Abstract
The flesh-flies of the genus Sarcophaga (Diptera: Sarcophagidae) are prevalent world-wide, have great medical and veterinary importance forming an important group of myiasis producers. The diploid chromosome complements of the genus Sarcophaga comprise of five homologous pairs of autosomes and a pair of sex chromosomes (XX in females and XY in males). Heterochromatin of Sarcophaga species has been studied and characterized. The modification of permanently condensed patterns of chromosomes of the two Sarcophaga species, Sarcophaga argyrostoma and Sarcophaga ruficornis, by means of a GC specific DNA ligand Mithramycin (0.05μg/ml) proved to be useful for the analysis of heterochromatic regions of the chromosomes after DAPI staining. The pericentric regions of all the autosomes revealed fluorescent bands in both the species while both the X- and Y- chromosomes revealed differential staining due to differences in base sequence organization in these areas. Therefore, the present investigation was carried out in a view to characterize the heterochromatic components of the two species in order to unravel the base specificity of the chromosomes that will facilitate the study of heterochromatin in the evolution of the genus Sarcophaga.
Introduction
The sarcophagid flies, commonly known as the flesh-flies, belong to the family Sarcophagidae of Calyptrate Diptera. These flies are of medical and veterinary importance and form an important group of myiasis producers (Cornaby Citation1974) second only to the related family Calliphoridae (Boyes & van Brink Citation1965). The cytogenetic findings in the family Sarcophagidae have revealed that all the 67 species investigated so far have the same basic diploid chromosome component of 2n=12 with only few exceptions. These flies show a remarkable uniformity of autosomes that are mostly euchromatic and a pair of sex chromosomes varying in size from dot like structures to very large chromosomes, which are almost heterochromatic (Kaul et al. Citation1978). Distribution and fine characterization of Sarcophaga heterochromatin by several banding techniques reveal considerable heterogeneity in the heterochromatic components (Kaul et al. Citation1989).
The total genetic endowment of organisms, the genome, is contained in the chromosomes. These chromosomes take part in genetic differentiation between species and are apparent as chromosomal rearrangements of gene sequences (Griffiths et al. Citation1999). The chromosomes of most organisms are characterized by the presence of heterochromatin, which is an interesting, complex and an enigmatic component of chromosomes. The variations in heterochromatic components of the genome play a vital role in species differentiation (Baimai Citation1998).
The initiation of C-banding technique by Arrighi and Hsu (Citation1971) generated tremendous interest among cytogeneticists for the study of heterochromatic components of different organisms. This technique offers a useful tool to localize constitutive heterochromatin on the metaphase chromosomes and another measure for unraveling differences between the mitotic chromosomes of the species. Subsequent studies establish that these constitutive heterochromatic areas are rich in repetitive DNA sequences, show late DNA replication and are devoid of structural genes. At about the same time, Caspersson et al. (Citation1968) showed differential fluorescence pattern with quinacrine mustard on mitotic chromosomes and since then, a plethora of fluorescence banding methods have been developed. These fluorescent banding techniques are in vogue to characterize the base specificity of the heterochromatin components of the chromosomes (Schweizer Citation1976a; Schweizer Citation1976b; Kaul et al. Citation1989; Souza et al. Citation1998; De Souza et al. Citation2003; Warchałowska-S′liwa et al. Citation2007).
Heterochromatin of Sarcophaga species has been extensively studied and characterized at the cytological and molecular level (Kaul et al. Citation1978, 1989; Samols & Swift Citation1979a; Schweizer Citation1976b; Gaur et al. Citation1985; Rai et al. Citation1985; Bultmann & Mezzanotte Citation1987; Tewari et al. Citation1988; Agrawal & Kaul Citation1990; Hershfield & Swift Citation1990; Maltempi & Avancini Citation2000; Rai Citation2001; Srivastava et al. Citation2013). Several base specific fluorochromes characterize the heterochromatic bands by the analysis of their GC or AT content (Schweizer Citation1976a; Schweizer Citation1976b; Schweizer Citation1980). In agreement with its base pair specificity, the DNA-ligand fluorochromes can be classified in two groups. One group corresponds to the AT -specific fluorochromes, such as 4’-6 diamidino-2-phenylindole (DAPI), Hoechst 33258 and Quinacrine, reveal banding patterns similar to G-banding (Latt & Wohleb Citation1975; Lee & Collins Citation1977; Lin et al. Citation1977). In contrast, another group is the GC-specific fluorochromes, such as chromomycin A3 (CMA3), mithramycin and olivomycin, show the complementary reverse banding (R-banding) along the chromosomes (Schweizer Citation1980). These base-specific fluorochromes have also been used to differentiate heterochromatic segments that were apparently homogeneous by standard Giemsa C-banding (Schweizer Citation1980; Galleni et al. Citation1992; Bella et al. Citation1995; Santos & De Souza Citation1998).
