Abstract
Capsule Nest survival of Water Rail and Little Crake nesting at small, midfield ponds was determined by vegetation density and proximity to the shore, respectively.
Aims To determine which habitat variables affect nest predation for two rallid species inhabiting small ponds.
Methods Habitat variables describing nest location (water depth, distance to the shore and distance to open water) and nest concealment (density and height of vegetation) were measured for natural and artificial nests. All nests were monitored to determine their fate and to identify nest predators. We used generalized linear models to assess nest survival rates and to evaluate the influence of particular variables.
Results Little Crake nest success was negatively correlated with distance to shore (the land-water edge). The survival of Water Rail nests and artificial nests was positively correlated with vegetation density. During three breeding seasons, predation rates on Little Crake nests varied from 22% to 58%, and on Water Rail nests from 36% to 91%. The main predator of natural and artificial nests was the Marsh Harrier (responsible for about 50% of natural and artificial nest failures).
Conclusion High Marsh Harrier predation rates (attacks from the air) may explain why water depth did not affect the survival rate of natural and artificial nests and why nest concealment was a factor positively influencing the nest success of the Water Rail and artificial nests. Little Crakes nest successfully closer to the shore, where vegetation is usually denser. Terrestrial mammals could more easily access Water Rail nests, which were situated in shallower water than Little Crake nests, and this may have contributed to the higher rate of nest loss in this species.
Predation is a major cause of nest failure in many different habitats and in various groups of birds (Ricklefs Citation1969, Nilsson Citation1984, Sargeant & Raveling Citation1992). In wetlands, water is a barrier which reduces the accessibility of many mammalian predators to birds’ nests, and this fact may positively affect nest success (Robertson Citation1972, Picman Citation1988, Koons & Rotella Citation2003). On the other hand, wetlands are attractive for some semi-aquatic mammalian predators, such as the American Mink Neovison vison, which can significantly reduce the breeding populations of many waterbirds (Bonesi & Palazon Citation2007). Birds that nest in wetlands are also exposed to avian predation (Opermanis et al. Citation2001, Zduniak Citation2006), and in some regions to snake predation (Hoover Citation2006). Besides predation, waterbirds’ nests are vulnerable to changes in water level, especially in tidal marshes, riverine systems, estuaries, landlocked lakes and midfield ponds (Gjerdrum et al. Citation2005, Rush et al. Citation2010). Consequently, water level affects food and nest site availability of waterbirds, the community structure of both waterbirds and their predators, and finally, the nest survival rates of many wetland bird species (Weller Citation1999, Fletcher & Koford Citation2004). Waterbird nest losses can vary among years from as much as 25% to over 90% (Sargeant & Raveling Citation1992).
In aquatic habitats, there are many micro-habitat features that may influence the probability of nest survival. These are, first of all, the characteristics of hydrophyte vegetation, i.e. vegetation type, its height and density (Guyn & Clark Citation1997, Honza et al. Citation1998, Salonen & Penttinen Citation1988, Albrecht et al. Citation2006, Pasinelli & Schiegg Citation2006), water depth (Albrecht et al. Citation2006, Hoover Citation2006, Polak Citation2007) and distance from the nest to the land and open water edges (Batáry et al. Citation2004, Albrecht et al. Citation2006, Schiegg et al. Citation2007). Nest concealment depends mostly on the structure of the vegetation in the nest vicinity and influences the probability of nest detection by both avian and mammalian predators, whereas nest placement – its distance from the land-water edge and the water depth around the nest – seems to be most important for protecting against terrestrial mammals (Sládeček et al. Citation2014). Additional factors, for example, nest distance to the nearest bushes or trees, placement of the nest above the water level and size of the nest, may also be important for predation risk (López-Iborra et al. Citation2004, Pasinelli & Schiegg Citation2006, Musilová et al. Citation2014).
Numerous investigations attempting to determine the factors influencing nest success in wetlands have been performed mainly at large reservoirs (usually over 25 ha) such as lakes (Salonen & Penttinen Citation1988, Kristiansenl Citation1998, Schiegg et al. Citation2007), fish ponds (Honza et al. Citation1998, Polak Citation2007) or bogs (Berg et al. Citation1992), whereas studies conducted at small water bodies are scarce (Musilová et al. Citation2014). Ponds, which are defined as permanent or seasonal water bodies with an area of up to 5 ha (Céréghino et al. Citation2008), are characterized by shallower water, different vegetation zonation (some of them may be even entirely overgrown) and narrower littoral zones compared to lakes and larger reservoirs (Søndergaard et al. Citation2005, Gamrat Citation2009). In this study, we tested whether the main habitat variables responsible for nest predation at large reservoirs (water depth, nest concealment and proximity to the edge) are also important in determining the nest fate of waterbirds nesting at small midfield ponds. We hypothesize that due to the very narrow strip of emergent vegetation and small stratification of water depth in the littoral zone, distances between the nest and the land and open water, as well as water depth at the nesting site, are not the main environmental features that affect nest success at small ponds, instead this is likely to be nest concealment.
To test our predictions, two Rallidae species were chosen as representatives of marsh nesting species – the Little Crake Porzana parva and the Water Rail Rallus aquaticus, for which midfield ponds are important breeding habitats (Jedlikowski et al. Citation2014). Both species have laterally flattened bodies and are highly adapted for moving through dense emergent vegetation (Taylor & van Perlo Citation1998). Vegetation density as well as water depth are important parameters for these species when deciding on nest site selection (Jedlikowski et al. Citation2014). Because the activity of birds may highly affect nest conspicuousness and so nest predation rates (Martin et al. Citation2000, Schmidt & Whelan Citation2005), and parents may alter their behaviour to compensate for the nest predation risk (Cresswell Citation1997), the significance of particular habitat variables may be masked. For this reason we also performed an artificial nest experiment.
