ABSTRACT
1. This review explores current and proposed on-farm interventions and assess the potential of these interventions against Campylobacter spp.
2. Interventions such as vaccination, feed/water-additives and, most importantly, consistent biosecurity, exhibit potential for the effective control of this pathogen and its dissemination within the food chain.
3. Due to the extensive diversity in the Campylobacter spp. genome and surface-expressed proteins, vaccination of poultry is not yet regarded as a completely effective strategy.
4. The acidification of drinking water through the addition of organic acids has been reported to decrease the risk of Campylobacter spp. colonisation in broiler flocks. Whilst this treatment alone will not completely protect birds, use of water acidification in combination with in-feed measures to further reduce the level of Campylobacter spp. colonisation in poultry may be an option meriting further exploration.
5. The use of varied types of feed supplements to reduce the intestinal population and shedding rate of Campylobacter spp. in poultry is an area of growing interest in the poultry industry. Such supplements include pro – and pre-biotics, organic acids, bacteriocins and bacteriophage, which may be added to feed and water.
6. From the literature, it is clear that a distinct, albeit not unexpected, difference between the performance of in-feed interventions exists when examined in vitro compared to those determined in in vivo studies. It is much more likely that pooling some of the discussed approaches in the in-feed tool kit will provide an answer.
7. Whilst on-farm biosecurity is essential to maintain a healthy flock and reduce disease transmission, even the most stringent biosecurity measures may not have sufficient, consistent and predictable effects in controlling Campylobacter spp. Furthermore, the combination of varied dietary approaches and improved biosecurity measures may synergistically improve control.
Introduction
Campylobacter spp. are Gram-negative, thermophilic, non-spore-forming bacteria that are visible under the microscope as slender, helical or curved rods. It generally colonises the caeca of avian species as a commensal microorganism, with broiler chickens being a particular vector of concern (Hermans et al. Citation2011a; Natsos et al. Citation2019). While most Campylobacter spp. carriage is asymptomatic in the broiler host, infection in some individuals can lead to damage of the gastrointestinal lining, causing diarrhoea (Humphrey et al. Citation2014; Sahin et al. Citation2015). In humans, Campylobacter spp. infection causes an acute form of enteritis known as campylobacteriosis, while more serious sequalae include Guillain-Barré syndrome, reactive arthritis and Miller-Fisher syndrome (Hermans et al. Citation2011a; Pedersen et al. Citation2018).
The incidence and prevalence of human campylobacteriosis has increased in both developed and developing countries over the last 10 years. Compared to other bacteria, Campylobacter spp. is less known for outbreaks, and more for large numbers of sporadic individual cases. However, outbreaks caused by Campylobacter spp. do exist, with 4,936 outbreaks recorded by the Centres for Disease Control and Prevention in the United States between 1999 and 2008 (Batz et al. Citation2012).
The overall economic burden of campylobacteriosis is quite substantial (EFSA Citation2011). In the UK, the median estimated cost to patients and the health service during the 2008–2009 period was £50 million (95% CI: £33-£75 m). The additional cost of related Guillain-Barré syndrome hospitalisations were estimated at £1.26 million (Tam and O’Brien Citation2016). The annual costs of campylobacteriosis in the United States (Hoffmann et al. Citation2012) and in the EU (EFSA Citation2014; Bolton Citation2015) are quite high, estimated to be in the region of 2.9 USD billion and €2.4 billion, respectively.
Campylobacter spp. enter the food chain through poultry colonisation at the farm level. The dose of Campylobacter spp. required to colonise chicks and chickens can be very low, but, once established, populations within the caeca can rapidly reach high levels (between 105 and 109 CFU/g; Woodall et al. Citation2005; Stern Citation2008; Newell et al. Citation2011; Taha-Abdelaziz et al. Citation2018a). Because chickens remain colonised until slaughter, this almost inevitably results in carcase contamination during processing, which can, in turn, allow pathogen transmission to humans (Allen et al. Citation2008; Stern Citation2008; Jorgensen et al. Citation2011; Ridley et al. Citation2011; Hermans et al. Citation2012; Sahin et al. Citation2015).
There is little Campylobacter spp. genotype homogeneity, with genetic transfer playing an important role in the development of novel genotypes within a short space of time. High competency species, including C. jejuni, are capable of utilising external DNA, such as the virulence plasmid pVir, DNA acquired from the environment or obtained through transformation, to modify the cell genotype. As such, it is difficult to characterise Campylobacter spp. by genotype (Burnham and Hendrixson Citation2018). Further complicating this is phase variation in Campylobacter spp. gene expression, with over 30 genes differentially regulated in response to the external environment. This is vital as part of the adaptive process when Campylobacter spp. isolates from an avian reservoir, for example, the broiler caecum, and enter a new environment, such as the meat processor plant. Phase variation is particularly effective with regard to modulating externally expressed structures, such as lipooligosaccharide, capsular polysaccharide and flagellin, as well as altering cell motility (Burnham and Hendrixson Citation2018). Regulation of the stator protein gene motA in response to environmentally-induced c-di-GMP reduces flagellar stability and alters a cell’s ability to move (Wirebrand et al. Citation2018; Burnham and Hendrixson Citation2018). This altered genotype may become more adept at surviving external stresses, such as those within human consumers or the external environment.
There have been attempts to circumvent this genotypic variation and classify C. jejuni according to alleles of highly conserved genes through Multilocus Sequence Typing (MLST). The MLST has allowed researchers to trace the occurrence of live-bird associated C. jejuni sequence types (STs) from the farm level through the slaughter and manufacturing process to the end-product. These studies have revealed that the majority of C. jejuni STs found in end-product meat are indistinguishable from those associated with live birds (Colles et al. Citation2010; Hastings et al. Citation2011). MLST studies allow for further analysis of flock characteristics and handling. Certain STs, for example, ST418 and ST 4227, are easily removed through washing and decontamination within the transport process. Some STs, such as ST-45, are associated with the caecal microbiome, and show resilience when exposed to decontamination stresses, such as disinfectant (Hastings et al. Citation2011). These resilient STs represent the most difficult to eradicate and are the most environmentally tolerant within the production cycle, and represent the worst-case scenario at a farm level, which may necessitate extra precautions or decontamination steps.
Many risk factors contribute to Campylobacter spp. colonisation of broiler flocks, indicating the difficulties in maintaining effective countermeasures against its entry into the broiler environment (Hermans et al. Citation2012; Natsos et al. Citation2018). In general, studies have indicated that horizontal transmission from environmental sources is the most significant cause of dissemination in flocks. Vertical transmission remains a controversial source of the microbe. (Bull et al. Citation2006; Callicott et al. Citation2006; Ridley et al. Citation2008; Workman et al. Citation2008; Zweifel et al. Citation2008; Ellis-Iversen et al. Citation2009; Allen et al. Citation2011; Patriarchi et al. Citation2011; Cox et al. Citation2012; Sahin et al. Citation2015). Bull et al. (Citation2006) and Callicott et al. (Citation2006) stated lack of evidence for vertical transmission of Campylobacter spp. in chickens while Cox et al. (Citation2012) believed vertical transmission has been overlooked because of two reasons. One reason is that there is no ideal culturing procedure for recovering and isolating Campylobacter spp. It is difficult to routinely culture from certain types of samples. Another reason is that researchers have not fully accepted the role of the fertile egg in transmission. Most factors commonly associated with Campylobacter spp. colonisation in broiler flocks are farm-based, including: (1) A lack of overall biosecurity on farms; (2) Presence of other animals in close proximity to poultry houses (including other poultry species, livestock, pets, and wildlife); (3) Increasing numbers of houses on a farm; (4) Slaughter age; (5) Size of flocks; (6) Partial depopulation (thinning); (7) Seasonal and climatic changes; (8) Use of ventilators; (9) Fly and insect population; (10) Use of old litter; (11) Farm equipment and (12) Transport vehicles and farm workers (Hald et al. Citation2004; Nichols Citation2005; Hald et al. Citation2008; Hazeleger et al. Citation2008; Stern Citation2008; Horrocks et al. Citation2009; Meerburg Citation2010; Newell et al. Citation2011; Patriarchi et al. Citation2011; Ridley et al. Citation2011; Wagenaar et al. Citation2013; Carron et al. Citation2018; Murphy et al. Citation2018).
Animal stress is an unavoidable aspect of broiler husbandry, and can have damaging effects upon the growth and well-being of the live bird. Common practices within the broiler house and in the lead up to slaughter cause increases in the production of stress-related hormones, such as corticosterone, leading to changes in animal behaviour, including increased pecking at self and others, agitation and defaecation (Scanes Citation2016; Rasschaert et al. Citation2020). Stress-inducing processes include shackling, cooping, transport, fasting/feed restriction and worker interactions, while excess heat, cold, light and movement restriction increase individual and group stress (Scanes Citation2016). Furthermore, corticosterone production, due to stress, is linked to an increase in gastrointestinal permeability. This causes increased defecation due to higher digesta water content and can cause the systemic infection of birds by gastrointestinal bacteria (Scanes Citation2016). This may cause illness in the bird if early in life, as chicks are particularly vulnerable to systemic Campylobacter spp. infection, or may spread to the end product (Humphrey et al. Citation2014; Sahin et al. Citation2015). These effects can have subsequent ramifications for the end-product, with faeces acting as a prominent vector for Campylobacter spp. between adjacent birds, especially in crated environments prior to slaughter, with increased pecking exacerbating this spread (Rasschaert et al. Citation2020).
The prevalence of Campylobacter spp.-positive poultry broiler flocks varies by region, season and production system (Bahrndorff et al. Citation2013; Murphy et al. Citation2018). In the United States, a 2001 survey indicated that nearly 90% of flocks are colonised on-farm (Stern et al. Citation2001). In Europe, the prevalence on-farm broiler flocks varies from 18 to > 90%, with Northern European countries having significantly lower figures than their Southern European counterparts (Newell and Fearnley Citation2003; Hermans et al. Citation2012; Sibanda et al. Citation2018). As contaminated poultry is the primary reservoir for human infections, the Food Safety Authority of Ireland (FSAI), European Food Safety Authority (EFSA), Health Protection Surveillance Centre (HPSC) and World Health Organisation (WHO) are actively seeking effective interventions within the poultry production chain. This is particularly important as antimicrobial-resistant strains of Campylobacter spp. (Murphy et al. Citation2018) add to the economic burden of foodborne disease globally (EFSA and ECDC Citation2017).
Since Campylobacter is frequently found as a commensal organism in the avian caecum, it makes sense to introduce interventions that prevent this colonisation in the first instance. The application of strict biosecurity measures is the most effective method to prevent this colonisation (see ) (Rivoal et al. Citation2005; Nauta et al. Citation2007; Hermans et al. Citation2012; WHO Citation2013). However, biosecurity measures alone will not be sufficient as broiler chickens are at constant risk of contamination (Pattison Citation2001; Sahin et al. Citation2003; Van Gerwe et al. Citation2005; Hermans et al. Citation2011b). Therefore, other promising specific pre-slaughter interventions (e.g. no-thin practices and improved transport hygiene) also must be considered. This literature review explores on-farm primary production practices to reduce the contamination of Campylobacter on poultry flocks.
Figure 1. The Flow chart illustrates steps in chicken production on farms. Red steps indicate points at which Campylobacter spp. can pose a risk. Green steps indicate points at which Campylobacter spp. can be controlled. Orange steps indicate the possibility of risk/control, which depends on behaviour/actions
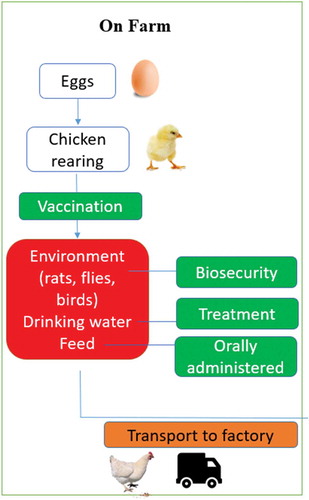
Vaccination
Vaccination of poultry may be considered a logical starting point in the struggle to prevent initial colonisation. Unlike Salmonella spp., for which there exists a broiler vaccine, there is no such facility currently available for Campylobacter spp. (Wagenaar et al. Citation2013; Olofsson Citation2015). Given the extensive diversity in Campylobacter spp. genome and surface-expressed proteins, it is not easy to develop vaccines that can prevent associated illness (Ringoir and Korolik Citation2003; Walker Citation2005). Several Campylobacter spp. target structures have been the focus of in ovo vaccination studies, including DNA, flagellar subunits and liposomes, as well as a whole-cell bacterin suspension. However, these vaccination candidates did not elicit a protective humoral response when used in vivo (Liu et al. Citation2019; Vandeputte et al. Citation2019). Recent promising research (see ) has shown that vaccination of chickens with recombinant Campylobacter spp. peptides can result in reduced C. jejuni caecal carriage compared to a non-vaccinated Campylobacter-challenged group. This indicated broiler protection from C. jejuni colonisation (Rice et al. Citation1997; Widders et al. Citation1998; Wyszyńska et al. Citation2004; Buckley et al. Citation2010; Neal-McKinney et al. Citation2014; Kobierecka et al. Citation2016; Taha-Abdelaziz et al. Citation2018a). Other promising vaccines include an oral candidate which utilises a combination of soluble PLGA-encapsulated oligodeoxynucleotides containing unmethylated CpG motifs and Campylobacter spp. lysate to achieve significant reduction in caecal carriage in both layer and broiler chickens through the induction of an IgG response (Taha-Abdelaziz et al. Citation2018b). However, vaccination of poultry against Campylobacter is not yet regarded as a completely effective strategy (Hermans et al. Citation2011b). Broiler chickens are specially bred for fast growth and often slaughtered between five and six weeks of age. Vaccination of broilers is challenging due to the cost. A study conducted by Bull et al. (Citation2006) found that Campylobacter spp. was rarely isolated from broiler flocks until the birds were at least three weeks old. Maternal antibodies in chicks are considered to have a role in preventing early colonisation of the chick’s caeca. Further work is needed in this area to find a consistently successful vaccine, as well as in the development and establishment of a suitable and robust vaccination policy (Svetoch and Stern Citation2010). Approaches which need to be optimised include time of vaccination (live chick vs. in ovo vaccination), a potential mixture with other poultry vaccines, such as those currently used to prevent Newcastle Disease, Marek Disease, infectious bronchitis and infectious bursitis, as well as the suitability of the vaccination mixture for the Campylobacter spp. challenge in question.
