Abstract
The concept of energy serves biologists as a powerful analytical model to describe phenomena that occurs in the natural world. Due to the concept’s relevance, educational standards of different countries identify energy as a core idea for the teaching and learning of biology and other science subjects. However, previous research on students’ energy understanding has mostly focused on physics contexts. This cross-sectional study extends insight to the field by providing a systematic analysis of students’ (N = 30, grades 5, 7, 9, 11) conceptions about energy in biological contexts. In order to connect the findings to previous research, the study analyses conceptions about four energy aspects that are regarded as central for understanding the concept in different disciplinary contexts, i.e. (1) energy forms/sources, (2) transfer/transformation, (3) degradation/dissipation and (4) energy conservation. The findings identify substantial changes in students’ conceptions about energy between the different grade levels, but also highlight conceptions that students consistently employed across age groups. The results are discussed in the light of previous research on students’ progressing energy understanding and the connection of their energy understanding across different disciplinary contexts. Lastly, the article provides implications for the further development of energy teaching in biological contexts.
Introduction
Biology describes processes in the living world that, without exception, rely on the transformation of energy. Energy flows through different trophic levels of an ecosystem. To build up and maintain ordered structures that support their lives, organisms need to take up and transform free energy, either by using sunlight in photosynthesis or through catabolic pathways that oxidise chemical compounds. As energy sources are often limited, the survival and evolutionary success of organisms depends also on the efficiency of an organism’s energy transformations (Harms Citation2016; Reece et al. Citation2011, 148–149). Energy is thus a major concept for the analysis of all biological processes, for example, when determining the extent to which specific changes can occur in a living system (Reece et al. Citation2011, 143, 146).
Due to the concept’s relevance in science, energy is considered as a core (or big) idea for the teaching and learning of biology (e.g. Department for Education (DfE) Citation2013; National Research Council (NRC) Citation2012). In schools, energy is a versatile concept because students can use it to analyse a large number of science and engineering contexts, for example, by describing changes of a system in terms of movement, temperature or chemical composition (DfE Citation2013, 10). Furthermore, the frequent occurrence of the energy concept connects biological topics across different grade levels, thus helping students to construct a more interconnected understanding of biology (see also organisation of expert knowledge: e.g. Bransford, Brown, and Cocking Citation2000, 9). Energy is also a core idea for the science and engineering subjects (e.g. DfE Citation2013, 3; NRC Citation2012) and is therefore considered as an important means to connect science contents across disciplinary boundaries (e.g. Nordine Citation2016, 35; NRC Citation2012; Opitz et al. Citation2016; Park and Liu Citation2016). In addition to school science instruction, the energy concept also significantly concerns students’ everyday lives, for example, when they speak about nutrition, sports, climate change or energy ‘consumption’ and ‘saving’ (Harms Citation2016). Unfortunately, different connotations for energy exist in everyday life (e.g. vitalistic conceptions such as ‘I have a lot of energy, I think I want to go running!’) and scientific concepts (i.e. energy as an abstract, conserved quantity), which can make it more difficult for students to learn about energy (e.g. Jin and Anderson Citation2012; Lancor Citation2015).
Despite the relevance of the energy concept for biology instruction, previous research has mostly focused on students’ energy understanding in physics contexts. To extend this research and to provide an empirical basis for the further development of energy instruction in biology, this study provides a systematic analysis of students’ conceptions about energy in biological contexts.
Literature review
Detailed summaries of research on students’ energy understanding have been provided in previous publications (e.g. Chen et al. Citation2014; Neumann et al. Citation2013; Nordine, Krajcik, and Fortus Citation2011). According to the focus of this study, this section reviews research that focuses specifically on the teaching and learning of energy in biological contexts (e.g. Boyes and Stanisstreet Citation1990; Jennison and Reiss Citation1991; Jin and Anderson Citation2012).
Even though energy is an abstract concept, students are likely to encounter several implicit references to energy before the concept’s explicit introduction in school (Lin and Hu Citation2003). It was hereby found that early energy instruction can have sustainable learning effects and thus help students to better understand science contexts even many years after the instruction (Novak Citation2005). More so, early energy learning opportunities concern numerous biological contexts and students use these learning opportunities to develop first stages of their energy understanding (Opitz et al. Citation2015).
Alternative conceptions about energy can derive from both formal learning opportunities and everyday contexts and they can persist throughout later instruction, as demonstrated, for example, by Boyes and Stanisstreet’s (Citation1990) analysis of students’ conceptions about energy sources for animals and plants. Burger (Citation2001) extended these findings by identifying central student conceptions of energy in life processes, for example, that energy is created by animals; that the animals take up heat as a source of energy; or that energy cycles in systems. While the author found specific student conceptions to change across grade levels, the students’ overall conceptual understanding of energy underwent only minor changes across from lower to upper secondary school. In learning about energy in biology, students have been found to apply hybrid (instead of single) frameworks of understanding energy (Nordine, Krajcik, and Fortus Citation2011). Similarly, students’ energy understanding hereby seems to differ between specific learning contexts of a disciplinary area (e.g. when speaking about energy in photosynthesis vs. energy in their nutrition; Lancor Citation2015; Neumann et al. Citation2013; Park and Liu Citation2016).