Finally, this study enabled us to estimate nest success and identify the nest predators of the Little Crake and Water Rail. Until now, only a few studies have focused on the nest survival rates of the Water Rail (Jenkins Citation1999, De Kroon Citation2006) and no data are currently available on the nest success of the Little Crake and their nest predators.
METHODS
Study area
We conducted the study in the central part of the Mazurian Lakeland of north-eastern Poland. This post-glacial region, with an entire area of about 13 180 km2, contains about 900 lakes (Choiński Citation1991) and nearly 84 000 natural ponds smaller than 1 ha (Solarski & Nowicki Citation1993). The inland ponds and small lakes, surrounded by arable fields, meadows, pastures and fallows, are the key elements in the agricultural landscape of this region. They perform manifold ecological functions, such as water reservoirs, corridors between larger lakes and refuges for many freshwater plant and animal species (Surmacki Citation1998, Scheffer et al. Citation2006). Changes in agricultural practices in last decades (more extensive land use after the State Agricultural Farms collapsed in the 1990s) resulted in the recovery of numerous small midfield water bodies that had been previously drained.
We collected data at 26 unnamed water bodies distributed near Lake Łuknajno between 53°47ʹ–53°˚53ʹ N and 21°33ʹ–21°44ʹ E (). Their area did not exceed 6 ha (range 0.18–5.75 ha, mean 1.93 ha). The maximum water depth (measured at winter) ranged between 40 and 130 cm, water acidity pH ranged from 6.3 to 8.2. All water bodies were located in natural depressions filled with organic sediments, mainly peat. Most of them were overgrown by typical hydrophytes, such as Bulrush Typha latifolia and Common Reed Phragmites australis, some of them also by Sedges Carex spp. and Grey Willow Salix cinerea.
Figure 1. Distribution of midfield ponds in the study area of the Mazurian Lakeland, NE Poland. White circles – ponds with the Little Crake nests, black circles – ponds with the Water Rail nests, grey circles – ponds with artificial nest. Circles with more than one colour represent ponds where nests of both species were found or where artificial nests were placed.
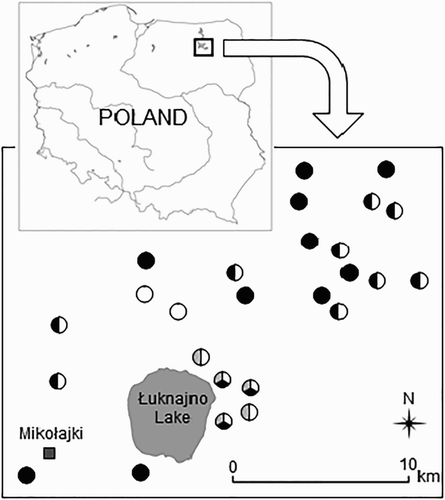
Sampling of natural nests
We carried out the fieldwork from late April to early August in 2012–2014 over three successive breeding seasons. Natural nests of the Little Crake and Water Rail were systematically and carefully searched for in the littoral vegetation after using call playback of both species (Jedlikowski et al. Citation2014). We found 70 Little Crake nests (19 in 2012, 36 in 2013 and 15 in 2014) at 16 water bodies and 62 Water Rail nests (12 in 2012, 18 in 2013 and 32 in 2014) at 22 water bodies. The position of the nests was precisely marked using a handheld GPS receiver (Topcon GRS-1; accuracy less than 0.5 m). We performed the first control of each nest two days after finding it to assess the stage of the clutch (egg laying, incubating or hatching stage), and age of eggs (using the egg flotation technique; Rush et al. Citation2007) in nests found with a complete clutch. Afterwards, each nest was checked at 5–7 days intervals until the clutch successfully hatched (at least one chick was observed) or depredated (even partial depredation resulted in nest abandonment). Crushed pieces of shells with remains of yolk found in the nest or close by in the water were evidence of egg loss. Another definite determinant of nest failure was a too short time span from the first day of egg laying to hatching date (less than 20 days for both species). To determine the species responsible for nest depredation, we used camera traps (model HCO ScoutGuard SG580M and LTL Acorn 6210 MM), which were installed and camouflaged at about 1-m distance from the nest. Camera traps were distributed randomly among the available nests and monitored 16 Water Rail nests (of which 7 were depredated) and 21 Little Crake nests (of which 3 were depredated) during the whole study period. Because batteries were the sole power source of the camera traps, continuous video surveillance was not possible, and therefore the act of nest predation was not always recorded. Marks left on shells by predators identified using a camera trap, served as comparative material and, together with keys found in the literature (Green et al. Citation1987, Opermanis et al. Citation2001), helped to identify those predators which robbed the nests but were not filmed. We distinguished mainly between marks left by Marsh Harriers Circus aeruginosus (see A and 2B) and predatory mammals (see C and 2D), which differed significantly and were clearly identified. We did not assign marks left by mammals to particular species because patterns of egg breakage may overlap among species (Lariviere Citation1999). When proper identification of predators was impossible (e.g. eggshells were excessively damaged or marks on eggshells indicated more than one potential predator), we assigned the predatory act to an ‘unknown predator’.
Figure 2. Marks left on eggshells by known predators (based on nests monitored by camera traps). Egg of the Little Crake (A) depredated by the Marsh Harrier, with thin, horizontal V-shaped groove. Egg of the Water Rail (B) with a deep V-shaped grooves, and specific small hole on the adverse side of the egg made by the Marsh Harrier's tip of the upper mandible (arrows). Egg of the Water Rail depredated by the Raccoon Dog (C) and by the Least Weasel (D), with rounded holes and heavily cut edges (photos by J.J.).
