Abstract
Among a long list of parasites and pathogens that threaten the European honey bee (Apis mellifera), European Foulbrood (EFB) has become an urgent apiary disease, as epidemic outbreaks are becoming increasingly common. EFB is a bacterial disease of larval honey bees, caused by a gram-positive, anaerobic bacterium, Melissococcus plutonius. The most effective current treatment for EFB, oxytetracycline hydrochloride, can disrupt the bee microbiome, cause bee mortality and residues may persist in honey harvested for human consumption. In this study, we explore the efficacy of more sustainable bee-derived solutions, including propolis, honey comb, and brood comb ethanol extracts. Propolis has been shown to successfully inhibit the growth of a similar larval bee disease, American Foulbrood (AFB). Using a series of dilutions of these extracts, we determined the minimum inhibitory concertation (MIC) of each bee-derived product on M. plutonius, as well as two model bacterial species, Staphylococcus saprophyticus (gram-positive) and Escherichia coli (gram-negative). Overall, we found that propolis extract was most effective at inhibiting the growth of gram-positive bacteria, and that M. plutonius was also susceptible to honey comb (MIC = 16.00 mg/mL) and brood comb (MIC = 45.33 mg/mL) extracts, but at much higher concentrations than that of propolis (MIC = 1.14 mg/mL). As previously demonstrated for AFB, propolis is a promising natural defense against EFB, which could have important implications for hive management.
Introduction
The social life history of the European honey bee (Apis mellifera) is highly conducive to the spread of pathogens, such as foulbrood (i.e., a bacterial disease of the larvae). Both American Foulbrood and European Foulbrood diseases, named for the regions in which they were initially discovered (Milbrath, Citation2021), are present in honey bee colonies worldwide. American Foulbrood (AFB) is typically more devastating, as the causative agent Paenibacillus larvae is spore-forming, and spores are known to remain viable on beekeeping equipment for at least 35 years (Haseman, Citation1961). While antibiotics such as oxytetracycline hydrochloride (OTC) can be effective against P. larvae in the vegetative stage, they are ineffective against spores (Lodesani & Costa, Citation2005). Additionally, tetracycline antibiotics decrease the core microbiota of the honey bee gut, resulting in increased susceptibility to opportunistic bacteria and elevated mortality (Raymann et al., Citation2017; Soares et al., Citation2021). These changes have the potential to cause long-term effects on colony fitness (Bulson et al., Citation2021). Because antibiotics may simply mask AFB infection in a hive (Lodesani & Costa, Citation2005), colonies diagnosed with AFB are often killed and hive equipment destroyed by fire (Hansen & Brødsgaard, Citation1999), resulting in a substantial economic loss for beekeepers (estimated at 5 million USD/year; Eischen et al., Citation2005).
In contrast, European Foulbrood (EFB) is caused by Melisococcus plutonius, a non-spore-forming bacterium (Bailey, Citation1957), and is treatable with OTC (Forsgren, Citation2010). Still, treatment with OTC can be economically damaging as antibiotic residues can prevent beekeepers from selling honey (Gilliam & Argauer, Citation1981; Matsuka & Nakamura, Citation1990; Milbrath, Citation2021; Sporns et al., Citation1986; Wilson, Citation1974). Additionally, EFB symptoms often return following OTC treatment, and reports of outbreaks have increased worldwide (Dahle et al., Citation2011; Grant et al., Citation2021; Grossar et al., Citation2020; Masood et al., Citation2022; Roetschi et al., Citation2008; Simone-Finstrom & Spivak, Citation2010; Wilkins et al., Citation2007). EFB infection causes discoloration and loss of internal body pressure in affected larvae, leaving them yellow-brown and flaccid (Forsgren, Citation2010). M. plutonius is a gram-positive, microaerophilic to anaerobic bacterium that thrives in the larval honey bee midgut (Bailey, Citation1983; Bailey & Collins, Citation1982; Forsgren, Citation2010). Infected larvae pass on the bacterium by either defecating or dying within their wax cells, and bacteria are then spread to healthy larvae by adult nurse bees through larval care activities (Bailey, Citation1983), often resulting in the spread of EFB within a colony and throughout an apiary.
Propolis is a resinous substance that bees collect from plant exudates, and ultimately mix with saliva and beeswax through manipulation in the hive (Simone-Finstrom & Spivak, Citation2010). Bees use propolis to seal cracks in the hive body, reduce the hive entrance, and line the rims of wax cells (Evans & Spivak, Citation2010; Seeley & Morse, Citation1976; Strehle et al., Citation2003). In a natural setting, feral colonies create a “propolis envelope” around the entire surface of their rough nesting cavity (e.g., a tree cavity; Seeley & Morse, Citation1976), but this behavior is exhibited much less inside hive boxes made from finely milled lumber. Instead, propolis is frequently deposited between boxes, hive covers, and man-made frames that hold beeswax combs. Due to this meticulous behavior, propolis can be easily harvested for human use in folk medicine (Marcucci, Citation1995; Przybyłek & Karpiński, Citation2019). Propolis contains antimicrobial compounds, such as flavonoids, phenolics, terpenoids, and aromatic acids, that are present in the source plant exudates (Marcucci, Citation1995; Przybyłek & Karpiński, Citation2019). Constituent compounds can vary greatly depending on hive location and the availability of plants from which the bees collect resin (Huang et al., Citation2014; Sforcin, Citation2016). For example, propolis in temperate regions, such as Ohio (Johnson et al., Citation1994), originates mostly from Populus species (Anđelković et al., Citation2017; Sforcin, Citation2016) and largely contains flavonoids and phenolics. However, even propolis sourced from closely related plant species can be unique in their chemical constituents and capacity to inhibit pathogens (Wilson et al., Citation2013, Citation2015). Anthropogenic land use can also impact the chemical attributes of propolis; an example from Iowa demonstrates that propolis sourced from areas with more cropland is characterized by lower chemical diversity and antimicrobial activity (Orth et al., Citation2022).
