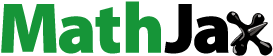
ABSTRACT
1. A 2 × 2 factorial arrangement was used to test the hypothesis that, in pelleted diets, legume starch is digested less rapidly and to a lesser extent than cereal starch, and that increased gelatinisation through extrusion would eliminate the differences between the starch sources. In addition, the trial examined whether a lower ratio of starch to nitrogen disappearance rate (SNDR) could improve feed conversion ratio (FCR).
2. At 17 d of age, male broilers were randomly distributed among four dietary treatments, consisting of either wheat or faba bean starch-rich fraction (FBS) as the sole starch source and pelleting or extrusion as processing methods. Each treatment had 10 replicate pens containing five birds each.
3. Extrusion resulted in a more extensive starch gelatinisation compared to pelleting, as expected.
4. No difference in weight gain at 29 d of age was observed between birds fed starch sources. However, birds fed wheat tended (P = 0.080) to have better FCR than those fed FBS, while the effect of processing methods was insignificant. Thus, there was no interaction between starch source and processing method on FCR.
5. In pelleted diets, FBS had lower and slower starch digestibility compared to wheat in all intestinal segments (P < 0.05). The interaction between starch source and processing method in all intestinal segments (P < 0.001) demonstrated that FBS responded more to gelatinisation through extrusion than did wheat. Thus, differences in starch digestibility between the wheat and FBS were eliminated with extrusion.
6. Feeding extruded diets significantly increased the upper jejunal expression of GLUT1, GLUT2 and SGLT1 compared to pelleted diets, which suggested that glucose absorption was less likely to be a limiting factor for starch utilisation.
7. Pelleting resulted in a lower ratio (P < 0.001) of SNDR compared to extrusion (on average 1.4-fold) but did not improve FCR.
Introduction
Grain legumes such as faba bean (Vicia faba) are considered a good source of protein and energy for poultry. However, faba bean use in broiler diet is limited due to a lower protein content compared to soybean meal (SBM) and to the presence of several anti-nutrients (Jezierny et al. Citation2010). In addition, as the type of crystal structure present in starch granules may influence its digestibility (Ao et al. Citation2007; Zhang et al. Citation2006), in vitro studies (Hoover and Zhou Citation2003; Li et al. Citation2018; Weurding Citation2002) showed that type-C starch from legumes is more slowly and to a lesser extent digested than type-A starch from cereals (Sun et al. Citation2006). Similarly, studies with broiler chickens showed that legume starch is generally more resistant to intestinal degradation than cereal starch (Carre et al. Citation1991; Carré et al. Citation1998; Wiseman Citation2006). It is known that the ratio of amylose to amylopectin is higher in legumes than in cereals (Ambigaipalan et al. Citation2011; Sandhu and Lim Citation2008; Simsek et al. Citation2013). Due to its compact and linear structure, amylose has a lower surface area for amylase (Thorne et al. Citation1983) and thus a lower digestibility in vitro and in vivo (Regmi et al. Citation2011; Topping et al. Citation1997). The significant progress in plant breeding, combined with the use of mechanical (dehulling) treatment were shown to have great potential in improving the nutritional value of faba beans (Crépon et al. Citation2010). Other hydrothermal processes like pelleting and extrusion have been reported to enhance the digestibility of faba beans due to heat-induced physico-chemical changes such as starch gelatinisation (Diaz et al. Citation2006; Hejdysz et al. Citation2016; Lacassagne et al. Citation1988). Air classification is another processing technique for the dry separation of particles of different densities and shapes, for example from finely ground dehulled faba bean, into a protein concentrate (FBP; light fraction) and a starchy flour (FBS; dense fraction) (Vose et al. Citation1976). These fractions can be used as a protein supplement or a concentrated energy source in broiler diets.
While high starch digestibility is always desirable, it has been proposed that feeding slowly digestible starch may improve FCR of broilers (Del Alamo et al. Citation2009; Liu and Selle Citation2015; Weurding Citation2002). These researchers hypothesised that, compared to slowly digested starch, rapidly digested starch would not provide enough energy in the form of glucose to the enterocytes in the lower part of the small intestine. Consequently, a larger proportion of amino acids will be used as an energy source for the enterocytes instead of for muscle growth. Contrary, due to its longer supply of glucose, slowly digested starch may spare amino acid oxidation, and result in improved broiler performance (Weurding et al. Citation2003a). Results from the studies addressing the aforementioned hypothesis were, however, contradictory (Del Alamo et al. Citation2009; Weurding et al. Citation2003b). According to Liu and Selle (Citation2015), starch digestion dynamics should be treated in combination with that of protein because of the intricate relationship between these macronutrients and their effect on FCR. The ratio of starch to nitrogen disappearance rate (SNDR) is an approach to quantify starch and nitrogen digestive dynamics and its relation to feed efficiency (Sydenham et al. Citation2017). For instance, some studies found that broiler performance improved linearly with a lower ratio of SNDR, while in others, the relationship was quadratic (Sydenham et al. Citation2017).
The hypothesis tested was that in pelleted diet, faba bean starch (FBS) will be digested less rapidly and to a lesser extent than wheat and that extrusion will increase the availability of starch and eliminate the difference in starch digestibility between the two starch sources. In addition, the hypothesis that low ratio of starch to nitrogen disappearance rate (SNDR) may improve feed conversion ratio (FCR) was also tested.
Materials and methods
According to Polish law and the EU directive (no 2010/63/EU), the experiment conducted within the study does not require approval of the Local Ethical Committee for Experiments on Animals in Poznań.
Processing of main ingredients and experimental diets
The dehulled faba beans (FB) were cracked using a roller mill (DT900-12; CPM-Roskamp, Waterloo, IA, United States) with an 8 mm gap between the rolls and cleaned from dust using a pre-cleaner Damas Vibam type 1013 (Damas A/S, Faaborg, Denmark). Next, the beans were milled with a Contraplex 630 C pin mill (Hosokawa Alpine, Augsburg, Germany) and finally the flour was air-classified using an Air Classifier 500 ATP (Hosokawa Alpine, Augsburg, Germany) to produce a light protein-rich fraction and a heavy starch-rich fraction. The wheat was pin-milled similarly to the FB without further processing. The chemical composition and particle size of the wheat and the FBS are presented in and , respectively.
Table 1. Analysed chemical composition (g/kg) of the wheat, dehulled faba bean parent meal (FBPM), and the air-classified faba bean starch-rich fraction (FBS)
Figure 1. Particle-size distribution of the starch sources. Volume weighted mean: 50 µm for the FBS and 240 µm for the wheat. Surface weighted mean: 21 µm for the FBS and 26 µm for the wheat
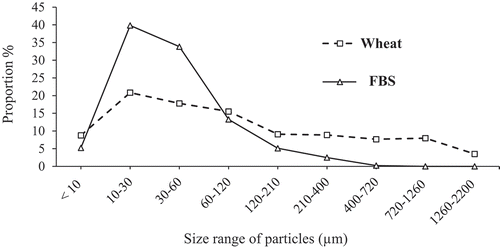
The SBM was ground to pass through a 1-mm sieve in a hammer mill (Münch-Edelstahl, Wuppertal, Germany licenced by Bliss, USA, 18.5 kW, 3000 RPM) before being mixed with other ingredients. Experimental diets were processed at the Centre for Feed Technology, Norwegian University of Life Sciences, Ås, Norway, and were formulated to be isonitrogenous and isoenergetic and to meet or exceed Ross 308 strain average recommendations (Aviagen Citation2019) for the starter and grower periods for major nutrients ().