Jin and Anderson (Citation2012) added to earlier findings by presenting an energy learning progression for biological processes that describes how students’ energy understanding progresses along four hierarchical levels of complexity: (1) commonly, elementary students (grade 4) used everyday conceptions of energy, in which they traced cause and effect chains in force-dynamic accounts and saw energy as an enabler. (2) Across secondary school, students prominently applied force-dynamic accounts in which students blended the analysis of energy and matter and described energy as a cause for occurring processes. (3) At the third level, students identified organic materials as energy sources, while still disregarding thermal energy and energy dissipation. (4) Only few of the secondary students (grades 9–11) achieved the highest progression level at which the scientific energy concept was employed to constrain processes, trace energy separately from matter, apply energy conservation and recognise heat dissipation.
Overall, even advanced students have been found to disconnect energy understanding in biological processes from an understanding of energy in the inanimate world. For example, students may be able to recite the law of energy conservation, while failing to apply this law to biological contexts (Chabalengula, Sanders, and Mumba Citation2011). A related problem for students’ energy understanding in biology has been attributed to the distinction between energy flow and matter cycles (Lin and Hu Citation2003).
Energy instruction also needs to build on understanding that students construct in physics and chemistry, where students traditionally receive many energy learning opportunities. Researchers have therefore argued to better coordinate energy instruction across different science subjects. Here, four aspects of the energy concept have been identified as relevant for energy teaching and learning in all science contexts. Students’ understanding of these aspects has been found to progress in the following sequence: (1) energy forms/sources, (2) energy transfer/transformation, (3) energy degradation/dissipation and (4) energy conservation (e.g. Opitz et al. Citation2015; Neumann et al. Citation2013; Nordine Citation2016, 32; Park and Liu Citation2016). Different science disciplines and school science subjects emphasise these energy aspects to varying degrees (Chen et al. Citation2014, 2; Harms Citation2016). Biology classes, for example, may address energy conservation less explicitly, while emphasising energy transfer and transformation (Opitz et al. Citation2015, Citation2016). Similarly, biology classes often confront students with energy flow, which incorporates an understanding of energy transfer/transformation and energy degradation/dissipation. At this point, it should be noted that only few school books, science curricula or standards explicitly address all four energy aspects, but often provide rather general learning expectations towards the energy concept. The four energy aspects have hence been suggested as important component ideas that can guide teachers and researchers in developing learning materials for students.
Research questions
In order to help students connect their understanding of energy across different disciplinary contexts and to support teachers in developing energy instruction accordingly, research has to identify conceptions that students employ about energy in different science contexts, i.e. biology, chemistry and physics. To extend prior research, we focus on conceptions that students employ about energy in biological contexts. By presenting conceptions in regard to the four energy aspects presented above (e.g. Chen et al. Citation2014; Nordine Citation2016, 33), we aim to connect our findings to earlier studies and make them informative for researchers from different disciplinary backgrounds.
The following research questions guided our analysis:
(1) | What conceptions do students employ in biological contexts with respect to the four central aspects of the energy concept: energy forms/sources, transfer/transformation, degradation/dissipation and energy conservation? | ||||
(2) | How does students’ understanding of these aspects differ between different grade levels at secondary schools (grades 5–11)? |
Method
As part of a mixed methods approach, our article presents findings to deepen insights from prior quantitative studies (Opitz et al. Citation2015, Citation2016). To address our research questions, we used a qualitative, cross-sectional study with German grade 5–11 students. The resulting one-to-one interviews were explored using qualitative content analysis (Mayring Citation2014).
Development of an interview guideline
The conducted interviews were based on a more structured interview guideline that still allowed students to provide continuing thoughts and the interviewer to ask follow-up questions or inquire about students’ statements. With regard to the scope of our study, we chose more structured interviews due to three reasons: First, we aimed to facilitate the comparison of student conceptions across several grade levels by following a clear set of guiding questions that limited variation between the interviews. Second, we planned to compare the results from this qualitative study to findings from our earlier quantitative study. We therefore required a more structured guideline to ensure that the interviews addressed similar contents as the earlier questionnaire study. Third, earlier experience showed that younger students had obvious problems to provide longer explanations about the abstract energy concept. To help the students, we decided to base our interviews on a larger number of questions that students could address through relatively short answers.
The interview guideline confronted students with a similar set of questions in each of three simple contexts: a running child, a growing tree and a growing child. These particular contexts stemmed from an earlier study on students’ energy understanding in carbon-transforming processes (Jin and Anderson Citation2012) and were selected as students can regularly encounter these contexts both in schools and in their everyday lives, as well as from primary to upper secondary level. In each of the three contexts, the interviews focused on energy in two sections. The first section included more general questions about energy (e.g. about energy sources for a growing tree) and originated from the interview guideline by Jin and Anderson (Citation2012). The second section contained more detailed questions concerning the four central energy aspects mentioned above. With regard to the planned method comparison, we used open-ended adaptations of questions from representative items of the previous questionnaire study (Opitz et al. Citation2015).