Propolis has been shown to combat various pathogenic threats to bees, including fungal parasites like Vairimorpha spp. and Ascosphaera apis, the causative agents of Nosemosis and larval chalkbrood disease, respectively (Borba et al., Citation2015; Simone-Finstrom & Spivak, Citation2012; Wilson et al., Citation2015). It has also demonstrated effects against bee viral infections, including the deformed wing virus and black queen cell virus (Borba et al., Citation2015; Drescher et al., Citation2017). Moreover, bees from hives that were supplemented with additional propolis were found to exhibit lower expression of immune genes (Simone et al., Citation2009). Most notably, in the case of our research, propolis has also demonstrated antibacterial effects against P. larvae, the causative agent of AFB. The presence of a propolis envelope inside the nest increased the antibacterial activity of larval food in AFB-infected colonies and decreased the levels of clinical infection signs (Borba & Spivak, Citation2017). In vitro activity against P. larvae was also shown using propolis samples collected throughout the US and from Brazil (Antúnez et al., Citation2008; Bastos et al., Citation2008; Wilson et al., Citation2015). Though EFB and AFB are distinct larval pathogens, there is a considerable amount of overlap between diseased hive management. Thus, any implications of using propolis to better manage AFB should be further studied to inform decisions on EFB treatment as well.
With a recent increase in EFB outbreaks and the need for more sustainable treatments, we aimed to test the antibacterial properties of extracts from propolis and other bee-derived products (i.e., wax brood combs and honey combs) against M. plutonius. Despite strong evidence supporting the replacement of old, used brood combs (di Pinto et al., Citation2011; Johnson et al., Citation2010; Koenig et al., Citation1986; Mullin et al., Citation2010; Pankiw et al., Citation1970; Smith & Wilcox, Citation1990; Wu et al., Citation2011), we are interested in the effects of wax combs previously used for brood rearing on honey bee health, due to the deposition of both antimicrobial substances (i.e., propolis and honey; Pasupuleti et al., Citation2017) and bee frass over time. Bees use propolis to increase the structural integrity of all wax combs (Strehle et al., Citation2003), but brood comb darkens in color and gradually becomes a composite material, as the wax accumulates larval silk, castings, and frass with repeated cycles of brood rearing (Hepburn & Kurstjens, Citation1988). Due to this build-up of waste materials, as well as the potential for build-up of pesticide residues, beekeepers are often encouraged to replace their used brood comb after several years (Jaycox, Citation1979) However, the presence and potential benefits of propolis in wax cells is a relatively unexplored research topic. Surveys of beekeepers indicate that older brood comb may be associated with improved winter survival (https://research.beeinformed.org/survey/), possibly due to the propolis constituents or other components accumulated by wax through repeated brood-rearing cycles.
In the present study, we tested the antibacterial properties of ethanol extracts of (1) propolis, (2) brood comb (wax comb in which immature bees had previously been reared), and (3) honey comb (wax comb used exclusively for honey storage) against M. plutonious, the bacterial agent causing EFB. Additionally, these extracts were tested against two model bacteria, gram-negative Escherichia coli, and gram-positive Staphylococcus saprophyticus. The latter species are not associated with honey bee disease; however, they are pathogenic bacteria in mammals and are frequently used in antimicrobial testing. Historically, propolis extracts have not demonstrated substantial inhibition of gram-negative bacteria (Przybyłek & Karpiński, Citation2019), therefore, E. coli was included to serve as a negative control. We measured the optical density of all bacterial cultures after exposure to propolis, brood comb, and honey comb extracts to determine the lowest concentration, or minimum inhibitory concentration (MIC), that inhibits bacterial growth.
Materials and methods
Colony setup
A total of six experimental colonies were established in standard eight-frame Langstroth-style hive equipment at three apiary locations, with two colonies at each site, in the spring of 2018. Apiaries were located at (1) the Waterman Agriculture and Natural Resources Laboratory in Columbus, Ohio (40.0104° N, 83.0400° W), (2) the Ohio Agricultural Research and Development Center in Wooster, Ohio (40.7818° N, 81.9305° W), and (3) the Muck Crops Agricultural Research Station in Willard, Ohio (41.010200° N, 82.731350° W). New waxed black plastic foundation (9FDBW, Pierco) in new wooden frames were fitted in either newly constructed or thoroughly cleaned deep hive boxes (KD-603, Mann Lake). A new or thoroughly cleaned metal queen excluder (HD-121, Mann Lake) was used to restrict the queen and rearing of immature bees, or brood, to the bottom box so that the upper box could only be used for honey storage ().
Wax and propolis collection and extraction
Using a freshly cleaned hive tool, empty combs (free of honey or brood) and propolis samples were collected from each site in late-winter of 2019 (approximately ten months later) and stored in darkness at −20 °C until extraction. Before comb samples or propolis were extracted, they were first ground to a fine powder using liquid nitrogen and a food processor (FP2500B, Black & Decker). Separate food processors were used for each sample type to prevent cross contamination. Two grams of each powdered sample were placed in 20 mL of 95% ethanol (1:10 w/v) and sonicated (HB-S-49DHT, Kenda) for 60 min at up to 60 °C. The resulting extract was gravity filtered using grade one filter paper to remove particulate matter, evaporated under a stream of nitrogen at 20 °C, and resuspended in 10 mL of 70% ethanol. Ethanol was chosen as it is a commonly used solvent in propolis research and many of the antimicrobial compounds present are soluble in it. In total, nine crude ethanol extracts were made, three each of honey comb, brood comb and propolis corresponding to the three apiary locations. Concentrations were measured after evaporating crude extracts. The average concentration of dry material in crude extracts was 16.0 mg/mL for honey comb, 45.33 mg/mL for brood comb, and 72.83 mg/mL for propolis. A series of eight two-fold dilutions were then made for testing against bacteria. Diluted extract concentrations are listed in . All extracts were stored in darkness at 4 °C.