The modification of permanently condensed patterns of chromosomes by means of a DNA ligand Mithramycin proved to be useful for the analysis of some features of this segment of the genome (Fox & Howarth Citation1985; Schmid et al. Citation1986; Cons & Fox Citation1990; Santos & De Souza Citation1998). In the present investigation our main aim was to identify heterochromatic regions affected by Mithramycin in two Sarcophaga, Meigen 1826 (Parasarcophaga, Johnston & Tiegs 1921) species, Sarcophaga (Parasarcophaga) argyrostoma and Sarcophaga (Parasarcophaga) ruficornis, and to analyze the further possibilities for resolving heterochromatin into different constituents and its role in the evolution of the genus Sarcophaga.
Materials and methods
Fly rearing
In the present work, two species of the flesh-flies belonging to the genus Sarcophaga i.e. S. ruficornis (Fabricius 1794) and S. argyrostoma (Robineau-Desvoidy 1830), were used. Both the species were collected from various localities of Allahabad and laboratory colonies of the two species were started from the progenies of wild inseminated females in insect rearing cages maintained at 27 ± 2°C. Sugar cubes and water were kept in the cages for feeding the adult flies. Fresh goat kidney pieces were kept in the cages for larviposition and the larva were reared in an uncrowded condition, fed with fresh kidney pieces for about six days. The mature third instar larvae stop feeding and start wandering in search of dry places. These were then transferred to clean glass jars with saw dust for pupation. The pupae were kept in petri-dish in the insect rearing cages for emergence. The adult flies emerged after about eleven days of pupation.
Chromosome preparation
Metaphase chromosome preparations were made from the neural ganglia of the third instar larvae. Neural ganglia were dissected out in insect saline, incubated in sodium citrate (2%) containing colchicine solution (0.05%) for 15 min in a cavity block. The ganglia were fixed in freshly prepared Carnoy’s fixative and kept on a clean slide in a drop of 60% acetic acid and macerated with the help of needles, the cell suspension was spread and air-dried.
DNA ligand Mithramycin pre-treatment
For further characterization of heterochromatin, the neural ganglia from the third instar larvae of both the species were dissected out in insect saline (0.9% NaCl) supplemented with 0.05 μg/ml mithramycin for 20 min. The mithramycin pretreated neural ganglia were processed for chromosome preparations and consequently stained with DAPI as described below.
Fluoroscence staining
The technique of Schmid et al. (Citation1983) was adopted for staining with DAPI. The air-dried preparations were stained with 0.2 μg/ml DAPI in McIlvaine’s buffer (pH 7.0) for 10 min, mounted in 1:1 solution of McIlvaine’s buffer and glycerol. The chromosome preparations were observed under fluorescence microscope with the filter combination of N UV-2A.
Results
S. ruficornis presented a model diploid chromosome complement of 12 chromosomes with five homologous pairs of bi-armed autosomes showing somatic pairing and a pair of telocentric sex chromosome, XX in the females and XY in the males. The Y chromosome is smaller than the X chromosome. In addition, the pericentromeric regions of autosomal pairs II and V reveal fluorescent bands with DAPI. The X chromosome shows bright fluorescence throughout its length. Except the distal tip, the entire Y chromosome reveals a bright fluorescence in males (Figure ). DNA ligand mithramycin pretreated neural ganglia reveal distinct fluorescence in the pericentric regions of all the autosomal pairs with DAPI at prometaphase stage in females (Figure ). The X chromosomes of females reveal a bright fluorescence, except for two very thin regions which show slightly dull fluorescence (Figure ). The main dissimilarity of mithramycin treated DAPI stained chromosomes from the normal chromosomes is that, at condensed metaphase, the fluorescent pericentric regions of the autosomes are not clearly demarcated from the rest of the chromosome and the X chromosome shows uniform bright fluorescence, while the entire Y chromosome shows fluorescence except the distal tip in males (Figure ).
