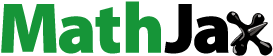
ABSTRACT
Nuclear reactions of gamma-rays with materials have attracted much attention in various application fields. To allow the use in those fields, JENDL photonuclear data file was updated from former JENDL/PD-2004 and was opened as JENDL/PD-2016 in 2017. The nuclear data were revised by adopting resonance analysis-like method for light nuclides and applying nuclear reaction models to the evaluations for heavier nuclides. In JENDL/PD-2016, standard and expanded versions are provided up to an incident energy of 140 MeV, covering 181 and 2681 nuclides, respectively. The evaluated results show better reproducibility to measured data, relative to JENDL/PD-2004. JENDL/PD-2016 is freely available from a website.
Graphical Abstract
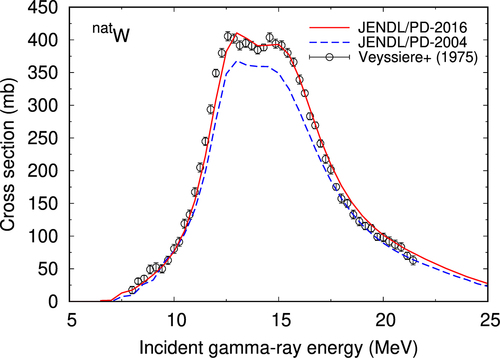
1. Introduction
Many experimental studies for photo-induced nuclear (photonuclear) reactions have used gamma-rays produced by methods with electron bremsstrahlung (e.g. [Citation1]), annihilation in flight of positrons (e.g. [Citation2]), and laser inverse-Compton scattering (e.g. [Citation3]). They have investigated especially the form of giant dipole resonance (GDR) related to collective motion of neutrons and protons in a nucleus. These efforts result in providing photo-neutron and photo-fission cross sections for a variety of nuclides. These cross section data were collected, and the typical compilation was made by Dietrich and Berman [Citation4].
Photonuclear data have drawn much attention from many application fields and subjects: shielding design of accelerator facilities [Citation5], assessment and management of activation materials [Citation6,Citation7], impact assessment of photo-neutrons in a fusion reactor [Citation8–10], estimation of transmutation of long-lived fission products [Citation11], estimation of patient dose during radiotherapy [Citation12,Citation13], nuclear inspection, and safeguards by non-destructive assay for detecting fissile materials [Citation14,Citation15], radioisotope production for medical use [Citation16], development of photo-induced neutron source [Citation17], and nucleosynthesis in hydrostatic and explosive stages of stars [Citation18,Citation19].
The development of photonuclear data library became active around the world in the late 1990s. International Atomic Energy Agency (IAEA) held collaborative research projects for making photonuclear data library and released the product with 164 nuclides in 1999 [Citation20,Citation21]. Around the same time, LA150 developed in the USA was released and it contains the data of 12 nuclides up to an incident energy of 150 MeV [Citation22]. Korean Atomic Energy Research Institute also published the photonuclear data library with 143 nuclides up to 140 MeV in 2000 [Citation23]. The release of Japanese evaluated file, JENDL/PD-2004 [Citation24], followed the above two libraries. These three libraries provided some of the data to develop the IAEA library. TENDL series have included comprehensive data up to 200 MeV for over 2600 nuclides based on nuclear reaction model calculations since 2008 [Citation25]. ENDF/B-VIII.0 prepares the photonuclear data for 164 nuclides [Citation26]. Updated collaborative research project hosted by IAEA from 2016 to 2019 developed photonuclear data library, IAEA/PD-2019, in which the nuclear data for 219 nuclides are included and maximum energy for some nuclides is extended up to 200 MeV [Citation27].
JENDL/PD-2004 is the first version of photonuclear data file in JENDL series and it was released in 2004 [Citation24]. In JENDL/PD-2004, the nuclear data for 68 nuclides are contained up to the gamma-ray energy of typically 140 MeV. The light and structural nuclides were mainly selected as well as 235,238U and 237Np. Since the release of JENDL/PD-2004, improvement of cross sections and extension of nuclide range are required with expanding application fields for photo-induced reactions. Hence, new photonuclear data file of JENDL was developed [Citation28] and released as JENDL/PD-2016 in December 2017.
This paper aims to explain the details on the development of JENDL/PD-2016. The evaluation methods of nuclear data and a data format are expressed in Section 2. Some evaluated results are shown in Section 3. Summary is presented in Section 4.
2. Evaluation methods and data format
First, the nomenclature related to photonuclear cross sections is defined in this section (see also [Citation20,Citation27]). Photo-absorption cross section is that gamma-rays are absorbed to nuclides. Photo-fission cross section is that gamma-rays trigger fission reaction on nuclides. Photo-neutron cross section represented by () is that reaction of nuclides with gamma-rays generates neutrons. In relation to the photo-neutron cross sections, the cross section of one-neutron emission reactions such as (
), (
) and (
) ones is collectively referred to as (
) reaction cross section. In the case of two-neutron emissions, it is expressed as (
). Photo-neutron yield cross section represented by (
) is that neutron multiplicity is taken into account for each photo-neutron cross section.
JENDL/PD-2016 contains the nuclear data of photo-absorption cross sections, production cross sections of light particles, gamma-rays and residual nuclides, and energy-angle distributions (i.e. double differential cross sections with respect to solid angle and emission energy) of light particles and gamma-rays. If nuclides are fissile, the fission-related data (average number of total neutrons, prompt neutrons and delayed neutrons, photo-fission cross section, and energy-angle distribution of fission neutrons) are added. The list of used MF and MT in the ENDF-6 format [Citation29] is summarized in . The maximum energy of the data is 140 MeV, which corresponds to the threshold of pion production. This upper limit is the same as JENDL/PD-2004 in which the upper limits exceeding 140 MeV are given for 19 nuclides (3He, 40Ca, 60Ni, 152,154–158,160Gd, 181Ta, 196,198–202,204Hg and 209Bi).
Table 1. List of MF and MT used in JENDL/PD-2016.
Two versions are prepared in JENDL/PD-2016, in which the number of nuclides is different. One is the standard version, in which the nuclear data of 181 nuclides in and close to the -stability line are prepared. This is for use to typical applications e.g. in estimating dose rate and photo-induced activation. The other is the expanded version aiming wider application fields regardless of conventional uses. The nuclear data of 2681 nuclides covering unstable nuclides far from the
-stability line are included. The nuclear data in the standard version are taken in the expanded version.
The nuclear data of 2H and 3He were adopted from JENDL/PD-2004. For 2H, MF3/MT3, MF3/MT28 and MF6/MT28 in the ENDF-6 format were adopted since 2H disintegrates into proton and neutron, regarding this process as the () reaction (MT28) with no residual nuclide. For 3He, the storage form of cross sections and energy-angle distributions for neutron, proton and deuteron productions was changed from JENDL/PD-2004, and consolidated into MF6/MT5. For the other nuclides, JENDL/PD-2004 contains the energy-angle distributions of emitted particles in MF6/MT201,203–207. In JENDL/PD-2016, these data are stored in MF6/MT5. The distributions of emitted gamma-ray are also given in MF6/MT5, except for 2H, 3He and light nuclides listed in .