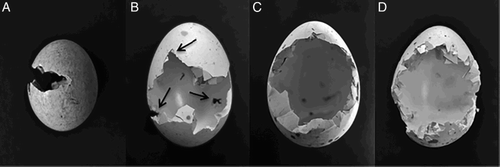
Artificial nest experiment
We conducted the artificial nest experiment in May in 2012 and 2013. Each year, we used 25 nests resembling the natural nests of the Little Crake and 25 nests resembling nests of the Water Rail. All artificial nests were placed at 5 water bodies (with a total area of 21.2 ha and total shoreline length of 8.5 km), where natural nests were also found (69% of Little Crake nests and 16% of Water Rail nests). Artificial nests were placed in the littoral zone, in places that resembled natural nest sites, according to the following rules: (a) nests of Little Crake were placed at deeper water level (above 30 cm, mainly about 50–60 cm) than nests of the Water Rail (below 30 cm); (b) distance between neighbouring nests was 100 m (measured along the shoreline); (c) nests of the Little Crake were placed alternately with nests of the Water Rail. The 100 m interval was maintained so as to avoid a potential density-dependence increase in the nest predation rate (Gunnarsson & Elmberg Citation2008). Artificial nests were situated regardless of distribution of natural nests. All nests were hidden in a clump of vegetation to simulate how natural nests are hidden. Artificial nests were left in place for three weeks and checked every seven days. Nests were removed after an act of predation (even partial predation of eggs) or after the three-week period (which was considered nest success).
Artificial nests were constructed using small wicker baskets with an external diameter of 11 cm, which was an average of 2 cm less than natural Little Crake nests, and 5 cm less than natural Water Rail nests (Jedlikowski, unpubl. data). Wicker baskets were attached to the top of stakes, which were driven into the ground at the bottom of the water body. All nests contained three quail Coturnix coturnix eggs, which are similar in size to Little Crake and Water Rail eggs. In 2013, one of the three quail eggs was emptied and filled with wax, which was helpful in identifying marks left by predators on the shells. To simulate the natural pigmentation of rails eggs, we selected the darkest quail eggs similar to the buff-brown eggs of Little Crake, while the others were whitened (using vinegar) to be more similar to the pinkish-cream eggs of the Water Rail. Eggs were left to dry for a few days to dissipate the vinegar odour. We identified the group of species responsible for nest depredation in the same way as done for the predators of natural nests. Ten artificial nests were also monitored by camera traps (five Little Crake nests and five Water Rail nests).
Vegetation measurements
At each of the natural and artificial nests, we recorded several habitat variables of the nest location at the pond and closely surrounding vegetation: (1) distance from the nest to the shore (land-water edge, measured with 1 m precision); (2) distance from the nest to open water (measured with 1 m precision); (3) mean water depth within a 3-m radius plot (average value from ten randomly selected points within the plot, measured to the nearest 1 cm); (4) proportional area covered by emergent vegetation within a 3-m radius around the nest (estimated with 5% precision); (5) height of emergent vegetation above the nest rim (estimated with 5 cm precision). All measurements were taken on the same day that the natural nests were found or on the initial day of placing the artificial nests. Average values of habitat variables taken at successful and depredated nests are summarized in .
Table 1. Habitat variables measured at successful and depredated Little Crake and Water Rail nests (mean ± se).
Data analysis
Nest survival models
We used the MARK Program (version 8.0) to develop nest survival models as a function of multiple covariates (White & Burnham Citation1999, Dinsmore & Dinsmore Citation2007). This program uses generalized linear models with a user-specified link function and error distribution to generate maximum likelihood estimates of regression coefficients and their sampling variances and covariances (we used the logit link function and binomial error distribution). We selected the best approximating model for inference using the Akaike information criterion for a small sample size (AICc; Burnham & Anderson Citation2002). The model with the lowest AICc value was considered the best and all models within 2.0 AIC units of that model were considered competitive. The MARK Program requires some basic input of information for each nest to generate its encounter history: (1) the day the nest was found; (2) the last day the nest was checked and found to be intact; (3) the last day the nest was checked; (4) the fate of the nest. For successful nests, we considered the last day the nest was checked to be the date of the last observation of an undepredated clutch. To properly model the data, we excluded from further analysis those nests which were found already depredated at the first check (six Little Crake nests and ten Water Rail nests) and those where chicks had already hatched (one Little Crake nest). Furthermore, we standardized breeding season dates (for three years in the case of natural nests and two years in the case of artificial nests) using the earliest location date for any year as the first day of the season, and the latest hatching or failure date in any year as the last day of the season. Thus, we defined a 92-day long breeding season for the Little Crake (from 5 May to 4 August) and a 95-day long breeding season for the Water Rail (from 24 April to 27 July). For artificial nests, we defined a 28-day exposure period (from 8 May to 4 June).
We began modelling by considering the potential interactions between habitat variables and bird species. We built two full models (one for natural nests and one for artificial nests) with five habitat covariates (water depth, vegetation density, vegetation height, distance to the land-water edge and distance to open water) and their interactions with species. Then we used the likelihood ratio test to check whether models without the interactions differed from the full model. We found a significant interaction with vegetation density for natural nests by species, but no interactions were significant for artificial nests. As a result, we decided to further analyse the natural Little Crake and Water Rail nests separately, and place all artificial nests into one group to analyse them together.
We performed a two-stage procedure in our analysis to identify a reasonably sized set of models which had the best fit (with ΔAICc < 2). In the first stage, we carried out an initial analysis to find the best model which describes nest survival during season and between years. These models included one of three within-season temporal variations in nest survival (constant, linear or quadratic) and combinations with year effect. In the second stage, we retained only the variables from the top models (year covariate for all three model sets with constant nest survival for Little Crake, quadratic nest survival for Water Rail and linear nest survival for artificial nests) and added three habitat variables describing location (water depth, distance from the nest to the land-water edge and to open water) and two habitat variables describing nest concealment in the littoral vegetation (vegetation height and vegetation density). We considered all possible models with fixed variables from the first stage. All tested models are reported in the Supplementary Online Appendix. Finally, the best models, with ΔAICc < 2, were averaged according to the multi-model interference approach and relative importance of habitat variables was calculated (Arnold Citation2010).