Table 1. Control measures to reduce Campylobacter prevalence and transmission in poultry flocks
Biosecurity
Farm biosecurity is a set of measures designed to protect a property from the entry and spread of pests and disease. It is considered to be the best strategy to prevent the colonisation and spread of Campylobacter spp. across poultry farms (Vandeplas et al. Citation2007; Hermans et al. Citation2011b). Biosecurity on a poultry farm must ultimately encompass appropriate decontamination methods from the point of entry to the poultry house, maintaining set hygiene standards within the house, through to bird removal at the end of the broiler rearing process.
Foot dips, footwear changes, step-over-barriers, hand wash facilities, drinker/feeder hygiene, optimised in-house and bird management (i.e. litter, water, temperature, humidity), poultry house boundary maintenance and traffic management/hygiene all are highlighted as key factors in biosecurity protocols for blocking Campylobacter spp. contamination (Sahin et al. Citation2002; Newell and Fearnley Citation2003; Barrios et al. Citation2006; Workman et al. Citation2008). For terminal hygiene, a five-step guide with appropriate standards and recommendations at each phase has been previously described; (1) dry clean-out; (2) wet clean/wash; (3) disinfection; (4) drying out; and (5) de-infest (Burke Citation2018).
Farmers and personnel move in and around broiler houses, up to 150 times over the lifetime of a flock. This constitutes a significant risk for Campylobacter spp. introduction and spread (Wagenaar et al. Citation2006). The implementation of personnel hygiene and broiler house disinfection protocols have been found to decrease the prevalence of Campylobacter spp. by 50% (Gibbens et al. Citation2001), while effective rodent, wild bird and fly control have all been associated with reduced risks of colonisation (see ) (Hald et al. Citation2001, Citation2007; Allain et al. Citation2014; Skarp et al. Citation2016). For example, in Nordic countries, the use of fly screens as a biosecurity measure is common practice, with 50 to 90% risk reduction achievable when used in combination with strict biosecurity measures (EFSA Citation2011). The introduction of fly-screens resulted in a reduction in Campylobacter spp.-positive flocks in Denmark (Bahrndorff et al. Citation2013), from 41.4% between 2003 and 2005 (before use of fly screens), to 10.3% between 2006 and 2009 (after introduction of fly screens), whereas the prevalence reduction in the control houses (without fly screens) was not significant (from 41.8% to 36.0%, P > 0.05) during the same period. However, the use of fly screens can have a significant negative impact upon ventilation within poultry houses, which may account for the fact that their implementation is not universal.
Another measure which increases Campylobacter spp. contamination on farm is flock thinning. Thinning is the early removal of a proportion of birds, which have reached the correct market weight, to create space for the remaining flock’s continued growth. The thinning process requires the entry of personnel and catching equipment into broiler houses, which can increase the risk of transmission within and between flocks. This leads to the contamination of up to 50% of flocks that were previously Campylobacter spp.-free (Allen et al. Citation2008; Hermans et al. Citation2011b, Citation2012; Skarp et al. Citation2016). In a recent study, Campylobacter spp. prevalence was found to increase to >85% in both high and low performance farms across all seasons at final depopulation, suggesting that infection was introduced during the thinning procedure (Smith et al. Citation2016). During thinning, and prior to slaughter, it is common practice to remove feed for up to 12 hours. This decreases the intestinal contents, and consequently reduces the risk of intestinal rupture during the in-factory evisceration process, thereby decreasing the probability of carcase contamination. Unfortunately, feed withdrawal introduces additional stress and is often associated with increased pecking of contaminated litter and coprophagy, resulting in an increased pathogen load in the intestine of the chickens (Corrier et al. Citation1999; Thompson and Applegate Citation2006).
A ‘zero-thinning’ policy in Iceland has had a positive effect on Campylobacter spp. reduction in broiler flocks, reducing the percentage of positive testing flocks from 40% to 15% per year (Strydom Citation2015). Whilst ‘zero-thinning’ is an option that is being considered by retailers, increasing pressure has been applied by industry partners to allocate additional payments to poultry farmers who ‘zero-thin’ to make up for lower performance due to reduced bird numbers.
Whilst on-farm biosecurity is essential to maintain a healthy flock and reduce disease transmission, even the most stringent biosecurity measures may not have sufficient, consistent, and predictable effects in controlling infection. This is not helped by the fact that assessment of the effectiveness of biosecurity measures in controlling flock prevalence is quite difficult under commercial settings in different production systems, and technique implementation can be cost prohibitive and hard to maintain (Newell et al. Citation2011; Dale et al. Citation2015; English et al. Citation2015). For example, a study conducted on Finnish poultry farms concluded that biosecurity costs approximately €3.55 per bird and added 8% to the total work time on broiler farms (Siekkinen et al. Citation2012). From a farmer’s perspective, biosecurity interventions should be considered an investment, rather than an additional cost to production, that results in reduced numbers of Campylobacter spp.-positive flocks, and, potentially, an overall improvement in bird performance and quality.
Drinking water treatment
While Campylobacter spp.-typing studies showed no evidence that isolates from poultry house water supplies have caused colonisation in individual or sequential flocks (Zimmer et al. Citation2003; Hermans et al. Citation2011b; Jansen et al. Citation2014), the level of infection in poultry may be alleviated by treating drinking water. Various methods have been investigated, including chlorination, acidification, addition of monocarpic acid and probiotic treatments. However, the efficacy and consistency of such treatments varies across studies. Water chlorination (using 0.2–0.4 ppm free chlorine), combined with effective cleaning of the drinking system, was found to reduce the proportion of Campylobacter spp.-positive birds in a flock from 81% to 7% in early studies (Pearson et al. Citation1993). In addition, the treatment gave a 103 to 104-fold reduction in contamination levels in the carcase post slaughter. However, chlorination of flock drinking water (with 2–5 ppm chlorine) in line with commercial production practices in the United States did not result in reduction of Campylobacter spp. in poultry (Stern et al. Citation2002). This may be because, although this organism is sensitive to chlorine treatment when in free suspension, it appears to be more resistant to this treatment when in mixed populations with other organisms, such as protozoa (King et al. Citation1988; Snelling et al. Citation2005; Vieira et al. Citation2015). However, there are different forms of chlorine available, which should be taken into consideration.
The acidification of drinking water through the addition of organic acids has been reported to decrease the risk of colonisation in broiler flocks (see ) (Allain et al. Citation2014; Jansen et al. Citation2014). The addition of lactic acid to drinking water during feed withdrawal has been shown to significantly reduce the incidence of Campylobacter spp. recovered from crop samples, with 62.3% of treated birds being colonised compared with 85.1% in the control groups (Byrd et al. Citation2001). In addition, it was found that permanent application of acidified drinking water did not negatively affect meat quality parameters or animal welfare. Whilst this treatment alone will not completely protect birds (Jansen et al. Citation2014), use of water acidification in combination with in-feed measures to further reduce the level of colonisation in poultry may be an option meriting further exploration.
Feed supplements
The use of various types of feed supplements to reduce the intestinal population and shedding rate of Campylobacter spp. in broilers is an area of growing interest in the poultry industry. Such supplements include pro- and pre-biotics, organic acids, bacteriocins and bacteriophage which may be added to bird feed and water (see ). These will be further discussed below.
Table 2. Feed additives orally administered to reduce Campylobacter prevalence and transmission in poultry flocks
Probiotics
Probiotics have been defined by the World Health Organisation as ‘live microorganisms which, when administered in adequate amounts, confer a health benefit on the host’ (Food and Agricultural Organization of the United Nations and World Health Organization Citation2001). Probiotics work on the basis of setting up effective competitive exclusion (CE) circumstances between microbial species in the birds’ gastrointestinal tract, the focus being to colonise the gut with ‘good’ bacteria while preventing ‘bad’ bacteria from finding a niche in which to grow (Bratz et al. Citation2015; Stef et al. Citation2016; Thomrongsuwannakij et al. Citation2016). A potential mechanism is the production of antagonism between pathogenic and probiotic bacteria, for example, through the secretion of antibacterial substances, adhesion site and receptor occupancy and competing for essential nutrients (Bermudez-Brito et al. Citation2012; Bratz et al. Citation2015). CE has been reported to reduce Salmonella spp. colonisation in chickens and turkeys (Doyle and Erickson Citation2008; Milbradt et al. Citation2014).
There are currently three microbial groups that are authorised as animal feed additives in the EU: lactic acid bacteria (LAB; mainly Enterococcus, Lactobacillus and Bifidobacterium spp.), Bacillus spp., and yeasts of the genus Saccharomyces. The reported effects of probiotics on intestinal Campylobacter spp. load reduction is not consistent and various depending on both the probiotic used and the strains targeted. For example, the administration of Bifidobacterium longum PCB 133 in feed was found to reduce C. jejuni by approximately 1 log in the faeces of experimentally infected chickens (Santini et al. Citation2010). An additional study screened 116 bacterial species, and reported six Bacillus spp. that reduced C. jejuni counts by at least 1 to 2 log in vivo (Arsi et al. Citation2015). Several commercially available dietary treatments, such as PoultryStar®, Lactobutyrin BRC, Biotronic® Top3, Excential Alliin Plus, Excential Butycoat, Adimix® Precision, Anta®Phyt product and Campylostat have been shown to significantly decrease colonisation in live broilers after 14 days of treatment when compared to the control group (Guyard-Nicodème et al. Citation2016). It is important to note that the four dietary treatments giving a mean reduction over 1 log CFU/g (Biotronic® Top3, Adimix® Precision, Anta®Phyt and Campylostat) presented high variability in Campylobacter spp. counts (>1.5 log CFU/g).
While further research is required to generate sufficient data to conclusively affirm probiotics as an effective tool in the control of Campylobacter spp. during poultry rearing, these studies indicated that the use of probiotics in CE trials is promising. Probiotic interventions require the development of probiotic species and strain mixtures that both survive the rigours of feed processing and the host environment, such as low pH, salt and enzymatic stress and temperature fluctuation, and provide a benefit to either the host or end-consumer (Shokryazdan et al. Citation2017). From this, LAB-based interventions may be considered as strong probiotic agents for use against colonisation in broiler chickens. These bacteria form a large part of the natural host microflora within the same caecal niche as Campylobacter spp. LAB are frequently characterised as probiotic candidates, with a number of them afforded ‘generally recognised as safe’ (GRAS) status as food additives. LAB are associated with the production of a broad range of antimicrobial compounds such as bacterocins, for example, lacticin 1317 and nisin, which have been documented as having inhibitory effects upon the growth of Campylobacter spp. in both in vitro and in vivo studies (Lohans et al. Citation2015; Saint-Cyr et al. Citation2016). The use of LAB species within probiotic mixtures can increase the efficacy of the treatment against Campylobacter and other putative pathogens, while reducing negative impacts upon the host.
Prebiotics
Encouraging the growth of beneficial microbes through the provision of specific nutrients (prebiotics) is thought to give additional and synergistic benefits on the control of Campylobacter spp. within the broiler (Hermans et al. Citation2011b; Arsi et al. Citation2015). Several studies have highlighted the benefits of adding prebiotics and probiotics to poultry diets (Guyard-Nicodème et al. Citation2016), although results depend upon the particular probiotic strains and prebiotic used and the target Campylobacter spp. selected. For example, when Bifidobacterium longum PCB 133 was combined with the prebiotic galactooligosaccharide, no noticeable increase in efficacy against Campylobacter spp. was observed (Baffoni et al. Citation2012).
