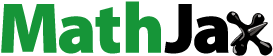
ABSTRACT
1. The objective of this study was to evaluate performance of a commercial loop-mediated isothermal amplification (LAMP) method as an alternative method for the detection of Campylobacter spp. in primary production samples, poultry rinses and raw poultry products, as compared to the US Department of Agriculture Food Inspection Service Microbiology Laboratory Guide Book PCR reference method, MLG 41A.
2. The Campylobacter spp. LAMP was used in conjunction with a ready-to-use enrichment broth that does not require microaerophilic incubation. After enrichment, boot swabs from poultry farms, carcase rinses and raw poultry products were tested by the LAMP method and the MLG 41A PCR method.
3. The ready-to-use enrichment broth enabled the growth of Campylobacter spp. within 22 to 28 hours under aerobic incubation conditions. The LAMP method enabled Campylobacter detection in the enriched samples of various poultry matrices and had equivalent sensitivity and specificity to the MLG 41A PCR method.
4. No significant difference (95% confidence interval) was found between the alternative and the MLG 41A PCR method, as determined by probability of detection analysis, except for neutralising buffered peptone water post-chill rinsates. For the post-chill neutralising buffered peptone water rinsates, the LAMP method had significantly higher confirmed portions.
Introduction
Campylobacter spp., comprising Gram-negative, thermotolerant, and microaerophilic bacteria, is globally recognised as a major cause of foodborne infection in humans. The Center for Disease Control in the USA (CDC) estimates that about 1.3 million illnesses occur each year in US due to campylobacteriosis (CDC Citation2017) resulting in significant economic burden (Batz et al. Citation2012; Hoffmann et al. Citation2015). The majority of campylobacteriosis is caused by Campylobacter jejuni (over 90%) and to a lesser extent by C. coli (2.3%) and C. lari (0.22%) (CDC Citation2017; Bolton Citation2015; EFSA and ECDC Citation2018). Campylobacter spp. infection is a significant problem in the poultry industry throughout the world (Batz et al. Citation2012; EFSA and ECDC Citation2018; Skarp et al. Citation2016). Raw poultry products are contaminated with Salmonella and Campylobacter spp. due to rupturing of contaminated gut contents (small intestine and caeca) during slaughter operations (Berrang et al. Citation2000; Rouger et al. Citation2017; Perez-Arnedo and Gonzalez-Fandos Citation2019). In addition, contamination of bird’s feathers and skin on the farm can introduce these bacteria to the processing facilities (Berrang et al. Citation2000). Proper sanitary operations and use of antimicrobial interventions are necessary to minimise contamination during slaughter and processing of the carcases into parts and in comminuted products.
Government agencies, such as the US Department of Agriculture (USDA) Food Safety and Inspection Service (FSIS), have implemented pathogen reduction performance standards for Salmonella and Campylobacter spp. levels in raw chicken parts, ground chicken and ground turkey (USDA FSIS Citation2015, Citation2019). Performance standards are used by the USDA FSIS to assess establishment performance for various poultry products. This has necessitated continued and increased surveillance of Campylobacter spp. in the poultry industry. While the advances in rapid methods such as immunoassays and PCR have enabled accurate detection of foodborne pathogens (Jasson et al. Citation2010; Wiedmann et al. Citation2014; Mangal et al. Citation2016; Souii et al. Citation2016), the current rapid methods for detection of Campylobacter using traditional enrichment broth require two to three days to provide results. Hence, there is a need for a faster and simpler technology for foodborne pathogen detection.
Loop-mediated isothermal amplification (LAMP) can amplify DNA under isothermal conditions (60 to 65°C) with high specificity and sensitivity in 60 min or less (Notomi et al. Citation2000; Mori and Notomi Citation2009; Mori et al. Citation2013; Notomi et al. Citation2015). Compared to PCR, LAMP performs better as it has greater ability to amplify DNA in the presence of interfering substances often found in clinical, food and environmental samples (Kaneko et al. Citation2007; Plutzer and Karanis Citation2009; Niessen et al. Citation2013; Yang et al. Citation2014, Citation2018). LAMP has been coupled to a bioluminescent assay for the detection of amplified products, enabling simultaneous detection and amplification, which provides real-time results in a short run time (Gandelman et al. Citation2010). The LAMP-bioluminescent method offers a simpler, faster and streamlined approach to pathogen detection (Lim et al. Citation2015; Sarowska et al. Citation2016; Yang et al. Citation2016; Hu et al. Citation2017, Citation2018).
Campylobacter spp. are fastidious organisms requiring microaerophilic conditions, a longer growing time, narrow temperature range and specialised media (Davis and DiRita Citation2008). A ready-to-use (RTU) enrichment medium that does not require additional supplements and antibiotics and allows growth without modified atmosphere incubation enables easier detection. The proprietary broth, 3M™ Campylobacter Enrichment Broth (CEB) enables growth under aerobic incubation with shorter incubation times (22 to 28 h) than conventional media, such as blood-free Bolton enrichment broth (BF-BEB) (AOAC PTM, Citation2018). The LAMP assay, 3M™ Molecular Detection Assay 2 – Campylobacter (MDA2CAM96) uses bioluminescence for detection of Campylobacter spp. and provides results in 60 minutes (AOAC PTM, Citation2018). The assay specifically detects three thermotolerant species; C. coli, C. jejuni and C. lari (and not C. upsaliensis), without species differentiation (AOAC PTM, Citation2018).
The objective of this study was to evaluate the performance of the LAMP method for the detection of Campylobacter spp. in poultry rinses and raw poultry products compared to the USDA FSIS Microbiology Laboratory Guidebook (MLG) reference PCR method (MLG 41A, BAX® System Real-time PCR assay) (MLG 41.04 Citation2016; MLG 41A.00 Citation2016). In addition, the LAMP method was evaluated for detection of Campylobacter spp. in primary production samples.
Materials and methods
The enrichment conditions for the LAMP method (MDA2CAM96, 3M Food Safety, St. Paul, MN, USA) (AOAC PTM Citation2018) and the MLG 41.04 reference method (MLG 41.04 Citation2016) for various matrices are summarised in .