Nest success
To compare daily survival rate (DSR) between two rallid species we took variables from the top models for the Little Crake and Water Rail (constant time trend, year, vegetation density, distance to the land-water edge and distance to open water) and built one full model with an additional species covariate. We used a likelihood ratio test to check whether the model without species as a factor differed from the full model.
We used the DSR estimated from the best model for natural Water Rail and Little Crake nests to calculate the probability that the nests would survive the incubation period (from the first day of egg incubation to the first day of hatching) as well as the whole nesting period (from the day of egg laying to the first day of hatching). According to data for the nests found at initiation day, the average nesting period was 24 days for the Little Crake (range 23–25, n = 14) and 28 days for the Water Rail (range 25–30, n = 7). The incubation period was thus estimated as 19 days for the Little Crake (we assumed that birds started incubation after laying the 5th egg; own obs.; Dittberner & Dittberner Citation1990) and 20 days for the Water Rail (incubation started with a full clutch; Vincent Citation1937).
In the case of the Little Crake, for which the model indicated a constant DSR, we estimated nest survival as DSRd, where d is the average length of incubating/nesting period (Dinsmore et al. Citation2002). Variance was estimated with the delta method (Powell Citation2007). In the case of the Water Rail, DSR varied temporally during the standardized breeding season, so the nest survival was calculated as the average of predicted nest survival for each nest using a similar method as the one used in Stauffer et al. (Citation2011). Accordingly, we first assigned a natural nest initiation date and first day of incubation period to the appropriate day of the corresponding, standardized breeding season. For nests found after initiation day, we estimated this using a backdating method, assuming that the birds lay one egg per day and knowing the average length of the incubation period. Secondly, we estimated the expected number of nests initiated on the same date as each observed nest. We used Horvitz-Thompson estimator as , where
was the probability that the ith nest would survive long enough to be found. We calculated
by taking the product of model DSRs from the known or predicted date of nest initiation up until we found the nest. For all nests found on initiation day,
was assumed as 1. Nest survival (NS) was than estimated as
where
was the predicted nest survival for the ith nest, calculated as the product of appropriate number of consecutive model averaged DSRs (for the nesting or incubation period), beginning from the date of nest initiation (nest survival) or first day of egg incubation (incubation survival) for the ith nest. Because the delta method (Powell Citation2007) does not provide a good estimate of variance for predicting nest survival when DSR varies through time (Dinsmore et al. Citation2002), we calculated only between-nest variation using an approximate standard error (se) of the weighted mean as
. The 95% confidence intervals were estimated as
, where
RESULTS
Nest predation
Chicks hatched in 41 of 70 Little Crake nests (59%) and 21 of 62 Water Rail nests (34%). Among the unsuccessful nests, clutches were depredated more often during incubation (55% of Little Crake nests and 30% of Water Rail nests), than during egg laying (4% and 22%, respectively). The stage when the clutch was depredated was unknown for 17% of Little Crake nests and 24% of Water Rail nests. According to estimates of the maximum likelihood the Little Crake was characterized by 26% higher nest survival during incubation and 35% higher nest survival during the whole nesting period than the Water Rail (), and both differences were significant (χ2 = 6.8, df = 1, P < 0.01; χ2 = 15.9, df = 1, P < 0.001).The main cause of natural nest failure was predation by the Marsh Harrier (). We identified this raptor at one Little Crake nest and two Water Rail nests monitored by camera traps. At other unsuccessful nests (where no video films of predation were obtained) marks of the Marsh Harrier beak were identified on eggshells in 13 Little Crake nests and 20 Water Rail nests. At one Water Rail nest, we filmed the predation of the Least Weasel Mustela nivalis, and at another nest, of the Raccoon Dog Nyctereutes procyonoides. We also assigned ten more Water Rail nests and one Little Crake nest as having been depredated by unidentified mammals (based on eggshell marks). Additionally two Little Crake nests failed due to flooding. The cause of clutch loss was not identified in 12 Little Crake nests and 7 Water Rail nests because only small pieces of crushed eggshells remained, which did not allow the predator to be reliably distinguished.
Figure 3. The causes of Water Rail (white bars, n = 41) and Little Crake (grey bars, n = 29) nest failures. Presented data include both predators identified by camera traps and by marks left on eggshells.
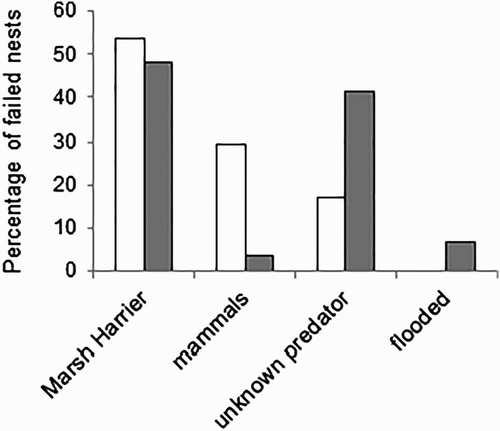
Table 2. Maximum likelihood estimates of nest survival (NS) during standardized incubation and nesting periods for natural Little Crake and Water Rail nests.
In the case of the artificial nest experiment, only 20% of nests survived the entire exposure period (9 nests resembling Little Crake nests and 11 nests resembling Water Rail nests). The main nest predator was the Marsh Harrier, which depredated 55% of unsuccessful nests (at two nests, the Marsh Harrier was recorded by camera traps and for the remaining nests, it was identified by the marks left on eggshells). The rest of the unsuccessful nests were severely damaged (sometimes we found only a stake without the wicker baskets) and usually without eggs, which precluded us from identifying the nest predator.