Table 2. Experimental diet composition, analysed and calculated nutrient content
Table 3. Analysed amino acid1 composition (g/kg DM) of the diets
The diets contained titanium dioxide (TiO2), as an indigestible marker. The mash was steam-conditioned in a double pass pellet-press conditioner (Münch-Edelstahl, Wuppertal, Germany) at 81°C and then pelleted using a pellet press (Münch-Edelstahl, Wuppertal, Germany, 1.2 t/h, 2 × 17 kW, RMP 350) equipped with a 3 mm die (42 mm thickness), at a production rate of 400 kg/h for the wheat-based diet. Due to the low flowability of FBS, the production rate was decreased to 200 kg/h to reduce excessive friction within the pellet die and to avoid pellet mill blockage. Specific energy consumption values were 38 and 77 kWh/t for the wheat- and FBS-based diets, respectively. Post-pelleting temperatures were 89°C and 94°C for the wheat- and FBS-based diet, respectively, and were measured by collecting a sample of hot pellets from immediately below the pellet press into an insulated box fitted with a thermometer. The extruded diet was steam heated at 89°C in an extruder pre-conditioner (Bühler BCTC 10, Uzwil, Switzerland) prior to processing in a co-rotating twin-screw extruder (Bühler BCTG 62/20 D, 5 sections, 72 kW DC, Uzwil, Switzerland) fitted with 12 dies x 3 mm and with a feeder rate of 145 kg/h for the wheat- and FBS-based diets. The temperatures in the five sections of the extruder were 92°C, 112°C, 95°C, 90°C and 64°C for the wheat diet and 95°C, 110°C, 100°C, 96°C and 64°C for the FBS diet. Specific mechanical energy values (KWh/t) were 65 and 62, and die temperatures were 91°C and 95°C for the wheat- and FBS-based diets, respectively. Moisture content during extrusion was kept at around 290 g/kg by addition of steam and water (ambient temperature) in amounts of 60 g/kg and 100 g/kg in the conditioner. During pelleting, around 43 g/kg of steam were added in the conditioner to achieve an average total moisture of 150 g/kg.
Birds, housing and management
One-day-old male broilers (Ross 308) were allocated to 40 floor pens (1 x 1 m) bedded with chopped wheat straw (7–15 cm length). The pens were arranged in the centre of an environmentally controlled broiler house (PIAST PASZE Sp. z o.o., Experimental Unit no. 0616, Olszowa, Poland) that contained 9000 birds of the same age and strain as those in the experiment. A temperature of 33°C was maintained during the first week, then reduced by 3–4°C weekly to a minimum temperature of 21°C. The birds were maintained on a commercial-pelleted diet produced by Piast Pasze feed mill (Lewkowiec, Poland) until 16 d, and fresh water was provided ad libitum throughout the experimental period. At 17 d, the birds were randomly distributed among four dietary treatments with 10 replicate pens per treatment and five birds per pen. Due to a low amount of available raw material, the quantity of experimental diet produced would have been insufficient if more birds were to be used per pen. The four treatments consisted of a 2 × 2 factorial arrangement with wheat- or FBS as starch sources and pelleting or extrusion as processing methods.
Performance and sample collection
The birds and the feed were weighed on a pen basis on d 17 and 29. At 30 d, 20 randomly selected birds per treatment (two birds/pen) were weighed, killed by cervical dislocation and the gizzard removed, freed from surrounding fat and weighed full and empty. As will be described below, samples for light microscopy, enzyme activity and RNA analysis were taken from one bird and the rest of the digesta from both birds were collected and pooled for digestibility analysis. The jejunum and ileum were clamped at the end of the duodenal loop, at Meckel’s diverticulum and at the ileocaecal junction to prevent the passage of contents along the intestine, then weighed. Each segment was then divided into two parts of equal length and the digesta was expressed by gentle manipulation into a pre-weighed plastic container and stored at −20°C until analysis. About 500 mg of digesta from the upper and lower jejunum (Uj and Lj) and ileum (Ui and Li) were transferred to a 2 ml Sarstedt tube containing 1.6 ml fixation solution (1.25% glutaraldehyde, 2% paraformaldehyde in 0.1 M PIPES-buffer at pH 7.4) and kept at 4°C for 48 h. After centrifugation at 3600 rpm for 4 min, the fixation solution was carefully removed using disposable pipette and then 1.5 ml of buffer solution (0.4 M PIPES-buffer at pH 7.4) was added to each tube, vortexed and then stored at 4°C until light microscopy analysis. Around 200 mg of representative samples of digesta from the Lj were transferred to 2 ml Sarstedt tube, frozen on dry ice then stored at −80°C until enzyme activity analysis. A cross-section (2 cm in length) was taken from the midpoint of the Uj, rinsed with ice-cold PBS and then cut into three sections of less than 4 mm in thickness. These sections were transferred to a corresponding 2 ml Sarstedt tube containing 1.6 ml RNAlater solution (Merck, Germany) and kept at 4°C for 48 h. The tubes were then stored at −80°C until RNA extraction.
RNA extraction, cDNA synthesis, real-time qPCR, primers and gene expression calculation
Total RNA was extracted using the RNeasy Plus Universal Kits (Qiagen, Hilden, Germany) following the manufacturer’s instructions. The concentration and quality were assessed using a NanoDrop 8000 Spectrophotometer (Thermo Fisher Scientific, Waltham, MA, USA) and 2100 Bioanalyzer Instrument (Agilent Technologies, Santa Clara, CA, USA). Before cDNA synthesis, all samples were normalised to the same RNA concentration. The cDNA synthesis was performed using the AffinityScript QPCR cDNA Synthesis kit (Agilent Technologies, Santa Clara, CA, USA) according to the manufacturer’s guidelines. The real-time qPCR reactions were performed in 96-well plate. Eight randomly selected samples out of 10 replicates were used per treatment and were analysed in duplicate. Each reaction was carried out in a total volume of 20 µl containing 10 µl of LightCycler 480 SYBR Green I Master (Roche Diagnostics, Basel, Switzerland), 3 µL H2O, 2 µl primer mix (forward & reverse, 5 µM) and 5 µL cDNA (diluted 1:10). The qPCR reactions were analysed using the CFX96 Touch Real-Time PCR Detection System (Bio-Rad, Hercules, CA, USA). The cycling conditions were 95°C in 3 min followed by 40 cycles of 95°C in 10 s and 60–64°C (depending on the primers) in 30 sec. To confirm amplification specificity, a melting curve analysis was performed. The primers used in the current experiment were sourced from several studies and are shown with the selected genes in . Fold change in gene expression was calculated using the relative quantification (2−ΔΔCT) method (Livak and Schmittgen Citation2001). Cycle threshold (CT) values of each group were normalised against the housekeeping genes glyceraldehyde 3-phosphate dehydrogenase (GAPDH) and hydroxymethylbilane synthase (HMBS), and the average ΔCT of the control group (wheat-pelleted) served as the calibrator for each target gene in the treatment groups.