All students were interviewed with the same interview guideline. However, in each section of the interview, we also used some more advanced questions (e.g. on energy in chemical bonds) that were skipped if students had difficulty answering them. The developed interview guideline was iteratively tested and refined in trial interviews with three grade 9 students. Exemplary questions are provided in Figure and interested readers can access the full interview guideline in online material 1.
Sample and data collection
Interviews were conducted with students at the beginning of grades 5 (n = 12), 7, 9 and 11 (n = 6 each) and lasted for approximately 60 min. Table comprises characteristics of the sub-samples from each grade level. While the sample size is sufficient to detect students’ conceptions employed at different grade levels, it is important to note that the study cannot make more general claims about students’ understanding or how this understanding progresses across grade levels. The results should be interpreted accordingly.
Table 1. Overview of the subsamples at each grade level.
The sample for grade 5 was twice as large as in the upper grades to take into account both the bandwidth of young students’ conceptions, as well as their tendency to be shy in talking about a difficult concept like energy. With respect to our goal to compare student conceptions from different grade levels, we tried to limit school effects by selecting students from only one primary and one secondary metropolitan school, both from a similar socio-economic background.
All but the grade 5 interviewees had taken part in an earlier questionnaire study (Opitz et al. Citation2016) three months before the interviews, thus allowing us to compare our participants’ scores on this questionnaire with the larger sample from the questionnaire study (see Table ). We selected participants that were motivated and not shy to answer our questions. Thus, our participants received slightly higher test scores than the larger sample in the questionnaire study. More importantly, however, the range of the interviewed students’ test scores indicates that the participating students represent a wide range of student abilities, thus supporting our goal of detecting a broad range of student conceptions.
The federal state where the interviews were conducted (Hamburg, northern Germany) employs learning standards that feature energy as a core idea for science/biology learning. As the respective curricula for Hamburg primary and secondary schools highlight the energy concept numerous times, we assume that the interviewed students had multiple learning opportunities for the energy concept across the investigated grade bands (see Opitz et al. Citation2016 for details).
Analysis
Categorising students’ conceptions. The interviews were analysed using qualitative content analysis (Mayring Citation2014) and MAXQDA 11 software. Based on verbatim transcriptions of the interviews, we first identified units of analysis (coding units, N = 2293), each representing a single conception expressed by a student (Mayring Citation2014, 51). As we used the four energy aspects from previous research as a framework (e.g. Neumann et al. Citation2013, see research questions), these aspects served as (deductively formed) superordinate categories for our category system and guided the further analysis.
Students’ replies from the interviews were then used to inductively construct categories of students’ understanding for each of these four energy aspects (Berg Citation2001, 246). The categories were formulated, revised and ordered hierarchically using positive (‘Apply if …’) and negative (‘Do not apply if …’) coding rules, as well as anchor examples of student conceptions (Mayring Citation2014, 37).
Reliability of the category system. A newly developed category system can be considered ‘saturated’ when coding of further interview material does not lead to the identification of new categories (Berg Citation2001, 252; Mayring Citation2014, 81). In our study, this stage was reached after the analysis of roughly a third of our sample. However, we additionally categorised the remaining interview material from our sample to ensure the robustness of the developed category system and thus make it easier to compare student conceptions from different grade levels.
The initial category system was iteratively refined on the basis of three consecutive expert ratings (Mayring Citation2014, 114), each conducted with different researchers. Due to the four energy aspects’ overlap in meaning, the rating was conducted for each energy aspect separately. Based on a random selection of 32% of all coding units, the third inter-rating on the final category system indicated a high internal reliability with substantial to excellent inter-coder agreement (Fleiss et al. Citation2004): Fleiss’ kforms/sources = 0.89, ktransfer/transformation = 0.76, kdegradation/dissipation = 0.76, kconservation = 0.89.
Selection of categories. Students demonstrated a large number of different conceptions. While the resulting category system represents a necessary step to address our research questions, it is complex and we specifically present it only as a supplemental online resource (see online material 1). For this article, we present a selection of the identified categories as examples for students’ understanding of each of the four energy aspects. This selection aimed to reduce specificity while maintaining the range of student conceptions. Categories were selected if they were meaningful in regard to the research questions and if they were based on multiple student utterances and thus ensured a robust foundation of the category.
Results
Energy forms and sources
Previous studies have presented student conceptions about energy sources (e.g. Boyes and Stanisstreet Citation1990). For example, it was found that even university students think that animals not only use food as an energy source, but also water; or that the main energy source for plants would not only be sunlight, but also soil, water or air. While we also interviewed students about energy sources (e.g. for human or plant growth), these conceptions were similar to earlier findings. To access these findings, we thus direct interested readers to online material 2 and focus this section on students’ statements about different forms of energy.