Nest survival models
The top-ranked MARK models for natural Little Crake nests are presented in . There was no strongly supported model, as eight models were within two AICc units. Model averaging indicated that DSR increased with decreasing distance to the land-water edge (see and ). The effect of other covariates on DSR (negative effects of distance to open water, vegetation height and vegetation density, and positive effect of water depth) was weak, because 95% confidence intervals of all these covariates overlapped zero. According to the best model, DSR was constant during breeding season (DSR = 0.976, se = 0.006, CI = 0.962–0.985). The comparison between years indicated that nest success was highest in 2012, 0.781 (se = 0.111, 95% CI = 0.591–1.032) and lowest in 2014, 0.419 (se = 0.149, 95% CI = 0.213–0.825).
Figure 4. DSR (solid line) and 95% confidence intervals (dashed lines) in relation to: (A) the distance of Little Crake nests to the land-water edge, (B) vegetation density in the vicinity of Water Rail nests and (C) vegetation density in the vicinity of artificial nests. All survival rates were predicted according to the top models; curves in (B) and (C) were generated from model averaged DSR values.
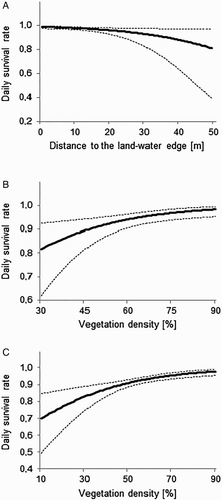
Table 3. Summary of model selection results of the DSR for natural nests of the Little Crake, Water Rail and artificial nests. Models are compared according to Akaike's information criterion corrected for small sample size (AICc), the difference in AICc from the best supported model (ΔAICc), model weight (wi), number of parameters (k) and model deviance. Only models with ΔAICc < 2 are presented.
Table 4. Model averaged parameter (based on models with ΔAICc < 2) and cumulative weights (from all possible models) of predictors from models of nest survival of the Little Crake, Water Rail and artificial nests. Covariates ranked according to cumulative weights.
The best approximating MARK models for natural Water Rail nests are presented in . Model averaging showed that DSR was positively affected by vegetation density (see and ). Moreover, nest survival was positively related to distance to open water and water depth, and negatively related to distance to the land-water edge; however, the effect of these covariates was weak (95% confidence intervals of these covariates overlapped zero). According to the best model, DSR was lowest at the beginning of the breeding season (DSR = 0.852, se = 0.071, CI = 0.655–0.946), then increased to achieve the highest values in the first half of June (DSR = 0.968, se = 0.009, CI = 0.943–0.982), and started to decrease to the end of July. DSR was also strongly influenced by year covariate and based on the top-ranked model, varied from 0.086 (se = 0.036, 95% CI = 0.040–0.188) in 2014 to 0.641 (se = 0.031, 95% CI = 0.583–0.704) in 2013. A likelihood ratio test indicated also that DSR of the Water Rail was significantly lower than that of the Little Crake (χ2 = 5.7, df = 1, P = 0.017).
The most relevant models for artificial nests are presented in . Model averaging showed that DSR was higher for nests in denser vegetation (see and ). Top-ranked models also suggest a negative effect of vegetation height and a positive effect of distance to the land-water edge and to open water on DSR, though the effect was weak in all cases (95% confidence intervals of these covariates overlapped zero).
DISCUSSION
In wetlands, as in other habitats, nest survival generally depends on the proper choice of nesting site by birds. Aside from good protection against random abiotic events, such as heavy winds, rains, floods, etc., a well selected nesting site must prevent brood predation, which is considered to be the main factor affecting nest success in birds. The results of our study showed that at midfield ponds, the natural nests of two rallid species and artificial nests were depredated mostly by one avian predator – the Marsh Harrier. This fact explains why good nest concealement increased the survival rates of the Water Rail and artificial nests. Nests situated in denser littoral vegetation were probably more difficult for the Marsh Harrier to detect. Opermanis (Citation2004) obtained a similar result, showing that artificial nests covered with nest material were less often depredated by this raptor than nests with openly visible eggs. Reduction of nest visibility also improved the nest survival of the Great Bittern Botaurus stellaris (Polak Citation2007), the Common Pochard Aythya ferina (Albrecht et al. Citation2006) and the Great Reed Warbler Acrocephalus arundinaceus (Batáry & Báldi Citation2005). Thus, this factor seems to be important for many different bird species that nest in wetlands. Nevertheless, the Water Rail, which nested in denser littoral vegetation than the Little Crake, had lower nest success. This inconsistency may result from the fact that Water Rail nests were more often depredated by mammals than Little Crake nests. Mammals use olfactory cues to find their prey, therefore, vegetation cover does not protect Water Rail nests against these predators, because they are placed over shallow water close to the bank.
Contrary to the Water Rail, nest concealment was not found to affect the Little Crake. The most important factor affecting the survival of Little Crake nests was distance to the land-water edge – (nests situated closer to the shore had lower predation rates). This result does not support other findings that nests situated closer to littoral zone edges suffer higher predation than those placed in the reed bed interior (Batáry et al. Citation2004, Báldi & Batary Citation2005). However, the distance from nests to the habitat edges does not always influence nest predation in wetlands (Picman et al. Citation1993). Our finding may be explained by the negative correlation between the distance from the nest to the land-water edge and vegetation density (r = −0.34, P < 0.01), thus, nest sites closer to land were in denser vegetation and so were probably better protected against the Marsh Harrier.