Table 4. Sequences of primers used for quantitative real-time PCR
Chemical analyses
Feed samples were ground in a cutting mill (Pulverisette 19, Fritsch Industriestr 8, 55 743 Idar-Oberstein, Germany) through a 0.5 mm sieve. Gross energy (GE) was determined using an adiabatic bomb calorimeter (Parr 6400, Moline, USA) standardised with benzoic acid. Dry matter and ash content of the feed were determined after drying overnight at 105°C and after 6 h ashing at 550°C, respectively. Nitrogen content was determined by the Dumas method using a Vario El Cube (Elementar Analysensysteme GmbH, Hanau, Germany 2016). Amino acids in the diets were analysed on a Biochrom 30 amino acid analyser (Biochrom Ltd., Cambridge, UK). Ether extract was determined after extraction with 80% petroleum ether and 20% acetone in an Accelerated Solvent Extractor from Dionex (ASE200; Sunnyvale, CA, USA). Fibre content was determined using a fibre analyser system (Ankom200; ANKOM Technologies, Fairport, NY, USA) with filter bags (Ankom F58; ANKOM Technologies). Starch content was analysed enzymatically based on the use of thermostable α-amylase and amylo-glucosidase (Mccleary et al. Citation1994) and TiO2 content was determined as described by Short et al. (Citation1996). Freeze-dried jejunal and ileal contents were pulverised using a mortar and pestle, and analysed in duplicates for starch (without 80% ethanol washing), nitrogen and TiO2 as described above. Intestinal digesta samples from the Lj were prepared as described by Pérez De Nanclares et al. (Citation2017) for enzyme activities analysis. Amylase and trypsin activities were assayed colourimetrically using amylase and trypsin commercial assay kits (Abcam, Cambridge, UK) according to manufacturer’s instructions and were expressed as unit/g jejunal chyme. For light microscopy analysis, a representative digesta sample (100 μl) was taken from each tube and transferred to a glass slide to which 100 μl of iodine solution was added for staining. The mixture was covered by a cover glass and starch granules were observed at 100 x magnification at room temperature using a light microscope (Leica DM6B) equipped with LAS X analysis software, which was used for image capturing and counting by visual evaluation of slides. The particle size distribution of the wheat and FBS was determined by the laser diffraction method using a Malvern Mastersizer 2000 (Malvern Instruments Ltd., Worcestershire, UK) as described by Hetland et al. (Citation2002). The degree of starch gelatinisation (DG; as a proportion of total starch) was measured by differential scanning calorimetry (DSC 823e Module, Mettler-Toledo, Switzerland) as described by Kraugerud and Svihus (Citation2011).
Calculations
The apparent digestibility coefficients of starch and nitrogen were calculated using the following formula:
Apparent digestibility coefficient =
was the ratio of the nutrient to TiO2 in the diet or in the digesta.
Apparent disappearance rates of starch and nitrogen along the intestinal tract were calculated using the following formula (Sydenham et al. Citation2017): Apparent disappearance rate (g/bird/d) = dietary concentration of the nutrient (g/kg) x feed intake over the final 24 h of feeding (g/bird) x digestibility coefficient of the nutrient. Starch: nitrogen disappearance rate (SNDR) ratios in the small intestine were calculated from these data.
Statistical analysis
Statistical analyses were performed using general linear models in R version 2.3.2. A two-way analysis of variance (ANOVA) was performed to determine the main effects and interactions of starch sources and processing methods (as independent variables) on growth parameters, digestive characteristics, nutrient digestibility, enzyme activities and gene expression. Means were separated by Tukey post-hoc test. Differences between means were considered significant at P < 0.05 and tendencies if P values were between 0.05 and 0.10. Pen was used as the experimental unit for all data.
Results
Growth performance
Body weight gain was not affected by the starch source, but extrusion increased (P = 0.032) weight gain compared to pelleting, partly due to the interaction effect on feed intake (P = 0.042) where birds given FBS consumed more feed only when the diets were extruded (). There was no significant effect of processing method on FCR, but birds fed FBS tended (P = 0.080) to have poorer FCR than those fed wheat. There was no difference in the relative weight of the gizzard or the jejunum and ileum between treatments (data not shown).
Table 5. The effect of starch source and processing method on the growth performance1 of male broilers from 17 to 29 d
Apparent starch digestibility
In all intestinal segments, starch digestibility was significantly lower for the FBS compared to the wheat only in the pelleted diet (). This resulted in a significant interaction between starch source and processing method in the Uj, Ui and Li, and in a tendency for an interaction (P = 0.057) in the Lj. Starch disappearance rate followed the same pattern as starch digestibility (data not shown).
Table 6. The effect of starch source and processing method on starch digestibility along the intestinal tract of 30-d-old male broilers1.
Microscopy analysis of intestinal digesta
The amount of starch granules decreased as digesta progressed from the Uj to the Lj, and the amount of starch granules was higher in pelleted than extruded diets as expected ()).
Figure 2. a) Light microscopy images showing starch granules (black dots) in digesta from the upper and lower jejunum (Uj, Lj) and ileum (Ui, Li) collected from birds fed pelleted diets based on either wheat or faba bean starch (FBS). Each column represents one replicate pen with samples collected from one bird per pen. Magnification x 10. b) Light microscopy images showing starch granules (black dots) in digesta from the upper and lower jejunum (Uj, Lj) and ileum (Ui, Li) collected from birds fed extruded diets based on either wheat or faba bean starch (FBS). Each column represents one replicate pen with samples collected from one bird per pen. Magnification x 10
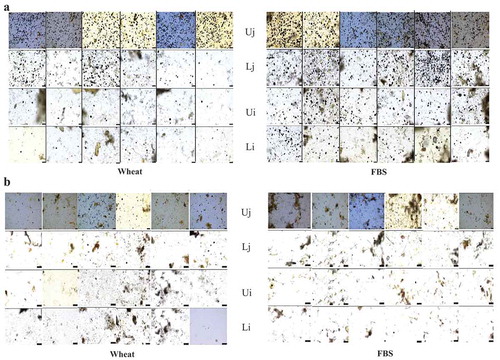
Gene expression of glucose transporters in the upper jejunum
There was a significant upregulation of glucose transporter 1 (GLUT1;1.6-fold), glucose transporter 2 (GLUT2; 1.8-fold) and sodium glucose transporter 1 (SGLT1; 1.4-fold) genes in the Uj of bird fed extruded diets compared to those fed pelleted diets (). No interaction between processing and starch source was observed on the gene expression of any of the selected glucose transporters.
Table 7. The effect of starch source and processing method on the expression1 of glucose transporter 1 (GLUT1), glucose transporter 2 (GLUT2) and sodium glucose transporter 1 (SGLT1) in the upper jejunum of 30-d-old male broilers.2
Apparent nitrogen digestibility
Compared to extruded diets, pelleted diets had a higher (P = 0.012) nitrogen digestibility in the Uj, while neither starch source nor processing method (P > 0.05) affected nitrogen digestibility in the Lj or Ui (). However, nitrogen digestibility in the Li was significantly higher (P = 0.027) in birds fed the FBS-based diet compared with those fed the wheat-based diet. Nitrogen disappearance rate did not differ between treatments (data not shown).