Table 2. List of evaluated nuclides based on the resonance analysis-like method.
In the development of JENDL/PD-2016, three methods were applied for evaluation; (i) evaluation by resonance analysis-like method for light nuclides, (ii) evaluation by the nuclear reaction model code CCONE [Citation30], and (iii) evaluation by the nuclear reaction model code ALICE-F [Citation31]. Each method will be explained below.
2.1. Evaluation by resonance analysis-like method
Since light nuclides with mass number smaller than, e.g., 50 have sparse excited levels and result in wide level spacings, measured photo-neutron cross sections show resonance shapes. In the resonance regions of those nuclides a usual statistical model is not applicable. A method similar to the analysis of neutron resonance (namely resonance analysis-like method) is required to follow the shape of cross sections. In order to make systematic evaluations for light stable nuclides from Li to Ca, the following methodologies were applied, and are briefly explained in this paper. The details are found in Murata et al. [Citation32].
When measured data of photo-absorption, photo-neutron and photo-neutron yield cross sections were available, the evaluations were made by resonance model and quasi-deuteron (QD) model [Citation33,Citation34]. The resonance cross section for photo-absorption was adopted with multi-level consideration [Citation35] and given by
where is the incident gamma-ray energy,
is the spin of the target, subscript
stands for the value in an excited state inferred from measured data and in the known excited states of ENSDF [Citation36],
is the spin,
is the gamma-ray transition strength between the ground state and an excited state
,
,
, and
are the resonance energy in MeV, its wave number and resonance width in MeV, respectively.
On the other hand, when measured data were not available, a Lorentzian model with theoretical GDR parameters in RIPL-2 [Citation37] was applied, or GDR structure was deduced by level information in ENSDF [Citation36]. The photo-absorption cross sections () of the GDR expressed by the Lorentzian model are described by
where in mb is the normalization factor to meet the GDR sum rule [Citation38],
is the ratio of long to short axes for nucleus deformation and
,
and
are the resonance energy and width in MeV, respectively, for collective motions along long and short axes. The photo-absorption cross sections (
) by the QD process were calculated by
where is the Levinger parameter;
,
, and
are neutron, atomic, and mass numbers of target, respectively; and
is the free deuteron photodisintegration cross section in mb given by
is the normalization factor in mb MeV
,
is the binding energy of deuteron (2.223 MeV), and
is the Pauli blocking function [Citation34]. The factor
was adjusted to reproduce measured data in the region where the QD process is dominant. The present GDR sum rule with the effect of the QD process is described by
where is the normalization factor (typically
-110 mb MeV). The integration range is from the lowest energy to 140 MeV. This GDR sum rule was approximately imposed for the photo-absorption cross sections. After the determination of photo-absorption cross section, (
) and (
) reaction cross sections were evaluated using measured data. The cross sections of the remaining reactions were estimated by calculating branching ratios to the photo-absorption cross section by ALICE-F.
The decays to discrete levels by particle emissions are dominant in the low incident gamma-ray energy region. Those effects are expected to be seen in energy-angle distributions. However, since the information of discrete levels cannot be treated in ALICE-F, the energy-angle distributions for emitted light particles were calculated by CCONE. The normalized distributions were adopted for light nuclides.
The evaluated nuclides based on this framework are listed in , in which the nuclear data of 13C, 15N, 17,18O, 20–22Ne, 32–34,36S, 35,37Cl, 36,38,40Ar, and 42–44,46Ca were newly available in JENDL/PD-2016.
2.2. Evaluation by CCONE
The nuclear reaction model code CCONE [Citation30] was used to obtain photonuclear data of nuclides listed in , in which most of nuclides are stable with . CCONE comprises the Hauser-Feshbach statistical model and preequilibrium model. The incident gamma-ray energy region is from 1 MeV to the maximum energy. It should be noted that a simple photo-absorption cross section calculated by CCONE is compiled even in the energy region below a particle emission threshold (e.g. typically 5–10 MeV) where nuclear resonance fluorescence (NRF) is known to be prominent. In the present evaluation, the effect coming from NRF was not taken into account due to small cross sections, compared with particle emission ones.
Table 3. List of nuclides evaluated by CCONE.
In the statistical model calculations, information of excitation energy and gamma-ray decay branching ratios for discrete levels was taken from RIPL-3 [Citation39]. Nuclear level density above the discrete levels was calculated by the composite form of constant temperature and Fermi-gas models [Citation40], in which the Fermi-gas model was revisited by Mengoni and Nakajima [Citation41]. Emission particles are considered to be neutron, proton, deuteron, triton, 3He and -particle. Emission probabilities of these particles were obtained by optical model calculations with potentials of Koning and Delaroche (KD03) [Citation42] for neutrons and protons, Han et al. [Citation43] for deuterons, folding model based on KD03 for tritons, Xu et al. [Citation44] for 3He, and Avrigeanu and Avrigeanu [Citation45] for
-particles. Gamma-ray strength function is an important factor to estimate the photo-absorption (and photo-neutron) cross sections. In this evaluation, E1, M1, and E2 transitions were taken into account for the gamma-ray decays from continuum states. For the E1 transition, modified Lorentzian model (MLO1) [Citation46] was utilized. The GDR parameters were adjusted so as to reproduce measured photo-absorption, photo-neutron, and photo-neutron yield cross sections. When measured data were not available, the systematics of the GDR parameters taken from RIPL-3 was employed. For M1 and E2 transitions, the formulations of Kopecky and Uhl [Citation47] were applied.
The preequilibrium process was calculated with the two-component exciton model [Citation48,Citation49]. The initial particle-hole configuration was set to be -
, which was assumed in the neutron shell. This configuration may be unphysical for photonuclear reactions, but it is validated based on the results that measured data of energy-angle distributions could be well reproduced rather than adopting more appropriate 1
-1
and 2
-2
configurations for the GDR and QD process, respectively.
The energy-angle distributions for emissions of the light particles and gamma-ray were calculated by the above models and were stored in MF6/MT5. Specially for light nuclides in , the normalized distributions were included only for light particles as explained in Section 2.1.
The average number of delayed neutrons per fission for 232Th, 233–236,238U, 237Np, 238,239,241Pu, and delayed neutron spectrum for 233–236,238U, 237Np, 239,241Pu were brought from the neutron nuclear data library, JENDL-4.0 [Citation50]. The average number of prompt neutrons per fission () was estimated as the linear function with respect to gamma-ray energy below (
) reaction threshold for 232Th, 233–236,238U, 237Np, and 238,239,241Pu [Citation51,Citation52]. Above the (
) reaction threshold, an exponential function (EquationEquation (6)
(6)
(6) [Citation53,Citation54]) was assumed and smoothly connected with the linear one at the threshold energy. For the other fissile nuclides, the following functions were applied:
where if
,
if
,
,
is the threshold energy of (
) reaction,
and
are in MeV−1,
and
are in MeV. The mass number dependences of
and
were derived by least square fits to the known parameters [Citation51,Citation52].
For the QD process, the same formalism as EquationEquation (3)(3)
(3) was used, but the normalization factor
, in which
by default in CCONE, was modified to reproduce measured data. Note that
reduces to 120
if adopting
.
2.3. Evaluation by ALICE-F
For nuclides up to 266Lr () other than listed in , the following calculation method was applied to deriving the nuclear data.