More recent research has focussed on the use of prebiotics as natural antibiotic growth promoter (AGP) replacements. Various mannanoligosaccharide products either used alone or in combination with other ingredients, for example, the probiotic GalliPro and prebiotic TechnoMos have been shown to serve as alternatives to the AGP Neoxyval (Abudabos et al. Citation2015). This is due to the enhancement of broiler performance through improvements in the bird’s intestinal morphology, in addition to the establishment of microbial balance associated with the modulation of intestinal microflora and the inhibition of pathogens. These types of additives could contribute to a reduction in Campylobacter spp. colonisation, with probiotics having been already reported to prevent pathogenic bacteria such as Clostridium perfringens and Salmonella spp. from colonising the gut (Abudabos Citation2013).
Based on the research discussed (), addition of prebiotics to poultry feed generally results in a detectable decrease in Campylobacter spp. populations in the poultry gastrointestinal tract. This indicated that adding prebiotics as feed supplements can potentially limit Campylobacter spp. in the poultry gastrointestinal tract. However, it remains unclear whether these reductions in colonisation will result in reduction of human campylobacteriosis cases. Prebiotics may be a promising strategy for controlling Campylobacter spp. in poultry, but it remains to be determined whether consistent overall reduction can be achieved in commercial operations.
Bacteriocins
Bacteriocins (BCN) are a group of antimicrobial peptides produced by bacteria when present in intensely competitive niches (Cleveland et al. Citation2001). Considerable research effort has been invested to investigate the use of bacteriocins as a food additive for protection against pathogens (Lewus et al. Citation1991; Scannell et al. Citation2000, Citation2001; Deegan et al. Citation2006; Coelho et al. Citation2007; Xie et al. Citation2009; Zacharof and Lovitt Citation2012; Goyal et al. Citation2018; Kimura and Yokoyama Citation2019). As a prophylactic treatment for poultry, significant progress has been made in the development of potent anti-Campylobacter bacteriocins from commensal microbes isolated from the chicken intestinal tract (Lin Citation2009; Zommiti et al. Citation2016). Although some bacteriocins, such as defensins and cathelicidins produced by the avian host, have been shown to dramatically reduce Campylobacter spp. colonisation in poultry, practical application for on-farm control has not been satisfactorily evaluated, most likely due to the high production cost of bacteriocins (Hoang et al. Citation2011).
Organic acids
Organic acids (including lactic acid, citric acid, malic acid, acetic acid, peroxyacetic acid, fumaric acid, gluconic acid, levulinic acid, pyruvic acid, caproic acid, caprylic acid and capric acid) are designated by the USFDA as GRAS feed additives. The antimicrobial efficacy of organic acids have been studied for decades (Scannell et al. Citation1997; Tamblyn and Conner Citation1997; Castillo et al. Citation1998, Citation1999; Ellebracht et al. Citation1999; González-Fandos et al. Citation2009; Mani-Lopez et al. Citation2012; Mohan and Pohlman Citation2016), with a particular emphasis on carcase decontamination. It is generally thought that the principal mechanism of action of organic acids occurs as a result of cytoplasmic acidification followed by the uncoupling of energy production and regulation, and through the toxic accumulation of dissociated acid anions within the cell. Organic acids have been tested as additives in drinking water and feed for Campylobacter and Salmonella spp. reduction in chickens, based on the assumption that ingested organic acids lower the pH of the chicken gut, rendering this niche more hostile to colonisation (Byrd et al. Citation2001; Chaveerach et al. Citation2004; Hilmarsson et al. Citation2006; Van Immerseel et al. Citation2006; De Los Santos et al. Citation2008a, Citation2008b; Van Deun et al. Citation2008; Skånseng et al. Citation2010; Hermans et al. Citation2011a; Jansen et al. Citation2014).
Results from a comparative study on the effects of the commercial probiotic Primalac®, organic acid Selko®-pH and plant extract Sangrovit® treatments found that, on day 49 of broiler life, all supplemented treatments showed a reduction in caecal content Campylobacter spp. colonisation. In addition, faecal samples showed reductions on days 35 and 42, which was in line with the age of slaughter for most mass-produced broilers (Gharib et al. Citation2012).
Although in vitro studies have demonstrated that organic acids, medium chain fatty acids (MCFA) or MCFA monoglycerides have strong bactericidal effects on Campylobacter spp., inconsistent results have been reported from in vivo trials. For example, caprylic acid in feed lowered the C. jejuni load in chicken caeca, and reduced carcase contamination during slaughter (De Los Santos et al. Citation2008a, Citation2008b). Conversely, the use of butyrate, acetate, propionate and l-lactate did not protect broilers from caecal colonisation, despite the marked bactericidal effect of butyrate in vitro (Van Deun et al. Citation2008). This was possibly due to the protective effect of mucus and the rapid absorption of butyrate by enterocytes. However, butyrate was able to protect Caco-2 cells from Campylobacter spp. invasion and translocation, two major virulence mechanisms, but not from a decline in transepithelial resistance (Van Deun et al. Citation2008). In addition, the use of a probiotic preparation containing Pediococcus acidilactici and Saccharomyces boulardii followed by the addition of acidifiers, such as formic and lactic acids, resulted in a significant reduction in Campylobacter spp. shedding and re-isolation, as well as reducing lesions (Abd EI-Ghany et al., Abd et al. Citation2015). These studies highlighted the potential of using the ‘hurdle effect’ to manage sequential supplementation of poultry flocks to prevent colonisation and transmission of Campylobacter spp. in poultry flocks.
Plant and marine extracts
A wide range of plant extracts and compounds have demonstrated strong bactericidal activity against Campylobacter spp. in vitro (Friedman et al. Citation2002; Sirirak and Voravuthikunchai Citation2011; Hermans et al. Citation2012; Robyn et al. Citation2013); however, the effectiveness of treatments varies considerably.
Natural plant extracts have been shown to have effects against other enteric pathogens. Thymol and carvacrol are phenolic plant extracts that act by altering cell membrane permeability, resulting in cellular content leakage and cell death (Lambert et al. Citation2001; Ultee et al. Citation2002). Chitosan is a natural by-product derived from the deacetylation of chitin that can be obtained from crab and shrimp shell waste (Younes and Rinaudo Citation2015). Although the precise mode of action of chitosan is not completely understood, it is hypothesised that it may alter the permeability of the outer cell membrane of bacterial pathogens, disrupting cellular physiology and causing cell death. C. jejuni and C. coli have been reported as being sensitive to chitosan in vitro (Ganan et al. Citation2009; Mengíbar et al. Citation2011). In vivo, a 0.5% dose of chitosan was found to reduce caecal Campylobacter spp. counts in broiler chickens. In addition, RT-qPCR analysis revealed that chitosan down-regulated the expression of chicken colonisation genes when compared to a control (Arambel et al. Citation2015). This suggested that chitosan supplementation could be a potential strategy to reduce the enteric colonisation of Campylobacter spp. in pre-harvest chickens.
Bacteriophage
Bacteriophages can be applied as both pre- and post-harvest interventions to reduce the transmission of specific foodborne pathogens (Teng-hern et al. Citation2014). Bacteriophages invade bacterial cells and interfere with bacterial metabolism, causing bacterial lysis. Carrillo et al. (Citation2005) were the first to perform bacteriophage treatment in chickens and discovered an effective reduction of Campylobacter spp. counts in the caecal contents of treated broiler chickens using pre-harvest interventions. The use of a combination of phages can provide a greater decrease in Campylobacter spp. levels in the caecal contents of infected broiler chickens than a single-phage approach (Wagenaar et al. Citation2005; Fischer et al. Citation2013). Phage type has a variable effect on Campylobacter spp. control as well. Wagenaar et al. (Citation2005) found that phage 71 can reduce C. jejuni colonisation by 1 log over the period of 30 days while the addition of phage 69 resulted in a reduction of 1.5 log in CFU counts over the same period. These observations were in agreement with other studies that showed the colonisation of both C. jejuni and C. coli in chickens was successfully reduced upon the exposure to species-specific bacteriophages (see ) (El-shibiny et al. Citation2009; Carvalho et al. Citation2010). Several studies on post-harvest interventions using bacteriophages against Campylobacter spp. were conducted to control pathogen contamination on food surfaces, such as chicken skin (Atterbury et al. Citation2003; Goode et al. Citation2003; Endersen et al. Citation2014). Although studies showed a small decrease in Campylobacter spp. levels on skin with post-harvest phage treatment, the reduction was greater when freezing at −20°C was used in conjunction with the bacteriophage treatment (Atterbury et al. Citation2003).
Summary of in-feed treatments
It is always a difficult task to draw definitive conclusions from experimental research spanning different research eras, taking into account differences in experimental design and variations between trials within and between research groups. However, from the literature, it is clear that a distinct, albeit not unexpected, difference between the performance of in-feed interventions exists when examined in vitro compared to those determined from in vivo studies. This would explain why the global poultry industry is finding it difficult to establish consensus on a single acceptable feed additive that will obtain significant reductions in commercial settings. It is much more likely that pooling some of the discussed approaches within an in-feed tool kit will provide an answer. As it stands, there have been some positive results published, such as the combination of selected probiotics, plant extracts and organic acid products. In one study, Campylobacter spp. counts were not reduced by any fructooligosaccharide treatments (0.125%, 0.25%, or 0.5%) or mannanoligosaccharide (MOS) concentrations (0.04%, 0.08%, or 0.16%), but were reduced by the combination of Lactobacillus salivarius subsp. salicinius with 0.04% MOS in feed (Arsi et al. Citation2015). The results of this study indicated that selection and application of bacterial isolates in combination with selected prebiotics can reduce the carriage of caecal Campylobacter spp. in pre-harvest broilers (Arsi et al. Citation2015). Both bodyweight and feed intake in the probiotic-treated group were higher than in the control, while the villus height of the duodenum and jejunum in the probiotic and plant extract-treated groups were improved. It was concluded that the supplementation of organic acids in drinking water and the addition of probiotics and plant extract to broiler feed may reduce the incidence of Campylobacter spp. colonisation (Gharib et al. Citation2012).
Transportation to factory
At time of harvest, birds must be caught and transported to the poultry processing plant in crates. High stress during the catching process can cause defecation, increasing faecal contamination of adjacent birds and surfaces. Feed withdrawal is practised to minimise the amount of faeces produced and consequently prevent spread, but significant opportunity still exists for Campylobacter spp. spread within a flock. This may increase the microbial load of birds entering the slaughterhouse (Slader et al. Citation2002; Hastings et al. Citation2011; Newell et al. Citation2011). However, flocks that are contaminated immediately prior to slaughter tend to exhibit a lower level of carcase contamination than birds that had been colonised on farm, presumably because contamination is restricted to feathers (Hansson et al. Citation2007).
The design of the transport crate may facilitate the spread of faeces between birds, and the recycling of contaminated crates between the processing plant and the rearing farms poses a risk of Campylobacter spp. transmission, especially if depopulation takes place over an extended period. Over a two-year period, a study by Hastings et al. (Citation2011) indicated that 23.5% of empty crates used during thinning were contaminated (Allen et al. Citation2008; Hastings et al. Citation2011). Campylobacter can survive on transport cages in dried faeces at ambient temperatures (18–31°C) for up to 8 hours, with bacterial levels only being reduced by half after 24 hours (Berrang et al. Citation2004). Both transport vehicles and crates should be washed and disinfected after every journey in order to reduce cross-contamination between Campylobacter spp.-positive and negative flocks, and decrease the amount of contamination introduced into the slaughter facility (Allen et al. Citation2008). However, a number of studies have shown that many crates remain contaminated with Campylobacter spp. after cleaning, as they are very difficult to effectively sanitise (Corry et al. Citation2002; Hansson et al. Citation2005; Hastings et al. Citation2011).
Conclusions
The burden of Campylobacter spp. within the developed world is a constant pressure on health services, especially with emerging trends of frequent hospitalisations and the development of drug resistance in humans. The on-farm and in-transport interventions discussed could be vital interventions in the reduction of the incidence of contamination within the food-production chain. The role of the farm environment as the primary source of infection highlights its key role as a control point for the pathogen. Interventions such as feed and water-additives, vaccines and, most importantly, consistent biosecurity measures, exhibit potential for the effective control of this pathogen and its dissemination within the food chain. Furthermore, the combination of various dietary approaches and improved biosecurity measures may improve Campylobacter spp. transmission synergistically.
However, it can be hard to assess the impact of these interventions on a global scale. When analysing the literature, it is often difficult to obtain an accurate comparison of treatment effects between studies, due to differences that exist in experimental design, sampling protocols and target microbes. For example, some studies use naturally contaminated chicken while others artificially inoculate chickens with the pathogen of interest. In addition, the parts of the chicken (i.e. fillets, wings, whole carcases) used in the experiments vary from study to study. This can make it difficult to fully ascertain the effect of these proposed interventions on Campylobacter spp. transmission in retail poultry meat. The issue of cost of implementation must be taken into account, both in terms of loss of earnings and expenditure on the application of the discussed steps. This may be displeasing to producers, who may not want to take on the burden of the implementation of these processes. This may be remedied through provision of incentives, although the burden of cost may fall to the government, retailers or, ultimately, consumers.
