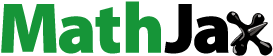
ABSTRACT
Neutron nuclear data of Xe isotopes have been evaluated in the energy region, including the resolved resonance one, from 1 keV to 20 MeV by using the theoretical nuclear reaction models. The phenomenological optical model potential was employed to calculate the total cross section for natural Xe with the coupled-channels method. The cross sections for channels of capture, (n, 2n), (n, p) and (n, α) reactions were calculated and compared with available experimental results including recently measured data. The elastic scattering angular distributions and particle emission spectra were calculated, although there is no experimental information available. Reaction cross sections of evaluated libraries were considered for comparison with the calculated results. The presently calculated cross sections reproduce better the available experimental data.
1. Introduction
Natural element of xenon with atomic number Z = 54 is composed of nine stable isotopes whose number is the second largest one, following tin. The stable isotopes of natural Xe with mass numbers A = 129, 131, 132, 134 and 136 are significant fission products. In fission reactors, cumulative yields of 134Xe, for instance, at thermal and fast neutron energies are 7.87 and 7.66% [Citation1], respectively, for 235U. This notable yields have heightened urgency of data evaluation.
The neutron nuclear data evaluation of 131, 132, 133, 134, 135, 136Xe was carried out first time in 1984 for JENDL-2 [Citation2]. In this evaluation, the optical and statistical model code CASTHY [Citation3] was utilized to calculate total, elastic and inelastic scattering cross sections. In 1990, the data of remaining isotopes of 124, 126, 128, 129, 130Xe were evaluated and incorporated in JENDL-3.1 [Citation4], where the pre-equilibrium and multi-step evaporation model code PEGASUS [Citation5] was used for competing reactions. These data were eventually compiled into the subsequent revisions of JENDL-3.2 [Citation6] and JENDL-3.3 [Citation7]. These data were re-evaluated in the latest release of JENDL-4.0 [Citation8], where total, elastic, inelastic, capture and particle emissions, as well as other competing reaction cross sections were calculated by nuclear reaction model codes POD [Citation9] and OPTMAN [Citation10]. Obviously, the evaluated data in JENDL-4.0 are expected to be better than those of previous evaluations due to the use of advanced nuclear reaction models. Nevertheless, JENDL-4.0 still has inconsistency with measured data for some reactions, and the experimental data after the release were not taken into account.
Integral test on activation cross sections of Xe isotopes was carried out in the liquid metal cooled fast reactors JOYO and YAYOI [Citation11] to identify capsule rupture. These experiments were performed in the fast neutron energy region. There was discrepancy in data between the experiment in YAYOI and calculation with JENDL-3.2. The comparison revealed that the evaluated capture cross sections of 124, 126Xe may be large in the keV energy region.
The work on large-scale searches for neutrinoless double-β decay experiments is currently operating in which 136Xe is being considered as one of the most promising candidates. One of the experiments is named as “Kamioka Liquid Scintillator Antineutrino Detector Zero Electron Neutrino” (KamLAND-Zen) [Citation12] at Kamioka mine in Japan and another one is called “Enriched Xenon Observatory” (EXO) [Citation13] in USA. Although these experiments are being carried out in underground, neutron-induced background may have a severely influence on the detection events. Therefore, the relevant cross-section data such as capture and (n, 2n) reactions are required with a reliable accuracy.
Several neutron nuclear data evaluations [Citation14–17] have been performed since the inception of nuclear reaction model code CCONE [Citation18]. Those comprehensive works have given better prediction than those of previous results. It is noticed that the data evaluation of Xe isotopes is yet to be performed by the CCONE code. The aim of this work is to get further improvements in nuclear data of xenon by using the CCONE code.
In this paper, we performed evaluation of neutron nuclear data for Xe isotopes in which relevant nuclear reaction models were adopted to calculate cross sections for various reaction channels. Section 2 explains how the evaluation is performed by using nuclear reaction models with input parameters. The calculation results are compared with the experimental data and preceding evaluated ones in Section 3. Finally, the conclusion is drawn in Section 4.
2. Theoretical nuclear models and evaluation procedures
It is necessary to use several nuclear reaction models, in order to calculate cross-sections of different reaction channels and particle emission spectra induced by neutrons up to 20 MeV. The coupled-channels method [Citation19] is employed for the calculation of total, shape elastic and direct inelastic scattering cross sections. Outgoing channels are delineated by other nuclear reaction models. The compound and pre-equilibrium processes are characterized by the statistical model, namely Hauser–Feshbach theory [Citation20] and two-component exciton model [Citation21], respectively. Moreover, the distorted wave Born approximation is employed to the direct reaction process for vibrational levels. The present calculation is performed by the theoretical nuclear reaction model code, CCONE, where all of these models are included.
