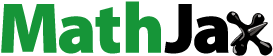
ABSTRACT
In grazed pasture-based systems, urinary-nitrogen (N) voided in concentrated patches is a key source of nitrous oxide (N2O), a potent greenhouse gas (GHG). Development of strategies for reducing emissions from urine patches is an ongoing focus of international research. Plants can help mitigate N2O emissions by reducing urinary-N excretion or by lowering the N2O emission factor of urine. This paper reviews the current understanding of plant-effects on urinary-N2O emissions and confirms that plants can influence emissions. Recent New Zealand studies suggest that plantain and fodder beet show particular potential for grazed systems. A key mechanism by which plantain affects the N2O emission factor could be root exudates that inhibit nitrification and/or increase N immobilisation. Further studies are needed to elucidate the mechanisms involved, and to help maximise the GHG reduction potential from plant management options in pasture-based systems.
Introduction
In grazed pasture-based systems, animals often consume more nitrogen (N) than they need for animal growth and production. Consequently, a high percentage of the extra N consumed is voided in the animals’ urine resulting in small areas of pasture soil containing large amounts of urinary-N (e.g. Whitehead Citation1995; Selbie et al. Citation2015). These animal urine patches are the key sources of nitrous oxide (N2O) emissions in grazed pastoral systems (e.g. de Klein and Ledgard Citation2005; Krol et al. Citation2016; Chadwick et al. Citation2018). N2O is a potent greenhouse gas (GHG) that is produced by the biological soil processes of nitrification and denitrification (Baggs and Philippot Citation2010) and the development of mitigation strategies for reducing N2O emissions from urine patches is an ongoing focus of international research (e.g. van Groenigen et al. Citation2006; de Klein and Eckard Citation2008; Luo et al. Citation2008; Liu and Zhou Citation2014; Marsden et al. Citation2017; Di and Cameron Citation2018).
Strategies to mitigate N2O can be broadly categorised as those that reduce the total amount of urinary N excreted by animals and those that manipulate soil processes through reduced nitrification or denitrification rates. Plant species have the potential to affect both types of mitigation strategies. More specifically, the N content of plant species can affect the total amount of N consumed by the animal and thus the amount of urinary N excreted (Dijkstra et al. Citation2013). In addition, the amount of urinary N excreted can be affected by plant chemical components (such as tannins) that increase the partitioning of excreta N into dung relative to urine (e.g. Carulla et al. Citation2005). Furthermore, plants can also affect N2O emissions by influencing nitrification and denitrification rates in soil, e.g. through competition for N or water, oxygen consumption, root exudates, changes in pH and carbon (C) availability (Abalos et al. Citation2014).
In the past decade, a significant amount of work has been conducted to assess the impact of different plant species on urinary N output and N2O emissions (e.g. Maljanen et al. Citation2004; Abalos et al. Citation2014; Di et al. Citation2016; Krol et al. Citation2016; Bowatte et al. Citation2018; Chadwick et al. Citation2018; Luo et al. Citation2018). Some studies have shown that simply the presence of plants can influence N2O emissions from soils. For example, Maljanen et al. (Citation2004) found that annual N2O emissions from organic soils sown in grass and barley were only 12%–36% of those measured from bare organic soil in Finland. Similarly, Bowatte et al. (Citation2018) found that bare soil receiving urine produced the largest N2O emissions (5 kg N2O-N ha−1 over a 6-week measurement period) compared to urine applied to plots supporting plants (0.7–3.2 kg N2O-N ha−1 over a 6-week period). Some studies found that N2O emissions from different plant species differed due to the effect of the plants on the N content in the urine (e.g. Di et al. Citation2016), while other studies have suggested that N2O emissions can be reduced due to the effects of the plants themselves (e.g. Abalos et al. Citation2014; Luo et al. Citation2018). The results to-date on the impacts of plants on N2O emissions and on the potential underlying mechanisms are not always conclusive. This paper therefore reviews the current understanding of the impact of plant species on N2O emissions from urine patches in grazed pastoral systems to identify hypotheses for the potential mechanisms underlying the key findings. The review distinguishes between the two broad categories of plant effects on N2O emissions from predominantly grazed pastoral systems: plants that alter total urinary N excretion (i.e. reduce total urinary output); and plants that modify the N2O emission factor (i.e. reduce the proportion of the urinary N that is emitted as N2O) through influencing soil nitrification and denitrification rates.
Plants that alter total urinary N excretion
The effect of animal N intake on total urinary N output
Several studies have shown a strong positive relationship between animal N intake and the amount of N excreted (e.g. Castillo et al. Citation2000; Kebreab et al. Citation2001; Mulligan et al. Citation2004; Huhtanen et al. Citation2008). As a result, reducing the amount of N consumed in the diet is an effective way of reducing N2O emissions from excreta, provided dietary N, crude protein (CP) levels and energy content (e.g. sugars and starch (SS)) remain sufficient to meet the animal’s metabolic requirements. For example, recent research by Dalley et al. (Citation2017) suggested that feeds most likely to result in lower urinary-N excretion have plant characteristics with <12% CP and >50% SS. However, lactating cows require higher dietary CP contents of 16%–20% which highlights the importance of selecting feeds that meet animal metabolic requirements (de Klein and Eckard Citation2008; Dalley et al. Citation2017).