Figure 1. (a) Photomicrograph of metaphase chromosomes of male Sarcophaga ruficornis with DAPI staining (Bar on all the figures represents 10μ). (b) DNA ligand mithramycin pretreated female S. ruficornis followed by DAPI staining revealing bright fluorescence in the pericentric regions of all the autosomal pairs. (c) The X chromosome revealing a bright fluorescence, except for two very thin regions which show slightly dull fluorescence in female. (d) DNA ligand mithramycin pretreated male S. ruficornis followed by DAPI staining showing the indistinguishable pericentric regions of autosomes and bright fluorescence of the X chromosome, the entire Y chromosome shows fluorescence except the distal tip.
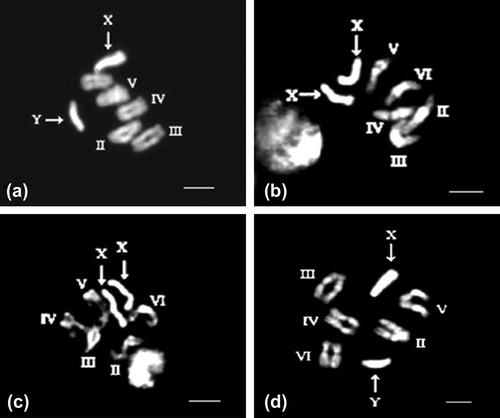
S. argyrostoma chromosome complement also comprises 12 chromosomes, with three pairs of metacentric (pairs II, V and VI), two pairs of submetacentric autosomes (III and IV), a pair of telocentric sex chromosomes, XX in females and XY in males. Y chromosome is the smallest chromosome in the complement. The pericentric regions of autosomal pairs II, V and VI reveal bright fluorescent bands with DAPI while the rest of the autosomes show moderate fluorescent bands. The telocentric X chromosome shows bright fluorescence throughout its length except the distal tip while the Y chromosome is uniformly bright (Figure a). The DNA ligand mithramycin pretreated neural ganglia in this species reveal brightly fluorescent bands in the pericentric area of all the five autosomal pairs and the entire X chromosome (Figure b) exhibiting a major difference from normal DAPI stained chromosomes. However, in the less condensed prometaphases, the X chromosome shows differential staining, such that the distal tip shows a dull fluorescence (Figure c). The entire Y chromosome shows a uniform bright fluorescence (Figure d).
Figure 2. (a) Photomicrograph of DAPI stained metaphase chromosomes of male Sarcophaga argyrostoma. (b) DNA ligand mithramycin pre-treatment followed by DAPI staining showing brightly fluorescent bands in the pericentric area of all the five autosomal pairs and the entire X chromosome in female. (c) The decondensed prometaphase chromosomes obtained after mithramycin treatment of neural ganglia of female S. argyrostoma followed by DAPI staining reveals differential staining in the X chromosome, such that the distal tip shows a dull fluorescence. (d) The entire Y chromosome shows a uniform bright staining in male.
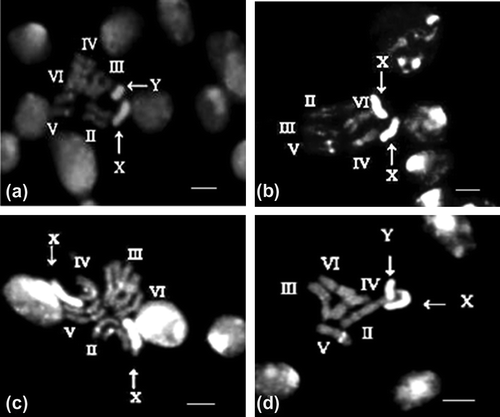
The distribution pattern of heterochromatin in chromosomal complements of both the species is represented in Table .
Table 1. Heterochromatin distribution pattern induced by Mithramycin in mitotic chromosome complement of the two Sarcophaga species.