Figure (a) presents student conceptions regarding manifestations of potential energy. Alternative names for chemical energy, such as ‘growth energy’ or ‘food energy’ were widely used across the grade levels and their appearance only decreased among 11th graders. In the participating schools at Hamburg, students received formal chemistry education from grade 7 onwards. Likewise, the term ‘chemical energy’ was not yet employed by 5th graders, but its application increased across grades (grade 11: 6/6 students). In this regard, few younger students, but half of the 11th graders associated air/oxygen with ‘chemical energy’.
Figure 2. Student conceptions concerning manifestations of potential energy (Figure (a)) and kinetic energy (Figure (b)).
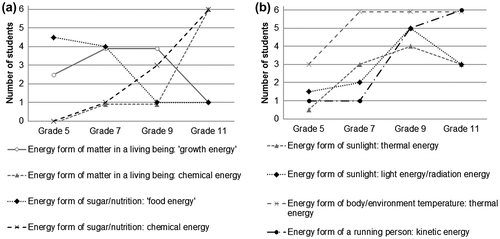
Even young students were able to identify some manifestations of kinetic energy (e.g. thermal energy) correctly (Figure (b)), but student statements about the kinetic energy of a running person or light/radiation energy of sunlight were only more widely observed from grade 7 onwards. In the latter case, roughly half the 7–11th graders also mentioned thermal energy when they described sunlight. Across all grade levels, few students employed anthropocentric views that directly equated energy with power or attributed the energy behind human movement directly to concepts like ‘sportsmanship’.
Energy transfer and transformation
The findings in Figure (a) indicate that older students more often used an acceptable view that describes energy transfer as a dislocation of the same energy form (grade 5: 1/6 students, grade 11: 5/6 students). However, alternative or abbreviated conceptions on energy transfer were sometimes additionally employed by roughly half the students (e.g. ‘energy transfer means moving an object to another location’). The previously described (e.g. Burger Citation2001) inaccurate conception of energy as a cycling or recycled substance was only mentioned by 3 students.
Figure 3. Student conceptions concerning energy transfer (Figure (a)) and energy transformation (Figure (b)).
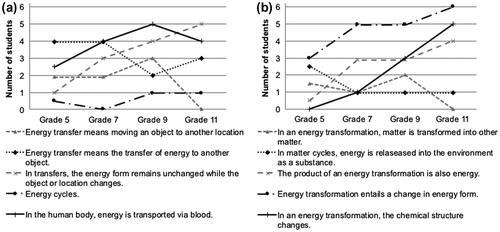
Figure (b) presents students’ conceptions regarding energy transformations. Younger students still seemed to have some difficultly with energy transformations, as their statements often disregarded that the product of energy transformations is still energy. While most participants successfully provided explanations that distinguished between energy transfer and transformation, many seemed to face difficulties in providing details when they traced energy through an organism. As in the following example from KL (grade 9, pseudonyms used here and below), students referred to the transport of energy via blood, while actually focusing on substances like vitamins or nutrients. Hereby, even older student only rarely mentioned energy transformations or how exactly energy can be transported by the blood (e.g. in what molecules the energy is stored):
A child uses energy from her food to run. What do you think how this energy becomes the kinetic energy of the running child?
The body extracts specific substances it needs from the food. The rest is simply digested. I would say that they [the required substances] then get into the blood. And the blood flows through the entire body. This is also where the oxygen and other things are. And when they [meaning unclear, possibly oxygen, substances or energy] are used up, they leave the bloodstream again.
Ok. So the child uses different energy sources in order to be able to run. Can you tell where, or in what substances, one could rediscover this energy after it has been used by the child?
Maybe when you breath, heat gets lost. Heat is exhaled.
Where does this heat go?
Simply into the air.
And what happens there?
It’s cooling down.
Across grades, students increasingly combined their descriptions of energy transformations with changes in chemical bonds. However, these explanations were frequently built on an abbreviated conception, for example, by considering only the breaking of bonds to be responsible for the release of energy. Student CP (grade 9), for example, already used this common shorthand view on energy in chemical bonds in his analysis of a digestion process. However, CP did not (yet) include a conceptualisation of bond energy, in which energy is released when reaction products are chemically more tightly bound and hence possess less bond energy than the reaction educts.
[CP had earlier referred to energy in chemical bonds of nutrients.] Can you imagine what has to happen to these bonds to make the energy accessible?
In this sense, they [the bonds] have to be broken down. So … you can always break up things and I think that they are broken down in the body … in the intestines, because acid is added there. The acid breaks down the bonds and they [the constituents] are then taken up.
Energy degradation and dissipation
Figure (a) describes student conceptions connected to energy degradation. At all grade levels, roughly half of the participating students equated energy degradation with energy loss or consumption. A conceptualisation of degradation on the basis of energy transformations or the release of energy into the environment was only more prominently described by grade 11 students. However, more mature students also increasingly used limited alternative views, for example, the conception that energy degradation is caused by releasing energy-rich matter into the environment.