2.1. Coupled-channels optical model
The coupled-channels optical model is based on the rigid rotator model [Citation22] which is included in the CCOM code [Citation23]. The CCOM code is used for Xe isotopes as a target and neutron as projectile in the incident channel. The code was utilized to evaluate the total cross section as well as to calculate the neutron transmission coefficients which are applied to statistical model calculation. The functional form of the optical model potential (OMP) is adopted from Kunieda et al. [Citation24]. The real volume VR and imaginary surface WD terms of OMPs are given by
(1)
(1)
(2)
(2) where Z, N and A are the number of protons, neutrons and nucleons in the target nucleus, respectively. The imaginary volume and the real and imaginary spin–orbit terms are included in the same functional forms of Kunieda et al. The quantity E† is the projectile energy relative to the Fermi one. Symbols V(0 − 3)R,
,
, WIDD, Cviso, Cwiso, λR, D, nuclear radius rR, D, V, SO and diffuseness aR, D, V, SO are the OMP parameters. The values of these parameters were taken from global ones of Kunieda et al. Some values were, however, modified from the original ones for better agreement with the experimental data. The modified values are given in , together with the original ones. The coupling schemes used in the coupled-channels calculation along with the deformation parameters β2 are listed in . The deformation parameters were originally taken from Raman et al. [Citation25], although their values were adjusted so as to reproduce experimental total cross sections of natural Xe.
Table 1. Values of OMP parameters.
Table 2. Coupled levels and deformation parameters.
It is worth to be mentioned that the modified Kunieda potential is used only for neutron incident and corresponding decay channels. On the other hand, emission channel OMPs from nuclides other than targets are taken from Koning and Delaroche [Citation26] for neutron and proton, Lohr and Haeberli [Citation27] for deuteron, Becchetti and Greenlees [Citation28] for triton and He-3, and Avrigeanu and Avrigeanu [Citation29] for α-particle.
The calculated total neutron cross sections for natural Xe are shown in . In this figure, the obtained result is compared with the experimental data of Vaughn et al. [Citation30] and the evaluated data of JENDL-4.0 [Citation8], JENDL-3.3 [Citation7] and ENDF/B-VII.1 [Citation31]. In addition, other OMPs, e.g., Kunieda et al. and Koning and Delaroche, are also used to calculate the total cross sections for comparison. It is observed that the present results and JENDL-4.0 show better agreement with the experimental data than the cross sections calculated with other OMPs, particularly, at the energy region below 2 MeV.
2.2. Pre-equilibrium model
It is well established that an intermediate process existing between direct and compound ones is referred to as the pre-equilibrium process which takes place after the first stage of the reaction and before statistical equilibrium of the compound nucleus. In the present calculation, the contributions of the pre-equilibrium process to the reaction cross section have been taken into account by using the two-component exciton model [Citation21]. The global parametrization prescribed by Koning and Duijivestijn [Citation32] is incorporated in the calculation of cross section for pre-equilibrium components. In the framework, the single-particle state densities are described by introducing the parameters Cν for neutrons and Cπ for protons as in the following equations:
(3)
(3)
(4)
(4)
In order to fit the experimental data, these parameters were adjusted for Xe isotopes. The parameter values adopted are listed in . The default value of these parameters is unity.
Table 3. Pre-equilibrium parameters.
Furthermore, the particle pick-up and knock-out processes [Citation33] were also considered in this evaluation. The knock-out process was employed only for α-particle.
2.3. Statistical model
The compound nuclear reaction was calculated on the basis of Hauser–Feshbach formalism [Citation20] including width fluctuation correction. Neutrons, protons, deuterons, tritons, 3He, α-particle and γ-ray emission channels were included. It is noted that the neutron transmission coefficients for target Xe isotopes were imported from coupled-channels optical model calculation as mentioned in Section 2.1.
2.3.1. Discrete levels and level density
It is inevitable to provide input of the discrete levels and level density parameters for 47 nuclei, for instance, 122 − 137Xe, 122 − 136I and 120 − 135Te. The discrete-level information was taken from the Reference Input Parameters Library, RIPL-3 [Citation34].
The composite formula concerning nuclear level density introduced by Gilbert and Cameron [Citation35] was adopted. The level density in constant temperature model can be expressed as
(5)
(5) where T is the nuclear temperature, E0 is the energy shift and U is the corrected excitation energy obtained from excitation energy Ex and pairing energy Δ as U = Ex − Δ. The level density parameter a in Fermi-gas model has energy dependence, and is expressed by the following equation:
(6)
(6) where a* and γ are the asymptotic level density parameter and the shell correction damping factor, respectively. The shell correction energy Esh was obtained from the difference between experimental mass and theoretical smoothed mass. The theoretical mass was derived from the empirical formula of Myers and Swiatecki [Citation36] and the values of experimental mass were taken from the evaluated data of Audi et al. [Citation37]. The parameter a* was determined so as to get better agreement with average level spacing of s-wave neutron resonances which was derived from experiments. On the other hand, the systematic value for a* was adopted from the work of Mengoni and Nakajima [Citation38], if there were no experimental data available. The nuclear level density parameters used in this evaluation are tabulated in
for individual nuclei along with the energies of the adopted highest discrete levels Emax.
Table 4. Level density parameters for each isotope.