The photo-absorption cross section was calculated by the Lorentzian model of EquationEquation (2)(2)
(2) with GDR parameters in RIPL-3 and by the QD model. The GDR parameters were adjusted to match measured photo-absorption and photo-neutron cross sections. The QD model was the same as EquationEquation (3)
(3)
(3) , but
is modified as follows:
for light nuclides,
for nuclides with
, and
for the other nuclides. The definition of the GDR sum rule is the same as EquationEquation (5)
(5)
(5) , but the normalization factor
was changed as follows:
mb MeV for light nuclides,
for nuclides with
, and
for nuclides with
. For the photo-absorption cross sections excluding photo-fission ones, the condition of GDR sum rule was approximately satisfied.
The ALICE-F code consists of a evaporation model [Citation55] and a hybrid model for preequilibrium process. ALICE-F was employed to obtain ratios of particle-emission and residual production cross sections to the photo-absorption one, as well as energy-angle distributions of emitted particles calculated by the systematics of Kalbach [Citation56]. As the emission particles, neutrons, protons, deuterons, tritons, 3He, -particles and gamma-rays were taken into account. The incident energy range is from a smallest threshold energy of particle emissions to the maximum energy, where the
-values of photonuclear reactions were calculated with the atomic mass evaluated by Wapstra and Audi [Citation57].
The calculation of photo-fission cross section was taken into account for nuclides with by the FISCAL2003 code [Citation58].
3. Examples of evaluation results
In the present evaluation, the measured data were taken from EXFOR [Citation59]. Some of these data were recommended to multiply a factor by cross sections published in the 1960s and 1970s, in order to correct the normalization of cross sections (e.g. [Citation60]). In addition to this, cross sections were renormalized to be consistent with other more reliable or up-to-date measured data. The measured data, for which renormalization was made, are listed in .
Table 4. Normalization factors applied in the present evaluation.
shows the photo-neutron cross sections by () reaction for 28Si in JENDL/PD-2016, compared with JENDL/PD-2004 and measured data [Citation73–76]. Since the (
) reaction cross section is not normally included in many of photonuclear data files except for TENDL, the production cross section of residual nuclides is substituted for a comparison purpose. In the case of 28Si, the (
) reaction cross section corresponds to the production cross section of 27Si. Hereafter such substitution is made, when the reaction product coincides with the residual. The data of Pywell et al. [Citation75], Caldwell et al. [Citation76], and Goryachev et al. [Citation73] were measured with neutron-detection techniques, by which the cross sections of (
) reaction can be obtained. The data of Webb et al. [Citation74] were measured by activation technique and correspond to pure (
) reaction cross sections. The threshold energies of (
) and (
) reaction are 24.6 and 26.5 MeV, respectively. In the region between 17.2 and 24.6 MeV, (
) reaction is only responsible for neutron production. The data of Goryachev et al. [Citation73] are larger than those of Pywell et al. [Citation75] and Caldwell et al. [Citation76] below 21 MeV, below which it seems that JENDL/PD-2004 reproduces the data of Goryachev et al. [Citation73]. In JENDL/PD-2016, the data of Webb et al. [Citation74] and Caldwell et al. [Citation76] were adopted for evaluation and are well reproduced with resonance analysis-like method. Above 21 MeV, the present evaluation gives consistent results with the data of Caldwell et al. [Citation76].
Figure 1. Comparison of the () reaction cross section for 28Si in JENDL/PD-2016 with JENDL/PD-2004 and measured data [Citation73–76]. The data of Webb et al. [Citation74] are for (
) reaction, and the others are for (
) reactions.
![Figure 1. Comparison of the (γ,n) reaction cross section for 28Si in JENDL/PD-2016 with JENDL/PD-2004 and measured data [Citation73–76]. The data of Webb et al. [Citation74] are for (γ,n) reaction, and the others are for (γ,1nx) reactions.](/cms/asset/457cbcda-fdf5-4e7e-8483-3cfc192bad0c/tnst_a_2161657_f0001_oc.jpg)
Although the half-lives of generated 13N and 15O are short in the order of minutes, attention has been paid to the activation of air in electron linear accelerator (linac) facilities. From this point of view, the photonuclear cross sections of nitrogen, oxygen, and the other constituents of air were evaluated with the detailed analyses [Citation32] in JENDL/PD-2016. Some evaluation examples of these nuclides were already presented in Refs [Citation32,Citation77]. Here, the neutron emission energy-angle distributions by photonuclear reaction of 14N at incident energy of 22.5 MeV and emitted angle of 90° are shown for JENDL/PD-2016 and JENDL/PD-2004, together with measured data [Citation1] in . The energy-angle distributions (MF6) for light particle emissions in JENDL/PD-2016 were adopted from the results calculated by CCONE as already mentioned. The neutrons following these distributions are expected to accompany the productions of 13N. It is found that the continuum component of the distribution in JENDL/PD-2016 is extremely small compared to heavier nuclides and that the direct transitions to discrete levels of 13N by neutron emissions from 14N is dominant at a low incident energy region, supplemented with the de-excitation neutrons from discrete levels of 13C generated by the 14N() reaction. A prominent peak by de-excitation neutrons from 13C is seen around 6.5 MeV in the measured data. The present result unfortunately shows a tiny contribution of the de-excitation. JENDL/PD-2004 does not have any signature of the transitions from the discrete levels. As the incident energy goes up, the effect of direct transition to discrete levels becomes small. On the other hand, JENDL/PD-2004 represents a usual evaporation peak around 1 MeV. However, this peak does not exist in the measured data. Hence, the present data better explain the global feature of measured distribution than JENDL/PD-2004. Another feature can be seen in JENDL/PD-2004 which shows an abrupt drop above the neutron emission energy of 8 MeV. This drop comes from a data interpolation between the incident energies of 22 and 24 MeV given in MF6.
Figure 2. Comparison of the energy-angle distribution of emitted neutrons for 14N in JENDL/PD-2016 with JENDL/PD-2004 and measured data [Citation1]. The distributions are illustrated at the incident gamma-ray energy of 22.5 MeV and neutron emission angle of 90°. The data of Sherman et al. [Citation1] were derived with difference spectrum by subtraction of 21.5-MeV bremsstrahlung spectrum from 23.5-MeV one. The normalization of measured data was made on the basis of JENDL/PD-2016.
![Figure 2. Comparison of the energy-angle distribution of emitted neutrons for 14N in JENDL/PD-2016 with JENDL/PD-2004 and measured data [Citation1]. The distributions are illustrated at the incident gamma-ray energy of 22.5 MeV and neutron emission angle of 90°. The data of Sherman et al. [Citation1] were derived with difference spectrum by subtraction of 21.5-MeV bremsstrahlung spectrum from 23.5-MeV one. The normalization of measured data was made on the basis of JENDL/PD-2016.](/cms/asset/2a943d6f-d678-4145-a566-d1d9d4381a66/tnst_a_2161657_f0002_oc.jpg)
Manganese is contained in structural materials, although its amount is small. For comprehensive estimation of activation induced by photo-neutrons in an electron linac facility, the photonuclear data of Mn are required. compares the photo-neutron yield, () and (
) reaction cross sections of 55Mn in JENDL/PD-2016 with JENDL/PD-2004 and measured data [Citation78–80]. The photo-neutron yield cross section below 20 MeV in JENDL/PD-2004 explains the data of Alvarez et al. [Citation80]. In contrast, above 20 MeV it is unexpectedly large. The same situation is encountered for the (
) and (
) reaction cross sections in JENDL/PD-2004. Such errors should be improved. The present photo-neutron yield cross section is consistent with the data of Alvarez et al. [Citation80] above 18 MeV and Ishkhanov et al. [Citation79]. The (
) and (
) reaction cross sections in JENDL/PD-2016 are also reasonable.