Table 1. Sample preparation and enrichment protocols for Campylobacter spp. detection
The CEB (3M Food Safety) and BF-BEB (Oxoid Ltd., Hampshire, UK) were prepared following manufacturer’s instructions. For CEB, 41 g of powder was suspended in 1.0 l of sterile purified water and used within 24 h of preparation. For BF-BEB, double-strength broth with double strength supplements (2X BF-BEB) was prepared and used within 48 h of preparation. For the LAMP method, samples were enriched in CEB as per recommended protocols and analysed using the LAMP assay. For all samples analysed with LAMP, randomly selected samples were additionally analysed with the external amplification control, 3M™ Molecular Detection Matrix Control (3M Food Safety), to assess potential sample interference during the amplification reaction. For the MLG 41.04 reference method, samples were enriched in BF-BEB as per recommended protocol and analysed using the BAX® System Real-time PCR assay for Campylobacter spp. (Hygiena LLC, Camarillo, CA, USA) as described in MLG 41A.00 (MLG 41A.00 Citation2016). All samples analysed by the molecular method were culture-confirmed per the MLG 41.04 reference method (MLG 41.04 Citation2016). Total aerobic counts in all matrices were also determined by serially diluting the sample homogenates in Butterfield’s buffer and plating on 3M™ Petrifilm™ Aerobic Count Plates (3M Food Safety). The plates were incubated for 48 h at 35 ± 1°C and colonies were enumerated.
Sample collection and preparation
Inoculum preparation
Three isolates, C. jejuni (ATCC® 33291™), C. coli (ATCC® 43478™), and C. lari (ATCC® 35221™) were used in this study. The ATCC strains obtained as KWIK-STICK™ product (Microbiologics, St. Cloud, MN, USA) were streaked onto tryptic soy agar (TSA) with 5% sheep blood (Edge Biologicals, Memphis, TN, USA) and incubated for 24 h at 42°C in a cell culture tri-gas incubator (HeraCell 240i CO2 Incubator, Thermo Fisher Scientific, Asheville, NC, USA) under microaerophilic atmosphere (10% CO2, 5% O2 and 85% N2). To prepare each of Campylobacter spp. inoculum, an isolated colony from TSA with 5% sheep blood was inoculated into 10 ml of 1X BF-BEB with supplements (Oxoid Ltd.) using a sterile inoculating loop and incubated for 24 h at 42°C in the cell culture tri-gas incubator under microaerophilic atmosphere. After incubation, serial 10-fold dilutions of cultures were prepared in buffered peptone water (BPW) and 100 μl of the dilutions was spread plated on TSA with 5% sheep blood and incubated at 42°C for 24 h in the cell culture tri-gas incubator, under a microaerophilic atmosphere. The colonies on the plates were counted, and an average count of each dilution was used to determine the appropriate amount of inoculum added to each sample.
Boot swabs
Thirty samples of boot swabs were collected in one farm (n = 30) using sterile boot swab (cotton-poly blend fabric sock style boot cover) pre-moistened with double-strength skim milk (Solar Biologics Inc., Massena, NY, USA). Briefly, a technician placed disposable plastic boot cover over the shoes to protect personal clothing and prevent cross-contamination of the sample from shoe sole carryover. Then, the technician removed a sterile pre-moistened boot swab from the twirl-tie bag and placed it over the boot cover and walked the entire length of one side of the poultry house. After sample collection, the boot swab was removed, immediately transferred to the original twirl-tie bag and transported to the laboratory for further processing. A total of 100 ml CEB were added to each of the bags. Six of the boot swabs were used as controls (uninoculated) to detect natural contamination and 24 boot swabs were inoculated with 100 μl of Campylobacter suspension, described above, at 1 to 10 CFU/bag. All the boot swabs were incubated under aerobic conditions at 41.5°C for 24 h.
Poultry sample collection
Whole chicken carcase and parts rinsates and raw poultry products were collected from a local commercial broiler abattoir. The samples were collected from the processing line at three sampling points (rehang, post-chill intervention and poultry parts processing). The USDA FSIS has implemented a change in medium from BPW to nBPW for carcase rinsates (USDA FSIS Citation2016). Both BPW and nBPW rinsates were evaluated in an unpaired study to compare the LAMP method against the MLG 41A PCR reference method (MLG 41.04 Citation2016; MLG 41A.00 Citation2016) for detection of Campylobacter spp. Control samples (uninoculated) were set up for each sample type to screen for natural contamination with wild type Campylobacter spp. isolates.
Rinsate collection
To collect carcase or parts rinsates, carcases or 1.8 kg of parts were collected from the poultry processing line, allowed to drain for at least 1 min and aseptically transferred into a sterile poultry rinse bag (Nasco, Fort Atkinson, WI, USA). A total of 400 ml of cold (2–8°C) BPW (3M Food Safety) or nBPW (Neogen, Lansing, MI, USA) was added to each bag by pouring approximately half of the solution into the interior cavity and half onto the exterior of the carcase. The bag was closed to prevent leakage and the carcase or parts were rinsed by gently shaking for 1 min using a 1-foot arm-arcing motion. The rinsate was aseptically transferred back into the original container, capped tightly and labelled. Rinsates were then transported in a cooler with ice to the laboratory and used for further testing.
Rinsate testing
The flow chart for detection of Campylobacter spp. in rehang and post-chill rinsates is shown in . A total of 240 rinsates were analysed (120 for BPW and 120 for nBPW). For each buffer, 30 rinsates from rehang carcases, 15 from post-chill carcases and 15 from post-chill parts were analysed by each of the method (60 rinsates for each method for each buffer).
LAMP method
For the LAMP method, 30 ml of the rinsate was added to a sterile 200 ml Whirl-Pak® bag (Nasco) and processed for enrichment as follows. Six rehang carcase, six post-chill carcase and six post-chill parts rinsates were set up as controls (uninoculated) to screen for natural contamination. Twenty-four rehang carcase rinsates (eight for each of C. jejuni, C. coli or C. lari) and nine post-chill carcases (three for each of C. jejuni, C. coli or C. lari) and nine post-chill parts (three for each of C. jejuni, C. coli or C. lari) rinsates were inoculated with 100 µl of bacterial suspension, described above, at 1 to 5 CFU per 30 ml of rinsates. Each of the control and inoculated rinsate was then combined with 30 ml of CEB. The bag was gently mixed, rolled down to squeeze air to minimise air space, closed and incubated aerobically at 41.5°C for 24 h.