2.3.2. Gamma-ray strength function
The generalized Lorentzian form [Citation39] was used for γ-ray strength function fE1 of E1 radiation. The parameter values of the energy, width and peak cross section for resonances were taken from the systematics for spherical and deformed nuclei [Citation40] with deformation value. In addition, the strength functions for M1 and E2 radiations were also incorporated from the work of Kopecky and Uhl [Citation39]. It is to be mentioned that the calculated capture cross sections were adjusted to the experimental data by introducing a normalization factor fγ which was just multiplied to the total strength functions. In this evaluation, the values of fγ such as 1.43, 1.35, 2.15, 2.00, 1.15, 1.49, 0.58 and 1.55 were used for fitting to capture cross sections and Maxwellian-averaged ones of 124Xe, 126Xe, 128Xe, 129Xe, 130Xe, 132Xe, 134Xe and 136Xe, respectively. We found the fluctuation of fγ values, when the γ-ray strength function with systematic giant dipole resonance parameters were consistently used. It is not confirmed whether these fluctuations have physical meanings. The Maxwellian-averaged cross section was averaged over an energy region including resolved resonance one. Lack of resonance contribution may be responsible for the fluctuation of fγ values.
3. Evaluated results and discussion
The calculated neutron-induced reaction cross sections of 124 − 136Xe for various channels, e.g., capture, (n, 2n), (n, p) and (n, α) reactions, were compared with the experimental data. In these comparisons, experimental data were retrieved from EXFOR database [Citation41]. The present results are visualized with the currently available major evaluated data (JENDL-4.0, ENDF/B-VII.1 and JEFF-3.2 [Citation42]) as well as measured data.
3.1. Capture cross section
The cross section of 124Xe is compared with the recent experimental data measured by Bhike et al. [Citation43] and data of evaluated libraries in . The calculated cross section is in good agreement with the newly measured data. However, the cross sections of JENDL-4.0, ENDF/B-VII.1 and JEFF-3.2 are deviated from the measured data. The cross sections for 128, 129, 130Xe were investigated by Reifarth et al. [Citation44] as a function of neutron energy in the range from 3 to 225 keV by using time-of-flight method. The present cross sections are compared with those experimental results for the corresponding isotopes, and contrasted with major evaluated nuclear data as illustrated in –. In these figures, the calculated cross sections reproduce the experimental data satisfactory. Furthermore, JENDL-4.0 evaluation describes experimental data in this energy range well. However, the cross section of ENDF/B-VII.1 for 128Xe and 130Xe are slightly higher than experimental data except the energy below 35 keV for 128Xe, where evaluation explains the experimental data. In addition, the data of ENDF/B-VII.1 for 129Xe are estimated to be smaller cross section than experimental data.
Figure 2. Comparison of the present 124Xe(n,γ)125Xe reaction cross section with the evaluated and experimental data.
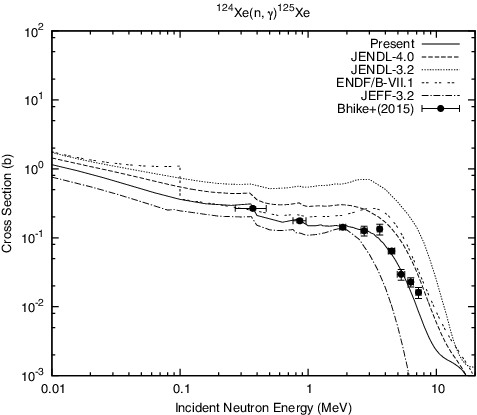
Figure 3. Comparison of the present 128Xe(n,γ)129Xe reaction cross section with the evaluated and experimental data.
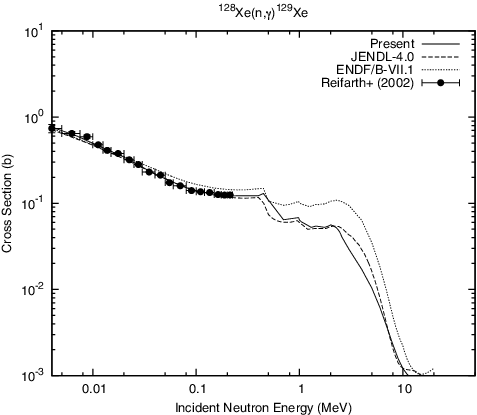
Figure 4. Comparison of the present 129Xe(n,γ)130Xe reaction cross section with the evaluated and experimental data.
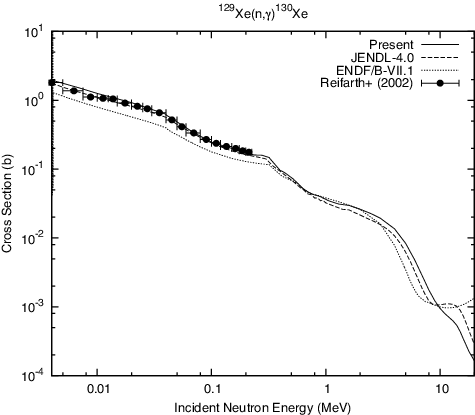
Figure 5. Comparison of the present 130Xe(n,γ)131Xe reaction cross section with the evaluated and experimental data.