Figure 2. OD600 of (A) M. plutonius, (B) S. saprophyticus and (C) E. coli at mid-log phase when treated with a two-fold dilution series of 70% ethanol extracts of honey comb, brood comb and propolis. Comb and propolis were collected from three locations, with three technical replicates each (n = 9). Ethanol (70%) was used as a solvent control (EtOH) and oxytetracycline hydrochloride (1 mg/mL) was used as a positive control (OTC). Untreated wells were used as a standard growth control (Std.). MICs and controls significantly different (p < 0.05, Dunn’s Multiple Comparison test with Bonferonni correction) from solvent control are indicated with asterisks (*).
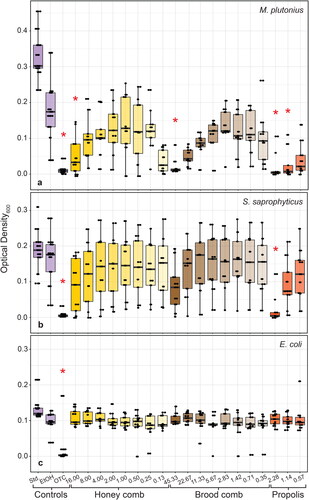
Bacterial cultures
M. plutonius was isolated from EFB-infected larvae collected at the Ohio State University Wooster Campus in the spring 2018. This microaerophilic-anaerobic bacterium was isolated and cultured by inoculating a modified basal medium (Forsgren et al., Citation2013) with fresh infected larvae that were ground with a sterile pestle in 1x PBS solution. Growth medium (Table S1) contained yeast extract, glucose, sucrose, L-cysteine, 1 M KH2PO4 and 2.5 M KOH (AC400405000, D16-500 and P285-500, Fisher Scientific; P5958 and W326305, Sigma Aldrich). To prevent the growth of secondary bacteria, nalidixic acid was dissolved in 0.1 M NaOH (AAB2509606 and S318-100, Fisher Scientific), filter sterilized, and added to the medium at a concentration of 3 µg/ml. M. plutonius was cultured in a vinyl anaerobic chamber (Coy Laboratory Products, Grass Lake, MI) at 37 °C (Table S1). Sequencing using primers from Haynes et al. (Citation2013) confirmed the identity of bacteria in culture (GenBank accession: MN886237). Cultures were stored in 50% glycerol for long-term storage at −80 °C. Strains of two aerobic bacterial species, E. coli (CSH36) and S. saprophyticus (ATCC15305), were obtained from collections of the Department of Microbiology at The Ohio State University and cultured using standard protocols in Mueller-Hinton media (OXCM0405B, Fisher Scientific) at 37 °C.
Bacterial growth inhibition assays
We determined the MIC of each treatment using a broth microdilution method modeled after the Clinical and Laboratory Standards Institute (Cockerill et al., Citation2012) as in Sozanski et al. (Citation2020). Before testing the inhibitory properties of each extract dilution series, bacterial isolates were first inoculated in broth media appropriate for each bacterium (aerobic Meuller-Hinton media or anaerobic M. plutonius media) and grown overnight at 37 °C under shaking aerobic conditions (E. coli and S. saprophyticus) or still anaerobic conditions (M. plutonius). To inoculate microplate test wells (96-well flat-bottomed microplates, Thermo Scientific™ 243656) at 5 x 105 CFU/mL, we first diluted overnight cultures to a 0.5 McFarland standard (1.0 × 108 CFU/mL) and further diluted cultures by a factor of five. We then added 5 µL of these diluted cultures to plate wells containing 5 µL of either antimicrobial treatments or controls and 190 µL of appropriate broth media, resulting in a final concentration of 5 x 105 CFU/mL. Each column of wells on the 96-well plate contained the series of two-fold diluted propolis, brood comb or honey comb extracts, with column one containing the most concentrated treatment and column eight containing the most dilute treatment. Columns nine and 10 contained a positive oxytetracycline (OTC) control (1 mg/mL) and a negative solvent control (70% ethanol), respectively. The final two columns on the plate served as a standard growth control and contamination control. Standard growth control wells contained 195 µL of appropriate broth with 5 µL of diluted culture. Finally, contamination control wells contained 200 µL of appropriate broth. Each 96-well plate was considered one technical replicate. For every extract and bacterium tested, there were three biological replicates, corresponding to the three different source apiaries, and three technical replicates (n = 9).
To measure bacterial growth, plates were placed in an ELx808i™ Absorbance Microplate Reader (BioTek Instruments) with incubation at 37 °C. Plates were shaken and an absorbance reading was made at 600 nm (OD600) every five minutes for 12 h (Quigley, Citation2008). Standard growth controls for each plate were used to determine the mid-log phase of bacterial growth, allowing MIC determination at that time point for each treatment.
Statistical analysis and MIC determination
We first analyzed standard growth wells to determine the mid-log phase of each plate using the R package Growthcurver v3.6.0 (Sprouffske & Wagner, Citation2016). We recorded OD600 readings for bacterial growth in all wells at their corresponding mid-log phase and tested each plate for growth differences among treatments using a Kruskal-Wallis test (supplemental data, including raw growth curves, and R scripts, are openly available in Figshare at https://doi.org/10.6084/m9.figshare.19387442). Dunn’s Multiple Comparison post-hoc test with Bonferroni correction was used to determine the MIC of each treatment against all three bacteria. We defined the MIC as the lowest treatment concentration that was significantly different from the solvent control. Propolis dilutions one through five were ultimately excluded from analysis, as these ethanol extracts were oversaturated with propolis, resulting in inconsistent OD readings. All statistics were performed in R v4.0.3 (R Core Team, Citation2019).