Water depth was not important for the nest survival of either Rallid nor for the artificial nests at midfield ponds. This result is contrary to many previous findings for waterbirds nesting at large water bodies, such as lakes and fish ponds (Polak Citation2007, Austin & Buhl Citation2011). Possibly, the differentiation in water depth at nest sites for the Water Rail was too small to affect nest accessibility by terrestrial predators (water depth below nests ranged from 0 to 36 cm, with a mean of 15.7 cm). For example, water depth below the nest depredated by the Least Weasel was 15 cm, and 27 cm below the nest depredated by the Raccoon Dog. Little Crake nests situated in locations with deeper water than the nests of the Water Rail (mean 45.4 cm) were probably sufficiently protected against terrestrial mammals (though not from semi-aquatic mammals, such as the American Mink). This explains why mammals were less important nest predators for this species than birds, and water depth was not a key factor affecting nest success.
The apparent nest success of artificial nests was lower than the success rate of natural nests because predators could probably find them more easily. They were also found more quickly by the Marsh Harrier than by terrestrial predators, because none of the artificial nest were identified as having been depredated by mammals. Therefore, despite differences in water depth at artificial nest sites, their survival was more dependent on vegetation density.
Our study also provides one of the first quantitative measurements of nest success and nest predators of both Rallids. The 3-year maximum likelihood estimated for Water Rail nest survival (0.21) was much lower than for populations in England (apparent nest success = 0.76, n = 55; Jenkins Citation1999) and in the Netherlands (Mayfield nest success recalculated for a 28-day nesting period = 0.65, n = 37; De Kroon Citation2006). The 3-year maximum likelihood estimated for Little Crake nest success (0.56) was comparable to those recorded for other Porzana species, such as the Spotted Crake Porzana porzana (apparent nest success = 0.52, n = 48; Szabó Citation1969/Citation70) and the Sora Porzana carolina (Mayfield nest success for a 28-day nesting period = 0.53, n = 108; Conway et al. Citation1994). Additionally, the results of our study show that the high variability of nest success between years may explain the differences in the nest survival of Rallids as estimated by different investigators.
Moreover, until now, there were also no reliable data about the nest predators of both Rallids. The main nest predator at our study sites was the Marsh Harrier, which is a common breeding species in Mazurian Lakeland and regularly observed hunting at all studied water bodies (Jedlikowski, unpubl. data). Predation by mammals was recorded less frequently and mainly at natural Water Rail nests. However, eggshells were almost completely destroyed or disappeared in many nests, and these observations suggest that the Least Weasel, Racoon Dog and other carnivores such as the American Mink, Red Fox Vulpes vulpes or European Badger Meles meles, which were abundant in the study area, should be considered as potential nest predators. Documented nest predators of related species – Virginia Rail Rallus limicola include mustelids, the Racoon Procyon lotor and birds from Accipitridae and Troglodytidae families (Conway & Eddleman Citation1994). A few studies also indicate the Brown Rat Rattus norvegicus and the Water Vole Arvicola amphibius as potential Little Crake nest predators (Szabó Citation1969/Citation70, Taylor & Van Perlo Citation1998). In the study area, only the Water Vole was abundant (Brzeziński, unpubl. data), however, the role of this rodent in nest predation was unclear. This species was recorded by camera traps in three Water Rail nests, two Little Crake nests and four artificial nests. Nevertheless, eggs in these nests remained intact, even though Water Voles spent a lot of time resting in the artificial nests.
This research shows that for birds inhabiting small midfield ponds, the most important habitat variables affecting nest predation may differ from those usually reported for waterbirds breeding at larger reservoirs (especially the lack of the impact of water depth). A combination of vegetation cover effect (as a protection against avian predators) and the edge-distance effect (due to mammal access to shallow littoral areas) may play the key roles in nest survival in small wetlands such as ponds.
Supplemental data
Tables showing all models that were tested can be accessed at 10.1080/00063657.2015.1031080.
Supplementary material
Download MS Word (140 KB)ACKNOWLEDGEMENTS
We are grateful to M. Brambilla for advice in data analysis and to two anonymous referees for valuable comments on the manuscript. The study was carried out at the Biological and Chemical Research Centre, University of Warsaw, established within the project co-financed by European Union from the European Regional Development Fund under the Operational Programme Innovative Economy, 2007–2013.
Additional information
Funding
REFERENCES
- Albrecht, T., Horák, D., Kreisinger, J., Weidinger, K., Klvana, P. & Michot, T.C. 2006. Factors determining Pochard nest predation along a wetland gradient. J. Wildlife Manage. 70: 784–791. doi: 10.2193/0022-541X(2006)70[784:FDPNPA]2.0.CO;2
- Arnold, T. 2010. Uninformative parameters and model selection using Akaike's information criterion. J. Wildlife Manage. 74: 1175–1178. doi: 10.1111/j.1937-2817.2010.tb01236.x
- Austin, J.E. & Buhl, D.A. 2011. Nest survival of American Coots relative to grazing, burning, and water depths. Avian Conserv. Ecol. 6: 1.
- Báldi, A. & Batáry, P. 2005. Nest predation in European reedbeds: different losses in edges but similar losses in interiors. Folia Zool. 54: 285–292.
- Batáry, P. & Báldi, A. 2005. Factors affecting the survival of real and artificial great reed warbler's nests. Biologia 60: 215–219.
- Batáry, P., Winkler, H. & Baldi, A. 2004. Experiments with artificial nests on predation in reed habitats. J. Ornithol. 145: 59–63. doi: 10.1007/s10336-003-0010-9
- Berg, Å., Nilsson, S.G. & Boström, U. 1992. Predation on artificial wader nests on large and small bogs along a south-north gradient. Ornis Scand. 23: 13–16. doi: 10.2307/3676421
- Bonesi, L. & Palazon, S. 2007. The American mink in Europe: Status, impacts, and control. Biol. Conserv. 134: 470–483. doi: 10.1016/j.biocon.2006.09.006
- Burnham, K.P. & Anderson, D.R. 2002. Model Selection and Multi-Model Inference: A Practical Information-Theoretic Approach. Springer, New York, NY.