Figure 4. Student conceptions connected to the conceptualisation of energy degradation (Figure (a)) and the irreversibility of energy degradation (Figure (b)).
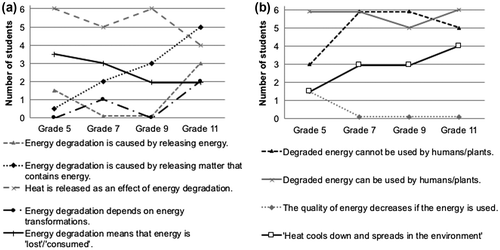
Despite their difficulties with energy degradation, students at all grade levels described the ‘release of heat’ as an effect of degradation. Here, younger students often expressed uncertainty about the causes for the release of heat, or they provided unspecific explanations (e.g. ‘activity’). In contrast, most of the older students correctly referred to energy transformation processes as the cause (see online material 2).
While older students increasingly and correctly addressed energy dissipation by the release and spread of thermal energy into the environment (Figure (b)), only one student explicitly stated that thermal energy had the lowest value for an organism because it wouldn’t be able to use this energy for further transformations. Similarly, only a single student mentioned the important idea that energy degradation increases the temperature and thereby allows chemical reactions to occur faster (see online material 2).
Across grades, few changes in students’ conceptions were observable regarding irreversibility as a central principle of energy degradation (Figure (b)). Almost all students hereby expressed the at least partly opposing conceptions that degraded energy could/could not be used anymore for further energy transformations. For example, student LK (grade 9) first provided a rather detailed explanation of how degraded energy cannot be transformed further (example 1). Later on, LK was one of roughly half the interviewees who correctly explained that degraded energy helps to maintain body temperature and is hence important for animals (see online material 2). However, LK also suggested that degraded energy would also more generally allow body processes to function (example 2):
Example 1:
Can you imagine what happens when energy is ‘degraded’?
The energy can then no longer be used, the value is removed and it [the energy] isn’t worth anything anymore.
Ok. What exactly does that mean if it [the energy] isn’t ‘worth anything anymore’?
You couldn’t use it to [hesitates] ... the body couldn’t use it again, similar to carbon dioxide that has been used up: Before, it was oxygen, and afterwards it can no longer be used.
Example 2:
[The interview here focusses on energy transfer and the student mentioned that heat is transferred from a moving muscle].
Ok, so what happens when this heat is transferred?
The body takes it [the heat] up and transforms it.
And into what form does the body transform this energy?
That’s thermal energy [note: at this point, the student likely confuses energy transfer and transformation]. I think it [the body] can generally transform this energy so that the body has a certain temperature and so that it can function.
Energy conservation
Similar to students’ understanding of energy degradation, the conceptions concerning energy conservation (Figure (a)) were also partly opposing each other: almost all students mentioned in at least one of the three contexts that energy cannot be consumed or created. However, when they described energy in one of the other two contexts, all of the younger students and two-third of the older students also stated the opposite, i.e. that energy can be created, consumed or ‘lost’ (see online material 2). As can be seen, for example, in KG’s explanation, half the students in grade 5 explicitly stated that energy loss causes the energy to stop existing:
Figure 5. Student conceptions regarding energy conservation (Figure (a)) and the analysis of energy within system boundaries (Figure (b)).
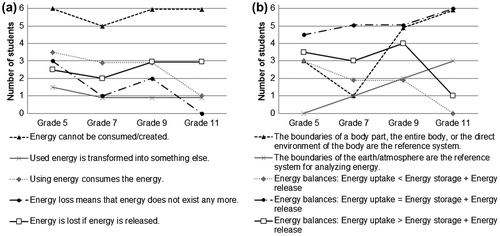
[The student had earlier mentioned that energy is ‘lost’ when a child grows. The interviewer enquired accordingly]. What do you imagine happens when energy is ‘lost’?
For example, let’s look at teeth: We grow milk teeth and afterwards, we grow the real, adult teeth. And if one of the milk teeth is falling out, energy would get lost there.
Is that because the energy in the tooth is no longer useful to the body?
Yes.
Ok, but is this energy–after the tooth fell out–still in the tooth or is it gone?
I don’t think it’s there anymore.
Alternative conceptions of consumption/loss (e.g. the conception that energy consumption refers to the transformation of energy into another entity) were only used by few interviewees. Even though so many students mentioned conceptions of energy ‘loss’, only two elaborated their statements in a way that suggests they meant energy degradation, but only used inaccurate language in their explanation (see online material 2). However, for several students in our study, conceptions of energy loss were clearly related to their use of vitalistic energy conceptions (e.g. the feeling of being physiologically or psychologically exhausted; see also Jin and Anderson Citation2012) instead of the scientific energy concept. If students employ such alternative energy connotations that include notions of energy ‘loss’, it is likely harder for them to acknowledge conservation of energy in a science phenomenon. Student KI (grade 5) provides an example:
[The student had mentioned that energy can get lost while a child is running].