It must be acknowledged that the multifaceted avenues of contamination that poultry meat is exposed to prior to consumption, such as the slaughter process and food handling, contribute to it being such a prevalent cause of disease beyond the on-farm causes discussed above (Lu et al. Citation2019). The evisceration and plucking steps of poultry carcase preparation are known critical control points, in which high levels of microbe transfer between contaminated and ‘clean’ carcases occur. Manual manipulation and poor food hygiene during preparation of chicken and adjacent foodstuffs (such as salad) contribute greatly to the spread of Campylobacter spp. among meals and preparation surfaces. These steps cannot be controlled by the aforementioned on-farm interventions. Thus, it is vital that in-factory interventions and high standards of food safety are implemented beyond the poultry husbandry steps discussed in this review. Campylobacter spp. contamination is a constant public safety issue, and a farm-to-fork approach is necessary to ensure that this prolific pathogen is managed.
Acknowledgments
The author, Ting Lu, has received funding from China Scholarship Council (CSC).
Disclosure statement
No potential conflict of interest was reported by the authors.
Additional information
Funding
References
- Abd, E. I., W. A. Ghany, M. H. Awaad, and S. R. Nagwa. 2015. “Efficacy of Certain Feed Additives for the Prevention of Campylobacter Jejuni Infection in Broiler Chickens.” Asian Journal of Animal Sciences 9 (6): 427–433. doi:10.3923/ajas.2015.427.433.
- Abudabos, A. M. 2013. “Use of a Competitive Exclusion Product (Aviguard®) to Prevent Clostridium Perfringens Colonization in Broiler Chicken under Induced Challenge.” In Pakistan Journal of Zoology 45: 371–376.
- Abudabos, A. M., H. A. Al-Batshan, and M. A. Murshed. 2015. “Effects of Prebiotics and Probiotics on the Performance and Bacterial Colonization of Broiler Chickens.” South African Journal Of Animal Science 45 (4): 419-428. doi:10.4314/sajas.v45i4.8.
- Allain, V., M. Chemaly, M.-J. Laisney, S. Rouxel, S. Quesne, and S. Le Bouquin. 2014. “Prevalence of and Risk Factors for Campylobacter Colonisation in Broiler Flocks at the End of the Rearing Period in France.” British Poultry Science 55 (4): 452–459. doi:10.1080/00071668.2014.941788.
- Allen, V. M., A. M. Ridley, J. A. Harris, D. G. Newell, and L. Powell. 2011. “Influence of Production System on the Rate of Onset of Campylobacter Colonization in Chicken Flocks Reared Extensively in the United Kingdom.” British Poultry Science 52 (1): 30–39. doi:10.1080/00071668.2010.537306.
- Allen, V. M., H. Weaver, A. M. Ridley, J. A. Harris, M. Sharma, J. Emery, N. Sparks, M. Lewis, and S. Edge. 2008. “Sources and Spread of Thermophilic Campylobacter Spp. During Partial Depopulation of Broiler Chicken Flocks.” Journal of Food Protection 71 (2): 264–270. doi:10.4315/0362-028X-71.2.264.
- Arambel, H. R., A. M. Donoghue, K. Arsi, A. Upadhyay, and A. Woo. 2015. “Chitosan Supplementation Reduces Enteric Colonization of Campylobacter Jejuni in Broiler Chickens and Down-Regulates Expression of Colonization Genes.” Advances in Food Technology and Nutritional Sciences 104–111. doi:10.17140/AFTNSOJ-1-119.
- Arsi, K., A. M. Donoghue, A. Woo-Ming, P. J. Blore, and D. J. Donoghue. 2015. “The Efficacy of Selected Probiotic and Prebiotic Combinations in Reducing Campylobacter Colonization in Broiler Chickens.” Journal of Applied Poultry Research. 24 (3): 327–334. doi:10.3382/japr/pfv032.
- Arsi, K., A. M. Donoghue, K. Venkitanarayanan, A. Kollanoor-Johny, A. C. Fanatico, P. J. Blore, and D. J. Donoghue. 2014. “The Efficacy of the Natural Plant Extracts, Thymol and Carvacrol against Campylobacter Colonization in Broiler Chickens.” Journal of Food Safety 34 (4): 321–325. doi:10.1111/jfs.12129.
- Atterbury, R. J., P. L. Connerton, C. E. R. Dodd, C. E. D. Rees, and I. F. Connerton. 2003. “Application of Host-Specific Bacteriophages to the Surface of Chicken Skin Leads to a Reduction in Recovery of Campylobacter Jejuni.” Applied and Environmental Microbiology 69 (10): 6302–6306. doi:10.1128/AEM.69.10.6302-6306.2003.
- Baffoni, L., F. Gaggìa, D. D. Gioia, C. Santini, L. Mogna, and B. Biavati. 2012. “A Bifidobacterium-Based Synbiotic Product to Reduce the Transmission of C. Jejuni along the Poultry Food Chain.” International Journal of Food Microbiology 157 (2): 156–161. doi:10.1016/J.IJFOODMICRO.2012.04.024.
- Bahrndorff, S., L. Rangstrup-Christensen, S. Nordentoft, and B. Hald. 2013. “Foodborne Disease Prevention and Broiler Chickens with Reduced Campylobacter Infection.” Emerging Infectious Diseases 19 (3): 425–430. doi:10.3201/eid1903.111593.
- Barrios, P. R., J. Reiersen, R. Lowman, J. R. Bisaillon, P. Michel, V. Fridriksdóttir, and E. Gunnarsson. 2006. “Risk Factors for Campylobacter Spp. Colonization in Broiler Flocks in Iceland.” Preventive Veterinary Medicine 74 (4): 264–278. doi:10.1016/j.prevetmed.2005.12.003.
- Batz, M. B., S. Hoffmann, and J. G. Morris. 2012. “Ranking the Disease Burden of 14 Pathogens in Food Sources in the United States Using Attribution Data from Outbreak Investigations and Expert Elicitation.” Journal of Food Protection 75 (7): 1278–1291. doi:10.4315/0362-028X.JFP-11-418.
- Baurhoo, B., P. R. Ferket, and X. Zhao. 2009. “Effects of Diets Containing Different Concentrations of Mannanoligosaccharide or Antibiotics on Growth Performance, Intestinal Development, Cecal and Litter Microbial Populations, and Carcass Parameters of Broilers.” Poultry Science 88 (11): 2262–2272. doi:10.3382/ps.2008-00562.
- Bermudez-Brito, M., J. Plaza-Díaz, S. Muñoz-Quezada, C. Gómez-Llorente, and A. Gil. 2012. “Probiotic Mechanisms of Action.” Metab 61: 160–174.
- Berrang, M. E., J. K. Northcutt, and J. A. Cason. 2004. “Recovery of Campylobacter from Broiler Feces during Extended Storage of Transport Cages.” Poultry Science 83 (7): 1213–1217. doi:10.1093/ps/83.7.1213.
- Bolton, D. J. 2015. “Campylobacter Virulence and Survival Factors.” Food Microbiology 48: 99–108. doi:10.1016/j.fm.2014.11.017.
- Bratz, K., G. Gölz, P. Janczyk, K. Nöckler, and T. Alter. 2015. “Analysis of in Vitro and in Vivo Effects of Probiotics against Campylobacter Spp.” Berl. Münc. Tierärztl. Wochenschr 128 (3–4): 155–162. doi:10.2376/0005-9366-128-155.
- Buckley, A. M., J. Wang, D. L. Hudson, A. J. Grant, M. A. Jones, D. J. Maskell, and M. P. Stevens. 2010. “Evaluation of Live-attenuated Salmonella Vaccines Expressing Campylobacter Antigens for Control of C. Jejuni in Poultry.” Vaccine 28 (4): 1094–1105. doi:10.1016/j.vaccine.2009.10.018.
- Bull, S., V. Allen, G. Domingue, F. Jørgensen, R. Ure, R. Whyte, D. Tinker, J. Corry, T. Humphrey, and J. Frost. 2006. “Sources of Campylobacter Spp. Colonizing Housed Broiler Flocks during Rearing Sources of Campylobacter Spp.” Colonizing Housed Broiler Flocks during Rearing 72 (1): 645–652. doi:10.1128/AEM.72.1.645.
- Burke, M. 2018. ROI Poultry Industry National Biosecurity Plan 2018. St David's Poultry Team Ireland. Accessed April 10, 2019. Available at: https://www.stdavids-poultryteam.ie/roi-poultry-industry-national-biosecurity-plan-2018/
- Burnham, P. M., and D. R. Hendrixson. 2018. “Campylobacter Jejuni: Collective Components Promoting a Successful Enteric Lifestyle.” Nature Reviews. Microbiology 16 (9): 551–565. doi:10.1038/s41579-018-0037-9.
- Byrd, J. A., B. M. Hargis, D. J. Caldwell, R. H. Bailey, K. L. Herron, J. L. McReynolds, and R. L. Brewer. 2001. “Effect of Lactic Acid Administration in the Drinking Water during Preslaughter Feed Withdrawal on Salmonella and Campylobacter Contamination of Broilers.” Poultry Science 80 (3): 278–283. doi:10.1093/ps/80.3.278.
- Callicott, K. A., V. Frioriksdóttir, J. Reiersen, R. Lowman, J. R. Bisaillon, E. Gunnarsson, E. Berndtson, K. L. Hiett, D. S. Needleman, and N. J. Stern. 2006. “Lack of Evidence for Vertical Transmission of Campylobacter Spp. In Chickens.” Applied and Environmental Microbiology 72 (9): 5794–5798. doi:10.1128/AEM.02991-05.
- Carrillo, C. L., R. J. Atterbury, E. Dillon, A. Scott, I. F. Connerton, and P. L. Connerton. 2005. “Bacteriophage Therapy to Reduce Campylobacter Jejuni Colonization of Broiler Chickens Bacteriophage Therapy to Reduce Campylobacter Jejuni Colonization of Broiler Chickens †.” Applied and Environmental Microbiology 71 (11): 6554–6563. doi:10.1128/AEM.71.11.6554-6563.2005.
- Carron, M., Y. M. Chang, K. Momanyi, J. Akoko, J. Kiiru, J. Bettridge, and G. Chaloner. 2018. “Campylobacter, a Zoonotic Pathogen of Global Importance: Prevalence and Risk Factors in the Fast-Evolving Chicken Meat System of Nairobi, Kenya.” PLoS Neglected Tropical Diseases 12 (8): 1–19. doi:10.1371/journal.pntd.0006658.
- Carvalho, C. M., B. W. Gannon, D. E. Halfhide, S. B. Santos, C. M. Hayes, J. M. Roe, and J. Azeredo. 2010. “The in Vivo Efficacy of Two Administration Routes of a Phage Cocktail to Reduce Numbers of Campylobacter Coli and Campylobacter Jejuni in Chickens.” BMC Microbiology 10 (1): 232. doi:10.1186/1471-2180-10-232.
- Castillo, A., L. M. Lucia, K. J. Goodson, J. W. Savell, and G. R. Acuff. 1998. “Comparison of Water Wash, Trimming, and Combined Hot Water and Lactic Acid Treatments for Reducing Bacteria of Fecal Origin on Beef Carcasses.” Journal of Food Protection 61 (7): 823–828. doi:10.4315/0362-028X-61.7.823.
- Castillo, A., L. M. Lucia, K. J. Goodson, J. W. Savell, and G. R. Acuff. 1999. “Decontamination of Beef Carcass Surface Tissue by Steam Vacuuming Alone and Combined with Hot Water and Lactic Acid Sprays.” Journal of Food Protection 62 (2): 146–151. doi:10.4315/0362-028X-62.2.146.
- Chaveerach, P., D. A. Keuzenkamp, H. A. Urlings, L. J. Lipman, and F. Van Knapen. 2002. “In Vitro Study on the Effect of Organic Acids on Campylobacter Jejuni/coli Populations in Mixtures of Water and Feed.” Poultry Science 81 (5): 621–628. doi:10.1093/ps/81.5.621.
- Chaveerach, P., D. A. Keuzenkamp, L. J. A. Lipman, and F. Van Knapen. 2004. “Effect of Organic Acids in Drinking Water for Young Broilers on Campylobacter Infection, Volatile Fatty Acid Production, Gut Microflora and Histological Cell Changes.” Poultry Science 83 (3): 330–334. doi:10.1093/ps/83.3.330.
- Cleveland, J., J. T. Montville, I. F. Nes, and M. L. Chikindas. 2001. “Bacteriocins: Safe, Natural Antimicrobials for Food Preservation.” International Journal of Food Microbiology 71 (1): 1–20. doi:10.1016/S0168-1605(01)00560-8.
- Coelho, M. L. V., J. Dos Santos Nascimento, P. C. Fagundes, D. J. Madureira, S. S. de Oliveira, M. A. V. de Paiva Brito, and M. D. C. de Freire Bastos. 2007. “Activity of Staphylococcal Bacteriocins against Staphylococcus Aureus and Streptococcus Agalactiae Involved in Bovine Mastitis.” Research in Microbiology 158 (7): 625–630. doi:10.1016/j.resmic.2007.07.002.