Results
Growth of M. plutonius was inhibited by OTC (p < 0.001) and all three hive product extracts (). The MIC of honey comb extract (p = 0.007) and brood comb extract (p < 0.001) was 16.00 mg/mL and 45.33 mg/mL, respectively. The MIC of propolis extract against M. plutonius was the second-lowest concentration tested, 1.14 mg/mL (p < 0.001). Growth of S. saprophyticus was inhibited only by OTC (p < 0.001) and propolis extract (; p = 0.001). The MIC of propolis extract against S. saprophyticus was 2.28 mg/mL. Growth of E. coli was not significantly inhibited by any extract but was inhibited by OTC (; p = 0.001). Statistical analyses also revealed a significant effect by plate for each bacterium (p < 0.001), as well as a significant effect of extract source location for S. saprophyticus (p < 0.001) and M. plutonius (p = 0.032).
Discussion
In this study, we set out to test the antibacterial effects of ethanol extracts of three hive products—propolis, brood comb, and honey comb—against M. plutonius, the causative agent of EFB in honey bees. Overall, M. plutonius growth was susceptible to inhibition by extracts of all three hive products. To our knowledge, this is the first study to test the antibacterial properties of propolis and wax combs against M. plutonius. The highest concentrations of both honey comb (16.00 mg/mL) and brood comb (45.33 mg/mL) extracts successfully inhibited the growth of M. plutonius, but MICs for these extracts were an order of magnitude higher than that of propolis extract (1.14 mg/mL; ). We demonstrated strong antibacterial properties of propolis against M. plutonius, at roughly the same concentration as our positive control OTC (1.0 mg/mL). The gram-positive model bacterium, S. saprophyticus, was more susceptible than E. coli to inhibition by propolis extract (MIC = 2.28 mg/mL; ), which is consistent with previous work showing that propolis extracts have more inhibitory activity against gram-positive bacteria (Rahman et al., Citation2010; Ristivojević et al., Citation2016; Sforcin et al., Citation2000; Tosi et al., Citation1996; Velikova et al., Citation2000). This tolerance is likely due to the structure of gram-negative bacterial cells, which are characterized by a thin layer of peptidoglycans and an extra plasma membrane, that is lacking in gram-positive bacterial cells (Harrop et al., Citation1989); however, the composition of these outer membranes—and thus the tolerance to propolis activity—may be species-dependent (Mirzoeva et al., Citation1997). In line with previous work, propolis extracts did not inhibit E. coli growth. Future studies should consider the use of more bee-relevant model bacteria species, such as Serratia marcescens (Raymann et al., Citation2018).
We observed significant differences between experimental plates, as well as between materials collected from different apiary locations. For M. plutonius growth, the significant difference in hive product source locations is between two rural sites (Wooster and Willard, OH, p < 0.001). For S. saprophyticus growth, the significant difference is between the urban site and both rural sites (Columbus and Wooster, OH, p < 0.001; Columbus and Willard, OH, p < 0.001). Though our hives were located within 200 miles of one another, it is likely that propolis extracts from each location differ in their chemical fingerprints. Without chemical analysis, we cannot rule out the possibility that our propolis extracts differ in their abilities to inhibit bacteria (Wilson et al., Citation2013, Citation2015).
The antibiotic properties of propolis are well-established (Marcucci, Citation1995; Przybyłek & Karpiński, Citation2019), but this is not the case for honey comb and brood comb. Our results suggest that antibacterial compounds are much more abundant in propolis but are also present in wax combs and can serve to inhibit the growth of M. plutonius at higher concentrations. Understanding the origins of this activity could inform beekeeping practices, such as brood comb replacement.
Beeswax itself is made up of hydrocarbon chains, free fatty acids, free fatty alcohols and wax esters, but also contains plant-derived materials (e.g., nectar, pollen, resin) that are incorporated as honey bees build and fill combs (Fratini et al., Citation2016). Due to its lipophilic composition, beeswax captures many compounds, including pheromones and acaracides (Svečnjak et al., Citation2019). Therefore, we assume that antimicrobial effects of wax comb extracts are likely due to other compounds incorporated during and after comb construction. To disentangle any antibacterial effects of beeswax itself from those of other compounds taken up by or incorporated into the wax, future research should focus on collecting wax scales directly from bees (as in Svečnjak et al., Citation2019).
In a healthy colony, honey and pollen are often stored in wax cells that were previously used by developing brood (Schneider, Citation2015), but by using a queen excluder (; a common practice for hobbyists and large-scale beekeepers) it is possible to encourage bees to store honey in cells that were never used for brood rearing. We used queen excluders to separately test the effects of comb used for food storage (honey comb) vs. comb used for brood development (brood comb). Like propolis, honey is another bee-derived substance that has long been used in human medicine (Bogdanov et al., Citation2008). The antimicrobial properties of honey derived from its low pH, flavonoids present in pollen and nectar, as well as enzymes originating from both plants and honey bee hypopharyngeal gland secretions (Aurongzeb & Azim, Citation2011). Though we collected honey comb free of honey to make our ethanol extracts, there were likely traces of honey present, and previous honey storage may have incorporated compounds, such as flavonoids, into the wax cells.
Brood comb, on the other hand, is a composite material containing silk, frass, propolis and exogenous plant material (Hepburn & Kurstjens, Citation1988), resulting in wax cells that are darker and thicker than those that are used strictly for honey storage. However, studies on the quantity of propolis in brood combs or honey combs, and whether those quantities differ between comb type, do not exist. The propolis envelope that surrounds the entire nest cavity of a feral colony has been hypothesized to have several homeostatic functions, including antimicrobial protection, water-repellency and even communication (Seeley & Morse, Citation1976). This extensive envelope is not present in managed colonies, but we know propolis is incorporated into the rims of wax cells, possibly to serve a structural function (Strehle et al., Citation2003) or to confer an antimicrobial benefit (Evans & Spivak, Citation2010). These hypotheses remain relatively unexplored (Hepburn & Kurstjens, Citation1988). Though there are potential benefits from propolis build-up in well-used, composite comb, there is still concern over the build-up of pesticide residues and pathogen persistence in old brood combs, which currently informs brood comb replacement practices.