MLG PCR method
For the MLG 41A PCR method, 30 ml of rinsate was transferred to a sterile 70 ml vented Falcon tissue culture flask (Corning Inc., Coning, NY, USA). Control and inoculated samples were set up similarly as described for the LAMP method. Each of the control and inoculated rinsate was then combined with 30 ml of 2X BF-BEB (Oxoid Ltd.). The tissue culture flasks were closed, the medium was gently mixed with the rinsate and incubated at 42°C for 46 hours in the cell culture tri-gas incubator under microaerophilic atmosphere.
Raw poultry products
An unpaired study compared the LAMP method for detection of Campylobacter spp. in raw poultry products against the MLG 41A PCR reference method (MLG 41.04 Citation2016; MLG 41A.00 Citation2016). Control samples (uninoculated) were set up for each product mixture to screen for natural contamination with wild type isolates of Campylobacter spp.
Raw poultry product mixture preparation
Three types of raw products, mechanically separated chicken (MSC), marinated chicken breast and ground turkey were portioned to obtain a 325 g sample, which was transferred to a 4 l sterile Whirl-Pak® filter bag (Nasco). The samples were prepared as described in the MLG 41.04 reference method (MLG 41.04 Citation2016). A total of 1.625 l of cold BPW (2–8°C) was added to each bag and the sample was gently massaged to mix with the media and minimise introduction of air. The raw poultry product mixtures were further processed as described below.
Raw poultry product mixture testing
The flow chart for detection of Campylobacter spp. in raw poultry products is shown in . A total of 60 product mixtures (30 for each method) were analysed for raw poultry products.
LAMP method
For the LAMP method, 30 ml of the raw poultry product mixture was added to a sterile 200 ml Whirl-Pak® bag (Nasco) and processed for enrichment as follows. Two product mixture for each of ground turkey or marinated chicken and 10 product mixtures for MSC were set up as controls (uninoculated) to assess natural contamination of Campylobacter spp. For ground turkey and marinated chicken, each additional product mixture (30 ml), was inoculated with 100 μl of bacterial suspension, described above, at 1 to 2 CFU per sample of C. jejuni, C. coli or C. lari. For MSC samples (30 ml), three product mixtures were inoculated with about 1 to 2 CFU per sample of C. coli or C. lari and four product mixtures with C. jejuni. Each of the control and inoculated product mixture was then combined with 30 ml of CEB. The bag was gently mixed, rolled down to squeeze the air out and minimise headspace, closed and incubated aerobically at 41.5°C for 24 h.
MLG PCR method
For the MLG 41A PCR method, 30 ml of product mixture was transferred to a sterile 70 ml vented Falcon tissue culture flask (Corning Inc.). Control and inoculated samples were set up similarly as described for the LAMP method. Each of the control and inoculated product mixture was then combined with 30 ml of 2X BF-BEB (Oxoid Ltd.). The tissue culture flasks were closed and incubated at 42°C for 46 h in the cell culture tri-gas incubator under a microaerophilic atmosphere.
Campylobacter spp. detection
For the LAMP assay, 20 μl of sample after enrichment in CEB was collected and processed for detection using the 3M™ Molecular Detection System following the manufacturer’s instructions (3M Food Safety). For the MLG 41A PCR method, samples were analysed after enrichment in BF-BEB by the BAX® System Real-time assay for Campylobacter spp. following the manufacturer’s instructions (Hygiena, LLC). In addition, BF-BEB enrichments were analysed by the LAMP assay.
Regardless of the presumptive results obtained by the two methods, primary enrichments from both the LAMP and the MLG 41A PCR reference method were culture-confirmed as per the MLG 41.04 Culture reference method using growth on Campy Cefex agar, microscopy and latex agglutination on colonies (MLG 41.04 Citation2016). Each of the enriched samples were streaked onto a Campy Cefex agar (Edge Biologicals) and incubated at 42 ± 1°C for 48 h under microaerophilic conditions. Suspensions of typical colonies in 0.85% saline were examined under oil immersion using phase-contrast microscopy (Nikon Eclipse E400 microscope, Nikon Instruments, Melville, NY, USA) for typical Campylobacter spp. corkscrew morphology and darting motility. The same colony suspension used for microscopic examination was tested by latex agglutination immunoassay using Microgen® Bioproducts Campylobacter spp. latex identification test (Hardy Diagnostics, Santa Maria, CA, USA) following the manufacturer’s instructions.
Detection rates and statistical analyses
The alternative method (presumptive) results were compared to the gold standard culture reference method to determine false positive and false negative rates (Eijkelkamp et al. Citation2009). Presumptive results obtained for both carcase rinsates and raw poultry products for Campylobacter spp. detection with the LAMP and the MLG 41A PCR reference method were compared with the confirmed culture results and sensitivity (false negative rate) and specificity (false positive rate) (Eijkelkamp et al. Citation2009) was calculated using Equationequation 1(1)
(1) and Equation2
(2)
(2) , respectively.
True positives: culture-confirmed positive results;
True negatives: culture-confirmed negative results;
False negatives: presumptive negative results, positive culture results;
False positives: presumptive positive results, negative culture results.
For rinsate and product samples, the probability of detection (POD) was computed for both the LAMP method (POD alternative, PODa) and the MLG 41A PCR reference method (POD reference, PODr) and used as a statistical model to validate LAMP method (Wehling et al. Citation2011). The POD was calculated as the ratio of number of positives to total number of samples tested for each method at each analyte concentration. dPOD, the differential between the probability of detection (POD) for the alternative method (PODa) and the POD for the reference method (PODr) was computed. The lower and upper confidence limits (95% confidence interval) for PODa and PODr was calculated and used to calculate the lower and upper limit for dPOD as outlined in Wehling et al. (Citation2011). If the confidence interval of a dPOD did not contain zero, then the difference was deemed statistically significant at the 5% level (Wehling et al. Citation2011).