What do you imagine happens when energy ‘gets lost’?
You ... when you run, you’re soon exhausted because you have used too much power.
Ok. And that means the energy is simply gone then?
Suddenly, it [the energy] is gone. Then you have to stop, catch your breath, and then, somehow, you automatically have energy again after you have stopped and calmed down for a moment.
Considerations of energy conservation within non-idealised biological contexts rely on the definition of system boundaries. Our findings on the respective student conceptions did not indicate that older students necessarily used a scientifically more accurate view than younger students (Figure (b)). While older learners seemed to generally consider system boundaries more often, these boundaries were mostly limited to organism parts, the entire organism, or the organism’s direct environment. However, the older students only rarely broadened their perspective to include the atmosphere into their reference system and they did not mention energy transfers between the global system and the universe.
At all grade levels, the majority of students were able to correctly describe an energy balance in one of the three contexts. As, however, roughly half the students in grades 5, 7 and 9 also suggested an inaccurate energy balance in one of the other contexts, it appears that only the grade 11 students were able to describe energy balances with more certainty.
Discussion
Research Question 1: (What conceptions do students employ in biological contexts with respect to four central aspects of the energy concept?)
Similar to earlier studies (e.g. Lancor Citation2015; Neumann et al. Citation2013; Park and Liu Citation2016), students from all grade levels had more difficulties explaining about the aspects of energy degradation/dissipation and energy conservation, while an understanding of energy forms/sources and transfer/transformation appeared as more accessible for the students (see also Jin and Anderson Citation2012). Out interviewees furthermore seemed to make few connections between their understanding of individual energy aspects. For example, when students traced energy through the human body, they rarely used conceptions of energy flow in the sense of a combination of the analysis of energy transfer, degradation or conservation.
Our results provide several examples of varying, sometimes incoherent (Lakoff and Johnson Citation1980, 44) conceptions that characterise students’ understanding of individual energy aspects. Obvious examples can be found in conceptions connected to the irreversibility of energy degradation or conceptions connected to the consumption/loss of energy (see results). Earlier studies indicated that students’ energy understanding differs substantially with specific contexts (e.g. Lancor Citation2015; Neumann et al. Citation2013; Park and Liu Citation2016). The three different contexts employed by this study may hence be a potential explanation for the observed incoherence in students’ conceptions.
We interviewed students about their understanding of the energy concept in typical biological contexts. However, relatively few of the students’ most prominently employed conceptions appeared to be clearly connected to a biology-specific understanding. Instead, most of the student explanations were based on a discipline-general understanding of energy. As this observation is hardly quantifiable with the available data, it should be verified by future interview studies that compare students’ conceptions across different disciplinary contexts. However, as students’ energy concepts in biology, chemistry and physics contexts have previously been found to be strongly interrelated, a less biology-specific understanding would be in line with previous research (Lancor Citation2015; Opitz et al. Citation2016; Park and Liu Citation2016).
Research Question 2: How does students’ understanding of these aspects differ between different grade levels at secondary schools (grades 5–11)?
Due to the size of our sample and the cross-sectional design of our study, the presented findings should not be interpreted as reliable progression trends in understanding across grade levels (cf. Jin and Anderson Citation2012; Neumann et al. Citation2013). Instead, the findings are used to reveal increasingly employed acceptable conceptions and decreasingly used inaccurate alternative conceptions. Differences in students’ explanations between different grade levels were especially observable for the energy aspects forms/sources and transfer/transformations (see Figures (a), (b) and 3(a), (b)), but less so for energy degradation/dissipation and conservation. Here, conceptions that were more prominently found among older students often related only to sub-aspects of the accepted scientific view (see Figures (a), (b) and 3(a), (b)).
Besides the observed differences in student conceptions between different grade levels, our study also identified alternative conceptions that were used by students from all age groups to similar extents. Such alternative conceptions are often connected to an everyday understanding of energy (Lancor Citation2015) and encompass, for example, the conception that energy degradation equals energy loss (Figure (a)) or that energy is consumed when used (Figure (a)). A further hurdle for the development towards a more sophisticated energy understanding can be seen in students’ dependence on their understanding of related concepts like heat or force (see Figures (a), (b), compare online material 2, section energy degradation/dissipation; see also Megalakaki and Thibaut Citation2016).
Students’ difficulties with the energy concept have frequently been connected to their imprecise application of everyday vs. scientific connotations of the energy concept (e.g. Lancor Citation2015; Jin and Anderson Citation2012). While it is certainly difficult for learners to use adequate ‘language’ for the energy concept (i.e. differentiate between scientific and everyday connotations), our findings reveal only few examples where older students appeared to provide more adequate answers based only on more elaborate or precise wording (e.g. students’ explanations of ‘energy loss’, see online material 2). Instead, the older students also used different and often more acceptable conceptions than younger students. Thus, our results indicate that the progression trends observed by prior quantitative studies (e.g. Jin and Anderson Citation2012; Opitz et al. Citation2015) do not only reflect an increasingly more sophisticated use of language among older students, but also that the students’ understanding changes across grades as students develop new conceptions and abandon prior ones.