- Colles, F. M., N. D. McCarthy, S. K. Sheppard, R. Layton, and M. C. J. Maiden. 2010. “Comparison of Campylobacter Populations Isolated from a Free-range Broiler Flock before and after Slaughter.” International Journal of Food Microbiology 137 (2–3): 259–264. doi:10.1016/j.ijfoodmicro.2009.12.021.
- Corrier, D. E., J. A. Byrd, B. M. Hargis, M. E. Hume, R. H. Bailey, and L. H. Stanker. 1999. “Survival of Salmonella in the Crop Contents of Market-Age Broilers during Feed Withdrawal.” Avian Diseases 43 (3): 453. doi:10.2307/1592642.
- Corry, J. E. L., V. M. Allen, W. R. Hudson, M. F. Breslin, and R. H. Davies. 2002. “Sources of Salmonella on Broiler Carcasses during Transportation and Processing: Modes of Contamination and Methods of Control.” Journal of Applied Microbiology 92 (3): 424–432. doi:10.1046/j.1365-2672.2002.01543.x.
- Cox, N. A., L. J. Richardson, J. J. Maurer, M. E. Berrang, P. J. Fedorka-cray, R. J. Buhr, and J. A. Byrd. 2012. “Evidence for Horizontal and Vertical Transmission in Campylobacter Passage from Hen to Her Progeny.” Journal of Food Protection 75 (10): 1896–1902. doi:10.4315/0362-028.JFP-11-322.
- Dale, E. L., S. P. Nolan, R. D. Berghaus, and C. L. Hofacre. 2015. “On Farm Prevention of Campylobacter and Salmonella: Lessons Learned from Basic Biosecurity Interventions.” Journal of Applied Poultry Research 24 (2): 222–232. doi:10.3382/japr/pfv016.
- De Los Santos, F., D. A. Solis, M. Donoghue, K. Venkitanarayanan, M. L. Dirain, I. Reyes-Herrera, P. J. Blore, and D. J. Donoghue. 2008a. “Caprylic Acid Supplemented in Feed Reduces Enteric Campylobacter Jejuni Colonization in Ten-Day-Old Broiler Chickens.” Poultry Science 87 (4): 800–804. doi:10.3382/ps.2007-00280.
- De Los Santos, F. S. D., A. M. Donoghue, K. Venkitanarayanan, I. Reyes-Herrera, J. H. Metcalf, M. L. Dirain, V. F. Aguiar, P. J. Blore, and D. J. Donoghue. 2008b. “Therapeutic Supplementation of Caprylic Acid in Feed Reduces Campylobacter Jejuni Colonization in Broiler Chicks.” Applied and Environmental Microbiology 74 (14): 4564–4566. doi:10.1128/AEM.02528-07.
- Deegan, L. H., P. D. Cotter, C. Hill, and P. Ross. 2006. “Bacteriocins: Biological Tools for Bio-Preservation and Shelf-Life Extension.” International Dairy Journal 16 (9): 1058–1071. doi:10.1016/j.idairyj.2005.10.026.
- Doyle, M. P., and M. C. Erickson. 2008. “Summer Meeting 2007 - the Problems with Fresh Produce: An Overview.” Journal of Applied Microbiology 105 (2): 317–330. doi:10.1111/j.1365-2672.2008.03746.x.
- EFSA. 2011. “Scientific Opinion on Campylobacter in Broiler Meat Production: Control Options and Performance Objectives And/or Targets at Different Stages of the Food Chain.” EFSA Journal 9 (4): 1–141. doi:10.2903/j.efsa.2011.2105.
- EFSA. 2014. “EFSA Explains Zoonotic Diseases - Campylobacter.” EFSA Fact Sheets. doi:10.2805/59450.
- EFSA and ECDC. 2017. “The European Union Summary Report on Trends and Sources of Zoonoses, Zoonotic Agents and Food-Borne Outbreaks in 2016.” EFSA Journal 15(12): p.e05077. doi:10.2903/j.efsa.2018.5500.
- Ellebracht, E. A., A. Castillo, L. M. Lucia, R. K. Miller, and G. R. Acuff. 1999. “Reduction of Pathogens Using Hot Water and Lactic Acid on Beef Trimmings.” Journal of Food Science 64 (6): 1094–1099. doi:10.1111/j.1365-2621.1999.tb12289.x.
- Ellis-Iversen, J., F. Jorgensen, S. Bull, L. Powell, A. J. Cook, and T. J. Humphrey. 2009. “Risk Factors for Campylobacter Colonisation during Rearing of Broiler Flocks in Great Britain.” Preventive Veterinary Medicine 89 (3–4): 178–184. doi:10.1016/j.prevetmed.2009.02.004.
- El-shibiny, A., A. Scott, A. Timms, Y. Metawea, P. Connerton, and I. Connerton. 2009. “Application of a Group II Campylobacter Bacteriophage to Reduce Strains of Campylobacter Jejuni and Campylobacter Coli Colonizing Broiler Chickens.” Journal of Food Protection 72 (4): 733–740. doi:10.4315/0362-028X-72.4.733.
- Endersen, L., J. O’mahony, C. Hill, R. P. Ross, O. Mcauliffe, and A. Coffey. 2014. “Phage Therapy in the Food Industry.” Annual Review of Food Science and Technology 5 (1): 327–349. doi:10.1146/annurev-food-030713-092415.
- English, K. G., M. Singh, and J. B. Hess. 2015. Effect of Biosecurity and Management Practices on the Prevalence of Salmonella, Campylobacter, and Clostridium Perfringens in a Poultry Production System https://etd.auburn.edu/bitstream/handle/10415/4950/Master%27s Thesis Final Version_Katrina_English.pdf?sequence=2&isAllowed=y.
- Epps, S. V. R., B. T. PetrujkicÍ, I. Sedej, N. A. Krueger, R. B. Harvey, R. C. Beier, T. B. Stanton, T. D. Phillips, R. C. Anderson, and D. J. Nisbet. 2015. “Comparison of Anti-Campylobacter Activity of Free Thymol and Thymol-β-d-Glucopyranoside in Absence or Presence of β-Glycoside-Hydrolysing Gut Bacteria.” Food Chemistry 173: 92–98. doi:10.1016/j.foodchem.2014.10.007.
- Fernandez, F., R. Sharma, M. Hinton, and M. R. Bedford. 2000. “Diet Influences the Colonisation of Campylobacter Jejuni and Distribution of Mucin Carbohydrates in the Chick Intestinal Tract.” Cellular and Molecular Life Sciences CMLS 57 (12): 1793–1801. doi:10.1007/PL00000659.
- Fischer, S., S. Kittler, G. Klein, and G. Glünder. 2013. “Impact of a Single Phage and a Phage Cocktail Application in Broilers on Reduction of Campylobacter Jejuni and Development of Resistance.” PloS One 8 (10): e78543. doi:10.1371/journal.pone.0078543.
- Food and Agricultural Organization of the United Nations and World Health Organization. 2001. Health and Nutritional Properties of Probiotics in Food Including Powder Milk with Live Lactic Acid Bacteria World Health Organization. http://www.fao.org/3/a-a0512e.pdf.
- Friedman, M., P. R. Henika, and R. E. Mandrell. 2002. “Bactericidal Activities of Plant Essential Oils and Some of Their Isolated Constituents against Campylobacter Jejuni, Escherichia Coli, Listeria Monocytogenes, and Salmonella Enterica.” Journal of Food Protection 65 (10): 1545–1560. doi:10.4315/0362-028X-65.10.1545.
- Ganan, M., A. V. Carrascosa, and A. J. Marti´nez-rodri´guez. 2009. “Antimicrobial Activity of Chitosan against Campylobacter Spp and Other Microorganisms and Its Mechanism of Action.” Journal of Food Protection 72 (8): 1735–1738. doi:10.4315/0362-028X-72.8.1735.
- Gharib, N., K., . S. Rahimi, and P. Khaki. 2012. “Comparison of the Effects of Probiotic, Organic Acid and Medicinal Plant on Campylobacter Jejuni Challenged Broiler Chickens.” Journal of Agricultural Science and Technology 14: 1485–1496.
- Gibbens, J. C., S. J. S. Pascoe, S. J. Evans, R. H. Davies, and A. R. Sayers. 2001. “A Trial of Biosecurity as A Means to Control Campylobacter Infection of Broiler Chickens.” Preventive Veterinary Medicine 48 (2): 85–99. doi:10.1016/S0167-5877(00)00189-6.
- González-Fandos, E., B. Herrera, and N. Maya. 2009. “Efficacy of Citric Acid against Listeria Monocytogenes Attached to Poultry Skin during Refrigerated Storage.” International Journal of Food Science & Technology 44 (2): 262–268. doi:10.1111/j.1365-2621.2007.01673.x.
- Goode, D., V. M. Allen, and P. A. Barrow. 2003. “Reduction of Experimental Salmonella and Campylobacter Contamination of Chicken Skin by Application of Lytic Bacteriophages.” Applied and Environmental Microbiology 69 (8): 5032–5036. doi:10.1128/AEM.69.8.5032.
- Goyal, C., R. K. Malik, and D. Pradhan. 2018. “Purification and Characterization of a Broad Spectrum Bacteriocin Produced by a Selected Lactococcus Lactis Strain 63 Isolated from Indian Dairy Products.” Journal of Food Science and Technology 55 (9): 3683–3692. doi:10.1007/s13197-018-3298-4.
- Guyard-Nicodème, M., A. Keita, S. Quesne, M. Amelot, T. Poezevara, B. Le Berre, and J. Sánchez. 2016. “Efficacy of Feed Additives against Campylobacter in Live Broilers during the Entire Rearing Period: Part B.” Poultry Science 95 (4): 886–892. doi:10.3382/ps/pev346.
- Hald, B., D. D. Bang, K. Pedersen, J. Dybdahl, M. Madsen, and T. Study. 2004. “Flies and Campylobacter Infection of Broiler Flocks.” Emerging Infectious Diseases 10 (8): 1490–1492. doi:10.3201/eid1008.040129.
- Hald, B., E. Rattenborg, and M. Madsen. 2001. “Role of Batch Depletion of Broiler Houses on the Occurrence of Campylobacter Spp. In Chicken Flocks.” Letters in Applied Microbiology 32 (4): 253–256. doi:10.1046/j.1472-765X.2001.00896.x.
- Hald, B., H. Skovgård, K. Pedersen, and H. Bunkenborg. 2008. “Influxed Insects as Vectors for Campylobacter Jejuni and Campylobacter Coli in Danish Broiler Houses.” Poultry Science 87 (7): 1428–1434. doi:10.3382/ps.2007-00301.
- Hald, B., H. M. Sommer, and H. Skovgård. 2007. “Use of Fly Screens to Reduce Campylobacter Spp. Introduction in Broiler Houses.” Emerging Infectious Diseases 13 (12): 1951–1953. doi:10.3201/eid1312.070488.
- Hansson, I., I. Vågsholm, L. Svensson, and E. Olsson Engvall. 2007. “Correlations between Campylobacter Spp. Prevalence in the Environment and Broiler Flocks.” Journal of Applied Microbiology 103 (3): 640–649. doi:10.1111/j.1365-2672.2007.03291.x.
- Hansson, I., M. Ederoth, L. Andersson, I. Vagsholm, and E. Olsson Engvall. 2005. “Transmission of Campylobacter Spp. To Chickens during Transport to Slaughter.” Journal of Applied Microbiology 99 (5): 1149–1157. doi:10.1111/j.1365-2672.2005.02689.x.
- Hastings, R., F. M. Colles, N. D. McCarthy, M. C. J. Maiden, and S. K. Sheppard. 2011. “Campylobacter Genotypes from Poultry Transportation Crates Indicate a Source of Contamination and Transmission.” Journal of Applied Microbiology 110 (1): 266–276. doi:10.1111/j.1365-2672.2010.04883.x..
- Hazeleger, W. C., N. M. Bolder, R. R. Beumer, and W. F. Jacobs-Reitsma. 2008. “Darkling Beetles (Alphitobius Diaperinus) and Their Larvae as Potential Vectors for the Transfer of Campylobacter Jejuni and Salmonella Enterica Serovar Paratyphi B Variant Java between Successive Broiler Flocks.” Applied and Environmental Microbiology 74 (22): 6887–6891. doi:10.1128/AEM.00451-08.
- Heres, L., B. Engel, H. A. P. Urlings, J. A. Wagenaar, and F. Van Knapen. 2004. “Effect of Acidified Feed on Susceptibility of Broiler Chickens to Intestinal Infection by Campylobacter and Salmonella.” Veterinary Microbiology 99 (3–4): 259–267. doi:10.1016/j.vetmic.2003.12.008.