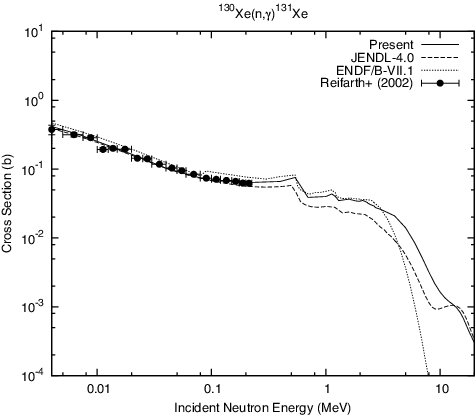
represents the comparison of calculated cross section of 136Xe with the evaluated libraries (JENDL-4.0 and ENDF/B-VII.1) and experimental data. Obviously, the present calculation expresses better the recent experimental result obtained by Bhike et al. [Citation45]. The experiment was performed by activation method in the incident neutron energy range of 0.4–14.8 MeV. In contrast, JENDL-4.0 and ENDF/B-VII.1 are unable to reproduce experimental results, while their cross sections agree with the results of Bhike et al. below 2 MeV. The cross sections for remaining Xe isotopes were calculated and depicted with the data of JENDL-3.2 for only 126Xe and JENDL-4.0 as shown in . The cross sections measured with Maxwellian neutron spectrum are only available for 126Xe, 132Xe and 134Xe. The Maxwellian-averaged cross sections calculated with resolved resonance parameters in JENDL-4.0 well reproduce those data.
Figure 6. Comparison of the present 136Xe(n,γ)137Xe reaction cross section with the evaluated and experimental data.
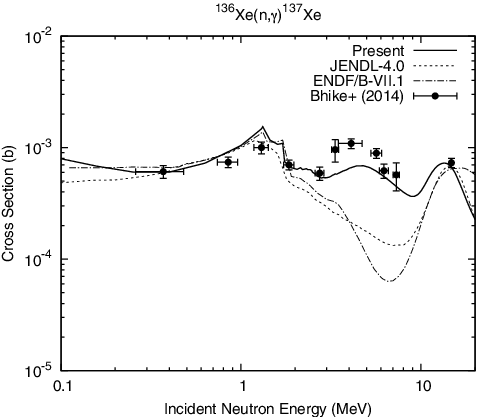
Figure 7. Comparison of the present capture cross sections of 126, 131, 132, 134Xe with the evaluated data.
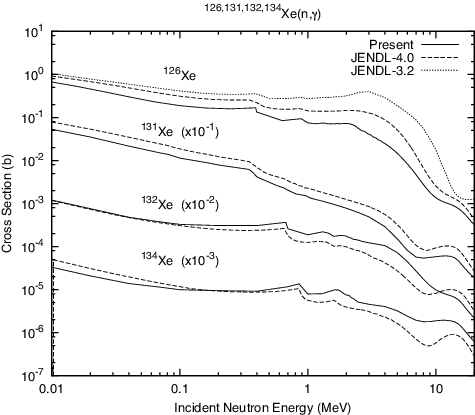
In integral test of YAYOI reactor experiment, the analysis was done by using JENDL-3.2 [Citation11]. A large discrepancy in C/E values of 2.2–2.6 and 2.1–2.3 was observed for the radioactivities of 125Xe and 127Xe, respectively, which were produced by the neutron capture reactions on 124Xe and 126Xe. It was considered that this result comes from their large capture cross sections. The present results for 124, 126Xe are smaller than the data of JENDL-3.2 by about 40% at 500 keV, at which the neutron spectrum of YAYOI has the largest peak, as seen in and . Thus, they may improve inconsistency in the C/E values at the experimental analysis of YAYOI.
3.2. (n, 2n) reaction cross section
The measurements of cross section for (n, 2n) reaction on 124Xe, 126Xe, 128Xe, 134Xe and 136Xe by activation method were done by Sigg and Kuroda [Citation46] and Kondaiah et al. [Citation47] at the incident neutron energies of 14.6 and 14.4 MeV, respectively. The calculated data are compared with those experimental data, evaluated libraries and other available experimental data as illustrated in –.
Figure 8. Comparison of the present 124Xe(n,2n)123Xe reaction cross section with the evaluated and experimental data.
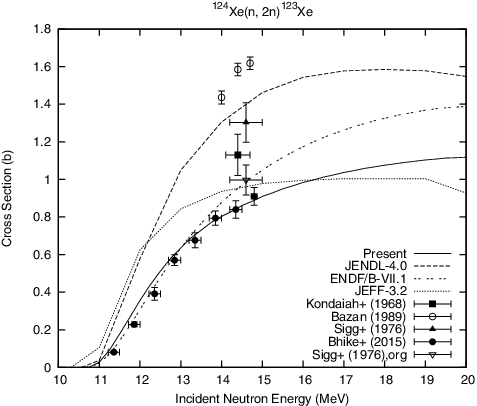
shows that the present result for 124Xe(n, 2n) reaction is in good agreement with the recent experimental data obtained by Bhike et al. [Citation43] and gives smaller cross sections than those of Bazan [Citation48] and the others. ENDF/B-VII.1 and JEFF-3.2 evaluations mostly agree with the original data of Sigg and Kuroda (open inverted triangle). In contrast, the data of JENDL-4.0 are much larger than those of Kondaiah et al. and Sigg and Kuroda, but marginally fitted to the data of Sigg and Kuroda (filled triangle) corrected by using up-to-date intensity Iγ = 48.9% for 148.9 keV gamma ray from the decay of 123Xe.