Table 8. The effect of starch source and processing method on the apparent nitrogen digestibility along the intestinal tract of 30-d-old male broilers.1
Ratio of starch: nitrogen disappearance rate (SNDR)
In the Uj and Lj, birds fed pelleted diets had a lower (P < 0.001) ratio of SNDR (by 1.75- and 1.25-fold, respectively) compared to those fed extruded diets (). In the ileum, significant (P < 0.001) interactions were observed, where a lower ratio of SNDR was noted in birds given FBS only when the diet was pelleted.
Table 9. The effect of starch source and processing method on the ratios of starch and nitrogen disappearance rates (SNDR) along the intestinal tract of 30-d-old male broilers.1
Enzyme activities
There was a tendency for higher (P = 0.055) amylase activity in jejunal digesta of birds fed FBS compared to those fed wheat (). Feeding wheat increased (P = 0.003) trypsin activity compared to feeding the FBS diet. Trypsin activity was also influenced by feed processing. Thus, birds fed extruded diets had higher (P = 0.019) trypsin activity than those fed pelleted diets.
Table 10. The effect of starch source and processing method on the activities of digestive enzymes (U/g chyme) in the digesta collected from the lower jejunum of 30-d-old male broilers.1
Discussion
As expected, and in agreement with other studies (Itani and Svihus Citation2019; Zimonja and Svihus Citation2009), extrusion resulted in a more extensive starch gelatinisation compared to pelleting. Although pelleted diets had a similar amount of gelatinised starch (209 and 207 g/kg in the wheat and FBS diet, respectively), extrusion increased the proportion of gelatinised starch in the FBS (943 g/kg) compared to the wheat diet (715 g/kg). Hasjim et al. (Citation2013) reported that rice flour samples with larger particle sizes had a greater physical barrier to heat and water diffusion than smaller particles. Due to air classification, FBS was finer than wheat, with a volume weighted mean of 50 compared to 240 µm and a surface weighted mean of 21 compared to 26 µm, respectively, and this may have contributed to the higher degree of gelatinisation in the FBS extruded diet.
Starch digestibility and disappearance rates confirmed that legume starch in pelleted diets was more slowly and digested to a lesser extent than cereal starch. In contrast, extrusion increased starch digestibility and disappearance rate compared to pelleting for the FBS, probably due to the extensive destruction of the crystalline structure of the granules as indicated by the higher extent of gelatinisation. It should be highlighted that, in pelleted diets, more than 90% of the starch was digested in the jejunum, the major site for starch digestion. This value was higher than what is reported in general (80%) for jejunal starch digestibility (Del Alamo et al. Citation2009; Stefanello et al. Citation2015). This may have been due to differences in the processing method and ingredient characteristics between the current experiment and other studies. In extruded diets, starch was digested faster and to a higher extent in the jejunum, with values exceeding 97%.
The higher expression of glucose transporters in birds fed extruded diets may be an adaptive mechanism to maximise the absorption of luminal (SGLT1) glucose generated from rapidly digested starch, and to increase the basolateral (GLUT1 and GLUT2) transport of glucose into the blood. Importantly, this finding suggested that glucose absorption is less likely to be a limiting factor for starch utilisation in broiler chickens (Gilbert et al. Citation2007; Suvarna et al. Citation2005).
Contrary to the findings of Itani and Svihus (Citation2019), there was no difference in starch digestibility between pelleted and extruded wheat diet in the current experiment. Thus, it can be suggested that the presence of other factors may have maximised the digestion of wheat starch in the pelleted diet and masked the effect of increased gelatinisation in the extruded wheat diet. To avoid the confounding effect of different grinding methods, the wheat was finely ground using a pin mill in the same way as the faba beans. Studies have shown that a well-developed gizzard can efficiently grind coarse wheat particles to very fine particle sizes, thereby enhancing starch utilisation (Amerah et al. Citation2007; Svihus Citation2006). Because the diets in this experiment did not stimulate gizzard development, it suggested that the degree of fineness of the wheat, which was much higher than in any previously reported work, has outweighed the need for a well-functioning gizzard to grind the wheat to facilitate digestion. As a result, wheat starch digestibility was almost complete, even in the pelleted diet. It is worth mentioning that decreasing wheat particle size does not always increase starch digestibility (Abdollahi et al. Citation2019), and in other instances, may negatively affect starch digestibility due to increased digesta viscosity (Yasar Citation2003) although not consistently (Amerah et al. Citation2008). Thus, besides particle size, factors like method of grinding, wheat characteristics, enzymes addition and more should be taken into consideration.
The resistance of legume starch to digestion compared to cereal starch has been highlighted by the difference in particle size distribution. FBS was finer than the wheat, with volume weighted means of 50 and 240 µm and surface weighted means of 21 and 26 µm, respectively. Fine grinding of starchy ingredients increases the susceptibility of starch to enzymatic hydrolysis (Angelidis et al. Citation2016; Lee et al. Citation2019). However, even with a high ileal starch digestibility for the pelleted FBS, wheat starch was still more digestible. This explained the significant interaction between starch source and processing method on starch digestibility throughout the intestinal segments.
Amylase activity tended to be higher in birds fed the FBS diet, which may have been a reflection of the higher amount of starch found in the intestinal contents, as indicated by the digestibility coefficients of starch, and as previously reported (Engberg et al. Citation2004; Karasov and Hume Citation1997). Despite this, starch digestibility in FBS was still lower than in wheat, which suggested inadequate amylase secretion. Alternatively, amylase may not be a limiting factor per se, but rather the amylase-resistant nature of legume starch when minimally gelatinised. The significantly higher starch digestibility in the extruded compared to the pelleted FBS diet corroborated this suggestion, especially that amylase activity did not differ between the two treatments.
Because both diets contained similar amount of SBM, nitrogen digestibility (NiD) was more likely affected by the protein fraction of the starch source. Accordingly, ileal NiD was higher in FBS diets compared to wheat diets, which indicated that bean protein (possibly due to the finer particle size of FBS) was more accessible to digestion compared to that from wheat. Corroborating this, feeding broilers finely milled pea seeds significantly improved the apparent ileal protein digestibility (89.5 vs. 70.2%) compared to coarse milling, probably due to the larger surface area of fine particles available to digestive enzymes (Crévieu et al. Citation1997). In contrast, compared to coarse grinding, fine grinding of wheat did not improve ileal protein digestibility in a wheat-SBM diet fed to young broilers (Péron et al. Citation2005). Moreover, dehulling, low-tannin content and heat treatment were described as contributing to the significant increase in protein digestibility of legumes, particularly faba bean and peas (Alonso et al. Citation2000; Carré et al. Citation1987; Crépon et al. Citation2010). The lower trypsin activity in the FBS-fed birds may explain the reduced need for excess enzymes when the digestibility of the substrate is high (Murugesan et al. Citation2014).