Discussion
The comparative morphometric analysis of the karyological data in the family Sarcophagidae has revealed that the gross morphology of autosomes is more or less similar in the family while the heterochromosomes, i.e. X and Y chromosomes, are distinct and reveal great variations in morphology from species to species, which prompted Boyes and van Brink (Citation1965) to emphasize the role of heterochromatin in chromosomal evolution of sarcophagids. It is well known that heterochromatin has played an important role in the evolution of sex chromosomes, as the addition of most heterochromatin might be the precursor for the specialization of sex chromosomes (John Citation1988; Jablonka & Lamb Citation1990). Several differential staining techniques were discovered to characterize the constitutive heterochromatin in the chromosome complement of various organisms. However, it was only in the late seventies that the chromosome complements of the sacorphagids were analyzed by C-banding techniques (Kaul et al. Citation1978). A comparative analysis of the localization of constitutive heterochromatin on the mitotic chromosomes with orcein staining and C-banding demonstrated the presence of pericentric constitutive heterochromatin on the autosomes and the sex chromosomes (Kaul et al. Citation1978).
The darkly staining constitutive heterochromatin with the C-banding technique is known to be enriched with highly repetitive DNA (Hennig & Walker Citation1970; Bianchi & Ayres Citation1971; Arrighi et al. Citation1974; Cordeiro et al. Citation1975; Brutlag et al. Citation1977; Lee & Collins Citation1977; Duhamel-Maestracci et al. Citation1979). The application of C-banding technique has shown that the centromeric regions of autosomes and the sex chromosomes show darkly stained areas representing constitutive heterochromatin. In some cases, the X- as well as Y-chromosome shows differential staining which prompts one to speculate that this differential staining may be due to some kind of difference in the base sequence organization in these areas (Hsu Citation1973). However, exception persists where either the constitutive heterochromatin may not reveal dark staining with C-band techniques (Ennis Citation1974) or the C-banded regions are devoid of highly repetitive DNA (Gall et al. Citation1971; Arrighi et al. Citation1974; Comings et al. Citation1977).
Further, characterization of the constitutive heterochromatin in the mitotic complements of sarcophagids with several fluorochromes such as Quinacrine, Hoechst 33258, DAPI revealed that the pericentric areas of some autosomes and large areas of the sex chromosomes, i.e., X and Y are characterized by bright fluorescence (Gaur et al. Citation1985; Rai et al. Citation1985; Tewari et al. Citation1988; Kaul et al. Citation1989; Agrawal & Kaul Citation1990; Srivastava Citation2009; Srivastava et al. Citation2013). The bright fluorescence has been attributed to highly AT- rich DNA sequences (Caspersson et al. Citation1968; Ellison & Barr Citation1972; Hilwig & Gropp Citation1972; Weisblum & de Haseth Citation1972; Weisblum & Haenssler Citation1974; Holmquist Citation1975; Latt & Wohlleb Citation1975; Gatti et al. Citation1976). In order to enhance the resolution of fluorescent bands, non-fluorescent GC-specific ligand Actinomycin D has been used in different organisms (Muller & Crothers Citation1968; Schweizer Citation1976a, Citation1976b; Lakhotia & Mishra Citation1980; Rai et al. Citation1985). The application of this technique in sarcophagids revealed that the same differential staining pattern observed with the Q-, H- and DAPI has been retained confirming the fact that the brightly fluorescent regions are indeed rich in AT-sequences (Tewari et al. Citation1988; Kaul et al. Citation1989; Agrawal & Kaul Citation1990; Rai Citation2001).
Mithramycin is a DNA ligand which binds to guanine residues of the helical DNA with strong specificity and the binding sites of the ligand increase with the GC content of DNA (Behr et al. Citation1969). The present work illustrates that the chromosomal preparations obtained from the neural ganglia pretreated with mithramycin reveal bright fluorescence with DAPI in the heterochromatic regions of all the five autosomes and the sex chromosomes in both the species. The staining property of DAPI (4’-6-diamidino 2-phenyl indole) is also attributed to AT specific binding. However, its fluorescence is not sensitive to variations in the base composition like that of quinacrine. It produces an intense and distinct pattern with negligible fading and its brightness is a general indicator of AT rich sequences (Lin et al. Citation1977; Schnedl et al. Citation1977).