In –, the calculated reaction cross sections reproduce well the data of Sigg and Kuroda for 126Xe, 128Xe and 134Xe. For 126Xe(n, 2n) reaction, JEFF-3.2 data agree with those of Sigg and Kuroda, and JENDL-4.0 is marginally accepted, while ENDF/B-VII.1 evaluation fitted to the data of Kondaiah et al. as in .
Figure 9. Comparison of the present 126Xe(n,2n)125Xe reaction cross section with the evaluated and experimental data.
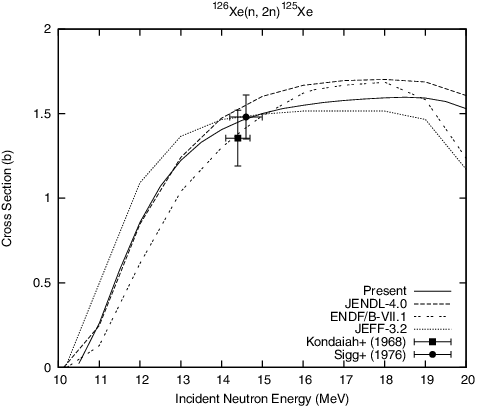
Figure 10. Comparison of the present 128Xe(n,2n)127Xe reaction cross section with the evaluated and experimental data.
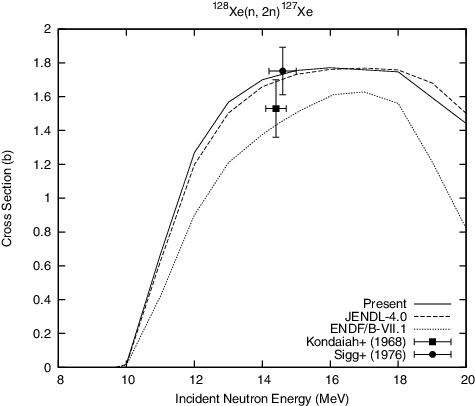
Figure 11. Comparison of the present 134Xe(n,2n)133Xe reaction cross section with the evaluated and experimental data.
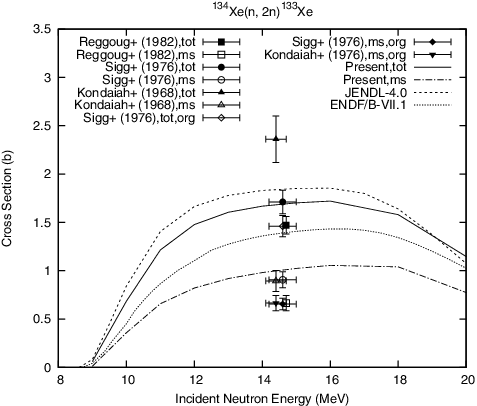
In 134Xe(n, 2n) reaction, Reggoug et al. [Citation49] and Sigg and Kuroda provided total reaction cross sections of 1.47 and 1.46 b at 14.7 and 14.6 MeV, respectively, whereas Kondaiah et al. reported the cross section 2.36 b at 14.4 MeV much larger than those of the former. Those cross sections might be incorrect, considering the systematics of (n, 2n) reaction cross section for 128 − 136Xe (typically 1.8 b at 14.5–15 MeV). Sigg and Kuroda adopted Iγ = 14% for the 233.2 keV gamma ray from the internal transition of 133mXe. The up-to-date value is 10.1%. This means that more plausible cross section for 133mXe production is larger by 38% (open circle in ). The revision for the 133gXe was also needed, but the measured data were not corrected due to their use of two gamma rays to derive activation cross section. Therefore, the revised 133mXe production cross section was simply added to the original 133gXe production one, in order to obtain the total 133Xe production cross section (filled circle). The 133mXe production data of Kondaiah et al. (open triangle) were also revised by the same way as the case of Sigg and Kuroda. It should be noted that the data of Reggoug et al. were not corrected since no information on decay monitors was available. The present data for 133Xe total production cross section were fitted to revised data of Sigg and Kuroda, while 133mXe cross section was marginally fitted to the revised data.
A recent experiment for 136Xe(n, 2n)135Xe reaction in the energy range of 9–14.9 MeV was performed by Bhatia et al. [Citation50] which were done by using neutron activation method. In this experiment, they measured cross sections to 526.6 keV isomeric states of 135Xe. Experimental data for total and isomer productions are depicted in . It is found that the calculated cross sections for isomer are fairly fitted with the experimental data. The data of Kondaiah et al. and Sigg and Kuroda are smaller than the Bhatia cross sections. The cross sections of present calculation and JENDL-4.0 are consistent with the data of Bhatia et al. for total production cross section of 135Xe. However, ENDF/B-VII.1 evaluation shows consistency with the data of Bhatia et al., particularly at energy below 10 MeV and at 14.9 MeV, at which 3H(d, n)4He reaction was employed as neutron sources, differing from the measurements at other energies by 2H(d, n)3He reaction. Furthermore, ENDF/B-VII.1 data agree with the work of Kondaiah et al.