Propolis as a potential preventative or treatment for EFB
With well-established antibacterial properties, and demonstrated activity against M. plutonius, propolis and propolis extracts should be further investigated for their use in the control of EFB in honey bee colonies. Our study did not identify the chemical constituents that may contribute to its antibacterial properties. Further studies should work to isolate the active compounds in antibacterial propolis extracts, and moreover, identify concentrations of each active compound that are effective against M. plutonius. Previously, well-known constituents of poplar propolis, pinocembrin, 3-O-acetyl pinobanksin, and several caffeic acid esters, were identified as the most active compounds in Bulgarian propolis against P. larvae (Bilikova et al., Citation2013). Similarly, several 3-acyl-dihydroflavanols were isolated from poplar propolis in Nevada and demonstrated inhibition against P. larvae (Wilson et al., Citation2017). Given the similarities in hive management for AFB and EFB, compounds found to inhibit P. larvae present another great starting point for testing effectiveness against M. plutonius.
There is now a growing body of literature supporting the benefits of propolis in honey bee immunity, and bee stocks artificially selected for higher propolis collection demonstrate increased survivorship and longevity (Nicodemo et al., Citation2014). Aside from breeding bees for increased propolis collection, two of the most practical ways to supplement colonies with propolis include applying propolis extract to colony walls or stimulating propolis collection through hive design (i.e., propolis traps with many services; Borba et al., Citation2015; Drescher et al., Citation2017). Colonies supplemented with propolis exhibit lower viral infection rates and immune gene expression (Borba et al., Citation2015; Drescher et al., Citation2017; Simone et al., Citation2009). As a bee-derived substance that is (1) already present in the hive, (2) continually changing in its chemical constituents (based on available flora), and (3) safe for humans when present in harvested honey (unlike OTC; Gilliam & Argauer, Citation1981; Matsuka & Nakamura, Citation1990; Sporns et al., Citation1986; Wilson, Citation1974), propolis shows promise as a sustainable preventative or control for EFB outbreaks. Field experiments using propolis traps or extracts, as well as in-vitro experiments (as in Grossar et al., Citation2020) in which EFB-infected larvae are treated with topical or ingestible propolis extracts, are the next step to evaluating the effectiveness of propolis to manage EFB.
Supplemental Material
Download MS Word (15 KB)Acknowledgements
Tyler Eaton sequenced and confirmed our M. plutonius isolate. Robert Filbrun assisted with colony placement and management at Muck Crops Agricultural Research Station. Thaddeus Ezeji, Christopher Okonkwo, and Christopher Madden contributed materials, time, and guidance in anaerobic microbiology. Larry Phelan and Gerald Carter contributed time and guidance in data analysis. Sreelakshmi Suresh assisted in data processing.
Disclosure statement
No potential competing interest was reported by the authors.
Additional information
Funding
References
- Anđelković, B., Vujisić, L., Vučković, I., Tešević, V., Vajs, V., & Gođevac, D. (2017). Metabolomics study of Populus type propolis. Journal of Pharmaceutical and Biomedical Analysis, 135, 217–226. https://doi.org/10.1016/j.jpba.2016.12.003
- Antúnez, K., Harriet, J., Gende, L., Maggi, M., Eguaras, M., & Zunino, P. (2008). Efficacy of natural propolis extract in the control of American Foulbrood. Veterinary Microbiology, 131(3–4), 324–331. https://doi.org/10.1016/j.vetmic.2008.04.011
- Aurongzeb, M., & Azim, M. K. (2011). Antimicrobial properties of natural honey: A review of literature. Pakistan Journal of Biochemistry and Molecular Biology, 44(3), 118–124.
- Bailey, L. (1957). The cause of European Foulbrood. Bee World, 38(4), 85–89. https://doi.org/10.1080/0005772X.1957.11094983
- Bailey, L. (1983). Melissococcus pluton, the cause of European foulbrood of honey bees (Apis spp. Journal of Applied Bacteriology, 55(1), 65–69.) https://doi.org/10.1111/j.1365-2672.1983.tb02648.x
- Bailey, L., & Collins, M. D. (1982). Reclassification of 'Streptococcus pluton’ (White) in a new genus Melissococcus pluton nom. rev.; comb. nov. Journal of Applied Bacteriology, 53(2), 215–217. https://doi.org/10.1111/j.1365-2672.1982.tb04679.x
- Bastos, E. M. A. F., Simone, M., Jorge, D. M., Soares, A. E. E., & Spivak, M. (2008). In vitro study of the antimicrobial activity of Brazilian propolis against Paenibacillus larvae. Journal of Invertebrate Pathology, 97(3), 273–281. https://doi.org/10.1016/j.jip.2007.10.007
- Bilikova, K., Popova, M., Trusheva, B., & Bankova, V. (2013). New anti-Paenibacillus larvae substances purified from propolis. Apidologie, 44(3), 278–285. https://doi.org/10.1007/s13592-012-0178-1ï
- Bogdanov, S., Jurendic, T., Sieber, R., & Gallmann, P. (2008). Honey for nutrition and health: A review. Journal of the American College of Nutrition, 27(6), 677–689. https://doi.org/10.1080/07315724.2008.10719745
- Borba, R. S., Klyczek, K. K., Mogen, K. L., & Spivak, M. (2015). Seasonal benefits of a natural propolis envelope to honey bee immunity and colony health. The Journal of Experimental Biology, 218(Pt 22), 3689–3699. https://doi.org/10.1242/jeb.127324
- Borba, R. S., & Spivak, M. (2017). Propolis envelope in Apis mellifera colonies supports honey bees against the pathogen, Paenibacillus larvae. Scientific Reports, 7(1). https://doi.org/10.1038/s41598-017-11689-w
- Bulson, L., Becher, M. A., McKinley, T. J., & Wilfert, L. (2021). Long-term effects of antibiotic treatments on honeybee colony fitness: A modelling approach. The Journal of Applied Ecology, 58(1), 70–79. https://doi.org/10.1111/1365-2664.13786
- Cockerill, F. R., Wikler, M. A., Alder, J., Dudley, M. N., Eliopoulos, G. M., Ferraro, M. J., Hardy, D. J., Hecht, D. W., Hindler, J. A., Patel, J. B., Powell, M., Swenson, J. M., Thomson, R. B., Traczewski, M. M., Turnidge, J. D., Weinstein, M. P., Zimmer, B. L. (2012). Methods for dilution antimicrobial susceptibility tests for bacteria that grow aerobically (9th ed., Vol. 32). National Committee for Clinical Laboratory Standards. www.clsi.org.