Figure 3. Comparison of the photo-neutron yield, () and (
) reaction cross sections for 55Mn in JENDL/PD-2016 (solid, long-dashed and dot-dashed lines, respectively) with JENDL/PD-2004 (short-dashed, dotted and dot-dot-dashed lines, respectively), and measured data [Citation78–80].
![Figure 3. Comparison of the photo-neutron yield, (γ,n) and (γ,2n) reaction cross sections for 55Mn in JENDL/PD-2016 (solid, long-dashed and dot-dashed lines, respectively) with JENDL/PD-2004 (short-dashed, dotted and dot-dot-dashed lines, respectively), and measured data [Citation78–80].](/cms/asset/ff055d61-c38d-487e-9872-c34a191a3ac1/tnst_a_2161657_f0003_oc.jpg)
Copper is frequently used in a head of medical electron linac to generate neutrons. The photonuclear data of Cu is important for shielding design of electron linac facility. There were experiments to measure the angular distributions of neutron ambient dose equivalent rate (hereafter neutron dose rate) and 27Al() reaction rate at a 45 MeV electron linac of Hokkaido University [Citation77,Citation81,Citation82]. In the experiments, neutrons were generated by a photonuclear reaction between bremsstrahlung photons and a natural Cu target (consisting of stable 63,65Cu) bombarded with 18, 28, and 38 MeV-electrons. It was found that the results simulated with MCNP5 [Citation83] and JENDL/PD-2004 were underestimated, compared with the measured rates in especially the 18 MeV-electron case [Citation81]. In order to enhance the consistency with the measured rates, photo-neutron yield cross section larger than JENDL/PD-2004 was desired in the GDR region. The (
) reaction and photo-neutron yield cross sections of 65Cu in JENDL/PD-2016 are shown with JENDL/PD-2004 and measured data [Citation84–86] in . The present evaluation gives larger cross sections than the data of Fultz et al. [Citation84], especially in the incident energy region where the (
) reaction is dominant. The relative difference in (
) reaction cross sections reaches to about 40% between 14 and 17 MeV incident energies. The discrepancy might be bigger than an acceptable value (up to 30% in ) for the correction of cross sections. In the MCNP5 simulation using JENDL/PD-2016, the angular distributions of neutron dose rate and 27Al(
) reaction rate increase and get closer to the measured ones in the 18 MeV-electron cases [Citation77,Citation82]. However, the resulting rates still remain underestimated. In , Katz and Cameron obtained (
) reaction cross sections much larger than JENDL/PD-2016, whereas they pointed out that there was uncertain contribution of the 64Cu production by the neutron capture reaction on 63Cu in their activation measurement with a natural Cu target. Therefore, their data are considered to be overestimated. It should be mentioned that the present evaluation for 63Cu also provides large cross sections for those reactions as is the case in 65Cu [Citation77].
Figure 4. Comparison of the () reaction and photo-neutron yield cross sections for 65Cu in JENDL/PD-2016 (solid and long-dashed lines, respectively) with JENDL/PD-2004 (short-dashed and dotted lines, respectively) and measured data [Citation84–86].
![Figure 4. Comparison of the (γ,n) reaction and photo-neutron yield cross sections for 65Cu in JENDL/PD-2016 (solid and long-dashed lines, respectively) with JENDL/PD-2004 (short-dashed and dotted lines, respectively) and measured data [Citation84–86].](/cms/asset/138e6eb8-e36d-423d-80b7-9f28eee6ab88/tnst_a_2161657_f0004_oc.jpg)
Tungsten is also used for neutron generation in a head of medical electron linac. The photonuclear data are requisite for the same purpose as Cu. JENDL/PD-2004 contains the data of only 182,184,186W, for which the total natural abundance is 85.6%. In JENDL/PD-2016 all natural isotopes were prepared, and the nuclear data of natural W are available in, e.g. simulations. compares the photo-neutron cross section of natural W in JENDL/PD-2016 with JENDL/PD-2004 and measured data [Citation87]. It should be noted that the data without the contributions of stable 180,183W for JENDL/PD-2004 are drawn. The measured photo-neutron data include the contributions of (), (
), (
), and (
) reactions below the incident energy of 20 MeV. According to the CCONE calculations, the cross sections of (
) and (
) reactions are normally negligible for heavier nuclides such as W isotopes (smaller than 1 mb). In the evaluation of JENDL/PD-2016, the photo-neutron cross sections were evaluated for each isotope, using measured data of photo-absorption and photo-neutron yield cross sections. The peak cross section of JENDL/PD-2004 is clearly smaller than JENDL/PD-2016 and the measured data due to the lack of 180,183W. This results in remarkable underestimation of amount of neutrons generated by tungsten sources.
Figure 5. Comparison of the photo-neutron cross section for natW in JENDL/PD-2016 (solid line) with JENDL/PD-2004 (dashed line) and measured data [Citation87].
![Figure 5. Comparison of the photo-neutron cross section for natW in JENDL/PD-2016 (solid line) with JENDL/PD-2004 (dashed line) and measured data [Citation87].](/cms/asset/1e6af945-ef39-41cf-a924-40786cf35742/tnst_a_2161657_f0005_oc.jpg)
The () reaction cross section of 197Au has been used as standard data for activation measurements. Many measurements with activation and neutron counting methods have been performed to obtain (
) and (
) reaction cross sections. First, the present (
) reaction cross section is depicted with JENDL/PD-2004 and the measured data [Citation2,Citation60,Citation70,Citation88–93] in . Note that the contributions from one-neutron emission reactions other than (
) reaction are possibly incorporated in the data particularly measured by Fultz et al. [Citation2], Veyssiere et al. [Citation70], and Berman et al. [Citation60]. However, the influence is likely to be small. JENDL/PD-2016 and JENDL/PD-2004 are consistent with the measured data within their uncertainties, although the most up-to-date data of Plaisir et al. [Citation93] have remarkably small cross sections between 14 and 17 MeV. It should be noted that there is the difference between JENDL/PD-2016 and JENDL/PD-2004 around the threshold energy of (
) reaction (8.07 MeV). This is because in JENDL/PD-2016 the cross sections were provided every 0.5 MeV in an incident energy below 20 MeV and the cross section was set to be zero at and below 8.5 MeV for the 196Au production.
Figure 6. Comparison of the () reaction cross section for 197Au in JENDL/PD-2016 (solid line) with JENDL/PD-2004 (dashed line) and measured data [Citation2,Citation60,Citation70,Citation88–93].
