsson T, Arnebrant T, Petersson L. The effect of delmopinol on salivary pellicles, the wettability of tooth surfaces in vivo and bacterial cell surfaces in vitro. Biofouling. 1991;3:251–260.
- Zisman WA. Relations of the equilibrium contact angle to liquid and solid constitution. In: Gould R, editor. Contact angle, wettability and adhesion. Washington (DC): American Chemical Society; 1964. p. 1–51.
- Glantz PO, Jendresen MD, Baier RE. A clinical method for the study of in vivo adhesiveness of teeth. Acta Odontol Scand. 1980;38:371–378.
- Bradley JV. Distribution-free statistical test. London. Prentice-Hall; 1968. p. 76–78.
- Ullberg S, Larsson B, Tjälve H. Autoradiography. In: HJ Glenn, editor. Biologic application of radiotracers. Boca Raton (FL): CRC Press; 1982. p. 56–108.
- Levine MJ. Salivary molecules. A structure/function synopsis. In: Malamud D, Tabak LA, editors. Saliva as a diagnostic fluid. New York: Annals of the New York Academy of Sciences; 1993. p. 11–16.
- Christersson CE, Dunford RG, Glantz PO, et al. Effect of critical surface tension on retention of oral microorganisms. Eur J Oral Sci. 1989;97:247–256.
- Christersson CE, Dunford RG. Salivary film formation on defined solid surfaces in the presence and absence of microorganisms. Biofouling. 1991;3:237–250.
- Svensson O, Halthur T, Sjödin T, et al. The adsorption of delmopinol at the solid/liquid interface – the role of the acid-base equilibrium. J Colloid Interface Sci. 2010;350:275–281.
- Santos O, Lindh L, Halthur T, et al. Adsorption from saliva to silica and hydroxyapatite surfaces and elution of salivary films by SDS and delmopinol. Biofouling. 2010;26:697–710.
- Vassilakos N, Arnebrant T, Rundegren J. In vitro interactions of delmopinol hydrochloride with salivary films adsorbed at solid/liquid interfaces. Caries Res. 1993;27:176–182.
- Bonesvoll P. Oral pharmacology of chlorhexidine. J Clin Pharmacol. 1977;4:49–65.
- Rushton A. Safety of hibitane. II. Human experience. J Clin Periodontol. 1977;4:73–79.
- Aframian DJ, Davidowitz T, Benoliel R. The distribution of oral mucosal pH values in healthy saliva secretors. Oral Dis. 2006;12:420–423.
- Bartlett J, van der Voort Maarschalk K. Understanding the oral mucosal absorption and resulting clinical pharmacokinetics of asenapine. AAPS PharmSciTech. 2012;13:1110–1115.