- Hermans, D., A. Martel, K. Van Deun, M. Verlinden, F. Van Immerseel, A. Garmyn, W. Messens, M. Heyndrickx, F. Haesebrouck, and F. Pasmans. 2010. “Intestinal Mucus Protects Campylobacter Jejuni in the Ceca of Colonized Broiler Chickens against the Bactericidal Effects of Medium-chain Fatty Acids.” Poultry Science 89 (6): 1144–1155. doi:10.3382/ps.2010-00717.
- Hermans, D., F. Pasmans, W. Messens, A. Martel, F. Van Immerseel, G. Rasschaert, M. Heyndrickx, K. Van Deun, and F. Haesebrouck. 2012. “Poultry as a Host for the Zoonotic Pathogen Campylobacter Jejuni.” Vector-Borne and Zoonotic Diseases 12 (2): 89–98. doi:10.1089/vbz.2011.0676.
- Hermans, D., K. Van Deun, A. Martel, F. Van Immerseel, W. Messens, M. Heyndrickx, F. Haesebrouck, and F. Pasmans. 2011a. “Colonization Factors of Campylobacter Jejuni in the Chicken Gut.” Veterinary Research 42 (1): 82. doi:10.1186/1297-9716-42-82.
- Hermans, D., K. Van Deun, W. Messens, A. Martel, F. Van Immerseel, F. Haesebrouck, G. Rasschaert, M. Heyndrickx, and F. Pasmans. 2011b. “Campylobacter Control in Poultry by Current Intervention Measures Ineffective: Urgent Need for Intensified Fundamental Research.” Veterinary Microbiology 152 (3–4): 219–228. doi:10.1016/j.vetmic.2011.03.010.
- Hilmarsson, H., H. Thormar, J. H. Thrainsson, and E. Gunnarsson. 2006. “Effect of Glycerol Monocaprate (Monocaprin) on Broiler Chickens: An Attempt at Reducing Intestinal Campylobacter Infection.” Poultry Science 85 (4): 588–592. doi:10.1093/ps/85.4.588.
- Hoang, K. V., N. J. Stern, and J. Lin. 2011. “Development and Stability of Bacteriocin Resistance in Campylobacter Spp.” Journal of Applied Microbiology 111 (6): 1544–1550. doi:10.1111/j.1365-2672.2011.05163.x.
- Hoffmann, S., M. B. Batz, and J. G. Morris. 2012. “Annual Cost of Illness and Quality-Adjusted Life Year Losses in the United States Due to 14 Foodborne Pathogens.” Journal of Food Protection 75 (7): 1292–1302. doi:10.4315/0362-028X.JFP-11-417.
- Horrocks, S. M., R. C. Anderson, D. J. Nisbet, and S. C. Ricke. 2009. “Incidence and Ecology of Campylobacter Jejuni and Coli in Animals.” Anaerobe 15 (1–2): 18–25. doi:10.1016/j.anaerobe.2008.09.001.
- Humphrey, S., G. Chaloner, K. Kemmett, N. Davidson, N. Williams, A. Kipar, T. Humphrey, and P. Wigley. 2014. “Campylobacter Jejuni Is Not Merely a Commensal in Commercial Broiler Chickens and Affects Bird Welfare.” MBio 5 (4): 1–7. doi:10.1128/mBio.01364-14.
- Jansen, W., F. Reich, and G. Klein. 2014. “Large-Scale Feasibility of Organic Acids as a Permanent Preharvest Intervention in Drinking Water of Broilers and Their Effect on Foodborne Campylobacter Spp. Before Processing.” Journal of Applied Microbiology 116 (6): 1676–1687. doi:10.1111/jam.12490.
- Jorgensen, F., J. Ellis-Iversen, S. Rushton, S. A. Bull, S. A. Harris, S. J. Bryan, A. Gonzalez, and T. J. Humphrey. 2011. “Influence of Season and Geography on Campylobacter Jejuni and C. Coli Subtypes in Housed Broiler Flocks Reared in Great Britain.” Applied and Environmental Microbiology 77 (11): 3741–3748. doi:10.1128/AEM.02444-10.
- Khoury, C. A., and R. J. Meinersmann. 1995. “A Genetic Hybrid of the Campylobacter Jejuni FlaA Gene with LT-B of Escherichia Coli and Assessment of the Efficacy of the Hybrid Protein as an Oral Chicken Vaccine.” Avian Diseases 39 (4): 812–820. doi:10.2307/1592418.
- Kimura, K., and S. Yokoyama. 2019. “Trends in the Application of Bacillus in Fermented Foods.” Current Opinion in Biotechnology 56: 36–42. doi:10.1016/j.copbio.2018.09.001.
- King, C. H., E. B. Shotts, R. E. Wooley, and K. G. Porter. 1988. “Survival of Coliforms and Bacterial Pathogens within Protozoa during Chlorination.” Applied and Environmental Microbiology 54 (12): 3023–3033. doi:10.1128/AEM.54.12.3023-3033.1988.
- Kobierecka, P. A., A. K. Wyszynska, J. Gubernator, M. Kuczkowski, O. Wisniewski, M. Maruszewska, and A. Wojtania. 2016. “Chicken Anti-Campylobacter Vaccine - Comparison of Various Carriers and Routes of Immunization.” Frontiers in Microbiology 7 (MAY): 1–13. doi:10.3389/fmicb.2016.00740.
- Lambert, R. J. W., P. N. Skandamis, P. J. Coote, and G. J. E. Nychas. 2001. “A Study of the Minimum Inhibitory Concentration and Mode of Action of Oregano Essential Oil, Thymol and Carvacrol.” Journal of Applied Microbiology 91 (3): 453–462. doi:10.1046/j.1365-2672.2001.01428.x.
- Layton, S. L., M. J. Morgan, K. Cole, Y. M. Kwon, D. J. Donoghue, B. M. Hargis, and N. R. Pumford. 2011. “Evaluation of Salmonella-vectored Campylobacter Peptide Epitopes for Reduction of Campylobacter Jejuni in Broiler Chickens.” Clinical and Vaccine Immunology 18: 449–454. doi:10.1128/CVI.00379-10.
- Lewus, C. B., A. L. A. N. Kaiser, and T. J. Montville. 1991. “Inhibition of Food-Borne Bacterial Pathogens by Bacteriocins from Lactic Acid Bacteria Isolated Inhibition of Food-Borne Bacterial Pathogens by Bacteriocins from Lactic Acid Bacteria Isolated from Meat.” Applied and Environmental Microbiology 57 (6): 1683–1688. doi:10.1128/AEM.57.6.1683-1688.1991.
- Lin, J. 2009. “Novel Approaches for Campylobacter Control in Poultry.” Foodborne Pathogens and Disease 6 (7): 755–765. doi:10.1089/fpd.2008.0247.
- Line, J. E., E. A. Svetoch, B. V. Eruslanov, V. V. Perelygin, E. V. Mitsevich, I. P. Mitsevich, and V. P. Levchuk. 2008. “Isolation and Purification of Enterocin E-760 with Broad Antimicrobial Activity against Gram-Positive and Gram-Negative Bacteria.” Antimicrobial Agents and Chemotherapy 52 (3): 1094–1100. doi:10.1128/AAC.01569-06.
- Line, J. E., J. S. Bailey, N. A. Cox, N. J. Stern, and T. Tompkins. 1998. “Effect of Yeast-Supplemented Feed on Salmonella and Campylobacter Populations in Broilers.” Poultry Science 77 (3): 405–410. doi:10.1093/ps/77.3.405.
- Liu, X., L. J. Adams, X. Zeng, and J. Lin. 2019. “Evaluation of in Ovo Vaccination of DNA Vaccines for Campylobacter Control in Broiler Chickens.” Vaccine 37 (29): 3785–3792. doi:10.1016/j.vaccine.2019.05.082.
- Lohans, C. T., M. J. van Belkum, J. Li, and J. C. Vederas. 2015. “Characteriation of Bacterial Antimicrobial Peptides Active against Campylobacter Jejuni.” Canadian Journal of Chemistry 93: 381–388. doi:10.1139/cjc-2014-0411.
- Loretz, M., R. Stephan, and C. Zweifel. 2010. “Antimicrobial Activity of Decontamination Treatments for Poultry Carcasses: A Literature Survey.” Food Control 21 (6): 791–804. doi:10.1016/j.foodcont.2009.11.007.
- Lu, T., M. Marmion, M. Ferone, P. Wall, and A. G. M. Scannell. 2019. “Processing and Retail Strategies to Minimize Campylobacter Contamination in Retail Chicken.” Journal of Food Processing and Preservation 43 (12): e14251. doi:10.1111/jfpp.14251.
- Mani-Lopez, E., H. S. García, and A. López-Malo. 2012. “Organic Acids as Antimicrobials to Control Salmonella in Meat and Poultry Products.” Food Research International 45 (2): 713–721. doi:10.1016/j.foodres.2011.04.043.
- Meerburg, B. G. 2010. “Rodents are a Risk Factor for the Spreading of Pathogens on Farms.” Veterinary Microbiology 142 (3–4): 464–465. doi:10.1016/j.vetmic.2009.06.038.
- Mengíbar, M., M. Ganan, B. Miralles, A. V. Carrascosa, A. J. Martínez-Rodriguez, M. G. Peter, and A. Heras. 2011. “Antibacterial Activity of Products of Depolymerization of Chitosans with Lysozyme and Chitosanase against Campylobacter Jejuni.” Carbohydrate Polymers 84 (2): 844–848. doi:10.1016/j.carbpol.2010.04.042.
- Milbradt, E. L., J. R. Zamae, J. P. Araújo Júnior, P. Mazza, C. R. Padovani, V. R. Carvalho, C. Sanfelice, D. M. Rodrigues, A. S. Okamoto, and R. L. Andreatti Filho. 2014. “Control of Salmonella Enteritidis in Turkeys Using Organic Acids and Competitive Exclusion Product.” Journal of Applied Microbiology 117 (2): 554–563. doi:10.1111/jam.12537.
- Mohan, A., and F. W. Pohlman. 2016. “Role of Organic Acids and Peroxyacetic Acid as Antimicrobial Intervention for Controlling Escherichia Coli O157: H7 on Beef Trimmings.” LWT-Food Science and Technology 65: 868–873. doi:10.1016/j.lwt.2015.08.077.
- Morishita, T. Y., P. P. Aye, B. S. Harr, C. W. Cobb, and J. R. Clifford. 1997. “Evaluation of an Avian-specific Probiotic to Reduce the Colonization and Shedding of Campylobacter Jejuni in Broilers.” Avian Diseases 41 (4): 850–855. doi:10.2307/1592338.
- Murphy, C. P., C. Carson, B. A. Smith, B. Chapman, J. Marrotte, M. McCann, C. Primeau, P. Sharma, and E. J. Parmley. 2018. “Factors Potentially Linked with the Occurrence of Antimicrobial Resistance in Selected Bacteria from Cattle, Chickens and Pigs: A Scoping Review of Publications for Use in Modelling of Antimicrobial Resistance (IAM.AMR Project).” Zoonoses and Public Health 65 (8): 957–971. doi:10.1111/zph.12515.
- Natsos, G., K. C. Koutoulis, E. Sossidou, M. Chemaly, and N. K. Mouttotou. 2018. “Campylobacter Spp. Infection in Humans and Poultry.” Journal of the Hellenic Veterinary Medical Society 67 (2): 65–82. doi:10.12681/jhvms.15624.
- Natsos, G., N. K. Mouttotou, S. Ahmad, Z. Kamran, A. Ioannidis, and K. C. Koutoulis. 2019. “The Genus Campylobacter: Detection and Isolation Methods, Species Identification & Typing Techniques.” Journal of the Hellenic Veterinary Medical Society 70 (1): 1327–1338. doi:10.12681/jhvms.20337.
- Nauta, M. J., W. F. Jacobs-Reitsma, and A. H. Havelaar. 2007. “A Risk Assessment Model for Campylobacter in Broiler Meat.” Risk Analysis 27 (4): 845–861. doi:10.1111/j.1539-6924.2006.00834.x.
- Neal-McKinney, J. M., D. R. Samuelson, T. P. Eucker, M. S. Nissen, R. Crespo, and M. E. Konkel. 2014. “Reducing Campylobacter Jejuni Colonization of Poultry via Vaccination.” PLoS One 9 (12): e114254. doi:10.1371/journal.pone.0114254.
- Newell, D. G., and C. Fearnley. 2003. “Sources of Campylobacter Colonization in Broiler Chickens.” Applied and Environmental Microbiology 69 (8): 4343–4351. doi:10.1128/AEM.69.8.4343-4351.2003.
- Newell, D. G., K. T. Elvers, D. Dopfer, I. Hansson, P. Jones, S. James, and J. Gittins. 2011. “Biosecurity-Based Interventions and Strategies to Reduce Campylobacter Spp. On Poultry Farms.” Applied and Environmental Microbiology 77 (24): 8605–8614. doi:10.1128/AEM.01090-10.
- Nichols, G. L. 2005. “Fly Transmission of Campylobacter.” Emerging Infectious Diseases 11 (3): 361–364. doi:10.3201/eid1103.040460.