![Figure 6. Comparison of the (γ,n) reaction cross section for 197Au in JENDL/PD-2016 (solid line) with JENDL/PD-2004 (dashed line) and measured data [Citation2,Citation60,Citation70,Citation88–93].](/cms/asset/30778ece-b8ba-4959-abbf-6f36e1bff78b/tnst_a_2161657_f0006_oc.jpg)
Next, the comparisons of energy-angle and energy distributions of photo-neutrons emitted from 197Au in JENDL/PD-2016 are made with JENDL/PD-2004 and measured dataFootnote1 [Citation94,Citation95] in . In these comparisons, the incident energies of each measurement were taken from the end-point energies of electron bremsstrahlung. The energy-angle distributions in the incident energy of 14 MeV for JENDL/PD-2016 and JENDL/PD-2004 were derived at neutron emission angle of 90°. The energy distributions in the incident energy of 20 MeV were obtained by the summation of energy-angle distributions over neutron emission angles from 30° to 150° at 15° intervals. In the 14 MeV case, the present data give a consistent results with the measured data, although JENDL/PD-2004 shows abrupt drop around the neutron emission energy of 4 MeV. In the 20 MeV case, it is found that a non-statistical decay component seen above 4 MeV has an important contribution. A preequilibrium component in JENDL/PD-2016 reproduces the measured data in the region. In contrast, the distribution in JENDL/PD-2004 underestimates the measured data between 4.5 and 10 MeV, since the effect from preequilibrium process is weaker.
Figure 7. Comparison of the neutron emission energy-angle and energy distributions of 197Au in JENDL/PD-2016 with JENDL/PD-2004 and measured data [Citation94,Citation95]. The energy-angle distribution (left axis) was measured with the bremsstrahlung end-point energy of 14 MeV at the neutron emission angle of 90°. The energy distribution integrated over neutron emission angles of 30° to 150° (right axis) was measured with bremsstrahlung end-point energy of 20 MeV. Both data are based on relative measurements, and are normalized to the integral value calculated by the present distributions over measured neutron emission energy ranges.
![Figure 7. Comparison of the neutron emission energy-angle and energy distributions of 197Au in JENDL/PD-2016 with JENDL/PD-2004 and measured data [Citation94,Citation95]. The energy-angle distribution (left axis) was measured with the bremsstrahlung end-point energy of 14 MeV at the neutron emission angle of 90°. The energy distribution integrated over neutron emission angles of 30° to 150° (right axis) was measured with bremsstrahlung end-point energy of 20 MeV. Both data are based on relative measurements, and are normalized to the integral value calculated by the present distributions over measured neutron emission energy ranges.](/cms/asset/a0cf99a5-3e82-42a3-852d-5a1ad3bf2343/tnst_a_2161657_f0007_oc.jpg)
The photonuclear data of actinides such as 235,238U and 239Pu are considered to be important for nuclear inspection and safeguards, in order to estimate properly the amount of fissile materials. shows the evaluated result of the average number of prompt neutrons per fission () for 238U compared to JENDL/PD-2004, a least-square fit by Caldwell et al. [Citation51], and measured data [Citation51,Citation96,Citation97]. The present data below (
) reaction threshold are based on the least-square fit by Caldwell et al. [Citation51] and start to leave the fit at 11.3 MeV, following the present formulation (EquationEquation (6)
(6)
(6) ). In JENDL/PD-2004,
increases linearly below 26.2 MeV, although above the energy it is assumed to have a constant value (
). The data of Silano et al. [Citation97] show the sharp decrease below 5.2 MeV. This may be attributed to small photo-fission cross sections.
Figure 8. Comparison of the average number of prompt neutrons per fission for 238U in JENDL/PD-2016 (solid line) with JENDL/PD-2004 (dashed line), a least-square fit by Caldwell et al. [Citation51] (dotted line) and measured data [Citation51,Citation96,Citation97].
![Figure 8. Comparison of the average number of prompt neutrons per fission for 238U in JENDL/PD-2016 (solid line) with JENDL/PD-2004 (dashed line), a least-square fit by Caldwell et al. [Citation51] (dotted line) and measured data [Citation51,Citation96,Citation97].](/cms/asset/e2cc9136-eac2-4594-a094-6c19ce2e09b8/tnst_a_2161657_f0008_oc.jpg)
compares the photo-neutron yield cross section of 235U in JENDL/PD-2016 with JENDL/PD-2004 and measured data of Caldwell et al. [Citation98]. For fissile nuclides, the photo-neutron yield cross section stands for the total neutron production cross sections supplemented with the neutrons produced by fission reaction. The cross section of JENDL/PD-2016 is consistent with the measured data [Citation98]. In contrast, JENDL/PD-2004 is larger than measured data especially around 14 MeV, around which the cross section has a peak.
Figure 9. Comparison of the photo-neutron yield cross section for 235U in JENDL/PD-2016 (solid line) with JENDL/PD-2004 (dashed line) and measured data [Citation98].
![Figure 9. Comparison of the photo-neutron yield cross section for 235U in JENDL/PD-2016 (solid line) with JENDL/PD-2004 (dashed line) and measured data [Citation98].](/cms/asset/bc6fb70c-8c4d-4d18-af88-a517c8bcc8c7/tnst_a_2161657_f0009_oc.jpg)
The photo-fission cross section for 235U in JENDL/PD-2016 is depicted up to 140 MeV in , together with JENDL/PD-2004 and measured data [Citation98–107]. The evaluated result of JENDL/PD-2016 as well as JENDL/PD-2004 well reproduces the measured data even in the region above 40 MeV where the QD process is predominant. The difference between JENDL/PD-2016 and JENDL/PD-2004 is found below 7 MeV. The cross section of JENDL/PD-2016 is smaller than JENDL/PD-2004. However, it is still valid, compared with measured data in which relatively wide spread of cross sections can be seen around the critical energy.
Figure 10. Comparison of the photo-fission cross section for 235U in JENDL/PD-2016 (solid line) with JENDL/PD-2004 (dashed line) and measured data [Citation98–107].
![Figure 10. Comparison of the photo-fission cross section for 235U in JENDL/PD-2016 (solid line) with JENDL/PD-2004 (dashed line) and measured data [Citation98–107].](/cms/asset/ff1fdbe4-b732-463c-a469-2837a54d512f/tnst_a_2161657_f0010_oc.jpg)
4. Summary
Photonuclear data file was developed in order to meet various and expanding needs. JENDL/PD-2016 was released in December 2017. The file consists of standard and expanded versions, having photonuclear data of 181 and 2681 nuclides, respectively. The maximum energy is fixed at 140 MeV. The evaluations were performed with the resonance analysis-like method and the nuclear reaction model codes CCONE and ALICE-F, using available measured data. The nuclear data cover almost all photo-induced reactions required for applications, that is, photo-absorption, photo-neutron (proton, deuteron, triton, 3He and -particle and gamma-rays) yield cross sections, photo-fission cross sections, the average number of total, prompt and delayed neutrons per fission, energy-angle distributions of emitted particles and gamma-rays, delayed neutron fission spectrum (if available from JENDL-4.0) and production cross sections of residual nuclides. The data revised from JENDL/PD-2004 result in better agreements with the measured ones. JENDL/PD-2016 is available from a website of Nuclear Data Center in Japan Atomic Energy Agency: https://wwwndc.jaea.go.jp/ftpnd/jendl/jendl-pd-2016.html.