3.3. (n, p) reaction cross section
stands for the comparison of 128Xe(n, p) reaction cross section of the present calculation with experimental data provided by Sigg and Kuroda along with those of the evaluated libraries. The data of Sigg and Kuroda were revised by using the up-to-date intensity Iγ = 12.6% for 442.9 keV gamma ray from the decay of 128I (filled circle). The cross section increases by 62%. The present result reproduces well the revised data. The other evaluations, however, explain the original smaller cross section (open circle).
Figure 13. Comparison of the present 128Xe(n,p)128I reaction cross section with the evaluated and experimental data.
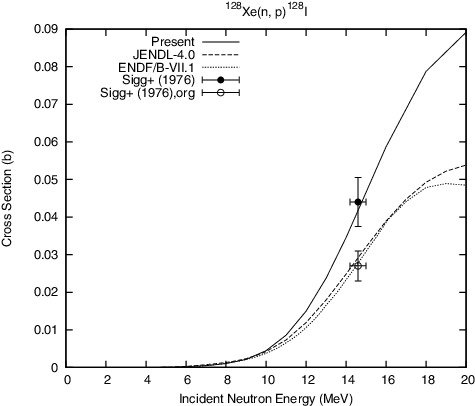
shows comparison of 131Xe(n, p) reaction cross section between the experimental data and calculated values. The cross sections of the present evaluation and ENDF/B-VII.1 agree with the data of Sigg and Kuroda. Although JEFF-3.2 is fairly consistent with the measured data, the shape of the excitation function is different compared to other libraries at energies above 15 MeV. In contrast, JENDL-4.0 evaluation is unable to reproduce the two data points.
3.4. (n, α) reaction cross section
The cross section of (n, α) reaction was calculated for natural Xe and compared with experimental data of Mack and Tornow [Citation51] as shown in . In addition to the statistical and pre-equilibrium α-emissions, the direct-like α-cluster emission by knock-out process was incorporated with a factor of 6.7 enhancement for all Xe stable isotopes, in order to better explain the energy dependence of cross section, while α-emission spectra have not been available. The presently calculated data and JENDL-4.0 reproduce well the measured cross section. In contrast, JEFF-3.2 and ENDF/B-VII.1 gave higher cross sections than the measured one, particularly at incident energy above 14 and 13 MeV, respectively.
3.5. Elastic scattering angular distribution
The angular distributions of elastically scattered neutrons were calculated at incident neutron energies 1 and 14 MeV as shown in and are compared with those of JENDL-4.0 and ENDF/B-VII.1. Unfortunately, there are no experimental data available. The differences among three data are not so large despite the use of different OMPs.
3.6. Neutron-production double-differential cross section
illustrates the comparison between the calculated neutron-production double-differential cross sections (DDXs) and those of JENDL-4.0 for natural Xe. The DDX as well as γ-ray spectrum below for natural element was derived by considering their natural isotopic abundances. The discrete inelastic and elastic scattering contributions of emission DDXs were broadened by using Gaussian distribution with resolution proportional to emission energies multiplied by 0.02. It is to be noted that there is no experimental information available neither for natural Xe nor its isotopes. Furthermore, the DDX of natural Xe for other evaluated libraries is not available due to incomplete data of each isotope. In this comparison, the neutron energies of incident channels at 5 and 14 MeV were considered. It is observed that the calculated neutron emission DDX provides almost similar result with that of JENDL-4.0, except that the contribution of pre-equilibrium component in JENDL-4.0 is slightly smaller than that in the present results.
3.7. Gamma-ray spectrum
shows the comparison of calculated γ-ray spectra for natural Xe with those of JENDL-4.0 where the incident neutron energies of 0.5, 5 and 14 MeV were considered. This comparison tells that the present results are very similar in pattern to spectra of the evaluated library. It is to be noticed that there is no experimental data available so far for the γ-ray emission spectra of 124 − 136Xe as well as of natural Xe. As is the case with the neutron emission DDX, it was not possible to get the γ-ray spectrum for natural Xe from other evaluated libraries because there was no other library which has complete information of spectra for all isotopes except JENDL-4.0.
4. Conclusion
The neutron cross sections for nine stable Xe isotopes (124, 126, 128, 129, 130, 131, 132, 134, 136Xe) were calculated by using theoretical nuclear reaction model code, CCONE, and then compared with the available experimental information to validate the calculated results. In addition, major evaluated data libraries were also included in the comparison to visualize the differences between the previous and present evaluations. The evaluation was made in the energy region from 1 keV to 20 MeV. The coupled-channels optical model code, CCOM, was used to calculate the total cross section with modifications of some OMP parameters given by Kunieda et al. in order to get the better agreement with the experimental data. The contributions of pre-equilibrium and direct processes in the statistical model calculations were considered to obtain reaction cross sections, gamma-ray and particle emission spectra. The present evaluation, indeed, can reasonably explain the experimental data of total, capture, (n, 2n), (n, p) and (n, α) reaction cross sections. The angular distributions of elastically scattered neutrons, neutron emission DDX and γ-ray emission spectra were calculated at particular incident neutron energies for natural Xe and compared with evaluated libraries where no experimental information was found.