- Nuengjamnong, C., and T. Luangtongkum. 2014. “Effects of Effective Microorganisms on Growth Performances, Ammonia Reduction, Hematological Changes and Shedding of Salmonella Enterica and Campylobacter Spp. In Broilers.” The Thai Journal of Veterinary Medicine 44: 15–22.
- Olofsson, J. 2015. Amoebae as Hosts and Vectors for Spread of Campylobacter Jejuni PhD diss., Acta Universitatis Upsaliensis.
- Patriarchi, A., Á. Fox, B. Maunsell, S. Fanning, and D. Bolton. 2011. “Molecular Characterization and Environmental Mapping of Campylobacter Isolates in a Subset of Intensive Poultry Flocks in Ireland.” Foodborne Pathogens and Disease 8 (1): 99–108. doi:10.1089/fpd.2010.0637.
- Pattison, M. 2001. “Practical Intervention Strategies for Campylobacter.” Journal of Applied Microbiology Symposium Supplement 90 (30): 121S–125S. doi:10.1046/j.1365-2672.2001.01360.x.
- Pearson, A. D., M. Greenwood, T. D. Healing, D. Rollins, M. Shahamat, J. Donaldson, and R. R. Colwell. 1993. “Colonization of Broiler Chickens by Waterborne Campylobacter Jejuni.” Applied and Environmental Microbiology 59 (4): 987–996. doi:10.1128/AEM.59.4.987-996.1993.
- Pedersen, S. K., J. A. Wagenaar, H. Vigre, L. Roer, M. Mikoleit, A. Aidara-Kane, A. L. Cawthorne, F. M. Aarestrup, and R. S. Hendriksen. 2018. “Proficiency of WHO Global Foodborne Infections Network External Quality Assurance System Participants in the Identification and Susceptibility Testing of Thermo-Tolerant Campylobacter Spp. From 2003-2012.” Journal of Clinical Microbiology 56: 11. doi:10.1128/JCM.01066-18.
- Rasschaert, G., L. De Zutter, L. Herman, and M. Heyndrickx. 2020. “Campylobacter Contamination of Broilers: The Role of Transport and Slaughterhouse.” International Journal of Food Microbiology 322: 108564. doi:10.1016/j.ijfoodmicro.2020.108564.
- Rice, B. E., D. M. Rollins, E. T. Mallinson, L. Carr, and S. W. Joseph. 1997. “Campylobacter Jejuni in Broiler Chickens: Colonization and Humoral Immunity following Oral Vaccination and Experimental Infection.” Vaccine 15: 1922–1932. doi:10.1016/S0264-410X(97)00126-6.
- Ridley, A., V. Morris, J. Gittins, S. Cawthraw, J. Harris, S. Edge, and V. Allen. 2011. “Potential Sources of Campylobacter Infection on Chicken Farms: Contamination and Control of Broiler-Harvesting Equipment, Vehicles and Personnel.” Journal of Applied Microbiology 111 (1): 233–244. doi:10.1111/j.1365-2672.2011.05038.x.
- Ridley, A. M., V. M. Allen, M. Sharma, J. A. Harris, and D. G. Newell. 2008. “Real-Time PCR Approach for Detection of Environmental Sources of Campylobacter Strains Colonizing Broiler Flocks.” Applied and Environmental Microbiology 74 (8): 2492–2504. doi:10.1128/AEM.01242-07.
- Ringoir, D. D., and V. Korolik. 2003. “Colonisation Phenotype and Colonisation Potential Differences in Campylobacter Jejuni Strains in Chickens before and after Passage in Vivo.” Veterinary Microbiology 92 (3): 225–235. doi:10.1016/S0378-1135(02)00378-4.
- Rivoal, K., C. Ragimbeau, G. Salvat, P. Colin, and G. Ermel. 2005. “Genomic Diversity of Campylobacter Coli and Campylobacter Jejuni Isolates Recovered from Free-Range Broiler Farms and Comparison with Isolates of Various Origins.” Applied and Environmental Microbiology 71 (10): 6216–6227. doi:10.1128/AEM.71.10.6216-6227.2005.
- Robyn, J., G. Rasschaert, D. Hermans, F. Pasmans, and M. Heyndrickx. 2013. “In Vivo Broiler Experiments to Assess Anti-Campylobacter Jejuni Activity of a Live Enterococcus Faecalis Strain.” Poultry Science 92 (1): 265–271. doi:10.3382/ps.2012-02712.
- Rosenquist, H., L. Boysen, C. Galliano, S. Nordentoft, S. Ethelberg, and B. Borck. 2009. “Danish Strategies to Control Campylobacter in Broilers and Broiler Meat: Facts and Effects.” Epidemiology and Infection 137 (12): 1742–1750. doi:10.1017/S0950268809002684.
- Sahin, O., I. I. Kassem, Z. Shen, J. Lin, G. Rajashekara, and Q. Zhang. 2015. “Campylobacter in Poultry: Ecology and Potential Interventions.” Avian Diseases 59 (2): 185–200. doi:10.1637/11072-032315-Review.
- Sahin, O., P. Kobalka, and Q. Zhang. 2003. “Detection and Survival of Campylobacter in Chicken Eggs.” Journal of Applied Microbiology 95 (5): 1070–1079. doi:10.1046/j.1365-2672.2003.02083.x.
- Sahin, O., T. Y. Morishita, and Q. Zhang. 2002. “Campylobacter Colonization in Poultry: Sources of Infection and Modes of Transmission.” Animal Health Research Reviews 3 (2): 95–105. doi:10.1079/AHRR200244.
- Saint-Cyr, M. J., M. Guyard-Nicodème, S. Messaoudi, M. Chemaly, J. M. Cappelier, X. Dousset, and N. Haddad. 2016. “Recent Advances in Screening of anti-Campylobacter Activity in Probiotics for Use in Poultry.” Frontiers in Microbiology 7: 553. doi:10.3389/fmicb.2016.00553.
- Santini, C., L. Baffoni, F. Gaggia, M. Granata, R. Gasbarri, D. D. Gioia, and B. Biavati. 2010. “Characterization of Probiotic Strains: An Application as Feed Additives in Poultry against Campylobacter Jejuni.” International Journal of Food Microbiology 141: S98–108. doi:10.1016/j.ijfoodmicro.2010.03.039.
- Scanes, C. G. 2016. “Biology of Stress in Poultry with Emphasis on Glucocorticoids and the Heterophil to Lymphocyte Ratio.” Poultry Science 95 (9): 2208–2215. doi:10.3382/ps/pew137.
- Scannell, A. G. M., C. Hill, D. J. Buckley, and E. K. Arendt. 1997. “Determination of the Influence of Organic Acids and Nisin on Shelf-Life and Microbiological Safety Aspects of Fresh Pork Sausage.” Journal of Applied Microbiology 83 (4): 407–412. doi:10.1046/j.1365-2672.1997.00248.x.
- Scannell, A. G. M., C. Hill, R. P. Ross, G. Schwarz, and E. K. Arendt. 2001. “Effect of Nitrite on a Bacteriocinogenic Lactococcus Lactis Transconjugant in Fermented Sausage.” European Food Research and Technology 213 (1): 48–52. doi:10.1007/s002170100306.
- Scannell, A. G. M., C. Hill, R. P. Ross, S. Marx, W. Hartmeier, and E. K. Arendt. 2000. “Development of Bioactive Food Packaging Materials Using Immobilised Bacteriocins Lacticin 3147 and Nisaplin.” International Journal of Food Microbiology 60: 241–249. doi:10.1016/S0168-1605(00)00314-7.
- Schoeni, J. L., and A. C. Wong. 1994. “Inhibition of Campylobacter Jejuni Colonization in Chicks by Defined Competitive Exclusion Bacteria.” Applied and Environmental Microbiology 60: 1191–1197. doi:10.1128/AEM.60.4.1191-1197.1994.
- Scupham, A. J., J. A. Jones, E. A. Rettedal, and T. E. Weber. 2010. “Antibiotic Manipulation of Intestinal Microbiota to Identify Microbes Associated with Campylobacter Jejuni Exclusion in Poultry.” Applied and Environmental Microbiology 76: 8026–8032. doi:10.1128/AEM.00678-10.
- Shokryazdan, P., M. F. Jahromi, J. B. Liang, and Y. W. Ho. 2017. “Probiotics: From Isolation to Application.” Journal of the American College of Nutrition 36 (8): 666–676. doi:10.1080/07315724.2017.1337529.
- Sibanda, N., A. McKenna, A. Richmond, S. C. Ricke, T. Callaway, A. C. Stratakos, O. Gundogdu, and N. Corcionivoschi. 2018. “A Review of the Effect of Management Practices on Campylobacter Prevalence in Poultry Farms.” Frontiers in Microbiology 9: 2002. doi:10.3389/fmicb.2018.02002.
- Siekkinen, K. M., J. Heikkilä, N. Tammiranta, and H. Rosengren. 2012. “Measuring the Costs of Biosecurity on Poultry Farms: A Case Study in Broiler Production in Finland.” Acta veterinaria Scandinavica 54 (1): 1–8. doi:10.1186/1751-0147-54-12.
- Sirirak, T., and S. P. Voravuthikunchai. 2011. “Eleutherine Americana: A Candidate for the Control of Campylobacter Species.” Poultry Science 90 (4): 791–796. doi:10.3382/ps.2010-01166.
- Skånseng, B., M. Kaldhusdal, B. Moen, A. G. Gjevre, G. S. Johannessen, M. Sekelja, P. Trosvik, and K. Rudi. 2010. “Prevention of Intestinal Campylobacter Jejuni Colonization in Broilers by Combinations of In‐feed Organic Acids.” Journal of Applied Microbiology 109 (4): 1265–1273. doi:10.1111/j.1365-2672.2010.04766.x.
- Skarp, C. P. A., M. L. Hänninen, and H. I. K. Rautelin. 2016. “Campylobacteriosis: The Role of Poultry Meat.” Clinical Microbiology and Infection 22 (2): 103–109. doi:10.1016/j.cmi.2015.11.019.
- Slader, J., G. Domingue, F. Jørgensen, K. McAlpine, R. J. Owen, F. J. Bolton, and T. J. Humphrey. 2002. “Impact of Transport Crate Reuse and of Catching and Processing on Campylobacter and Salmonella Contamination of Broiler Chickens.” Applied and Environmental Microbiology 68 (2): 713–719. doi:10.1128/AEM.68.2.713-719.2002.
- Smith, S., L. L. McV Messam, J. Meade, J. Gibbons, K. McGill, D. Bolton, and P. Whyte. 2016. “The Impact of Biosecurity and Partial Depopulation on Campylobacter Prevalence in Irish Broiler Flocks with Differing Levels of Hygiene and Economic Performance.” Infection Ecology & Epidemiology 6: 31454. doi:10.3402/iee.v6.31454.
- Snelling, W. J., J. P. McKenna, D. M. Lecky, and J. S. G. Dooley. 2005. “Survival of Campylobacter Jejuni in Waterborne Protozoa.” Applied and Environmental Microbiology 71 (9): 5560–5571. doi:10.1128/AEM.71.9.5560-5571.2005.
- Stef, L., C. Julean, A. Cean, D. Mot, D. S. Stef, E. Simiz, F. Simiz, I. Pet, A. Marcu, and N. Corcionivoschi. 2016. “Evaluation of the Administration Effects of Probiotics against Campylobacter Jejuni on the Immune System of Broiler Chickens.” Scientific Papers: Animal Science and Biotechnologies 49 (1): 16–21.
- Stern, N. J., M. R. S. Clavero, J. S. Bailey, N. A. Cox, and M. C. Robach. 1995. “Campylobacter Spp. in Broilers on the Farm and After Transport.” Poultry Science 74 (6): 937–41. doi:10.3382/ps.0740937.
- Stern, N. J. 2008. “Salmonella Species and Campylobacter Jejuni Cecal Colonization Model in Broilers.” Poultry Science 87 (11): 2399–2403. doi:10.3382/ps.2008-00140.
- Stern, N. J., E. A. Svetoch, B. V. Eruslanov, V. V. Perelygin, E. V. Mitsevich, I. P. Mitsevich, V. D. Pokhilenko, V. P. Levchuk, O. E. Svetoch, and B. S. Seal. 2006. “Isolation of a Lactobacillus Salivarius Strain and Purification of Its Bacteriocin, Which Is Inhibitory to Campylobacter Jejuni in the Chicken Gastrointestinal System.” Antimicrobial Agents and Chemotherapy 50 (9): 3111–3116. doi:10.1128/AAC.00259-06.
- Stern, N. J., M. C. Robach, N. A. Cox, and M. T. Musgrove. 2002. “Effect of Drinking Water Chlorination on Campylobacter Spp. Colonization of Broilers.” Avian Diseases 46 (2): 401–404. doi:10.1637/0005-2086(2002)046[0401:EODWCO]2.0.CO;2.