Study limitations
The limitations of the sample size were discussed in the methods and results section. The comparatively high level of structure in the employed interview guideline limited the freedom of student replies. While this approach was necessary with regard to our study’s goals (see methods), richly qualitative studies could add to our findings by analysing more open interviews.
Our study only focused on basic levels of understanding energy degradation and dissipation. While entropy is a concept that clearly underlies energy degradation and dissipation, our study cannot provide more detailed insights into students’ understanding of this additional concept.
Finally, we are unfortunately unable to determine which students held back conception due to being shy or unsure about their understanding. Based on previous research experiences, we assumed that young students would be more likely to do so. Possibly, this is why some examples in our findings suggest that younger students might employ more elaborate conceptions than older students (e.g. about systems boundaries, Figure (b) and online material 2). While we hoped to partly address this issue by using a larger sample in grade 5, some limitations likely remain.
Implications for research and instruction
This study provides one of the first systematic analyses of conceptions that students use to describe energy in biological contexts. Students’ conceptions were presented in regard to four relevant energy aspects, thus making the findings also more meaningful for researchers and educators from different science backgrounds (e.g. Lancor Citation2015; Neumann et al. Citation2013; Nordine Citation2016, 33; Opitz et al. Citation2016). Based on the four energy aspects, we derive implications for the further development of energy instruction in biology:
Energy forms/sources: By grade 9, the majority of students correctly identified several energy forms. This intermediate understanding of energy may thus serve students as a scaffold for later energy learning (Jin and Anderson Citation2012). However, the students had difficulty to explain the nature of chemical energy, for example regarding the release of energy from chemical bonds or the role of oxygen in life processes. Student could therefore likely profit from more explicit instruction on these facets of chemical energy.
Energy transfer/transformation: Even students in the lower grade levels often gave correct scientific explanations about the meaning of energy transfer/transformation (Opitz et al. Citation2015). However, their conceptions about this aspect were still developing, as students rarely focused on energy flow. Biology instruction could profit from a stronger focus on this perspective to better connect students’ understanding of energy transfer/transformation, degradation/dissipation and conservation.
Energy degradation/dissipation: Our findings suggest that even older students had difficulties with this energy aspect, as the interviewees used a mixed set of correct and incorrect conceptions, for example, by employing potentially opposing views on the irreversibility and value of degraded energy. As students’ difficulties were also related to alternative conceptions about energy conservation, biology classes could support learners by addressing the meaning of energy degradation and by focusing on the respective processes in an organism that release thermal energy.
Energy conservation: Similar to energy degradation/dissipation, students’ conceptions about energy conservation were characterised by both scientifically acceptable and everyday explanations, thereby highlighting how students pick up conceptions about energy both from everyday and formal learning opportunities. Students could hence benefit from reflecting on language use in their analysis of energy (e.g. by discussing the meaning and adequacy of formulations like ‘energy loss’). The results furthermore highlight students’ incomplete considerations of system boundaries and suggest that instruction needs to foster their ability to distinguish between (idealised) isolated, closed and open systems.
Future studies could extend our research by analysing how instruction can help students to integrate their understanding between the different energy aspects (Nordine, Krajcik, and Fortus Citation2011). Analogously, research should identify ways to strengthen the coherence of energy teaching across disciplines (Lin and Hu Citation2003) in order to help students combine, for example, macro- and micro-level perspectives from biology and chemistry.
Funding
This work was supported by the Leibniz Institute for Science and Mathematics Education and the Hamburger Schulversuch.
Supplemental data
Supplemental data for this article can be accessed at http://dx.doi.org/10.1080/00219266.2016.1257504
1257504_supp_files.zip
Download Zip (187 KB)Acknowledgement
We would like to thank the city of Hamburg for funding the komdif research project and allowing easy access to the field. The support from all teachers and students made this research project a very enjoyable one. We are especially thankful to Silke Gatermann for her help in organising the study. For his extended support in data entry and interview analysis, we want to acknowledge Marc Rodemer.
References
- Berg, B. L. 2001. Qualitative Research Methods for the Social Sciences. 4th ed. Needham Heights, MA: Pearson Education Company.
- Boyes, E., and M. Stanisstreet. 1990. “Pupils’ Ideas Concerning Energy Sources.” International Journal of Science Education 12 (5): 513–529.10.1080/0950069900120505
- Bransford, J. D., A. L. Brown, and R. R. Cocking. 2000. How People Learn: Brain, Mind, Experience and School. Washington, DC: National Academy Press.
- Burger, J. 2001. Schülervorstellungen Zu Energie Im Biologischen Kontext - Ermittlungen, Analysen Und Schlussfolgerungen [Student Conceptions concerning Energy in Biological Contexts – Research, Analysis and Conclusions]. Bielefeld: University of Bielefeld, Dissertation.