Results
Primary production samples
The average background aerobic flora in boot swabs was 7.75 log10 CFU/ml. Campylobacter spp. were not detected in the control (uninoculated) boot swabs by the LAMP method (MDA2CAM96) and there was no growth on Campy Cefex agar, confirming the presumptive negative results. However, infection was detected in 23 out of 24 boot swabs inoculated with Campylobacter spp. and enriched in CEB by the LAMP method. All LAMP positive samples plated on Campy Cefex agar had typical colonies and colonies showed corkscrew morphology and positive agglutination test confirming presumptive result from the same sample ().
Table 2. Detection of Campylobacter spp. in primary production boot swabs using the LAMP method
Rehang carcase rinsates
BPW rinsates
The average background aerobic flora in rehang rinsate was approximately 3.13 log10 CFU/ml. Natural contamination of Campylobacter spp. was detected in six out of six control (uninoculated) rinsates collected from rehang carcases with both the LAMP and the MLG 41A PCR methods. Campylobacter spp. were detected in all 24 inoculated samples analysed by both methods. All presumptive results for both methods were in agreement with the MLG 41.04 culture confirmation results from the same sample. The sensitivity (false negative) and specificity (false positive) for both methods were computed using the number of presumptive and culture-confirmed results (EquationEquations 1(1)
(1) and Equation2
(2)
(2) above; Eijkelkamp et al. Citation2009). The sensitivity and specificity for detection in BPW rehang rinsates was 100% for both methods. Results for detection by the LAMP assay from the unpaired BF-BEB enrichments of the MLG 41.04 reference method were identical to that of the MLG 41A PCR reference method.
nBPW rinsates
The average background aerobic flora in rehang rinsate was 3.22 log10 CFU/ml. Natural contamination of Campylobacter spp. was detected in six out of six control (uninoculated) rinsates collected from rehang carcases by both the LAMP and the MLG 41A PCR methods. Campylobacter spp. was detected in all 24 inoculated samples analysed by the LAMP method and in 22 inoculated samples with the MLG 41A PCR method ().
Table 3. Comparison between the LAMP method, MDA2CAM96 and the MLG 41A PCR reference method for the detection of Campylobacter spp. in rehang and post-chill carcase nBPW rinsates
The LAMP method had two false positives, while the PCR method had three false positives, as the samples had no typical growth on Campy Cefex agar. Results of detection by the LAMP assay from BF-BEB enrichments of the MLG 41.04 reference method were identical to that of the MLG 41A PCR reference method ().
Post-chill carcase rinsates
BPW rinsates
The average background aerobic flora in post-chill rinsate was 2.5 log10 CFU/ml. Natural contamination with Campylobacter spp. was detected in six out of six control (uninoculated) whole bird rinsates from post-chill carcases using both methods. For chicken parts rinsate, natural contamination was detected in four out of six control (uninoculated) samples with the LAMP method and six out of six control (uninoculated) samples with the MLG 41A PCR method. Campylobacter spp. were detected in all 18 inoculated samples (nine for whole bird and nine for parts rinsates) analysed by both the methods. All presumptive results for both methods were in agreement with the MLG 41.04 culture confirmation results. The sensitivity and specificity for Campylobacter spp. detection in BPW post-chill rinsates was 100% for both methods. Results for detection by the LAMP assay from the unpaired BF-BEB enrichments of the MLG 41.04 reference method were identical to those from the MLG 41A PCR reference method.
nBPW rinsates
The average background aerobic flora in post-chill rinsate was 2.05 log10 CFU/ml. Natural contamination of Campylobacter spp. was detected in four out of six control (uninoculated) whole bird rinsates using the LAMP method, and in one out of three control samples (uninoculated) with the MLG 41A PCR reference method. For the parts rinsate, natural contamination was detected in two out of six samples using the LAMP method and in none of the six samples using the MLG 41A PCR reference method. For the 18 inoculated rinsate samples (nine for whole bird and nine for the parts rinsate), Campylobacter spp. were detected in eight whole bird and seven parts rinsates analysed using the LAMP method (). For the 21 inoculated rinsate samples (12 for whole bird and nine for parts rinsates), Campylobacter was detected in four whole bird and three parts rinsates analysed with the MLG 41A PCR reference method (). The LAMP method had two false positives and two false negatives, while the PCR method had seven false negatives (). The BF-BEB enrichments from the MLG 41.04 reference method tested with the LAMP assay for Campylobacter spp. had more confirmed positives than the PCR assay (). No difference was observed in false positives and false negatives between the three different species of Campylobacter spp. used in this study.
Raw poultry products
The average background aerobic flora in various raw poultry product mixture ranged from 3 to 4 log10 CFU/ml. Natural contamination with Campylobacter spp. was detected in all the 10 MSC control (uninoculated) samples with both the LAMP and the MLG 41A PCR reference methods but in none of the ground turkey or marinated breast samples. For the 16 inoculated samples, Campylobacter spp. was detected in all the 10 MSC samples and in two out of three samples for each of the ground turkey and marinated breast samples by both the methods. All presumptive results for both methods were in complete agreement with the MLG 41.04 culture confirmation results. The sensitivity and specificity for the detection of Campylobacter spp. in raw poultry products was 100% with both methods. Results for detection by the LAMP assay from the BF-BEB enrichments of the MLG 41.04 reference method were identical to that of the MLG 41A PCR reference method.
The LAMP method (MDA2CAM96) uses an external amplification control, Matrix Control, to assess potential sample interference during the DNA isothermal amplification reaction. All matrices evaluated in this study for Campylobacter spp. detection resulted in a valid result with the Matrix Control, indicating that no inhibition of amplification reaction occurred with any of the matrices tested in this study. Similarly, all matrices gave valid internal amplification result with the MLG 41A PCR reference method (BAX® System Real-time PCR assay for Campylobacter spp.).
Data analysis
Analysis of dPOD for carcase rinses and raw poultry products showed that the detection of Campylobacter spp. with the alternative LAMP method (CEB enrichment) was not significantly different from the MLG 41A PCR reference method (BF-BEB enrichment), except for nBPW post-chill rinsates ().