Acknowledgement
The authors are grateful to Dr Toru Murata for his many years’ contributions to photonuclear data evaluation of light nuclides.
Disclosure statement
No potential conflict of interest was reported by the author(s).
Notes
1. All information on the measurements by Zatsepina et al. [Citation94] and Lepestkin et al. [Citation95] relies on EXFOR [Citation59], because the details of their measurements and analyses are not available.
References
- Sherman NK, Gellie RW, Lokan KH, et al. De-excitation neutrons following 14N Photodisintegration. Phys. Rev. Lett. 1970 Jul;25:114–116.
- Fultz SC, Bramblett RL, Caldwell JT, et al. Photoneutron cross-section measurements on gold using nearly monochromatic photons. Phys. Rev. 1962 Aug;127:1273–1279.
- Utsunomiya H, Makinaga A, Goko S, et al. Photoneutron cross section measurements on the N = 82 nuclei 139La and 141Pr: implications for p-process nucleosynthesis. Phys. Rev. C. 2006 Aug;74:025806.
- Dietrich SS, Berman BL. Atlas of photoneutron cross sections obtained with monoenergetic photons. At. Data Nucl. Data Tables. 1988;38(2):199–338.
- Masuda K, Kii T, Ohgaki H, et al. Shielding analysis for a 40-MeV electron linac facility. Nucl. Technol. 2009;168(2):467–471.
- Almen A, Ahlgren L, Mattsson S. Absorbed dose to technicians due to induced activity in linear accelerators for radiation therapy. Phys. Med. Biol. 1991 Jun;36(6):815–822.
- Fischer HW, Tabot BE, Poppe B. Activation processes in a medical linear accelerator and spatial distribution of activation products. Phys. Med. Biol. 2006 Nov;51(24):N461–N466.
- Strachan JD, Meservey EB, Stodiek W, et al. Photo-neutron production in the PLT tokamak. Nucl. Fusion. 1977 Feb;17(1):140–144.
- Hendel HW, Jassby DL. The TOKAMAK as a Neutron Source. Princeton Plasma Phys. Lab. 1989. PPPL-2656.
- Breizman BN, Aleynikov P, Hollmann EM, et al. Physics of runaway electrons in tokamaks. Nucl. Fusion. 2019 Jun;59(8):083001.
- Sun XY, Luo W, Lan HY, et al. Transmutation of long-lived fission products in an advanced nuclear energy system. Sci. Rep. 2022;12:2240.
- D’Errico F, Luszik-Bhadra M, Nath R, et al. Depth dose-equivalent and effective energies of photoneutrons generated by 6–18 MV X-ray beams for radiotherapy. Health Phys. 2001;80(1):4–11.
- Fujibuchi T, Obara S, Sato H, et al. Estimate of photonuclear reaction in a medical linear accelerator using a water-equivalent phantom. Prog. Nucl. Sci. Technol. 2011;2:803–807.
- Kimura R, Sagara H, Chiba S. Precision requirement of the photofission cross section for the nondestructive assay. Vol. 146, EPJ Web of Conferences, Bruges, Belgium: 2017. p. 09041.
- Chin KW, Sagara H, Han CY. Application of photofission reaction to identify high-enriched uranium by bremsstrahlung photons. Ann. Nucl. Energy. 2021;158:108295.
- Bobeica M, Niculae D, Balabanski D, et al. Radioisotope production for medical applications at ELI-NP. Rom. Rep. Phys. 2016;68 Supplement:S847–S883.
- Sari A, Carrel F, Jouanne C, et al. Optimization of the photoneutron flux emitted by an electron accelerator for neutron interrogation applications using MCNPX and TRIPOLI-4 Monte Carlo Codes. In: Proceedings of the 4th International Particle Accelerator Conference (IPAC 2013): Shanghai, China, May 12-17, 2013; 2013. p. 3630–3632.
- Rauscher T, Thielemann FK. Predicted cross-sections for photon-induced particle emission. At. Data Nucl. Data Tables. 2004;88(1):1–81.
- Hayakawa T, Iwamoto N, Kajino T, et al. Empirical abundance scaling laws and implications for the gamma process in core-collapse supernovae. Astrophys J. 2008 Oct;685(2):1089–1102.
- IAEA. Handbook on photonuclear data for applications: cross sections and spectra. Vienna, Austria: IAEA; 2000. IAEA-TECDOC-1178.
- Obložinský P. Photonuclear Data. J. Nucl. Sci. Technol. Suppl. 2002;2:31–36.
- Chadwick MB. Neutron, proton, and photonuclear cross sections for radiation therapy and radiation protection: Los Alamos, NM: Los Alamos National Laboratory; 1998. LA-UR-98-4139.
- Han Y. KAERI photonuclear data library. Daejeon: KAERI; 2000. KAERI/TR-1512/2000.
- Kishida N, Murata T, Asami T, et al. JENDL Photonuclear Data File. AIP Conf. Proc. 2005;769(1):199–202.
- Koning AJ, Rochman D, Sublet JC, et al. TENDL: complete nuclear data library for innovative nuclear science and technology. Nucl. Data Sheets. 2019;155: 1–55.
- Brown DA, Chadwick MB, Capote R, et al. ENDF/B-VIII.0: the 8th major release of the nuclear reaction data library with CIELO-project cross sections, new standards and thermal scattering data. Nucl. Data. Sheets. 2018;148:1–142.
- Kawano T, Cho YS, Dimitriou P, et al. IAEA Photonuclear data library 2019. Nucl. Data Sheets. 2020;163:109–162.
- Iwamoto N, Kosako K, Murata T Photonuclear Data File. In: Proceedings of the 2015 Symposium on Nuclear Data; November 19-20, 2015, Ibaraki Quantum Beam Research Center, Tokai-mura, Ibaraki, Japan; 2016. p. 53–58. JAEA-Conf 2016-004.
- Member of the Cross Sections Evaluation Working Group. ENDF-6 Formats manual, data formats and procedures for the evaluated nuclear data files ENDF/B-VI, ENDF/B-VII and ENDF/B-VIII. Brookhaven National Laboratory; 2018. BNL-203218- 2018-INRE.
- Iwamoto O, Iwamoto N, Kunieda S, et al. The CCONE Code system and its application to nuclear data evaluation for fission and other reactions. Nucl. Data Sheets. 2016;131:259–288.
- Fukahori T ALICE-F Calculation of nuclear data up to 1 GeV. In: Proceedings of the Specialists Meeting on High Energy Nuclear Data; October 3-4, 1991, JAERI, Tokai; 1992. p. 114–122. JAERI-M 92-039.
- Murata T, Kosako K, Fukahori T Renewal of JENDL photonuclear data file 2004 (I) Elements of atomic number below 20. In: Proceedings of the 2010 Symposium on Nuclear Data; November 25-26, 2010, C-CUBE, Chikushi Campus, Kyushu University, Kasuga, Japan; 2011. p. 235–240. JAEA-Conf 2011-002.
- Levinger JS. The high energy nuclear photoeffect. Phys. Rev. 1951 Oct;84:43–51.