- Chabalengula, V., M. Sanders, and F. Mumba. 2011. “Diagnosing Students’ Understanding of Energy and its Related Concepts in Biological Contexts.” International Journal of Science and Mathematics Education 10 (2): 241–266.
- Chen, B., A. Eisenkraft, D. Fortus, J. S. Krajcik, K. Neumann, J. Nordine, and A. Scheff. 2014. Teaching and Learning of Energy in K-12 Education. New York: Springer.10.1007/978-3-319-05017-1
- (DfE) Department for Education. 2013. Science Programmes of Study: Key Stage 3 – National Curriculum in England. https://www.gov.uk/government/uploads/system/uploads/attachment_data/file/335174/SECONDARY_national_curriculum_-_Science_220714.pdf
- Fleiss, J. L., B. Levin, and M. C. Paik. 2004. “The Measurement of Interrater Agreement.” Chap. 18 in Statistical Methods for Rates and Proportions, 3rd ed. edited by J. L. Fleiss, B. Levin, and M. C. Paik, 598–626. Hoboken, NJ: Wiley.
- Harms, U. 2016. “Ohne Energie Gehts Nichts! [Nothing Works without Energy!]” Unterricht Biologie 411 (40): 2–11.
- Jennison, M., and M. J. Reiss. 1991. “Does Anyone Know What Energy is?” Journal of Biological Education 25 (9): 173–176.10.1080/00219266.1991.9655202
- Jin, H., and C. W. Anderson. 2012. “A Learning Progression for Energy in Socio-Ecological Systems.” Journal of Research in Science Teaching 49 (9): 1149–1180.10.1002/tea.v49.9
- Lakoff, G., and M. Johnson. 1980. Metaphors We Live by. Chicago: The University of Chicago.
- Lancor, R. 2015. “An Analysis of Metaphors Used by Students to Describe Energy in an Interdisciplinary General Science Course.” International Journal of Science Education: 1-27. doi:10.1080/09500693.2015.1025309.
- Lin, C.-Y., and R. Hu. 2003. “Students’ Understanding of Energy Flow and Matter Cycling in the Context of the Food Chain, Photosynthesis, and Respiration.” International Journal of Science Education 25 (12): 1529–1544.10.1080/0950069032000052045
- Mayring, P. 2014. Qualitative Content Analysis Theoretical Foundation, Basic Procedures and Software Solution. Klagenfurt: SSOAR.
- Megalakaki, O., and Thibaut, J. 2016. “Development and Differentiation of Force and Energy Concepts for Animate and Inanimate Objects in Children and Adolescents.” Research in Science Education 46 (4): 457–480. doi:10.1007/s11165-015-9467-9
- Neumann, K., T. Viering, W. J. Boone, and H. E. Fischer. 2013. “Towards a Learning Progression of Energy.” Journal of Research in Science Teaching 50 (2): 162–188.10.1002/tea.v50.2
- Nordine, J. 2016. Teaching Energy across the Sciences, K-12. Arlington, VA: National Science Teachers Association (NSTA).
- Nordine, J., J. Krajcik, and D. Fortus. 2011. “Transforming Energy Instruction in Middle School to Support Integrated Understanding and Future Learning.” Science Education 95 (4): 670–699.10.1002/sce.20423
- Novak, J. D. 2005. “Results and Implications of a 12-year Longitudinal Study of Science Concept Learning.” Research in Science Education 35 (1): 23–40.10.1007/s11165-004-3431-4
- (NRC) National Research Council. 2012. A Framework for K-12 Science Education: Practices, Crosscutting Concepts, and Core Ideas. Committee on a Conceptual Framework for New K-12 Science Education Standards. Board on Science Education, Division of Behavioral and Social Sciences and Education. Washington, DC: The National Academies Press.
- Opitz, S., U. Harms, K. Neumann, K. Kowalzik, and A. Frank. 2015. “Students’ Energy Concepts at the Transition between Primary and Secondary School.” Research in Science Education 45 (5): 691–715. doi:10.1007/s11165-014-9444-8.
- Opitz, S., Neumann, K., Bernholt, and U. Harms (2016). “How Do Students Understand Energy in Biology, Chemistry, and Physics? Development and Validation of an Assessment Instrument.” Chap. 3 in Students’ Progressing Understanding of the Energy Concept: An Analysis of Learning in Biological and Cross-Disciplinary Contexts, Doctoral Dissertation, 66–92. Germany: University of Kiel. http://macau.uni-kiel.de/receive/dissertation_diss_00019005.
- Park, M., and X. Liu. 2016. “Assessing Understanding of the Energy Concept in Different Science Disciplines.” Science Education 100 (3): 483–516. doi:10.1002/sce.21211.
- Reece, J. B., L. A. Urry, M. L. Cain, S. A. Wasserman, P. Minorsky, R. B. Jackson, and N. A. Campbell. 2011. Campbell Biology. Boston, MA: Benjamin Cummings/Pearson.