- Dahle, B., Sørum, H., Budge, G., & Weideman, J. E. (2011). How to get rid of EFB in Norway. In Proceedings of the 7th COLOSS Conference, p. 20.
- di Pinto, A., Novello, L., Terio, V., & Tantillo, G. (2011). ERIC-PCR genotyping of Paenibacillus larvae in Southern Italian honey and brood combs. Current Microbiology, 63(5), 416–419. https://doi.org/10.1007/s00284-011-9996-z
- Drescher, N., Klein, A. M., Neumann, P., Yañez, O., & Leonhardt, S. D. (2017). Inside honeybee hives: Impact of natural propolis on the ectoparasitic mite Varroa destructor and viruses. Insects, 8(1), 15–8010015. https://doi.org/10.3390/insects8010015
- Eischen, F. A., Graham, R. H., & Cox, R. (2005). Regional distribution of Paenibacillus larvae subspecies larvae, the causative organism of American Foulbrood, in honey bee colonies of the western United States. Journal of Economic Entomology, 98(4), 1087–1093. https://academic.oup.com/jee/article/98/4/1087/883400, https://doi.org/10.1603/0022-0493-98.4.1087
- Evans, J. D., & Spivak, M. (2010). Socialized medicine: Individual and communal disease barriers in honey bees. Journal of Invertebrate Pathology, 103, S62–S72. https://doi.org/10.1016/j.jip.2009.06.019
- Forsgren, E. (2010). European foulbrood in honey bees. Journal of Invertebrate Pathology, 103, S5–S9. https://doi.org/10.1016/j.jip.2009.06.016
- Forsgren, E., Budge, G. E., Charrière, J. D., & Hornitzky, M. A. Z. (2013). Standard methods for European foulbrood research. Journal of Apicultural Research, 52(1), 1–14. https://doi.org/10.3896/IBRA.1.52.1.12
- Fratini, F., Cilia, G., Turchi, B., & Felicioli, A. (2016). Beeswax: A minireview of its antimicrobial activity and its application in medicine. Asian Pacific Journal of Tropical Medicine, 9(9), 839–843. https://doi.org/10.1016/j.apjtm.2016.07.003
- Gilliam, M., & Argauer, R. J. (1981). Oxytetracycline residues in surplus honey, brood nest honey, and larvae after medication of colonies of honey bees, Apis mellifera, with antibiotic extender patties, sugar dusts, and syrup sprays. Environmental Entomology, 10(4), 479–482. https://academic.oup.com/ee/article/10/4/479/2392625 https://doi.org/10.1093/ee/10.4.479
- Grant, K. J., DeVetter, L., & Melathopoulos, A. (2021). Honey bee (Apis mellifera) colony strength and its effects on pollination and yield in highbush blueberries (Vaccinium corymbosum). PeerJ, 9, e11634. https://doi.org/10.7717/peerj.11634
- Grossar, D., Kilchenmann, V., Forsgren, E., Charrière, J. D., Gauthier, L., Chapuisat, M., & Dietemann, V. (2020). Putative determinants of virulence in Melissococcus plutonius, the bacterial agent causing European foulbrood in honey bees. Virulence, 11(1), 554–567. https://doi.org/10.1080/21505594.2020.1768338
- Hansen, H., & Brødsgaard, C. J. (1999). American foulbrood: A review of its biology, diagnosis and control. Bee World, 80(1), 5–23. https://doi.org/10.1080/0005772X.1999.11099415
- Harrop, A. J., Hocknull, M. D., & Lilly, M. D. (1989). Biotransformation in organic solvents: A difference between gram-positive and gram-negative bacteria. Biotechnology Letters, 11(11), 807–810. https://doi.org/10.1007/BF01026102
- Haseman, L. (1961). How long can spores of American foulbrood live? American Bee Journal, 101, 298–299.
- Haynes, E., Helgason, T., Young, J. P. W., Thwaites, R., & Budge, G. E. (2013). A typing scheme for the honeybee pathogen Melissococcus plutonius allows detection of disease transmission events and a study of the distribution of variants. Environmental Microbiology Reports, 5(4), 525–529. https://doi.org/10.1111/1758-2229.12057
- Hepburn, H. R., & Kurstjens, S. P. (1988). The combs of honeybees as composite materials. Apidologie, 19(1), 25–36. https://doi.org/10.1051/apido:19880102
- Huang, S., Zhang, C. P., Wang, K., Li, G. Q., & Hu, F. L. (2014). Recent advances in the chemical composition of propolis. Molecules (Basel, Switzerland), 19(12), 19610–19632. https://doi.org/10.3390/molecules191219610
- Jaycox, E. R. (1979). Comb foundation: Are we using enough? American Bee Journal, 119(7), 515–516.