Cytological preparations of S. ruficornis and S. argyrostoma treated with mithramycin and stained with DAPI reveal the longitudinal differentiation of chromosomes which is correlated to previous cytological data found in this species and interpreted as a possible consequence of the presence of satellite DNA in specific chromosome areas. It is interesting, however, to note that autosomal pairs III, IV and VI in the complement of S. ruficornis and III and IV in the complements of S. argyrostoma do not show bright fluorescence when stained only with DAPI. This may perhaps be due to the fact that the AT and GC rich sequences are intermingled in such a way that AT specific dyes are unable to bind to these areas. However, when the GC rich areas are suppressed by the DNA-ligand mithramycin, only then are the AT rich sequences able to bind to DAPI and therefore, fluoresce. Thus, once again it can be surmised that the constitutive heterochromatin in both the species is rich in AT sequences. The similar effect of DNA ligand distamycin A, which binds to the strategically situated GC base pairs within the clusters of AT base pairs, has already been observed in Drosophila chromosomes revealing that quinacrine and distamycin A have a very specific target, which is not necessarily the same for the two (Dolfini Citation1987).
Heterochromatin accumulation at the chromosomal level in the sex chromosomes and at the pericentric region of the autosomes can be successfully employed as genetic markers for the evolutionary divergence among the sarcophagids. Although, qualitative differences in the heterochromatin observed in the present work indicate some implications of heterochromatin in the phylogenetic relationship of closely related species, supporting the earlier studies which have elaborated a possible functional role of heterochromatin in the process of karyological evolution, detailed investigation particularly at the molecular level will provide an intriguing challenge for further studies of heterochromatin.
Conflict of Interest
The authors declare they have no conflict of interest.
Acknowledgements
The authors would like to thank Prof. R. R. Tewari, Department of Zoology, University of Allahabad and Dr. Uma Rani Agrawal, Reader, CMP Degree College, Allahabad for their constant support to accomplish this work. Financial support in the form of DST- FIST and SAP funds is also thankfully acknowledged.
References
- Agrawal UR, Kaul D. 1990. Q-bands in the mitotic and polytene chromosomes of Boettcherisca nepalensis (Sarcophagidae: Diptera). National Seminar on Genetics. Mysore, pp. 21 ( Abs.).
- Arrighi FE, Hsu TC. 1971. Localization of heterochromatin in human chromosomes. Cytogenetics. 10:81–86.
- Arrighi FE, Hsu TC, Pathak S, Sawada H. 1974. The sex chromosomes of the Chinese hamster: Constitutive heterochromatin deficient in repetitive DNA sequences. Cytogenet Cell Genet. 13:268–274.
- Baimai V. 1998. Heterochromatin Accumulation and Karyotypic Evolution in Some Dipteran Insects. Zool Studies. 37(2):75–88.
- Behr W, Honikel K, Hartmann G. 1969. Interaction of the RNA polymerase inhibitor chromomycin with DNA. Eur J Biochem. 9:82–92.
- Bella JL, Fernandez JL, Gosalvez J. 1995. C-banding plus fluorochromes staining shows differences In C-, G-, and R-bands in human and mouse metaphase chromosomes. Genome. 38:864–868.
- Bianchi NO, Ayres J. 1971. Polymorphic patterns of Heterochromatin distribution in guinea pig chromosomes. Chromosoma. 34:254–260.
- Boyes JW, van Brink JM. 1965. Chromosomes of calyptrate Diptera. Can J Genet Cytol. 7:537–550.
- Brutlag DL, Appels R, Dennis ES, Peacock WJ. 1977. Highly repeated DNA in Drosophila melanogaster. J Mol Biol. 112:31–47.
- Bultmann H, Mezzanotte R. 1987. Characterization and origin of extrachromosomalDNA granules in Sarcophaga bullata. J Cell Sci. 88:327–334.
- Caspersson T, Farber S, Foley GE, Kudynowski J, Modest EJ, Simonsson E, Wagh U, Zech L. 1968. Chemical differentiation along metaphase chromosomes. Exp Cell Res. 49:219–222.
- Comings DE, Harris DC, Okada TA, Holmquist G. 1977. Nuclear proteins. IV. Deficiency of non-histone proteins in condensed chromatin of Drosophila virilis and mouse. Exp Cell Res. 105:349–365.
- Cons BMG, Fox KR. 1990. The GC-selective ligand mithramycin alters the structure of AT -sequences flanking its binding sites. FEBS Lett. 264(1):100–104.