The obtained capture cross sections for 124, 126Xe are smaller than the data of JENDL-3.2 in the keV energy region where the neutron spectrum of YAYOI has a large contribution. Hence, this evaluation could be able to improve the overestimation of C/E value found by the analysis of YAYOI experiment. Moreover, the present capture cross section of 136Xe, for instance, describes the latest experimental data better than all other evaluated libraries. In addition, the evaluated total (n, 2n) reaction cross section of 136Xe is in good agreement with the experimental data. Therefore, the present data of 136Xe could provide relevant cross-section data for KamLAND-Zen and EXO experiments.
Acknowledgement
This work has been supported by JSPS KAKENHI [grant number 22360429].
Disclosure statement
No potential conflict of interest was reported by the authors.
References
- Katakura J. JENDL FP decay data file 2011 and fission yields data file, JAEA-Data/Code 2011-025. Tokai: Japan Atomic Energy Agency; 2012.
- Kikuchi Y, Nakagawa T, Asami T, et al. Second version of Japanese evaluated nuclear data library (JENDL-2). J Nucl Sci Technol. 1985;22:593–603.
- Igarasi S, Fukahori T. Program CASTHY – statistical model calculation for neutron cross sections and gamma ray spectrum - (JAERI 1321). Tokai: Japan Atomic Energy Research Institute; 1991.
- Asami T, Iijima S, Igarasi S, et al. [Japanese evaluated nuclear data library version 3, JENDL-3]. J At Energy Soc Jpn. 1989;31:1190–1217. Japanese.
- Nakagawa T, Iijima S, Sugi T, et al. PEGASUS: a preequilibrium and multi-step evaporation code for neutron cross section calculation (JAERI-Data/Code 99-031). Tokai: Japan Atomic Energy Research Institute; 1999.
- Nakagawa T, Shibata K, Chiba S, et al. Japanese evaluated nuclear data library version 3 revision-2: JENDL-3.2. J Nucl Sci Technol. 1995;32:1259–1271.
- Shibata K, Kawano T, Nakagawa T, et al. Japanese evaluated nuclear data library version 3 revision-3: JENDL-3.3. J Nucl Sci Technol. 2002;39:1125–1136.
- Shibata K, Iwamoto O, Nakagawa T, et al. JENDL-4.0: a new library for nuclear science and engineering. J Nucl Sci Technol. 2011;48:1–30.
- Ichihara A, Iwamoto O, Chiba S, et al. Program POD; a computer code to calculate cross sections for neutron-induced nuclear reactions (JAEA-Data/Code 2007-012). Tokai: Japan Atomic Energy Agency; 2007.
- Soukhovitski E, Chiba S, Iwamoto O, et al. Programs of OPTMAN and SHEMMAN version 8 (2004) (JAERI-Data/Code 2005-002). Tokai: Japan Atomic Energy Research Institute; 2005.
- Aoyama T, Ito C. Integral test on activation cross-section of tag gas nuclides using fast neutron spectrum fields. J Nucl Sci Technol. 2002;Suppl. 2:1025–1028.
- Gando A, Gando Y, Hanakago H, et al. Measurement of the double-β decay half-life of 136Xe with the KamLAND-Zen experiment. Phys Rev. 2012;C 85:045504.
- Auger M, Auty DJ, Barbeau PS, et al. Search for neutrinoless double-β decay in 136Xe with EXO-200. Phys Rev Lett. 2012;109:032505.
- Iwamoto N. Neutron nuclear data evaluation of cesium isotopes for JENDL-4.0. J Nucl Sci Technol. 2011;48:1238–1249.
- Iwamoto N. Evaluation of neutron nuclear data for technetium-99. J Nucl Sci Technol. 2012;49:244–252.
- Minato F. Evaluation of neutron nuclear data of praseodymium-141 and 143. J Nucl Sci Technol. 2013;50:873–882.
- Shibata K. Evaluation of neutron nuclear data on tellurium isotopes. J Nucl Sci Technol. 2015;52:490–502.
- Iwamoto O. Development of a comprehensive code for nuclear data evaluation, CCONE, and validation using neutron induced cross sections for uranium isotopes. J Nucl Sci Technol. 2007;44(5):687–697.
- Tamura T. Analyses of the scattering of nuclear particles by collective nuclei in terms of the coupled-channel calculation. Rev Mod Phys. 1965;37:679–708.
- Hauser W, Feshbach H. The inelastic scattering of neutrons, Phys Rev. 1952;87:366–373.
- Kalbach C. Two-component exciton model: basic formalism away from shell closures. Phys Rev. 1986;C33:818–833.
- Tamura T. Analyses of the scattering of nuclear particles by collective nuclei in terms of the coupled-channel calculation. Rev Mod Phys. 1965;37:679–708.
- Iwamoto O. Program CCOM – coupled-channels optical model calculation with automatic parameter search –. JAERI-Data/Code 2003-020. Tokai: Japan Atomic Energy Research Institute; 2003.
- Kunieda S, Chiba S, Shibata K, et al. Coupled-channels optical model analyses of nucleon-induced reactions for medium and heavy nuclei in the energy region from 1 keV to 200 MeV. J Nucl Sci Technol. 2007;44(6):838–852.