- Chadwick MB, Obložinský P, Hodgson PE, et al. Pauli-blocking in the quasideuteron model of photoabsorption. Phys. Rev. C. 1991;44:814–823.
- Fuller EG, Hayward E. The Giant resonance of the nuclear photoeffect. In: Endt PM, and PB Smith, editors. Nuclear Reactions Volume II. Chapter III. Amsterdam: North- Holland; 1962. pp. 113–194.
- IAEA. Evaluated Nuclear Structure Data File; 2010. Available from: https://www.nndc.bnl.gov/ensdf/.
- IAEA. Handbook for calculations of nuclear reaction data, RIPL-2. Vienna, Austria: IAEA; 2006. IAEA- TECDOC-1506.
- de Shalit A, Feshbach H. Theoretical nuclear physics, Volume 1, Nuclear structure. New York: Wiley; 1974.
- Capote R, Herman M, Obložinský P, et al. RIPL - Reference input parameter library for calculation of nuclear reactions and nuclear data evaluations. Nucl. Data Sheets. 2009;110(12):3107–3214.
- Gilbert A, Cameron AGW. A composite nuclear-level density formula with shell corrections. Can. J. Phys. 1965;43(8):1446–1496.
- Mengoni A, Nakajima Y. Fermi-gas model parametrization of nuclear level density. J. Nucl. Sci. Technol. 1994;31(2):151–162.
- Koning AJ, Delaroche JP. Local and global nucleon optical models from 1 keV to 200 MeV. Nucl. Phys. A. 2003;713(3):231–310.
- Han Y, Shi Y, Shen Q. Deuteron global optical model potential for energies up to 200 MeV. Phys. Rev. C. 2006;74:044615.
- Xu Y, Guo H, Han Y, et al. Helium-3 global optical model potential with energies below 250 MeV. Sci. China: Phys. Mech. Astronomy. 2011;54(11):2005.
- Avrigeanu M, Avrigeanu V. α-particle nuclear surface absorption below the Coulomb barrier in heavy nuclei. Phys. Rev. C. 2010;82:014606.
- Plujko VA, Ezhov SN, Kavatsyuk MO, et al. Testing and improvements of gamma-ray strength functions for nuclear model calculations. J. Nucl. Sci. Technol. 2002;39(sup2):811–814.
- Kopecky J, Uhl M. Test of gamma-ray strength functions in nuclear reaction model calculations. Phys. Rev. C. 1990;41:1941–1955.
- Kalbach C. Two-component exciton model: basic formalism away from shell closures. Phys. Rev. C. 1986;33:818–833.
- Koning AJ, Duijvestijn MC. A global pre-equilibrium analysis from 7 to 200 MeV based on the optical model potential. Nucl. Phys. A. 2004;744:15.
- Shibata K, Iwamoto O, Nakagawa T, et al. JENDL-4.0: a New library for nuclear science and engineering. J. Nucl. Sci. Technol. 2011;48(1):1–30.
- Caldwell JT, Dowdy EJ, Alvarez RA, et al. Experimental Determination of photofission neutron multiplicities for 235U, 236U, 238U, and 232Th using monoenergetic photons. Nucl. Sci. Eng. 1980;73(2):153–163.
- Berman BL, Caldwell JT, Dowdy EJ, et al. Photofission and photoneutron cross sections and photofission neutron multiplicities for 233U, 234U, 237Np, and 239Pu. Phys. Rev. C. 1986 Dec;34:2201–2214.
- Wahl AC. Systematics of Fission-Product Yields. Los Alamos, NM: Los Alamos National Laboratory; 2002. LA-13928.
- Ethvignot T, Devlin M, Duarte H, et al. Neutron multiplicity in the fission of 238U and 235U with neutrons up to 200 MeV. Phys. Rev. Lett. 2005 Feb;94:052701.
- Weisskopf VF, Ewing DH. On the yield of nuclear reactions with heavy elements. Phys. Rev. 1940 Mar;57:472–485.
- Kalbach C. Systematics of continuum angular distributions: extensions to higher energies. Phys. Rev. C. 1988 Jun;37:2350–2370.
- Wapstra AH, Audi G. The 1983 atomic mass evaluation (I). Atomic mass table. Nucl. Phys. A. 1985;432:1–54.
- Fukahori T. Historical overview of nuclear data evaluation in the intermediate energy region. AIP Conf. Proc. 2005;769(1):47–52.
- Otuka N, Dupont E, Semkova V, et al. Towards a more complete and accurate experimental nuclear reaction data library (EXFOR): International collaboration between Nuclear Reaction Data Centres (NRDC). Nucl. Data Sheets. 2014;120:272–276.
- Berman BL, Pywell RE, Dietrich SS, et al. Absolute photoneutron cross sections for Zr, I, Pr, Au, and Pb. Phys. Rev. C. 1987 Oct;36:1286–1292.
- Carlos P, Beil H, Bergère R, et al. A study of the photoneutron contribution to the giant dipole resonance of nuclei in the 64 ≤ A ≤ 86 mass region. Nucl. Phys. A. 1976;258(2):365–387.
- Lepretre A, Beil H, Bergere R, et al. The giant dipole states in the A = 90 mass region. Nucl. Phys. A. 1971;175(3):609–628.
- Berman BL, Bramblett RL, Caldwell JT, et al. Photoneutron Cross Sections for As75, Ag107, and Cs133. Phys. Rev. 1969 Jan;177:1745–1754.
- Leprêtre A, Beil H, Bergère R, et al. A study of the giant dipole resonance of vibrational nuclei in the 103 ≤ A ≤ 133 mass region. Nucl. Phys. A. 1974;219(1):39–60.
- Bergère R, Beil H, Carlos P, et al. Sections efficaces photoneutroniques de I, Ce, Sm, Er et Lu. Nucl. Phys. A. 1969;133(2):417–437.
- Bramblett RL, Caldwell JT, Berman BL, et al. Photoneutron Cross Sections of Pr141 and I127 from Threshold to 33 MeV. Phys. Rev. 1966 Aug;148:1198–1205.
- Carlos P, Beil H, Bergère R, et al. The giant dipole resonance in the transition region of the samarium isotopes. Nucl. Phys. A. 1974;225(1):171–188.
- Bramblett RL, Caldwell JT, Harvey RR, et al. Photoneutron Cross Sections of Tb159 and O16. Phys. Rev. 1964 Feb;133:B869–B873.
- Bramblett RL, Caldwell JT, Auchampaugh GF, et al. Photoneutron Cross Sections of Ta181 and Ho165. Phys. Rev. 1963 Mar;129:2723–2729.
- Veyssiere A, Beil H, Bergere R, et al. Photoneutron cross sections of 208Pb and 197Au. Nucl. Phys. A. 1970;159(2):561–576.
- Harvey RR, Caldwell JT, Bramblett RL, et al. Photoneutron Cross Sections of Pb206, Pb207, Pb208, and Bi209. Phys. Rev. 1964 Oct;136:B126–B131.
- Veyssière A, Beil H, Bergère R, et al. A study of the photofission and photoneutron processes in the giant dipole resonance of 232Th, 238U and 237Np. Nucl. Phys. A. 1973;199(1):45–64.
- Goryachev BI, Ishkhanov BS, Shevchenko VG, et al. Structure of (γ,n) Cross Sections in Si28, S32, and Ca40. Yadernaya Fizika. 1968;7:1168.