Table 4. Probability of detection for unpaired comparison between the LAMP method and the MLG 41A PCR reference method for the detection of Campylobacter spp. in poultry matrices
For the nBPW post-chill rinsates, the LAMP method had more positives than the MLG 41A PCR reference method. For all samples with BF-BEB enrichments, there was no significant difference between the alternative LAMP and the MLG 41A PCR reference method.
Discussion
LAMP uses a unique DNA polymerase for continuous DNA amplification that is resistant to matrix interference and inhibitors (Notomi et al. Citation2000; Mori and Notomi Citation2009; Mori et al. Citation2013; Niessen et al. Citation2013; Notomi et al. Citation2015). LAMP assays have the same or higher sensitivity compared to PCR assays and traditional culture methods in detecting foodborne pathogens, such as Salmonella spp., Listeria spp., and Listeria monocytogenes, from various food matrices (Zhang et al. Citation2011; Niessen et al. Citation2013; Yang et al. Citation2014, Citation2016, Citation2018; Hu et al. Citation2017, Citation2018; Domesle et al. Citation2018). In recent years, a few studies on the use of LAMP for Campylobacter spp. detection have been published (Yamazaki et al. Citation2009; Romero et al. Citation2016; Romero and Cook Citation2018; Mason et al. Citation2019). Using LAMP assays, Mason et al. (Citation2019) detected 12 CFU/ml of C. coli and C. jejuni in chicken carcase samples without culturing and emphasised the importance of an easy-to-use LAMP method for real-time detection of Campylobacter spp. in poultry processing facilities.
In the current study, the LAMP method was used for detection of Campylobacter spp. in poultry matrices after enrichment under aerobic incubation in contrast to other studies using LAMP for direct detection without enrichment (Romero et al. Citation2016; Romero and Cook Citation2018; Mason et al. Citation2019). The LAMP method was coupled to the new RTU enrichment broth (AOAC PTM, Citation2018) that does not require microaerophilic incubation, to enrich Campylobacter spp. The RTU enrichment broth enabled shorter incubation for growth (22 to 28 h) of Campylobacter spp. than traditional media, BF-BEB (46 to 50 h).
For the boot swab samples, no reference method comparison was conducted as there was no published reference method for detection of Campylobacter spp. in primary production samples. Hence, the LAMP assay results were compared with the MLG 41.04 culture reference method results from the same samples.
Using CEB enrichment broth and the LAMP method provided more confirmed positive results of Campylobacter spp. in post-chill nBPW enrichments than using BF-BEB enrichment. In addition to the BPW base, nBPW contains lecithin, sodium thiosulphate, and sodium bicarbonate (USDA FSIS Citation2016). There are limited studies on the effect of nBPW on the growth of Salmonella and Campylobacter spp. In a recent study, Bourassa et al. (Citation2019) used BF-BEB enrichment and traditional plating, and detected significantly fewer Campylobacter spp. positive carcases in nBPW rinsates than in BPW rinsates. This indicated that nBPW may lessen the ability to detect Campylobacter spp. in poultry rinsates.
In the USA, post-chill rinsates are collected after antimicrobial interventions, such as peroxyacetic acid (2000 ppm), acidified sodium chlorite (500 to 1200 ppm) or cetylpyridinium chloride (0.8%). The organisms will be more stressed due to this treatment, and may not recover well with nBPW-BF-BEB enrichment due to prolonged incubation and possible effect of neutralisers in nBPW. With CEB enrichment, there was greater than 80% recovery from inoculated post-chill nBPW rinsates as opposed to 33% with BF-BEB enrichments, as detected by molecular methods.
The LAMP and PCR method showed false negatives with post-chill nBPW rinsates and the numbers were much higher when using the PCR method (seven false negatives) compared to the LAMP method (two false negatives). Molecular methods typically have a limit of detection (LOD) of 104 CFU/ml (Mafu et al. Citation2009; Law et al. Citation2015; Liu et al. Citation2019) and it is possible that the growth levels in the enriched samples did not reach the minimum LOD needed for detection, leading to false negatives. Campylobacter spp. growth in carcase rinsates may be affected by one or a combination of the neutralisers present in the nBPW. False positives were observed only with nBPW rinsates, and carryover neutralisers from nBPW may affect growth of Campylobacter spp. on Campy Cefex agar. In addition, the cells may be more stressed in nBPW media leading to poor recovery and death of cells. False positives may have occurred due to death of cells leading to detection of free DNA by molecular methods.
Rehang carcase rinsates usually have a higher organic load than the post-chill rinsates, which may reduce the impact of nBPW on the growth of Campylobacter spp. in enriched circumstances, as the added neutralisers in nBPW may be bound to the organic material. Hence, no significant difference was observed with nBPW rehang carcase enriched samples for both methods.
Campylobacter spp. are fastidious organisms requiring complex media and microaerophilic conditions for optimal growth (Davis and DiRita Citation2008). Several media have been developed that contain ingredients such as blood, haemin, charcoal, ferrous sulphate, sodium pyruvate, and sodium metabisulfite, to protect the bacteria from the toxic effects of substances that form in the presence of oxygen and light (Bolton et al. Citation1984; Corry and Attabay Citation2001; Park Citation2005). In addition, an antibiotic supplement containing cefoperazone, vancomycin and trimethoprim is added to the medium to inhibit the growth of other background microflora. Firstly, the base medium broth is hydrated, autoclaved and cooled. Then, an antibiotic supplement (prepared in an organic solvent, such as alcohol) and other supplements, such as blood, are added to the sterile, cooled medium. The preparation of such culture media is tedious, time-consuming and can be expensive.
Bolton’s enrichment broth (BEB) containing lysed horse blood and antibiotics, or BF-BEB with antibiotics (MLG 41.04 Citation2016) or Preston broth (Anonymous Citation2017) with blood and antibiotics are typically used for enrichment. Several researchers have tried adding various supplements to these media to support growth under aerobic incubation conditions, and modified media have shown promise with pure cultures (Hinton Citation2012, Citation2013, Citation2014; Hill et al. Citation2017). However, except for BF-BEB and Preston broth, there are limited studies on the utility of other media types for poultry samples. Enrichment media that create low oxygen environments by use of scavengers allow for immediate equilibration of samples, unlike traditional media, which need diffusion of the gasses from the sachet or microaerophilic incubator. With less exposure to oxygen, these media can enhance the recovery of Campylobacter spp. and accelerate its growth.