- Céréghino, R., Biggs, J., Oertli, B. & Declerck, S. 2008. The ecology of European ponds: defining the characteristics of a neglected freshwater habitat. Hydrobiologia 597: 1–6. doi: 10.1007/s10750-007-9225-8
- Choiński, A. 1991. Katalog jezior Polski. Cz. II. Pojezierze Mazurskie. Wydawnictwo Naukowe UAM, Poznań.
- Conway, C.J. & Eddleman, W.R. 1994. Virginia rail. In Tacha, T.C. & Braun, C.E. (eds) Migratory Shore and Upland Game Bird Management in North America, 192–206. International Association of Fish and Wildlife Agencies, Washington, DC.
- Conway, C.J., Eddleman, W.R. & Anderson, S.H. 1994. Nesting success and survival of Virginia Rails and Soras. Wilson Bull. 106: 466–473.
- Cresswell, W. 1997. Nest predation: the relative effects of nest characteristics, clutch size and parental behaviour. Anim. Behav. 53: 93–103. doi: 10.1006/anbe.1996.0281
- De Kroon, G.H.J. 2006. Het broedsucces van de Waterral in Nederland. Limosa 79: 13–18.
- Dinsmore, S.J. & Dinsmore, J.J. 2007. Modeling avian nest survival in program MARK. Stud. Avian Biol. 34: 73–83.
- Dinsmore, S.J., White, G.C. & Knopf, F.L. 2002. Advanced techniques for modeling avian nest survival. Ecology 83: 3476–3488. doi: 10.1890/0012-9658(2002)083[3476:ATFMAN]2.0.CO;2
- Dittberner, H. & Dittberner, W. 1990. Öko-ethologische Beobachtungen am Nest der Kleinralle (Porzana parva). Bonner Zoologische Beiträge 41: 27–58.
- Fletcher, R.J. & Koford, R.R. 2004. Consequences of rainfall variation for breeding wetland blackbirds. Can. J. Zool. 82: 1316–1325. doi: 10.1139/z04-107
- Gamrat, R. 2009. Vegetation in small water bodies in the young glacial landscape of West Pomerania. In Łachacz, A. (ed.) Contemporary Problems of Management and Environmental Protection. Vol. 2. Wetlands – their Functions and Protection, 95–105. University of Warmia and Mazury, Olsztyn.
- Gjerdrum, C., Elphick, C.S. & Rubega, M. 2005. Nest site selection and nesting success in saltmarsh breeding sparrows: the importance of nest habitat, timing, and study site differences. Condor 107: 849–862. doi: 10.1650/7723.1
- Green, R.E., Hawell, J. & Johnson, T.H. 1987. Identification of predators of wader eggs from egg remains. Bird Study 34: 87–91. doi: 10.1080/00063658709476940
- Gunnarsson, G. & Elmberg, J. 2008. Density-dependent nest predation – an experiment with simulated Mallard nests in contrasting landscapes. Ibis 150: 259–269. doi: 10.1111/j.1474-919X.2007.00772.x
- Guyn, K.L. & Clark, R.G. 1997. Cover characteristics and success of natural and artificial duck nests. J. Field Ornithol. 68: 33–41.
- Honza, M., Øien, I.J., Moksnes, A. & Røskaft, E. 1998. Survival of Reed Warbler Acrocephalus scirpaceus clutches in relation to nest position. Bird Study 45: 104–108. doi: 10.1080/00063659809461083
- Hoover, J.P. 2006. Water depth influences nest predation for a wetland-dependent bird in fragmented bottomland forests. Biol. Conserv. 127: 37–45. doi: 10.1016/j.biocon.2005.07.017
- Jedlikowski, J., Brambilla, M. & Suska-Malawska, M. 2014. Fine-scale selection of nesting habitat in Little Crake Porzana parva and Water Rail Rallus aquaticus in small ponds. Bird Study 61: 171–181. doi: 10.1080/00063657.2014.904271
- Jenkins, R.K.B. 1999. The breeding biology of the Water Rail Rallus aquaticus in Britain and Ireland. Bird Study 46: 305–308. doi: 10.1080/00063659909461143
- Koons, D.N. & Rotella, J.J. 2003. Comparative nesting success of sympatric Lesser Scaup and Ring-necked Ducks. J. Field Ornithol. 74: 222–229. doi: 10.1648/0273-8570-74.3.222
- Kristiansenl, J.N. 1998. Egg predation in reedbed nesting Greylag Geese Anser anser in Vejlerne, Denmark. Ardea 86: 137–145.
- Lariviere, S. 1999. Reasons why predators cannot be inferred from nest remains. Condor 101: 718–721. doi: 10.2307/1370209
- López-Iborra, G.M., Pinheiro, R.T., Sancho, C. & Martínez, A. 2004. Nest size influences nest predation risk in two coexisting Acrocephalus warblers. Ardea 92: 85–92.
- Martin, T.E., Scott, J. & Menge, C. 2000. Nest predation increases with parental activity: separating nest site and parental activity effects. Proc. R. Soc. Lond. B 267: 2287–2293. doi: 10.1098/rspb.2000.1281
- Musilová, Z., Musil, P., Zouhar, J. & Poláková, S. 2014. Nest survival in the Reed Bunting Emberiza schoeniclus in fragmented wetland habitats: the effect of nest-site selection. Ornis Fenn. 91: 138–148.