Feeding FBS, a source of slowly digestible starch compared to wheat, seemed not to improve FCR. In fact, there was a tendency for FBS to impair feed efficiency compared to wheat. This was not in line with previous findings (Liu and Selle Citation2015; Weurding Citation2002). In addition, extrusion did not worsen FCR compared to pelleting, despite more rapid starch digestion. Hejdysz et al. (Citation2017) found that offering peas in extruded form (up to 500 g/kg diet) improved broiler performance, nutrient and energy utilisation and FCR compared to the raw form. Although apparent metabolisable energy (AME) was not measured in the current study, Truong et al. (Citation2016) reported that slowly digestible starch may improve AME and nitrogen corrected AME (AMEn). However, a recent experiment from the same lab showed significant improvements in AME and AMEn with 45% inclusion of rapidly digested purified maize-starch in a maize-SBM-based diet (Moss et al. Citation2018). Although equivocal, the impact of slowly digestible starch on FCR may vary depending on its amount, site and extent of digestion and on its digestion rate (kd). For instance, Weurding et al. (Citation2003b) reported that feeding diets with slowly digestible starch (kd = 1.05 h−1) to broilers resulted in 1.9% improvement in FCR compared to diets with rapidly digestible starch (kd = 1.99 h−1). On the other hand, a diet with a starch kd of 1.8 h−1 impaired broiler performance (FCR = 1.668) compared to that of 2.17 h−1 (FCR = 1.572) or even 2.56 h−1 (FCR = 1.579) (Del Alamo et al. Citation2009). It is clear that the relationship between slowly digested starch and FCR is not straightforward, as other dietary- or bird-related factors can influence the results.
As indicated earlier, while some studies found that FCR improved linearly with a lower ratio of SNDR, other studies proposed that this relationship is quadratic (Sydenham et al. Citation2017). Truong et al. (Citation2017), however, did not detect any difference in the performance of broilers fed six varieties of sorghum exhibiting different ratios of SNDR in all intestinal segments. In the current experiment, pelleting had a narrower ratio of SNDR compared to extrusion, particularly in the upper and lower jejunum, but no significant difference in FCR was detected.
Based on the expression level and type of nutrient transporters in the chicken intestine, Gilbert et al. (Citation2007) concluded that the jejunum is the primary site of sugar assimilation, while the ileum is a more important site for amino acid assimilation. In addition, based on the activity of mucosal enzymes, Uni (Citation2006) concluded that the jejunum has a higher capacity to digest disaccharides than the ileum, and Uni et al. (Citation1998) found that the RNA:DNA ratio was higher in the jejunum than the ileum, indicating significantly higher tissue activity in the former. Based on the above, slower starch digestion (low ratio of SNDR) in the upper small intestine may not be optimal for such a demanding tissue or for FCR in general. Thus, an adequate supply of glucose in the jejunum may spare more amino acids from oxidation and increase their appearance in the portal circulation, as seen reported by Yin et al. (Citation2019). This agreed with Wu (Citation1998), who emphasised that extensive catabolism of dietary essential amino acids in the first pass in the small intestine can significantly impair nutritional efficiency. In line with this, slowly digestible starch (resistant starch) significantly reduced glucose and amino acids net absorption into the portal vein in growing pigs. Accordingly, it has been suggested that resistant starch may increase the catabolism of amino acids by the small intestine and result in an impaired feed efficiency (Li et al. Citation2008). A significant portion of dietary amino acids was reported to be catabolised to meet the energy demands of the intestinal digestive and absorptive processes (Fuller and Reeds Citation1998), particularly glutamine and glutamate (Blasco et al. Citation2005; Reeds et al. Citation2000; Souba Citation1993; Stoll et al. Citation1999). Thus, it can be hypothesised that if the majority of amino acid oxidation is shifted to the ileum, a relatively less demanding tissue in terms of digestion and absorption compared to the jejunum (Gao et al. Citation2017), the negative impact on FCR may be lower. Corroborating this, Karunaratne et al. (Citation2018) found that rapid starch digestion improved FCR based on a positive correlation between gain:feed ratio and starch digestibility in the upper and lower jejunum of broilers fed wheat diets in mash form. Clearly, these findings are inconsistent and contradictory due to the complexity of the hypothesis and to the presence of confounding factors.
In conclusion, FBS in a pelleted broiler diet had lower starch digestibility and a slower disappearance rate compared to wheat in all intestinal segments. This magnitude was more pronounced in the jejunum. The interaction between starch source and processing method in all intestinal segments demonstrated that legume starch responded more to gelatinisation through extrusion than cereal starch. As a result, differences in starch digestibility between the wheat and FBS were eliminated with extrusion. The current data did not support the hypothesis that lower ratio of starch to nitrogen disappearance rate improved feed efficiency in broiler chickens.
Disclosure statement
No potential conflict of interest was reported by the authors.
Additional information
Funding
References
- Abdollahi, M. R., F. Zaefarian, H. Hunt, M. N. Anwar, D. G. Thomas, and V. Ravindran. 2019. “Wheat Particle Size, Insoluble Fibre Sources and Whole Wheat Feeding Influence Gizzard Musculature and Nutrient Utilisation to Different Extents in Broiler Chickens.” Journal of Animal Physiology and Animal Nutrition 103 (1): 146–161. doi:10.1111/jpn.13019.
- Alonso, R., A. Aguirre, and F. Marzo. 2000. “Effects of Extrusion and Traditional Processing Methods on Antinutrients and in Vitro Digestibility of Protein and Starch in Faba and Kidney Beans.” Food Chemistry 68 (2): 159–165. doi:10.1016/S0308-8146(99)00169-7.
- Ambigaipalan, P., R. Hoover, E. Donner, Q. Liu, S. Jaiswal, R. Chibbar, K. Nantanga, and K. Seetharaman. 2011. “Structure of Faba Bean, Black Bean and Pinto Bean Starches at Different Levels of Granule Organization and Their Physicochemical Properties.” Food Research International 44 (9): 2962–2974. doi:10.1016/j.foodres.2011.07.006.
- Amerah, A., V. Ravindran, R. Lentle, and D. Thomas. 2007. “Feed Particle Size: Implications on the Digestion and Performance of Poultry.” World’s Poultry Science Journal 63 (3): 439–455. doi:10.1017/S0043933907001560.
- Amerah, A., V. Ravindran, R. Lentle, and D. Thomas. 2008. “Influence of Particle Size and Xylanase Supplementation on the Performance, Energy Utilisation, Digestive Tract Parameters and Digesta Viscosity of Broiler Starters.” British Poultry Science 49 (4): 455–462. doi:10.1080/00071660802251749.
- Angelidis, G., S. Protonotariou, I. Mandala, and C. M. Rosell. 2016. “Jet Milling Effect on Wheat Flour Characteristics and Starch Hydrolysis.” Journal of Food Science and Technology 53 (1): 784–791. doi:10.1007/s13197-015-1990-1.
- Ao, Z., S. Simsek, G. Zhang, M. Venkatachalam, B. L. Reuhs, and B. R. Hamaker. 2007. “Starch with a Slow Digestion Property Produced by Altering Its Chain Length, Branch Density, and Crystalline Structure.” Journal of Agricultural and Food Chemistry 55 (11): 4540–4547. doi:10.1021/jf063123x.