- Cordeiro M, Wheeler L, Lee CS, Kastritsis CD, Richardson RH. 1975. Heterochromatic chromosomes and satellite DNA’s of Drosophila nasutoides. Chromosoma. 51:65–73.
- Cornaby BW. 1974. Carrion reduction by animals in contrasting tropical habitats. Biotropica. 6:51–63.
- De Souza MJ, De O, Haver PR, De Melo NF. 2003. Karyotype, C- and fluorescence banding patterns, NOR location and FISH in the grasshopper Xestotrachelus robustus (Romaleidae). Caryologia. 56(3):261–267.
- Dolfini FS. 1987. The effect of distamycin A on heterochromatin condensation of Drosophila chromosomes. Chromosoma. 95:57–62.
- Duhamel-Maestracci N, Simard R, Harbers K, Spencer JH. 1979. Localization of satellite DNAs in the chromosomes of the guinea pig. Chromosoma. 75:63–75.
- Ellison JR, Barr HJ. 1972. Quinacrine fluorescence of specific chromosome regions: Late replication and high AT content in Samoaia leonensis. Chromosoma. 36:375–390.
- Ennis TJ. 1974. Chromosome structure in Chilocorus (Coleoptera: Coccinellidae). I. Fluorescent and giemsa banding patterns. Canad J Genet Cytol. 16:651–661.
- Fox KR, Howarth NR. 1985. Investigations into the sequence-selective binding of mithramycin and related ligands to DNA. Nuc Acids Res. 13(24):8695–8714.
- Gall JG, Cohen EH, Polan ML. 1971. Repetitive DNA sequences in Drosophila. Chromosoma. 33:319–344.
- Galleni L, Stanyon R, Tellini A, Giordano C, Santini L. 1992. Karyology of the savi pine vole, Microtus savii (De Selys-Longchompl, 1838) (Rodentia, Arvico/idae) C-, G-, DA/DAPI, and AluI-bands. Cytogenet Cell Genet. 59:290–292.
- Gatti M, Pimpinelli S, Santini G. 1976. Characterization of Drosophila heterochromatin. I. Staining and decondensation with Hoechst 33258 and Quinacrine. Chromosoma. 57:351–375.
- Gaur P, Agrawal UR, Tewari RR, Kaul D. 1985. C-and Q-band polymorphisms in the mitotic chromosomes of flesh-flies belonging to the genus Parasarcophaga (Sarcophagidae: Diptera). La Kromosomo II. 39–40:1207–1213.
- Griffiths AJF, Gelbart WM, Miller JH, et al. 1999. Modern Genetic Analysis. W. H. Freeman: New York.
- Hennig W, Walker PMB. 1970. Variations in the DNA from two rodent families (Cricetidae and Muridae). Nature. 225:915–919.
- Hershfield B, Swift H. 1990. Characterization of a tandemly repeated DNA from the flesh fly Sarcophaga bullata. Chromosoma. 99:125–130.
- Hilwig I, Gropp A. 1972. Staining of constitutive heterochromatin in mammalian chromosomes with a new fluorochrome. Exp Cell Res. 75:122–126.
- Holmquist G. 1975. Hoechst 33258 fluorescent staining of Drosophila chromosomes. Chromosoma. 49:333–356.
- Hsu TC. 1973. Longitudinal differentiation of chromosomes. Ann Review Genet. 7:153–176.
- Jablonka E, Lamb MJ. 1990. The evolution of heteromorphic sex chromosomes. Biol Rev. 65:249–276.
- John B. 1988. The biology of heterochromatin. In: Heterochromatin Molecular and Structural Aspects (ed. R. S. Verma). Cambridge University Press, pp. 1–147.
- Kaul D, Chaturvedi R, Gaur P, Tewari RR. 1978. Cytogenetics of the Genus Parasarcophaga (Sarcophagidae: Diptera). Chromosoma. 68:73–82.
- Kaul D, Gaur P, Agrawal UR, Tewari RR. 1989. Characterization of Parasarcophaga heterochromatin. Chromosoma. 98:49–55.
- Lakhotia SC, Mishra A. 1980. Fluorescence patterns of heterochromatin in mitotic and polytene chromosomes in seven members of three subgroups of the melanogaster species group of Drosophila. Chromosoma. 81:137–150.