- Johnson, K. S., Eischen, F. A., & Giannasi, D. E. (1994). Chemical composition of North America bee propolis and biological activity towards larvae of greater wax moth (Lepidoptera: Pyralidae). Journal of Chemical Ecology, 20(7), 1783–1791. https://doi.org/10.1007/BF02059899
- Johnson, R. M., Ellis, M. D., Mullin, C. A., & Frazier, M. (2010). Pesticides and honey bee toxicity - USA. Apidologie, 41(3), 312–331. https://doi.org/10.1051/apido/2010018
- Koenig, J. P., Boush, G. M., & Erickson, E. H. (1986). Effect of type of brood comb on chalk brood disease in honeybee colonies. Journal of Apicultural Research, 25(1), 58–62. https://doi.org/10.1080/00218839.1986.11100694
- Lodesani, M., & Costa, C. (2005). Limits of chemotherapy in beekeeping: Development of resistance and the problem of residues. Bee World, 86(4), 102–109. https://doi.org/10.1080/0005772X.2005.11417324
- Marcucci, M. C. (1995). Propolis: Chemical composition, biological properties and therapeutic activity. Apidologie, 26(2), 83–99. https://doi.org/10.1051/apido:19950202
- Masood, F., Thebeau, J. M., Cloet, A., Kozii, I. v., Zabrodski, M. W., Biganski, S., Liang, J., Marta Guarna, M., Simko, E., Ruzzini, A., & Wood, S. C. (2022). Evaluating approved and alternative treatments against an oxytetracycline-resistant bacterium responsible for European foulbrood disease in honey bees. Scientific Reports, 12(1). https://doi.org/10.1038/s41598-022-09796-4
- Matsuka, M., & Nakamura, J. (1990). Oxytetracycline residues in honey and royal jelly. Journal of Apicultural Research, 29(2), 112–117. https://doi.org/10.1080/00218839.1990.11101206
- Milbrath, M. (2021). Honey bee bacterial diseases. In T. R. Kane & C. M. Faux (Eds.), Honey bee medicine for the veterinary practitioner (1st ed., pp. 277–293). John Wiley & Sons.
- Mirzoeva, O. K., Grishanin, R. N., & Calder, P. C. (1997). Antimicrobial action of propolis and some of its components: The effects on growth, membrane potential and motility of bacteria. Microbiological Research, 152(3), 239–246. https://doi.org/10.1016/S0944-5013(97)80034-1
- Mullin, C. A., Frazier, M., Frazier, J. L., Ashcraft, S., Simonds, R., vanEngelsdorp, D., & Pettis, J. S. (2010). High levels of miticides and agrochemicals in North American apiaries: Implications for honey bee health. PloS One, 5(3), e9754. https://doi.org/10.1371/journal.pone.0009754
- Nicodemo, D., Malheiros, E. B., De Jong, D., & Couto, R. H. N. (2014). Increased brood viability and longer lifespan of honeybees selected for propolis production. Apidologie, 45(2), 269–275. https://doi.org/10.1007/s13592-013-0249-y
- Orth, A. J., Curran, E. H., Haas, E. J., Kraemer, A. C., Anderson, A. M., Mason, N. J., & Fassbinder-Orth, C. A. (2022). Land use influences the composition and antimicrobial effects of propolis. Insects, 13(3), 239. https://doi.org/10.3390/insects13030239
- Pankiw, P., Bailey, L., Gochnauer, T. A., & Hamilton, H. A. (1970). Disinfection of honeybee combs by gamma irradiation. II. European foul brood disease. Journal of Apicultural Research, 9(3), 165–168. https://doi.org/10.1080/00218839.1970.11100263
- Pasupuleti, V. R., Sammugam, L., Ramesh, N., & Gan, S. H. (2017). Honey, propolis, and royal jelly: A comprehensive review of their biological actions and health benefits. Oxidative Medicine and Cellular Longevity, 2017, 1259510. https://doi.org/10.1155/2017/1259510
- Przybyłek, I., & Karpiński, T. M. (2019). Antibacterial properties of propolis. Molecules, 24(11), 2047. https://doi.org/10.3390/molecules24112047
- Quigley, T. (2008). Monitoring the growth of E. coli with light scattering using the SynergyTM 4 Multi-Mode Microplate Reader with Hybrid TechnologyTM. BioTek. www.biotek.com
- R Core Team. (2019). R: A language and environment for statistical computing. https://www.R-project.org/
- Rahman, M. M., Richardson, A., & Sofian-Azirun, M. (2010). Antibacterial activity of propolis and honey against Staphylococcus aureus and Escherichia coli. African Journal of Microbiology Research, 4(16), 1872–1878. https://www.researchgate.net/publication/228662460
- Raymann, K., Coon, K. L., Shaffer, Z., Salisbury, S., & Moran, N. A. (2018). Pathogenicity of Serratia marcescens strains in honey bees. mBio, 9(5), 01649–01618. https://doi.org/10.1128/mBio
- Raymann, K., Shaffer, Z., & Moran, N. A. (2017). Antibiotic exposure perturbs the gut microbiota and elevates mortality in honeybees. PLoS Biology, 15(3), e2001861. https://doi.org/10.1371/journal.pbio.2001861
- Ristivojević, P., Dimkić, I., Trifković, J., Berić, T., Vovk, I., Milojkovič-Opsenica, D., & Stanković, S. (2016). Antimicrobial activity of Serbian propolis evaluated by means of MIC, HPTLC, bioautography and chemometrics. PloS One, 11(6), e0157097. https://doi.org/10.1371/journal.pone.0157097
- Roetschi, A., Berthoud, H., Kuhn, R., & Imdorf, A. (2008). Infection rate based on quantitative real-time PCR of Melissococcus plutonius, the causal agent of European foulbrood, in honeybee colonies before and after apiary sanitation. Apidologie, 39(3), 362–371. https://doi.org/10.1051/apido:200819
- Schneider, S. S. (2015). The honey bee colony: Life history. In J. M. Graham (Ed.), The hive and the honey bee (pp. 73–109). Dadant & Sons.