A major advantage of the LAMP method includes next-day results and ease-of-use compared to current methods using traditional culture medium (BF-BEB or Preston broth), that require multiple preparation steps and microaerophilic incubation (MLG 41.04 Citation2016; Anonymous Citation2017). This and the AOAC performance tested method study (AOAC PTM, Citation2018) using LAMP provided better detection than the MLG 41.04 culture reference method. The LAMP method used in this study offered an easy-to-use analytical tool to assess the prevalence and detection of Campylobacter spp. in poultry products.
Acknowledgments
All the work was conducted at WBA Analytical Laboratories, Inc. an ISO 17025 accredited laboratory, under the direction of authors from 3M Food Safety. The authors gratefully acknowledge WBA management for support and use of facilities to conduct the study.
Disclosure statement
The authors, R. Rajagopal, C. A. Barnes and J. M. David are employees of 3M Food Safety which offers multiple commercial solutions, including 3M™ Molecular Detection system and 3M™ Molecular Detection Assays to the food industry. Jamie and Jessie Goseland are employees of WBA Analytical Laboratories. WBA Analytical Laboratories provides commercial microbiological and chemical analysis, product research and development and technical services.
References
- Anonymous. 2017. EN ISO 10272–1:2017. “Microbiology of the Food Chain – Horizontal Method for Detection and Enumeration of Campylobacter spp. - Part 1: Detection Method” International Organization for Standardization. Geneva, Switzerland.
- AOAC PTM. 2018. “AOAC Performance Tested Method Certificate No. 111803. 3M™ Molecular Detection Assay 2 – Campylobacter.” Accessed June 1 2020. http://members.aoac.org/aoac_prod_imis/AOAC_Docs/RI/19PTM/19C_111803_2MMDA2Camp.pdf.
- Batz, M., S. Hoffmann, and J. G. Morris Jr. 2012. “Ranking the Disease Burden of 14 Pathogens in Food Sources in the United States Using Attribution Data from Outbreak Investigations and Expert Elicitation.” Journal of Food Protection 75: 1278–1291. doi:10.4315/0362-028X.JFP-11-418.
- Berrang, M. E., R. J. Buhr, and J. A. Cason. 2000. “Campylobacter Recovery from External and Internal Organs of Commercial Broiler Carcass Prior to Scalding.” Poultry Science 79: 286-290. doi:10.1093/ps/79.2.286.
- Bolton, D. J. 2015. “Campylobacter Virulence and Survival Factors.” Food Microbiology 28: 99–108. doi:10.1016/j.fm.2014.11.017.
- Bolton, F. J., D. Coates, and D. N. Hutchinson. 1984. “The Ability of Campylobacter Supplements to Neutralize Photochemically Induced Toxicity and Hydrogen Peroxide.” Journal of Applied Bacteriology 56: 151–157. doi:10.1111/j.1365-2672.1984.tb04707.x.
- Bourassa, D. V., J. L. Lapidus, A. E. Kennedy-Smith, and A. Morey. 2019. “Efficacy of Neutralizing Buffered Peptone Water for Recovery of Salmonella, Campylobacter, and Enterobacteriaceae from Broiler Carcasses at Various Points along a Commercial Immersion Chilling Process with Peroxyacetic Acid.” Poultry Science 98: 393–397. doi:10.3382/ps/pey361.
- CDC 2017. “Campylobacter (Campylobacteriosis).” Accessed 1 June 2020. https://www.cdc.gov/campylobacter/.
- Corry, J. E., and H. L. Attabay. 2001. “Poultry as a Source of Campylobacter and Related Organisms.” Journal of Applied Microbiology 90: 96S–114S. doi:10.1046/j.1365-2672.2001.01358.x.
- Davis, L., and V. DiRita. 2008. “Growth and Laboratory Maintenance of Campylobacter jejuni.” Current Protocols in Microbiology 10: 8A.1.1‐8A.1.7. doi:10.1002/9780471729259.mc08a01s10.
- Domesle, K. J., Q. Yang, T. S. Hammack, and B. Ge. 2018. “Validation of a Salmonella Loop-mediated Isothermal Amplification Assay in Animal Food.” International Journal of Food Microbiology 264: 63–76. doi:10.1016/j.ijfoodmicro.2017.10.020.
- EFSA and ECDC. 2018. “The European Union Summary Report on Trends and Sources of Zoonoses, Zoonotic Agents and Food-borne Outbreaks in 2017.” EFSA Journal 16: 5500. doi:10.2903/j.efsa.2018.5500.
- Eijkelkamp, J. M., H. J. M. Aarts, and H. J. van der Fels-klerx. 2009. “Suitability of Rapid Detection Methods for Salmonella in Poultry Slaughterhouses.” Food Analytical Methods 2: 1–13. doi:10.1007/s12161-008-9040-5.
- Gandelman, O. A., V. L. Church, C. A. Moore, G. Kiddle, C. A. Carne, S. Parmar, H. Jalal, L. C. Tisi, and J. A. Murray. 2010. “Novel Bioluminescent Quantitative Detection of Nucleic Acid Amplification in Real-time.” Plos One 5, e14155. doi:10.1371/journal.pone.0014155.
- Hill, G. N., C. L. Bratcher, L. Wang, M. Singh, W. N. Tapp, and S. R. McKee. 2017. “Optimizing Enrichment of Campylobacter on Poultry.” Journal of Applied Poultry Research 26: 307–315. doi:10.3382/japr/pfw075.
- Hinton, A., Jr. 2012. “Aerobic Growth of Campylobacter in Media Supplemented with α-ketoglutaric, Lactic, and/or Fumaric Acids.” World’s Poultry Science Journal 68 (Suppl 1): 256–258.
- Hinton, A., Jr. 2013. “Aerobic Growth of Campylobacter in Media Supplemented with C3 Monocarboxylates and C4-dicarboxylates.” Journal of Food Protection 76: 685–690. doi:10.4315/0362-028X.JFP-12-430.