- Raman S, Nestor Jr CW, Tikkanen P. Transition probability from the ground to the first-excited 2+ state of even-even nuclides. At Data Nucl Data Tables. 2001;78:1–128.
- Koning AJ, Delaroche JP. Local and global nucleon optical models from 1 keV to 200 MeV. Nucl Phys A. 2003;713:231–310.
- Lohr JM, Haeberli W. Elastic scattering of 9-13 MeV vector polarized deuterons. Nucl Phys A. 1974;232:381–397.
- Becchetti FD Jr, Greenlees GW. A general set of 3He and triton optical-model potentials for A>40, E<40 MeV. In Barshall HH, Haeberli W, editors. Polarization phenomena in nuclear reactions. Madison: University of Wisconsin Press; 1971. p. 682–683.
- Avrigeanu M, Avrigeanu V. α-particle nuclear surface absorption below the Coulomb barrier in heavy nuclei. Phys Rev C. 2010;82:014606.
- Vaughn FJ, Imhof WL, Johnson RG, et al. Total neutron cross sections of helium, neon, argon, krypton and xenon. Phys Rev. 1960;118:683–686.
- Chadwick MB, Herman M, Obložinský P, et al. ENDF/B-VII.1 nuclear data for science and technology: cross sections, covariances, fission product yields and decay data. Nucl Data Sheets. 2011;112;2887–2996.
- Koning AJ, Duijvestijn MC. A global pre-equilibrium analysis from 7 to 200 MeV based on the optical model potential. Nucl Phys A. 2004;744:15–76.
- Kalbach C. The Griffin model, complex particles and direct nuclear reactions. Z Phys. 1977;A283:401–411.
- Capote R, Herman M, Obložinský P, et al. RIPL – input parameter library for calculation of nuclear reactions and nuclear data evaluations. Nucl Data Sheets. 110;2009:3107–3214.
- Gilbert A, Cameron AGW. A composite nuclear level density formula with shell corrections. Can J Phys. 1965;43:1446–1496.
- Myers WD, Swiatecki WJ. Nuclear masses and deformations. Nucl Phys. 1966;81:1–60.
- Audi G, Wapstra AH, Thibault C. The AME2003 atomic mass evaluation (II). Nucl Phys A. 2003;729:337–676.
- Mengoni A, Nakajima Y. Fermi-gas model parametrization of nuclear level density. J Nucl Sci Technol. 1994;31:151–162.
- Kopecky J, Uhl M. Test of gamma-ray strength functions in nuclear reaction model calculations, Phys Rev. 1990;C41:1941–1955.
- Belgya T, Bersillon O, Capote Noy R, et al. Handbook for calculations of nuclear reaction data, RIPL-2, International Atomic Energy Agency, IAEA-TECDOC-1506. Vienna, Austria: International Atomic Energy Agency; 2006.
- Otuka N, Dupont E, Semkova V, et al. Towards a more complete and accurate experimental nuclear reaction data library (EXFOR): international collaboration between nuclear reaction data centres (NRDC). Nucl Data Sheets. 2014;120:272–276.
- OECD Nuclear Energy Agency Data Bank. Available from http://www.oecd-nea.org/dbforms/data/eva/ evatapes/jeff_32/
- Bhike M, Fallin B, Gooden ME, et al. Comprehensive sets of 124Xe(n, γ)125Xe and 124Xe(n, 2n)123Xe cross-section data for assessment of inertial-confinement deuterium-tritium fusion plasma. Phys Rev. 2015;C 91:011601(R).
- Reifarth R, Heil M, Kaeppeler F, et al. Stellar neutron capture cross sections of 128, 129, 130Xe. Phys Rev. 2002;C 66:064603.
- Bhike M, Tornow W. Neutron-capture cross-section measurements of 136Xe between 0.4 and 14.8 MeV. Phys Rev. 2014;C 89:031602(R).
- Sigg RA, Kuroda PK. 14.6-MeV neutron activation cross sections for the Xenon isotopes. Nucl Sci Eng. 1976;60:235–238.
- Kondaiah E, Ranakumar N, Fink RW. Neutron activation cross sections at 14.4 MeV for Kr and Xe isotopes. Nucl Phys A. 1968;120(2):337–341.
- Bazan F. (n,2n) cross-section measurements of Krypton and Xenon isotopes. UCRL-53929. Berkeley and Livermore: U.C. Lawrence Radiation Laboratory; 1989. p. 162.
- Reggoug A, Paic G, Berrada M. Measurement of (n,2n) reaction cross sections by X-ray spectroscopy. Rabat: Mohammed V; 1982. ( Annual Report No. 5: 14).
- Bhatia C, Finch SW, Gooden ME, et al. 136Xe(n,2n)135Xe cross section between 9 and 15 MeV. Phys Rev. 2013;C 87:011601.
- Mack G, Tornow W. Die Reaktion 54Xe(n, α)52Te mit Neutronen der Energie 12,5 bis 18,0 MeV [The Reaction 54Xe(n, α)52Te at neutron energies from 12.5 to 18.0 MeV]. Z Phys. 1967;207:359–374.