- Seeley, T. D., & Morse, R. A. (1976). The nest of the honey bee (Apis mellifera L.). Insectes Sociaux, 23(4), 495–512. https://doi.org/10.1007/BF02223477
- Sforcin, J. M. (2016). Biological properties and therapeutic applications of propolis. Phytotherapy Research: PTR, 30(6), 894–905. https://doi.org/10.1002/ptr.5605
- Sforcin, J. M., Fernandes, A., Lopes, C. A. M., Bankova, V., & Funari, S. R. C. (2000). Seasonal effect on Brazilian propolis antibacterial activity. Journal of Ethnopharmacology, 73(1-2), 243–249. www.elsevier.com/locate/jethpharm https://doi.org/10.1016/s0378-8741(00)00320-2
- Simone, M., Evans, J. D., & Spivak, M. (2009). Resin collection and social immunity in honey bees. Evolution; International Journal of Organic Evolution, 63(11), 3016–3022. https://doi.org/10.1111/j.1558-5646.2009.00772.x
- Simone-Finstrom, M. D., & Spivak, M. (2012). Increased resin collection after parasite challenge: A case of self-medication in honey bees? PloS One, 7(3), e34601. https://doi.org/10.1371/journal.pone.0034601
- Simone-Finstrom, M., & Spivak, M. (2010). Propolis and bee health: The natural history and significance of resin use by honey bees. Apidologie, 41(3), 295–311. https://doi.org/10.1051/apido/2010016
- Smith, R. K., & Wilcox, M. M. (1990). Chemical residues in bees, honey and beeswax. American Bee Journal, 130(3), 188–192.
- Soares, K. O., Oliveira, C. J. B., Rodrigues, A., E., Vasconcelos, P. C., Silva, N. M. V. D., Cunha Filho, O. G. D., Madden, C., & Hale, V. L. (2021). Tetracycline exposure alters key gut microbiota in Africanized honey bees (Apis mellifera scutellata x spp.). Frontiers in Ecology and Evolution, 9, 716660. https://doi.org/10.3389/fevo.2021.716660
- Sozanski, K., Prado, L. P. d., Mularo, A. J., Sadowski, V. A., Jones, T. H., & Adams, R. M. M. (2020). Venom function of a new species of Megalomyrmex Forel, 1885 (Hymenoptera: Formicidae). Toxins, 12(11), 679. https://doi.org/10.3390/toxins12110679
- Sporns, P., Kwan, S., & Roth, L. A. (1986). HPLC analysis of oxytetracycline residues in honey. Journal of Food Protection, 49(5), 383–388. http://meridian.allenpress.com/jfp/article-pdf/49/5/383/1657041/0362-028x-49_5_383.pdf https://doi.org/10.4315/0362-028X-49.5.383
- Sprouffske, K., & Wagner, A. (2016). Growthcurver: An R package for obtaining interpretable metrics from microbial growth curves. BMC Bioinformatics, 17(1), 172. https://doi.org/10.1186/s12859-016-1016-7
- Strehle, M. A., Jenke, F., Fröhlich, B., Tautz, J., Riederer, M., Kiefer, W., & Popp, J. (2003). Raman spectroscopic study of spatial distribution of propolis in comb of Apis mellifera carnica (Pollm.). Biopolymers, 72(4), 217–224. https://doi.org/10.1002/bip.10379
- Svečnjak, L., Chesson, L. A., Gallina, A., Maia, M., Martinello, M., Mutinelli, F., Muz, M. N., Nunes, F. M., Saucy, F., Tipple, B. J., Wallner, K., Waś, E., & Waters, T. A. (2019). Standard methods for Apis mellifera beeswax research. Journal of Apicultural Research, 58(2), 1–108. https://doi.org/10.1080/00218839.2019.1571556
- Tosi, B., Donini, A., Romagnoli, C., & Bruni, A. (1996). Antimicrobial activity of some commercial extracts of propolis prepared with different solvents. Phytotherapy Research, 10(4), 335–336. https://doi.org/10.1002/(SICI)1099-1573(199606)10:4<335::AID-PTR828>3.0.CO;2-7
- Velikova, M., Bankova, V., Marcucci, M. C., Tsvetkova, I., & Kujumgiev, A. (2000). Chemical composition and biological activity of propolis from Brazilian Meliponinae. Zeitschrift Fur Naturforschung. C, Journal of Biosciences, 55(9–10), 785–789. www.znaturforsch.com https://doi.org/10.1515/znc-2000-9-1018
- Wilkins, S., Brown, M. A., & Cuthbertson, A. G. S. (2007). The incidence of honey bee pests and diseases in England and Wales. Pest Management Science, 63(11), 1062–1068. https://doi.org/10.1002/ps.1461
- Wilson, W. T. (1974). Residues of oxytetracycline in honey stored by Apis mellifera. Environmental Entomology, 3(4), 674–676. https://academic.oup.com/ee/article/3/4/674/2395300 https://doi.org/10.1093/ee/3.4.674
- Wilson, M. B., Brinkman, D., Spivak, M., Gardner, G., & Cohen, J. D. (2015). Regional variation in composition and antimicrobial activity of US propolis against Paenibacillus larvae and Ascosphaera apis. Journal of Invertebrate Pathology, 124, 44–50. https://doi.org/10.1016/j.jip.2014.10.005
- Wilson, M. B., Pawlus, A. D., Brinkman, D., Gardner, G., Hegeman, A. D., Spivak, M., & Cohen, J. D. (2017). 3-Acyl dihydroflavonols from poplar resins collected by honey bees are active against the bee pathogens Paenibacillus larvae and Ascosphaera apis. Phytochemistry, 138, 83–92. https://doi.org/10.1016/j.phytochem.2017.02.020
- Wilson, M. B., Spivak, M., Hegeman, A. D., Rendahl, A., & Cohen, J. D. (2013). Metabolomics reveals the origins of antimicrobial plant resins collected by honey bees. PLoS One, 8(10), e77512. https://doi.org/10.1371/journal.pone.0077512
- Wu, J. Y., Anelli, C. M., & Sheppard, W. S. (2011). Sub-lethal effects of pesticide residues in brood comb on worker honey bee (Apis mellifera) development and longevity. PloS One, 6(2), e14720. https://doi.org/10.1371/journal.pone.0014720