- Hinton, A., Jr. 2014. “Effect of Beef Extract Concentration on Growth of Campylobacter in Media Incubated Aerobically.” Journal of Food Protection 77 (Supplement A): 39.
- Hoffmann, S., B. Maculloch, and M. Batz. 2015. Economic Burden of Major Foodborne Illnesses Acquired in the United States, EIB-140. Washington, DC: United States Department of Agriculture. https://www.ers.usda.gov/webdocs/publications/43984/52807_eib140.pdf.
- Hu, L., L. M. Ma, S. Zheng, X. He, H. Wang, E. W. Brown, T. S. Hammack, and G. Zhang. 2017. “Evaluation of 3M Molecular Detection System and ANSR Pathogen Detection System for Rapid Detection of Salmonella from Egg Products.” Poultry Science 96: 1410–1418. doi:10.3382/ps/pew399.
- Hu, L., X. Deng, E. W. Brown, T. S. Hammack, L. M. Ma, and G. Zhang. 2018. “Evaluation of Roka Atlas Salmonella Method for the Detection of Salmonella in Egg Products in Comparison with Culture Method, Real-time PCR and Isothermal Amplification Assays.” Food Control 94: 123–131. doi:10.1016/j.foodcont.2018.06.039.
- Jasson, V., L. Jacxsens, P. Luning, A. Rajkovic, and M. Uyttendaele. 2010. “Alternative Microbial Methods: An Overview and Selection Criteria.” Food Microbiology 27: 710–730. doi:10.1016/j.fm.2010.04.008.
- Kaneko, H., T. Kawana, E. Fukushima, and T. Suzutani. 2007. “Tolerance of Loop-mediated Isothermal Amplification to a Culture Medium and Biological Substances.” Journal of Biochemical Biophysical Methods 70: 499–501. doi:10.1016/j.jbbm.2006.08.008.
- Law, J. W., N. S. Ab Mutalib, K. G. Chan, and L. H. Lee. 2015. “Rapid Methods for the Detection of Foodborne Bacterial Pathogens: Principles, Applications, Advantages and Limitations.” Frontiers in Microbiology 5: 770. doi:10.3389/fmicb.2014.00770.
- Lim, H. S., Q. Zheng, M. Miks-Krajnik, M. Turner, and H. G. Yuk. 2015. “Evaluation of Commercial Kit Based on Loop-mediated Isothermal Amplification for Rapid Detection of Low Levels of Uninjured and Injured Salmonella on Duck Meat, Bean Sprouts, and Fishballs in Singapore.” Journal of Food Protection 78: 1203–1207. doi:10.4315/0362-028X.JFP-14-535.
- Liu, Y., Y. Cao, T. Wang, Q. Dong, J. Li, and C. Niu. 2019. “Detection of 12 Common Food-borne Bacterial Pathogens by Taqman Real-time PCR Using a Single Set of Reaction Conditions.” Frontiers in Microbiology 10: 222. doi:10.3389/fmicb.2019.00222.
- Mafu, A. A., M. Pitre, and S. Sirois. 2009. “Real-time PCR as a Tool for Detection of Pathogenic Bacteria on Contaminated Food Contact Surfaces by Using a Single Enrichment Medium.” Journal of Food Protection 72: 1310–1314. doi:10.4315/0362-028X-72.6.1310.
- Mangal, M., S. Bansal, S. K. Sharma, and R. K. Gupta. 2016. “Molecular Detection of Foodborne Pathogens: A Rapid and Accurate Answer to Food Safety.” Critical Reviews in Food Science and Nutrition 56: 1568–1584. doi:10.1080/10408398.2013.782483.
- Mason, M. G., P. J. Blackall, J. R. Botella, and J. M. Templeton. 2019. “An Easy-to-perform, Culture-free Campylobacter Point-of-management Assay for Processing Plant Applications.” Journal of Applied Microbiology 128: 620–629. doi:10.1111/jam.14509.
- MLG 41.04. 2016. “Isolation and Identification of Campylobacter jejuni/coli/lari from Poultry Rinse, Sponge and Raw Product Samples.” Accessed 1 June 2020. https://www.fsis.usda.gov/wps/wcm/connect/0273bc3d-2363-45b3-befb-1190c25f3c8b/MLG-41.pdf?MOD=AJPERES.
- MLG 41A.00. 2016. “FSIS Procedure for the Use of a Polymerase Chain Reaction (PCR) Assay for Screening Campylobacter jejuni/coli/lari in Poultry Rinse, Sponge and Raw Product Samples.” Accessed 1 June 2020. https://www.fsis.usda.gov/wps/wcm/connect/ef62eaa8-4d65-4df9-a983-3908fc342b12/MLG-41A.pdf?MOD=AJPERES.
- Mori, Y., H. Kanda, and T. Notomi. 2013. “Loop-mediated Isothermal Amplification (LAMP): Recent Progress in Research and Development.” Journal of Infection and Chemotherapy 19: 404–411. doi:10.1007/s10156-013-0590-0.
- Mori, Y., and T. Notomi. 2009. “Loop-mediated Isothermal Amplification (LAMP): A Rapid, Accurate, and Cost-effective Diagnostic Method for Infectious Diseases.” Journal of Infection and Chemotherapy 15: 62–69. doi:10.1007/s10156-009-0669-9.
- Niessen, L., J. Luo, C. Denschlag, and R. F. Vogel. 2013. “The Application of Loop-mediated Isothermal Amplification (LAMP) in Food Testing for Bacterial Pathogens and Fungal Contaminants.” Food Microbiology 36: 191–206. doi:10.1016/j.fm.2013.04.017.
- Notomi, T., H. Okayama, H. Masubuchi, T. Yonekawa, K. Watanabe, N. Amino, and T. Hase. 2000. “Loop-mediated Isothermal Amplification of DNA.” Nucleic Acids Research 28: E63. doi:10.1093/nar/28.12.e63.
- Notomi, T., Y. Mori, N. Tomita, and H. Kanda. 2015. “Loop-mediated Isothermal Amplification (LAMP): Principle, Features, and Future Prospects.” Journal of Microbiology 53: 1–5. doi:10.1007/s12275-015-4656-9.