- Stern, N. J., P. Fedorka-Cray, J. S. Bailey, N. A. Cox, S. E. Craven, K. L. Hiett, M. T. Musgrove, S. Ladely, D. Cosby, and G. C. Mead. 2001. “Distribution of Campylobacter Spp. In Selected U.S. Poultry Production and Processing Operations.” Journal of Food Protection 64 (11): 1705–1710. doi:10.4315/0362-028X-64.11.1705.
- Strydom, W. 2015. “International Strategies to Reduce the Incidence of Campylobacter in Broiler Flocks.” Report.
- Svetoch, E. A., B. V. Eruslanov, V. V. Perelygin, E. V. Mitsevich, I. P. Mitsevich, V. N. Borzenkov, and V. P. Levchuk. 2008. “Diverse Antimicrobial Killing by Enterococcus Faecium E 50-52 Bacteriocin.” Journal of Agricultural and Food Chemistry 56 (6): 1942–1948. doi:10.1021/jf073284g.
- Svetoch, E. A., and N. J. Stern. 2010. “Bacteriocins to Control Campylobacter Spp. In Poultry-A Review.” Poultry Science 89 (8): 1763–1768. doi:10.3382/ps.2010-00659.
- Taha-Abdelaziz, K., A. Yitbarek, T. N. Alkie, D. C. Hodgins, L. R. Read, J. S. Weese, and S. Sharif. 2018a. “PLGA-Encapsulated CpG ODN and Campylobacter Jejuni Lysate Modulate Cecal Microbiota Composition in Broiler Chickens Experimentally Challenged with C. Jejuni.” Scientific Reports 8 (1): 1–9. doi:10.1038/s41598-018-30510-w.
- Taha-Abdelaziz, K., D. C. Hodgins, T. N. Alkie, W. Quinteiro-Filho, A. Yitbarek, J. Astill, and S. Sharif. 2018b. “Oral Administration of PLGA-encapsulated CpG ODN and Campylobacter Jejuni Lysate Reduces Cecal Colonization by Campylobacter Jejuni in Chickens.” Vaccine 36 (3): 388–394. doi:10.1016/j.vaccine.2017.11.073.
- Tam, C. C., and S. J. O’Brien. 2016. “Economic Cost of Campylobacter, Norovirus and Rotavirus Disease in the United Kingdom.” PLoS ONE 11 (2): 1–12. doi:10.1371/journal.pone.0138526.
- Tamblyn, K. C., and D. E. Conner. 1997. “Bactericidal Activity of Organic Acids against Salmonella Typhimurium Attached to Broiler Chicken Skint.” Journal of Food Protection 60 (6): 629–633.
- Teng-hern, T. L., C. Kok-gan, and L. L. Han. 2014. “Application of Bacteriophage in Biocontrol of Major Foodborne Bacterial Pathogens.” Journal of Molecular Biology and Molecular Imaging 1 (1): 1–9.
- Thompson, K. L., and T. J. Applegate. 2006. “Feed Withdrawal Alters Small-Intestinal Morphology and Mucus of Broilers.” Poultry Science 85 (9): 1535–1540. doi:10.1093/ps/85.9.1535.
- Thomrongsuwannakij, T., R. Chuanchuen, and N. Chansiripornchai. 2016. “Identification of Competitive Exclusion and Its Ability to Protect against Campylobacter Jejuni in Broilers.” Thai Journal of Veterinary Medicine 46 (2): 279–286.
- Thormar, H., H. Hilmarsson, and G. Bergsson. 2006. “Stable Concentrated Emulsions of the 1-monoglyceride of Capric Acid (Monocaprin) with Microbicidal Activities against the Food-borne Bacteria Campylobacter Jejuni, Salmonella Spp., And Escherichia Coli.” Applied and Environmental Microbiology 72: 522–526. doi:10.1128/AEM.72.1.522-526.2006.
- Ultee, A., M. H. J. Bennik, and R. J. A. E. M. Moezelaar. 2002. “The Phenolic Hydroxyl Group of Carvacrol Is Essential for Action against the Food-Borne Pathogen Bacillus Cereus.” Applied and Environmental Microbiology 68 (4): 1561–1568. doi:10.1128/AEM.68.4.1561-1568.2002.
- Van de Giessen, A. W., J. J. H. C. Tilburg, W. S. Ritmeester, and J. Van Der Plas. 1998. “Reduction of Campylobacter Infections in Broiler Flocks by Application of Hygiene Measures.” Epidemiology and Infection 121: 57–66. doi:10.1017/S0950268898008899.
- Van Deun, K., F. Haesebrouck, F. Van Immerseel, R. Ducatelle, and F. Pasmans. 2008. “Short-chain Fatty Acids and L-lactate as Feed Additives to Control Campylobacter Jejuni Infections in Broilers.” Avian Pathology 37: 379–383. doi:10.1080/03079450802216603.
- Van Gerwe, T., A. Bouma, D. Klinkenberg, J. A. Wagenaar, W. F. Jacobs-reitsma, and A. Stegeman. 2010. “Medium Chain Fatty Acid Feed Supplementation Reduces the Probability of Campylobacter Jejuni Colonization in Broilers.” Veterinary Microbiology 143 (2–4): 314–318. doi:10.1016/j.vetmic.2009.11.029.
- Van Gerwe, T. J. W. M., A. Bouma, W. F. Jacobs-Reitsma, J. Van Den Broek, D. Klinkenberg, J. A. Stegeman, and J. A. P. Heesterbeek. 2005. “Quantifying Transmission of Campylobacter Spp. Among Broilers.” Applied and Environmental Microbiology 71 (10): 5765–5770. doi:10.1128/AEM.71.10.5765-5770.2005.
- Van Immerseel, F., J. B. Russell, M. D. Flythe, I. Gantois, L. Timbermont, F. Pasmans, F. Haesebrouck, and R. Ducatelle. 2006. “The Use of Organic Acids to Combat Salmonella in Poultry: A Mechanistic Explanation of the Efficacy.” Avian Pathology 35 (3): 182–188. doi:10.1080/03079450600711045.
- Vandeplas, S., C. Marcq, R. D. Dauphin, Y. Beckers, P. Thonart, and A. Théwis. 2007. “Contamination of Poultry Flocks by the Human Pathogen Campylobacter Spp. And Strategies to Reduce Its Prevalence at the Farm Level.” Biotechnologie, Agronomie, Société et Environnement 12 (3): 317–334.
- Vandeputte, J., A. Martel, N. V. Rysselberghe, G. Antonissen, M. Verlinden, L. D. Zutter, M. Heyndrickx, F. Haesebrouck, F. Pasmans, and A. Garmyn. 2019. “In Ovo Vaccination of Broilers against Campylobacter Jejuni Using a Bacterin and Subunit Vaccine.” Poultry Science 98 (11): 5999–6004. doi:10.3382/ps/pez402.
- Vieira, A., A. M. Seddon, and A. V. Karlyshev. 2015. “Campylobacter–Acanthamoeba Interactions.” Microbiology (United Kingdom) 161 (2015): 933–947.
- Wagenaar, J. A., D. J. Mevius, and A. H. Havelaar. 2006. “Campylobacter in Primary Animal Production and Control Strategies to Reduce the Burden of Human Campylobacteriosis.” Revue Scientifique Et Technique (International Office of Epizootics) 25 (2): 581–594.
- Wagenaar, J. A., M. A. P. V. Bergen, M. A. Mueller, T. M. Wassenaar, and R. M. Carlton. 2005. “Phage Therapy Reduces Campylobacter Jejuni Colonization in Broilers.” Veterinary Microbiology 109 (3–4): 275–283. doi:10.1016/j.vetmic.2005.06.002.
- Wagenaar, J. A., N. P. French, and A. H. Havelaar. 2013. “Preventing Campylobacter at the Source: Why Is It so Difficult?” Clinical Infectious Diseases 57 (11): 1600–1606. doi:10.1093/cid/cit555.
- Walker, R. I. 2005. “Considerations for Development of Whole Cell Bacterial Vaccines to Prevent Diarrheal Diseases in Children in Developing Countries.” Vaccine 23 (26): 3369–3385. doi:10.1016/j.vaccine.2004.12.029.
- Wesley, I. V., M. Rostagno, H. S. Hurd, and D. W. Trampel. 2009. “Prevalence of Campylobacter Jejuni and Campylobacter Coli in Market-weight Turkeys On-farm and at Slaughter.” Journal of Food Protection 72: 43–48. doi:10.4315/0362-028X-72.1.43.
- WHO. 2013. The Global View of Campylobacteriosis: Report of an Expert Consultation. Utrecht, Netherlands: World Health Organization. https://apps.who.int/iris/handle/10665/80751
- Widders, P. R., L. M. Thomas, K. A. Long, M. A. Tokhi, M. Panaccio, and E. Apos. 1998. “The Specificity of Antibody in Chickens Immunised to Reduce Intestinal Colonisation with Campylobacter Jejuni.” Veterinary Microbiology 64: 39–50. doi:10.1016/S0378-1135(98)00251-X.
- Widders, P. R., R. Perry, W. I. Muir, A. J. Husband, and K. A. Long. 1996. “Immunisation of Chickens to Reduce Intestinal Colonisation with Campylobacter Jejuni.” British Poultry Science 37 (4): 765–778. doi:10.1080/00071669608417906.
- Wirebrand, L., S. Österberg, A. López-Sánchez, F. Govantes, and V. Shingler. 2018. “PP4397/FlgZ Provides the Link between PP2258 c-di-GMP Signalling and Altered Motility in Pseudomonas Putida.” Scientific Reports 8 (1): 1–10. doi:10.1038/s41598-018-29785-w.
- Woodall, C. A., M. A. Jones, P. A. Barrow, J. Hinds, G. L. Marsden, D. J. Kelly, N. Dorrell, B. W. Wren, and D. J. Maskell. 2005. “Campylobacter Jejuni Gene Expression in the Chick Cecum: Evidence for Adaptation to a Low-Oxygen Environment.” Infection and Immunity 73 (8): 5278–5285. doi:10.1128/IAI.73.8.5278-5285.2005.
- Workman, S. N., G. E. Mathison, and M. C. Lavoie. 2008. “An Investigation of Sources of Campylobacter in a Poultry Production and Packing Operation in Barbados.” International Journal of Food Microbiology 121 (1): 106–111. doi:10.1016/j.ijfoodmicro.2007.10.014.
- Wyszyńska, A., A. Raczko, M. Lis, and E. K. Jagusztyn-Krynicka. 2004. “Oral Immunization of Chickens with Avirulent Salmonella Vaccine Strain Carrying C. Jejuni 72Dz/92 cjaA Gene Elicits Specific Humoral Immune Response Associated with Protection against Challenge with Wild-type Campylobacter.” Vaccine 22 (11–12): 1379–1389. doi:10.1016/j.vaccine.2003.11.001.
- Xie, J., R. Zhang, C. Shang, and Y. Guo. 2009. “Isolation and Characterization of a Bacteriocin Produced by an Isolated Bacillus Subtilis LFB112 that Exhibits Antimicrobial Activity against Domestic Animal Pathogens.” African Journal of Biotechnology 8 (20): 5611–5619. doi:10.5897/AJB09.789.
- Younes, I., and M. Rinaudo. 2015. “Chitin and Chitosan Preparation from Marine Sources. Structure, Properties and Applications.” Marine Drugs 13 (3): 1133–1174. doi:10.3390/md13031133.
- Zacharof, M. P., and R. W. Lovitt. 2012. “Investigation of Shelf Life of Potency and Activity of the Lactobacilli Produced Bacteriocins through Their Exposure to Various Physicochemical Stress Factors.” Probiotics and Antimicrobial Proteins 4 (3): 187–197. doi:10.1007/s12602-012-9102-2.
- Zimmer, M., H. Barnhart, U. Idris, and M. D. Lee. 2003. “Detection of Campylobacter Jejuni Strains in the Water Lines of a Commercial Broiler House and Their Relationship to the Strains that Colonized the Chickens.” Avian Diseases 47 (1): 101–107. doi:10.1637/0005-2086(2003)047[0101:DOCJSI]2.0.CO;2.
- Ziprin, R. L., M. E. Hume, C. R. Young, and R. B. Harvey. 2002. “Inoculation of Chicks with Viable Non-Colonizing Strains of Campylobacter Jejuni: Evaluation of Protection against a Colonizing Strain.” Current Microbiology 44 (3): 221–223. doi:10.1007/s00284-001-0088-3.
- Zommiti, M., H. Almohammed, and M. Ferchichi. 2016. “Purification and Characterization of a Novel Anti-Campylobacter Bacteriocin Produced by Lactobacillus Curvatus DN317.” Probiotics and Antimicrobial Proteins 8 (4): 191–201. doi:10.1007/s12602-016-9237-7.
- Zweifel, C., K. D. Scheu, M. Keel, F. Renggli, and R. Stephan. 2008. “Occurrence and Genotypes of Campylobacter in Broiler Flocks, Other Farm Animals, and the Environment during Several Rearing Periods on Selected Poultry Farms.” International Journal of Food Microbiology 125 (2): 182–187. doi:10.1016/j.ijfoodmicro.2008.03.038.