- Aviagen. 2019. ROSS Nutrition Specifications. Aviagen, Scotland, UK. https://en.aviagen.com/assets/Tech_Center/Ross_Broiler/RossBroilerNutritionSpecs2019-EN.pdf
- Blasco, M., M. Fondevila, and J. A. Guada. 2005. “Inclusion of Wheat Gluten as a Protein Source in Diets for Weaned Pigs.” Animal Research 54 (4): 297–306. doi:10.1051/animres:2005026.
- Carré, B., J. Melcion, J. Widiez, and P. Biot. 1998. “Effects of Various Processes of Fractionation, Grinding and Storage of Peas on the Digestibility of Pea Starch in Chickens.” Animal Feed Science and Technology 71 (1–2): 19–33. doi:10.1016/S0377-8401(97)00140-5.
- Carré, B., R. Escartin, J. Melcion, M. Champ, G. Roux, and B. Leclercq. 1987. “Effect of Pelleting and Associations with Maize or Wheat on the Nutritive Value of Smooth Pea (Pisum Sativum) Seeds in Adult Cockerels.” British Poultry Science 28 (2): 219–229. doi:10.1080/00071668708416956.
- Carre, B., E. Beaufils, and J. P. Melcion. 1991. “Evaluation of Protein and Starch Digestibility and Energy Value of Pelleted or Unpelleted Pea Seeds from Winter or Spring Cultivars in Adult and Young Chickens.” Journal of Agricultural and Food Chemistry 39 (3): 468–472. doi:10.1021/jf00003a008.
- Crépon, K., P. Marget, C. Peyronnet, B. Carrouee, P. Arese, and G. Duc. 2010. “Nutritional Value of Faba Bean (Vicia Faba L.) Seeds for Feed and Food.” Field Crops Research 115 (3): 329–339. doi:10.1016/j.fcr.2009.09.016.
- Crévieu, I., B. Carré, A. M. Chagneau, J. Guéguen, and J. P. Melcion. 1997. “Effect of Particle Size of Pea (Pisum sativum L) Flours on the Digestion of Their Proteins in the Digestive Tract of Broilers.” Journal of the Science of Food and Agriculture 75 (2): 217–226. doi:10.1002/(SICI)1097-0010(199710)75:2<217::AID-JSFA867>3.0.CO;2-O.
- Dalgaard, T. S., K. Skovgaard, L. R. Norup, J. Pleidrup, A. Permin, T. W. Schou, D. F. Vadekær, G. Jungersen, and H. R. Juul-Madsen. 2015. “Immune Gene Expression in the Spleen of Chickens Experimentally Infected with Ascaridia Galli.” Veterinary Immunology and Immunopathology 164 (1–2): 79–86. doi:10.1016/j.vetimm.2015.01.003.
- Del Alamo, A. G., M. Verstegen, L. Den Hartog, P. P. De Ayala, and M. Villamide. 2009. “Wheat Starch Digestion Rate Affects Broiler Performance.” Poultry Science 88 (8): 1666–1675. doi:10.3382/ps.2008-00502.
- Diaz, D., M. Morlacchini, F. Masoero, M. Moschini, G. Fusconi, and G. Piva. 2006. “Pea Seeds (Pisum Sativum), Faba Beans (Vicia Faba Var. Minor) and Lupin Seeds (Lupinus Albus Var. Multitalia) as Protein Sources in Broiler Diets: Effect of Extrusion on Growth Performance.” Italian Journal of Animal Science 5 (1): 43–53. doi:10.4081/ijas.2006.43.
- Engberg, R. M., M. S. Hedemann, S. Steenfeldt, and B. B. Jensen. 2004. “Influence of Whole Wheat and Xylanase on Broiler Performance and Microbial Composition and Activity in the Digestive Tract.” Poultry Science 83 (6): 925–938. doi:10.1093/ps/83.6.925.
- Fuller, M., and P. J. Reeds. 1998. “Nitrogen Cycling in the Gut.” Annual Revue of Nutrition 18 (1): 385–411. doi:10.1146/annurev.nutr.18.1.385.
- Gao, T., M. Zhao, L. Zhang, J. Li, L. Yu, P. LV, F. Gao, and G. Zhou. 2017. “Effect of in Ovo Feeding of L-arginine on the Hatchability, Growth Performance, Gastrointestinal Hormones, and Jejunal Digestive and Absorptive Capacity of Posthatch Broilers.” Journal of Animal Science 95 (7): 3079–3092. doi:10.2527/jas.2016.0465.
- Gilbert, E., H. Li, D. Emmerson, K. W. E. B. B. JR, and E. Wong. 2007. “Developmental Regulation of Nutrient Transporter and Enzyme mRNA Abundance in the Small Intestine of Broilers.” Poultry Science 86 (8): 1739–1753. doi:10.1093/ps/86.8.1739.
- Hasjim, J., E. Li, and S. Dhital. 2013. “Milling of Rice Grains: Effects of Starch/flour Structures on Gelatinization and Pasting Properties.” Carbohydrate Polymers 92 (1): 682–690. doi:10.1016/j.carbpol.2012.09.023.
- Hejdysz, M., S. Kaczmarek, and A. Rutkowski. 2016. “Extrusion Cooking Improves the Metabolisable Energy of Faba Beans and the Amino Acid Digestibility in Broilers.” Animal Feed Science and Technology 212: 100–111. doi:10.1016/j.anifeedsci.2015.12.008.
- Hejdysz, M., S. Kaczmarek, M. Adamski, and A. Rutkowski. 2017. “Influence of Graded Inclusion of Raw and Extruded Pea (Pisum Sativum L.) Meal on the Performance and Nutrient Digestibility of Broiler Chickens.” Animal Feed Science and Technology 230: 114–125. doi:10.1016/j.anifeedsci.2017.05.016.
- Hetland, H., B. Svihus, and V. Olaisen. 2002. “Effect of Feeding Whole Cereals on Performance, Starch Digestibility and Duodenal Particle Size Distribution in Broiler Chickens.” British Poultry Science 43 (3): 416–423. doi:10.1080/00071660120103693.
- Hoover, R., and Y. Zhou. 2003. “In Vitro and in Vivo Hydrolysis of Legume Starches by α-amylase and Resistant Starch Formation in Legumes—a Review.” Carbohydrate Polymers 54 (4): 401–417. doi:10.1016/S0144-8617(03)00180-2.
- Itani, K., and B. Svihus. 2019. “Feed Processing and Structural Components Affect Starch Digestion Dynamics in Broiler Chickens.” British Poultry Science 1–10. doi:10.1080/00071668.2018.1556388.
- Jezierny, D., R. Mosenthin, and E. Bauer. 2010. “The Use of Grain Legumes as A Protein Source in Pig Nutrition: A Review.” Animal Feed Science and Technology 157 (3–4): 111–128. doi:10.1016/j.anifeedsci.2010.03.001.
- Karasov, W. H., and I. D. Hume. 1997. “Vertebrate Gastrointestinal System.” Comprehensive Physiology 1: 409–480. doi:10.1002/cphy.cp130107.