- Latt SA, Wohlleb JC. 1975. Optical studies of the interaction of 33258 Hoechst with DNA, chromatin, and metaphase chromosomes. Chromosoma. 52:297–316.
- Lee CS, Collins L. 1977. Q-and C-bands in the metaphase chromosomes of Drosophila nasutoides. Chromosoma. 61:57–60.
- Lin MS, Comings DE, Alfi OS. 1977. Optical studies of the interaction of 4’, 6-diamidino-2-phenyl indole with DNA and metaphase chromosomes. Chromosoma. 60:15–26.
- Maltempi PPP and Avancini RMP. 2000. Cytogenetics of the neotropical flesh fly Pattonella intermutans. Genet Mol Biol. 23:563–567.
- Muller W, Crothers DM. 1968. Studies of the binding of actinomycin and related compounds to DNA. J Mol Biol. 35:251–290.
- Rai V. 2001. DAPI fluorescence banding of Parasarcophaga argyrostoma chromosomes. Bioved. 12:49–52.
- Rai V, Agrawal UR, Kaul D. 1985. Characterization of Parasarcophaga ruficornis heterochromatin by DAPI/AMD staining. Chrom Inform Ser. 38:9–10.
- Santos N, De Souza MJ. 1998. Use of fluorochromes chromomycin A3 and DAPI to study constitutive heterochromatin and NORs in four species of bats (Phyllostomidae). Caryologia. 51 (3–4):265–278.
- Samols D, Swift H. 1979a. Genomic organization in the flesh fly Sarcophaga bullata. Chromosoma. 75:129–143.
- Samols D, Swift H. 1979b. Characterization of extrachromosomal DNA in the flesh fly Sarcophaga bullata. Chromosoma. 75:145–159.
- Schmid M, Haaf T, Geile B, Sims S. 1983. Chromosome banding in Amphibia. VIII. An unusual XY/XX sex chromosome system in Gastrotheca riobambae (Anura, Hylidae). Chromosoma. 88:69–82.
- Schmid M, Sims SH, Haaf T, Macgregor HC. 1986. Chromosome banding in Amphibia. Chromosoma. 94 (2):139–145.
- Schnedl W, Mikelsaar AV, Breitenbach M, Dann O. 1977. DIPI and DAPI: fluorescence banding with only negligible fading. Hum Genet. 36:167–172.
- Schweizer D. 1976a. Reverse fluorescent chromosome banding with chromomycin and DAPI. Chromosoma. 58:307–324.
- Schweizer D. 1976b. DAPI fluorescence of plant chromosomes pre stained with actinomycin D. Exp Cell Res. 102:408–413.
- Schweizer D. 1980. Simultaneous fluorescent staining of R bands and specific heterochromatic region (DA-DAPI bands) in human chromosomes. Cytogenet Cell Genet. 27:190–193.
- Souza MJ, Rufas JS, Orellana J. 1998. Constitutive heterochromatin, NOR location and FISH in the grasshopper Xyleus angulatus (Romaleidae). Caryologia. 51 (1):73–80.
- Srivastava R. 2009. Genomic characterisation of flesh-flies (Sarcophagidae: Diptera) D. Phil. Thesis, University of Allahabad, India.
- Srivastava R, Gaur P, Mishra N. 2013. Photo-oxidation and quinacrine fluorescence response in the mitotic chromosomes of the flesh-flies Sarcophaga. Int J Pharma Bio Sci. 4(1) ( B): 250 – 255.
- Tewari RR, Agrawal UR, Rai V, Kurahashi H. 1988. Fluorescence patterns of mitotic chromosomes of nine sarcophagids (Diptera). Genome. 30:250.
- Warchałowska-S′liwa E, Maryan′ska-Nadachowska A, Heller KG. 2007. Cytogenetic variability of the genus Saga Charp (Orthoptera, Tettigoniidae, Saginae): heterochromatin differentiation. Caryologia. 60 (1–2):29–36.
- Weisblum B, de Haseth PL. 1972. Quinacrine, a chromosome stain specific for deoxyadenylate - deoxythymidylate - rich regions in DNA. Proc Nat Acad Sci (USA). 69:629–632.
- Weisblum B, Haenssler E. 1974. Fluorometric properties of the bibenzimidazol derivative Hoechst 33258, a fluorescent probe specific for AT concentration in chromosomal DNA. Chromosoma. 46:255–260.