- Webb DV, Muirhead EG, Spicer BM. The photoneutron cross sections of 28Si and 24Mg. Nucl. Phys. A. 1970;159(1):81–96.
- Pywell RE, Berman BL, Jury JW, et al. Photoneutron cross sections for the silicon isotopes. Phys. Rev. C. 1983 Mar;27:960–975.
- Caldwell JT, Harvey RR, Bramblett RL, et al. (γ,n) cross sections for O16 and Si28. Phys. Letters. 1963;6(2):213–215.
- Kosako K, Murata T, Iwamoto N Status of New JENDL Photonuclear Data File. In: Hayakawa T, M Senzaki, and P Bolton et al., editors Proceedins of the Internatinal Symposium Nuclear Physics and Gamma-Ray Sources for Nuclear Security and Nonproliferation Tokai-mura, Japan. World Scientific; 2015. p. 261–268.
- Costa S, Ferroni F, Ferroni S, et al. Photoneutrons from medium elements up to 80 MeV photon energy. Phys. Letters. 1964;11(4):324–326.
- Ishkhanov BS, Kapitonov IM, Lazutin EV, et al. Photoneutron reaction cross section of 55Mn in the giant dipole resonance region. Bull. Russ. Acad. Sci. Phys. 1971;34:1988–1991.
- Alvarez RA, Berman BL, Faul DD, et al. Photoneutron cross sections for 55Mn and 59Co. Phys. Rev. C. 1979 Jul;20:128–138.
- Kosako K, Oishi K, Nakamura T, et al. Angular distribution of photoneutrons from copper and tungsten targets bombarded by 18, 28, and 38 MeV electrons. J. Nucl. Sci. Technol. 2011;48(2):227–236.
- Kosako K, Nakamura T. Comparison of photonuclear data files used in shielding calculation of a medical linac room. Nihon Genshiryoku Gakkai wabun rombunshi. 2021;20(1):23–33.
- X-5 Monte Carlo Team. MCNP-A General Monte Carlo N-particle Transport Code, Version 5. Los Alamos, NM: Los Alamos National Laboratory; 2003.
- Fultz SC, Bramblett RL, Caldwell JT, et al. Photoneutron cross sections for natural Cu, Cu63, and Cu65. Phys. Rev. 1964 Mar;133:B1149–B1154.
- Antonov AD, Balabanov NP, Gangrsky YP, et al. Studies of photonuclear reactions with emission of alpha particles in the region of the giant dipole resonance. Yadernaya Fizika. 1990;51:305.
- Katz L, Cameron AGW. The Solution of X-ray activation curves for photonuclear cross sections. Can. J. Phys. 1951;29(6):518–544.
- Veyssière A, Beil H, Bergère R, et al. Étude de la résonance géante dipolaire dans la région de transition autour de A = 190. J. Phys. Lett. 1975;36(11):267–270.
- Vogt K, Mohr P, Babilon M, et al. Measurement of the (γ,n) cross section of the nucleus 197Au close above the reaction threshold. Nucl. Phys. A. 2002;707(1):241–252.
- Hara KY, Harada H, Kitatani F, et al. Measurements of the 152Sm(γ,n) cross section with laser-Compton scattering γ rays and the photon difference method. J. Nucl. Sci. Technol. 2007;44(7):938–945.
- Kitatani F, Harada H, Goko S, et al. Measurement of the 80Se(γ,n) cross section using laser-Compton scattering γ-rays. J. Nucl. Sci. Technol. 2010;47(4):367–375.
- Kitatani F, Harada H, Goko S, et al. Measurement of 76Se and 78Se(γ,n) cross sections. J. Nucl. Sci. Technol. 2011;48(7):1017–1024.
- Itoh O, Utsunomiya H, Akimune H, et al. Photoneutron cross sections for Au revisited: measurements with laser Compton scattering γ-rays and data reduction by a least-squares method. J. Nucl. Sci. Technol. 2011;48(5):834–840.
- Plaisir C, Hannachi F, Gobet F, et al. Measurement of the 85Rb(γ,n)84mRb cross-section in the energy range 10-19 MeV with bremsstrahlung photons. Eur. Phys. J. A. 2012;48:68.
- Zatsepina GN, Igonin VV, Lazareva LE, et al. Direct photoeffect on heavy nuclei at small excitation energy. Low and medium energy nuclear reactions, Moscow: 1960. p. 479.
- Lepestkin AI, Sidorov VI. Nonequilibrium photoneutrons and statistical characteristics of Heavy Nuclei from photonuclear experiments. Izvestiya Rossiiskoi Akademii Nauk, Seriya Fizicheskaya. 1984;48:355.
- Khan AM, Knowles JW. Photofission of 232Th, 238U and 235U near threshold using a variable energy beam of γ-rays. Nucl. Phys. A. 1972;179(2):333–352.
- Silano JA, Karwowski HJ. Near-barrier photofission in 232Th and 238U. Phys. Rev. C. 2018 Nov;98:054609.
- Caldwell JT, Dowdy EJ, Berman BL, et al. Giant resonance for the actinide nuclei: photoneutron and photofission cross sections for 235U, 236U, 238U, and 232Th. Phys. Rev. C. 1980 Apr;21:1215–1231.
- Ries H, Mank G, Drexler J, et al. Absolute photofission cross sections for 235,238U in the energy range 11.5-30 MeV. Phys. Rev. C. 1984 Jun;29:2346–2348.
- Ries H, Kneissl U, Mank G, et al. Absolute photofission cross sections of 235,238U measured with tagged photons between 40 and 105 MeV. Phys. Lett. B. 1984;139(4):254–258.
- Leprêtre A, Bergère R, Bourgeois P, et al. Absolute photofission cross sections for 232Th and 235,238U measured with monochromatic tagged photons (20 MeV<Eγ<110 MeV). Nucl. Phys. A. 1987;472(3):533–557.
- Martins JB, Moreira EL, Tavares OAP, et al. Absolute photofission cross section of 197Au, natPb, 209Bi, 232Th, 238U, and 235U nuclei by 69-MeV monochromatic and polarized photons. Phys. Rev. C. 1991 Jul;44:354–364.
- Tavares OAP, Terranova ML, Casano L, et al. Fission of complex nuclei induced by 52-MeV monochromatic and polarized photons. Phys. Rev. C. 1991 Oct;44:1683–1686.
- Soldatov AS, Smirenkin GN. Yield and cross section for fission of odd nuclei by γ rays with energies up to 11 MeV. Yadernaya Fizika. 1992;55:3153.
- Frommhold T, Steiper F, Henkel W, et al. Photofission of 235U and 238U at intermediate energies: absolute cross sections and fragment mass distributions. Zeitschrift für Physik A Hadrons Nuclei. 1994;350(3):249–261.
- Cetina C, Heimberg P, Berman BL, et al. Photofission of heavy nuclei from 0.2 to 3.8 GeV. Phys. Rev. C. 2002 Apr;65:044622.
- Sanabria JC, Berman BL, Cetina C, et al. Photofission of actinide nuclei in the quasideuteron and lower part of the ∆ energy region. Phys. Rev. C. 2000 Feb;61:034604.