- Park, S. F. 2005. “Campylobacter jejuni Stress Responses during Survival in the Food Chain and Colonization.” In Campylobacter: Molecular and Cellular Biology, edited by J. M. Ketley and M. E. Konkel, 311–330. Norfolk, United Kingdom: Horizon Bioscience.
- Perez-Arnedo, I., and E. Gonzalez-Fandos. 2019. “Prevalence of Campylobacter spp. in Poultry in Three Spanish Farms, a Slaughterhouse and a Further Processing Plant.” Foods 8: 111. doi:10.3390/foods8030111.
- Plutzer, J., and P. Karanis. 2009. “Rapid Identification of Giardia Duodenalis by Loop-mediated Isothermal Amplification (LAMP) from Faecal and Environmental Samples and Comparative Findings by PCR and Real-time PCR Methods.” Parasitology Research 104: 1527–1533. doi:10.1007/s00436-009-1391-3.
- Romero, M. R., M. D’Agostino, A. P. Arias, S. Robles, C. F. Casado, L. O. Iturbe, O. G. Lerma, M. Andreou, and N. Cook. 2016. “An Immunomagnetic Separation/loop‐mediated Isothermal Amplification Method for Rapid Direct Detection of Thermotolerant Campylobacter spp. during Poultry Production.” Journal of Applied Microbiology 120: 469–477. doi:10.1111/jam.13008.
- Romero, M. R., and N. Cook. 2018. “A Rapid LAMP-based Method for Screening Poultry Samples for Campylobacter without Enrichment.” Frontiers in Microbiology 9: 2401. doi:10.3389/fmicb.2018.02401.
- Rouger, A., O. Tresse, and M. Zagorec. 2017. “Bacterial Contaminants of Poultry Meat: Sources, Species, and Dynamics.” Microorganisms 5: 50. doi:10.3390/microorganisms5030050.
- Sarowska, J., M. Frej-Mądrzak, A. Jama-Kmiecik, A. Kilian, D. Teryks-Wołyniec, and I. Choroszy-Król. 2016. “Detection of Salmonella in Foods Using a Reference PN-ISO Method and an Alternative Method Based on Loop-mediated Isothermal Amplification Coupled with Bioluminescence.” Advances in Clinical and Experimental Medicine 25: 945–950. doi:10.17219/acem/63000.
- Skarp, C. P. A., M. L. Hänninen, and H. I. K. Rautelin. 2016. “Campylobacteriosis: The Role of Poultry Meat.” Clinical Microbiology and Infection 22: 103–109. doi:10.1016/j.cmi.2015.11.019.
- Souii, A., M. B. M´hadheb-Gharbi, and J. Gharbi. 2016. “Nucleic Acid-based Biotechnologies for Food-borne Pathogen Detection Using Routine Time-intensive Culture-based Methods and Fast Molecular Diagnostics.” Food Science and Biotechnology 25: 11–20. doi:10.1007/s10068-016-0002-1.
- USDA FSIS. 2015. “Changes to the Salmonella and Campylobacter Verification Testing Program: Proposed Performance Standards for Salmonella and Campylobacter in Not-Ready-to-Eat Comminuted Chicken and Turkey Products and Raw Chicken Parts and Related Agency Verification Procedures and Other Changes to Agency Sampling. FSIS-2014-0023.” Federal Register 80: 3940–3950.
- USDA FSIS. 2016. “New Neutralizing Buffered Peptone Water to Replace Current Buffered Peptone Water for Poultry Verification Sampling.” Food Safety and Inspection Service Notice 41-16. Accessed 1 June 2020. https://www.fsis.usda.gov/wps/wcm/connect/2cb982e0-625c-483f-9f50-6f24bc660f33/41-16.pdf?MOD=AJPERES.
- USDA FSIS. 2019. “Changes to the Campylobacter Verification Testing Program: Revised Performance Standards for Campylobacter in Not-Ready-to-Eat Comminuted Chicken and Turkey and Related Agency Procedures.” FSIS-2018-0044. Federal Register 84: 38203–38210.
- Wehling, P., R. A. LaBudde, and M. T. Nelson. 2011. “Probability of Detection (POD) as a Statistical Model for the Validation of Qualitative Methods.” Journal of AOAC International 94: 335–347. doi:10.1093/jaoac/94.1.335.
- Wiedmann, M., S. Wang, L. Post, and K. Nightingale. 2014. “Assessment Criteria and Approaches for Rapid Detection Methods to be Used in the Food Industry.” Journal of Food Protection 77: 670–690. doi:10.4315/0362-028X.JFP-13-138.
- Yamazaki, W., M. Taguchi, T. Kawai, K. Kawatsu, J. Sakata, K. Inoue, and N. Misawa. 2009. “Comparison of Loop-mediated Isothermal Amplification Assay and Conventional Culture Methods for Detection of Campylobacter jejuni and Campylobacter coli in Naturally Contaminated Chicken Meat Samples.” Applied Environmental Microbiology 75: 1597–1603. doi:10.1128/AEM.02004-08.
- Yang, Q., F. Wang, W. Prinyawiwatkul, and B. Ge. 2014. “Robustness of Salmonella Loop-mediated Isothermal Amplification Assays for Food Applications.” Journal of Applied Microbiology 116: 81–88. doi:10.1111/jam.12340.
- Yang, Q., K. J. Domesle, and B. Ge. 2018. “Loop-mediated Isothermal Amplification for Salmonella Detection in Food and Feed: Current Applications and Future Directions.” Foodborne Pathogens and Disease 15: 309–331. doi:10.1089/fpd.2018.2445.
- Yang, Q., K. J. Domesle, F. Wang, and B. Ge. 2016. “Rapid Detection of Salmonella in Food and Feed by Coupling Loop-mediated Isothermal Amplification with Bioluminescent Assay in Real-time.” BMC Microbiology 16: 112. doi:10.1186/s12866-016-0730-7.
- Zhang, G., E. W. Brown, and N. González-Escalona. 2011. “Comparison of Real-time PCR, Reverse Transcriptase Real-time PCR, Loop-mediated Isothermal Amplification, and the FDA Conventional Microbiological Method for the Detection of Salmonella spp. in Produce.” Applied Environmental Microbiology 77: 6495–6501. doi:10.1128/AEM.00520-11.