- Karunaratne, N., D. Abbott, P. Hucl, R. Chibbar, C. Pozniak, and H. CLASSEN. 2018. “Starch Digestibility and Apparent Metabolizable Energy of Western Canadian Wheat Market Classes in Broiler Chickens.” Poultry Science 97 (8): 2818–2828. doi:10.3382/ps/pey115.
- Kheravii, S. K., R. A. Swick, M. Choct, and S.-B. Wu. 2018. “Upregulation of Genes Encoding Digestive Enzymes and Nutrient Transporters in the Digestive System of Broiler Chickens by Dietary Supplementation of Fiber and Inclusion of Coarse Particle Size Corn.” BMC Genomics 19 (1): 208. doi:10.1186/s12864-018-4592-2.
- Kraugerud, O., and B. Svihus. 2011. “Tools to Determine the Degree of Starch Gelatinization in Commercial Extruded Salmon Feeds.” Journal of the World Aquaculture Society 42 (6): 914–920. doi:10.1111/j.1749-7345.2011.00522.x.
- Lacassagne, L., M. Francesch, B. Carré, and J. Melcion. 1988. “Utilization of Tannin-containing and Tannin-free Faba Beans (Vicia Faba) by Young Chicks: Effects of Pelleting Feeds on Energy, Protein and Starch Digestibility.” Animal Feed Science and Technology 20 (1): 59–68. doi:10.1016/0377-8401(88)90127-7.
- Lee, Y.-T., M.-J. Shim, H.-K. Goh, C. Mok, and P. Puligundla. 2019. “Effect of Jet Milling on the Physicochemical Properties, Pasting Properties, and in Vitro Starch Digestibility of Germinated Brown Rice Flour.” Food Chemistry 282: 164–168. doi:10.1016/j.foodchem.2018.07.179.
- Li, P., S. Dhital, B. Zhang, X. He, X. Fu, and Q. Huang. 2018. “Surface Structural Features Control in Vitro Digestion Kinetics of Bean Starches.” Food Hydrocolloids 85: 343–351. doi:10.1016/j.foodhyd.2018.07.007.
- Li, T.-J., Q.-Z. Dai, Y.-L. Yin, J. Zhang, R.-L. Huang, Z. Ruan, Z. Deng, and M. Xie. 2008. “Dietary Starch Sources Affect Net Portal Appearance of Amino Acids and Glucose in Growing Pigs.” Animal 2 (5): 723–729. doi:10.1017/S1751731108001614.
- Liu, S., and P. Selle. 2015. “A Consideration of Starch and Protein Digestive Dynamics in Chicken-meat Production.” World’s Poultry Science Journal 71 (2): 297–310. doi:10.1017/S0043933915000306.
- Livak, K. J., and T. D. Schmittgen. 2001. “Analysis of Relative Gene Expression Data Using Real-time Quantitative PCR and the 2− ΔΔCT Method.” methods 25 (4): 402–408. doi:10.1006/meth.2001.1262.
- Mccleary, B., V. Solah, and T. GIBSON. 1994. “Quantitative Measurement of Total Starch in Cereal Flours and Products.” Journal of Cereal Science 20 (1): 51–58. doi:10.1006/jcrs.1994.1044.
- Moss, A. F., C. J. Sydenham, A. Khoddami, V. D. Naranjo, S. Y. Liu, and P. H. Selle. 2018. “Dietary Starch Influences Growth Performance, Nutrient Utilisation and Digestive Dynamics of Protein and Amino Acids in Broiler Chickens Offered Low-protein Diets.” Animal Feed Science and Technology 237: 55–67. doi:10.1016/j.anifeedsci.2018.01.001.
- Mott, C., P. Siegel, K. Webb JR, and E. Wong. 2008. “Gene Expression of Nutrient Transporters in the Small Intestine of Chickens from Lines Divergently Selected for High or Low Juvenile Body Weight.” Poultry Science 87 (11): 2215–2224. doi:10.3382/ps.2008-00101.
- Murugesan, G. R., L. F. Romero, and M. E. Persia. 2014. “Effects of Protease, Phytase and a Bacillus Sp. Direct-fed Microbial on Nutrient and Energy Digestibility, Ileal Brush Border Digestive Enzyme Activity and Cecal Short-chain Fatty Acid Concentration in Broiler Chickens.” PloS One 9 (7): e101888. doi:10.1371/journal.pone.0101888.
- Pérez De Nanclares, M., M. Trudeau, J. Hansen, L. Mydland, P. Urriola, G. Shurson, C. Åkesson, N. Kjos, M. Arntzen, and M. Øverland. 2017. “High-fiber Rapeseed Co-product Diet for Norwegian Landrace Pigs: Effect on Digestibility.” Livestock Science 203: 1–9. doi:10.1016/j.livsci.2017.06.008.
- Péron, A., D. Bastianelli, F.-X. Oury, J. Gomez, and B. Carré. 2005. “Effects of Food Deprivation and Particle Size of Ground Wheat on Digestibility of Food Components in Broilers Fed on a Pelleted Diet.” British Poultry Science 46 (2): 223–230. doi:10.1080/00071660500066142.
- Reeds, P. J., D. G. Burrin, B. Stoll, and F. Jahoor. 2000. “Intestinal Glutamate Metabolism.” The Journal of Nutrition 130 (4): 978S–982S. doi:10.1093/jn/130.4.978S.
- Regmi, P. R., B. U. Metzler-Zebeli, M. G. Gänzle, T. A. Van Kempen, and R. T. Zijlstra. 2011. “Starch with High Amylose Content and Low in Vitro Digestibility Increases Intestinal Nutrient Flow and Microbial Fermentation and Selectively Promotes Bifidobacteria in Pigs.” The Journal of Nutrition 141 (7): 1273–1280. doi:10.3945/jn.111.140509.
- Sandhu, K. S., and S.-T. Lim. 2008. “Digestibility of Legume Starches as Influenced by Their Physical and Structural Properties.” Carbohydrate Polymers 71 (2): 245–252. doi:10.1016/j.carbpol.2007.05.036.
- Short, F., P. Gorton, J. Wiseman, and K. Boorman. 1996. “Determination of Titanium Dioxide Added as an Inert Marker in Chicken Digestibility Studies.” Animal Feed Science and Technology 59 (4): 215–221. doi:10.1016/0377-8401(95)00916-7.
- Simsek, S., K. Whitney, and J.-B. OHM. 2013. “Analysis of Cereal Starches by High-performance Size Exclusion Chromatography.” Food Analytical Methods 6 (1): 181–190. doi:10.1007/s12161-012-9424-4.
- Souba, W. W. 1993. “Intestinal Glutamine Metabolism and Nutrition.” The Journal of Nutritional Biochemistry (USA) 4 (1): 2–9. doi:10.1016/0955-2863(93)90013-M.
- Stefanello, C., S. Vieira, G. Santiago, L. Kindlein, J. Sorbara, and A. Cowieson. 2015. “Starch Digestibility, Energy Utilization, and Growth Performance of Broilers Fed Corn-soybean Basal Diets Supplemented with Enzymes.” Poultry Science 94 (10): 2472–2479. doi:10.3382/ps/pev244.