- Nilsson, S.G. 1984. The evolution of nest-site selection among hole-nesting birds: the importance of nest predation and competition. Ornis Scand. 15: 167–175. doi: 10.2307/3675958
- Opermanis, O. 2004. Appearance and vulnerability of artificial duck nests to avian predator. J. Avian Biol. 35: 410–415. doi: 10.1111/j.0908-8857.2004.03243.x
- Opermanis, O., Mednis, A. & Bauga, I. 2001. Duck nests and predators: interaction, specialisation and possible management. Wildlife Biol. 7: 87–96.
- Pasinelli, G. & Schiegg, K. 2006. Fragmentation within and between wetland reserves: the importance of spatial scales for nest predation in reed buntings. Ecography 29: 721–732. doi: 10.1111/j.2006.0906-7590.04728.x
- Picman, J. 1988. Experimental study of predation on eggs of ground-nesting birds: effects of habitat and nest distribution. Condor 90: 124–131. doi: 10.2307/1368441
- Picman, J., Milks, M.L. & Leptich, M. 1993. Patterns of predation on passerine nests in marshes: effects of water depth and distance from edge. Auk 110: 89–94. doi: 10.2307/4088408
- Polak, M. 2007. Nest-site selection and nest predation in the Great Bittern Botaurus stellaris population in eastern Poland. Ardea 95: 31–38. doi: 10.5253/078.095.0104
- Powell, L.A. 2007. Approximating variance of demographic parameters using the delta method: a reference for avian biologists. Condor 109: 949–954. doi: 10.1650/0010-5422(2007)109[949:AVODPU]2.0.CO;2
- Ricklefs, R.E. 1969. An analysis of nesting mortality in birds. Smithson Contrib. Zool. 9: 1–48. doi: 10.5479/si.00810282.9
- Robertson, R.J. 1972. Optimal niche space of the redwinged blackbird (Agelaius phoeniceus). I. Nesting success in marsh and upland habitat. Can. J. Zool. 50: 247–263. doi: 10.1139/z72-036
- Rush, S.A., Cooper, R.J. & Woodrey, M.S. 2007. A nondestructive method for estimating the age of Clapper Rail eggs. J. Field Ornithol. 78: 407–410. doi: 10.1111/j.1557-9263.2007.00131.x
- Rush, S.A., Woodrey, M.S. & Cooper, R.J. 2010. Variation in the nesting habits of Clapper Rails in tidal marshes of the northern Gulf of Mexico. Condor 112: 356–362. doi: 10.1525/cond.2010.090078
- Salonen, V. & Penttinen, A. 1988. Factors affecting nest predation in the Great Crested Grebe: field observations, experiments and their statistical analysis. Ornis Fenn. 65: 13–20.
- Sargeant, A.B. & Raveling, D.G. 1992. Mortality during the breeding season. In Batt, B.D.J., Afton, A.D., Anderson, M.G., Ankney, C.D., Johnson, D.H., Kadlec, J.A. & Krapu, G.L. (eds) The Ecology and Management of Breeding Waterfowl, 396–422. University of Minnesota Press, Minneapolis.
- Scheffer, M., Van Geest, G.J., Zimmer, K., Jeppesen, E., Søndergaard, M., Butler, M.G., Hanson, M.A., Declerck, S. & De Meester, L. 2006. Small habitat size and isolation can promote species richness: second-order effects on biodiversity in shallow lakes and ponds. Oikos 112: 227–231. doi: 10.1111/j.0030-1299.2006.14145.x
- Schiegg, K., Eger, M. & Pasinelli, G. 2007. Nest predation in Reed Buntings Emberiza schoeniclus: an experimental study. Ibis 149: 365–373. doi: 10.1111/j.1474-919X.2007.00654.x
- Schmidt, K.A. & Whelan, C.J. 2005. Quantifying male Wood Thrush nest-attendance and its relationship to nest success. Condor 107: 138–144. doi: 10.1650/7582
- Sládeček, M., Kubelka, V., Mlíkovský, J. & Šálek, M. 2014. Coping with nest predation risk in a species-rich bird community inhabiting a Siberian wetland. Folia Zool. 63: 256–268.
- Solarski, H. & Nowicki, Z. 1993. Hydrologiczne i ekologiczne znaczenie oczek wodnych i mokradeł na Pojezierzu Mazurskim. Zeszyty Naukowe Komitetu “Człowiek i Środowisko” 6: 175–180.
- Søndergaard, M., Jeppesen, E. & Jensen, J.P. 2005. Pond or lake: does it make any difference? Archiv Für Hydrobiologie 162: 143–165. doi: 10.1127/0003-9136/2005/0162-0143
- Stauffer, G.E., Diefenbach, D.R., Marshall, M.R. & Brauning, D.W. 2011. Nest success of grassland sparrows on reclaimed surface mines. J. Wildlife Manage. 75: 548–557. doi: 10.1002/jwmg.70
- Surmacki, A. 1998. Breeding avifauna of small mid-field ponds in north-western Poland. Acta Ornithol. 33: 149–157.
- Szabó, L.V. 1969/70. Vergleichende Untersuchungen der Brutverhaltnisse der drei Porzana-Arten in Ungarn. Aquila 76–77: 73–115.
- Taylor, P.B. & van Perlo, B. 1998. Rails: A Guide to the Rails, Crakes, Gallinules and Coots of the World. Pica Press, Sussex.
- Vincent, J. 1937. Incubation period of Water Rail. Br. Birds 31: 62.
- Weller, M.W. 1999. Wetland Birds. Habitat Resources and Conservation Implications. Cambridge University Press, Cambridge.
- White, G.C. & Burnham, K.P. 1999. Program MARK: Survival estimation from populations of marked animals. Bird Study 46: 120–138. doi: 10.1080/00063659909477239
- Zduniak, P. 2006. The prey of hooded crow (Corvus cornix L.) in wetland: study of damaged egg shells of birds. Pol. J. Ecol. 54: 491–498.