- Stoll, B., D. G. Burrin, J. Henry, H. Yu, F. Jahoor, and P. J. Reeds. 1999. “Substrate Oxidation by the Portal Drained Viscera of Fed Piglets.” American Journal of Physiology-Endocrinology and Metabolism 277 (1): E168–E175. doi:10.1152/ajpendo.1999.277.1.E168.
- Sun, T., H. Lærke, H. Jørgensen, and K. Knudsen. 2006. “The Effect of Extrusion Cooking of Different Starch Sources on the in Vitro and in Vivo Digestibility in Growing Pigs.” Animal Feed Science and Technology 131 (1–2): 67–86. doi:10.1016/j.anifeedsci.2006.02.009.
- Suvarna, S., V. Christensen, D. Ort, and W. Croom. 2005. “High Levels of Dietary Carbohydrate Increase Glucose Transport in Poult Intestine.” Comparative Biochemistry and Physiology Part A: Molecular & Integrative Physiology 141 (3): 257–263. doi:10.1016/j.cbpb.2005.03.008.
- Svihus, B. 2006. “The Role of Feed Processing on Gastrointestinal Function and Health in Poultry.” In Avian Gut Function in Health and Disease, edited by G. C. Perry, 183–194. UK, Wallingford: CAB International.
- Sydenham, C. J., H. H. Truong, A. F. Moss, P. H. Selle, and S. Y. Liu. 2017. “Fishmeal and Maize Starch Inclusions in Sorghum-soybean Meal Diets Generate Different Responses in Growth Performance, Nutrient Utilisation, Starch and Protein Digestive Dynamics of Broiler Chickens.” Animal Feed Science and Technology 227: 32–41. doi:10.1016/j.anifeedsci.2017.03.003.
- Teng, P.-Y., S. Yadav, F. L. De Souza Castro, Y. H. Tompkins, A. L. Fuller, and W. K. Kim. 2020. “Graded Eimeria Challenge Linearly Regulated Growth Performance, Dynamic Change of Gastrointestinal Permeability, Apparent Ileal Digestibility, Intestinal Morphology, and Tight Junctions of Broiler Chickens.” Poultry Science 99 (9): 4203–4216. doi:10.1016/j.psj.2020.04.031.
- Thorne, M. J., L. Thompson, and D. Jenkins. 1983. “Factors Affecting Starch Digestibility and the Glycemic Response with Special Reference to Legumes.” The American Journal of Clinical Nutrition 38 (3): 481–488. doi:10.1093/ajcn/38.3.481.
- Topping, D. L., J. M. Gooden, I. L. Brown, D. A. Biebrick, L. McGrath, R. P. Trimble, M. Choct, and R. J. Illman. 1997. “A High Amylose (Amylomaize) Starch Raises Proximal Large Bowel Starch and Increases Colon Length in Pigs.” The Journal of Nutrition 127 (4): 615–622. doi:10.1093/jn/127.4.615.
- Truong, H. H., K. A. Neilson, B. V. Mcinerney, A. Khoddami, T. H. Roberts, D. J. Cadogan, S. Y. LIU, and P. H. Selle. 2017. “Comparative Performance of Broiler Chickens Offered Nutritionally Equivalent Diets Based on Six Diverse,‘tannin-free’sorghum Varieties with Quantified Concentrations of Phenolic Compounds, Kafirin, and Phytate.” Animal Production Science 57 (5): 828–838. doi:10.1071/AN16073.
- Truong, H. H., S. Y. Liu, and P. H. Selle. 2016. “Starch Utilisation in Chicken-meat Production: The Foremost Influential Factors.” Animal Production Science 56 (5): 797–814. doi:10.1071/AN15056.
- Uni, Z. 2006. “Early Development of Small Intestinal Function.” In Avian Gut Function in Health and Disease, edited by G. C. Perry, 29–42. UK, Wallingford: CAB International.
- Uni, Z., S. Ganot, and D. Sklan. 1998. “Posthatch Development of Mucosal Function in the Broiler Small Intestine.” Poultry Science 77 (1): 75–82. doi:10.1093/ps/77.1.75.
- Vose, J., M. Basterrechea, P. Gorin, A. Finlayson, and C. Youngs. 1976. “Air Classification of Field Peas and Horsebean Flours: Chemical Studies of Starch and Protein Fractions.” Cereal Chem 53 (6): 928–936. http://www.aaccnet.org/publications/cc/backissues/1976/Documents/chem53_928.pdf.
- Weurding, R. E. 2002. “Kinetics of Starch Digestion and Performance of Broiler Chickens“. Ph.D. Dissertation, Wageningen University.
- Weurding, R. E., H. Enting, and M. Verstegen. 2003a. “The Effect of Site of Starch Digestion on Performance of Broiler Chickens.” Animal Feed Science and Technology 110 (1–4): 175–184. doi:10.1016/S0377-8401(03)00219-0.
- Weurding, R. E., H. Enting, and M. Verstegen. 2003b. “The Relation between Starch Digestion Rate and Amino Acid Level for Broiler Chickens.” Poultry Science 82 (2): 279–284. doi:10.1093/ps/82.2.279.
- Wiseman, J. 2006. “Variations in Starch Digestibility in Non-ruminants.” Animal Feed Science and Technology 130 (1): 66–77. doi:10.1016/j.anifeedsci.2006.01.018.
- Wu, G. 1998. “Intestinal Mucosal Amino Acid Catabolism.” The Journal of Nutrition 128 (8): 1249–1252. doi:10.1093/jn/128.8.1249.
- Yasar, S. 2003. “Performance, Gut Size and Ileal Digesta Viscosity of Broiler Chickens Fed with a Whole Wheat Added Diet and the Diets with Different Wheat Particle Sizes.” International Journal of Poultry Science 2 (1): 75–82. https://www.researchgate.net/profile/Sulhattin_Yasar/publication/26557398_Performance_Gut_Size_and_Ileal_Digesta_Viscosity_of_Broiler_Chickens_Fed_with_a_Whole_Wheat_Added_Diet_and_the_Diets_with_Different_Wheat_Particle_Sizes/links/00b7d51ac820ca8412000000/Performance-Gut-Size-and-Ileal-Digesta-Viscosity-of-Broiler-Chickens-Fed-with-a-Whole-Wheat-Added-Diet-and-the-Diets-with-Different-Wheat-Particle-Sizes.pdf.
- Yin, D., P. H. Selle, A. F. Moss, Y. Wang, X. Dong, Z. Xiao, Y. Guo, and J. Yuan. 2019. “Influence of Starch Sources and Dietary Protein Levels on Intestinal Functionality and Intestinal Mucosal Amino Acids Catabolism in Broiler Chickens.” Journal of Animal Science and Biotechnology 10 (1): 26. doi:10.1186/s40104-019-0334-9.
- Zhang, G., M. Venkatachalam, and B. R. Hamaker. 2006. “Structural Basis for the Slow Digestion Property of Native Cereal Starches.” Biomacromolecules 7 (11): 3259–3266. doi:10.1021/bm060343a.
- Zimonja, O., and B. Svihus. 2009. “Effects of Processing of Wheat or Oats Starch on Physical Pellet Quality and Nutritional Value for Broilers.” Animal Feed Science and Technology 149 (3): 287–297. doi:10.1016/j.anifeedsci.2008.06.010.