The use of low N supplements such as maize, grain, kale, fodder beet, swedes or low N citrus pulp can reduce the N content in the diet and thus reduce total and urinary-N excretion by ruminants (e.g. Kebreab et al. Citation2001; Nielsen et al. Citation2003; Mulligan et al. Citation2004; Misselbrook et al. Citation2005; Steinshamn et al. Citation2006; Burke et al. Citation2008; Huhtanen et al. Citation2008; Luo et al. Citation2008; van der Weerden and Styles Citation2012; Edwards et al. Citation2014). However, reducing the N content in the diet in pasture-based systems is often challenging. This is largely because high-producing pasture species have herbage N concentrations that are generally in excess of animal protein requirements (i.e. > ca 3% N or 19% protein). In addition, reducing the amount of dietary N by using low-N supplements is often impractical, potentially costly and the production of these supplements can lead to additional N2O emissions.
Recent New Zealand studies that spot-sampled urinary N from animals grazing plantain (Plantago lanceolata L.), or mixed pastures that included about 20% plantain, showed a reduction of the N concentration in the urine (e.g. Totty et al. Citation2013; Box et al. Citation2016; Cheng, Judson, et al. Citation2017; Cheng, McCormick, et al. Citation2017), and these authors suggested that the total amount of urinary N excreted could also be reduced. However, in published studies where total N excretion was measured using animals in metabolism stalls or with urine sensors, no reduction in total N excretion was found for animals on a diet containing 15%–20% plantain dry matter (DM) (Bryant et al. Citation2018; Waghorn et al. Citation2018). The lack of a plantain effect on total N excretion in these studies may be due to the proportion of plantain in the diets used. A recent metabolism stall study using cows on diets with increasing proportions of plantain showed that total urinary N excretion was significantly reduced only when the proportion of plantain exceeded 30% of the diet DM (E. Minnee pers. comm.). In this study, total N intake by the animals was similar for the different diets, while urine volume increased with increasing proportion of plantain in the diet. Similarly, O’Connell et al. (Citation2016) found that sheep on a plantain diet had consistently higher urine volumes than sheep fed a ryegrass diet. As feed intake and feed water content were similar between the two diets, these authors suggested that plantain could cause diuresis. This diuretic effect of plantain could be a possible explanation why some of New Zealand studies mentioned above showed that animals on plantain had lower urine N concentrations than animals grazing ryegrass pastures.
(ii) The effect of increasing N partitioning into dung on total urinary N output
Partitioning more of the excreted N into dung relative to urine will reduce N2O emissions from pastures, as the emission factor for dung is lower than that for urine (Luo et al. Citation2009). For its national GHG inventory calculations, New Zealand has adopted country-specific N2O emissions factors of dung and urine of 0.25% and 1% of the excreted N emitted as N2O, respectively (Ministry for the Environment Citation2018). As the proportion of N excreted in urine decreases with total N intake (Jarvis et al. Citation1995; Selbie et al. Citation2015), reducing the N content of the diet will not only reduce total N intake but also partition relatively more of the excreta N into dung.
Feeding animals condensed tannin (CT)-rich diets can also increase N partitioning into dung (Carulla et al. Citation2005). Several studies have shown that inclusion of CT either as a dietary supplement or in forages fed to ruminants reduced urinary-N excretion, increased the amount of N excreted in the dung and improved N retention in the animal (e.g. Carulla et al. Citation2005; Misselbrook et al. Citation2005). Condensed tannins have also been found in plantain (Ramírez-Restrepo and Barry Citation2005) and this could be a further explanation of the reduced urinary N excretion that was observed from cows on a plantain containing diet (E. Minnee pers. com.).
Plants that modify the N2O emission factor of urine
For this category of plants we distinguished between (i) plant effects on the N concentration in the urine, (ii) root exudates that affect soil N processes, (iii) secondary metabolites present in the urine, and (iv) morphological effects of shoots and roots.
Plant effects on the urinary N concentration and the N2O emission factor
Results on the effect of the N concentration in urine, and thus the N loading rate of urine patches, on the N2O emission factor are inconclusive (e.g. de Klein et al. Citation2014; Di et al. Citation2016; Marsden et al. Citation2016). In a study using seven urine N loading rates (ranging from 0 to 1200 kg N ha−1 equivalent), de Klein et al. (Citation2014) showed that loading rate had a positive effect on the N2O emission factor for simulated cow urine patches on free draining soils with relatively low emissions (emission factors between 0.03% and 0.3%). However, in the same study, the N2O emission factor was independent of the N application rate for a poorly draining soil with higher N2O emissions (emission factors between 0.5% and 0.9%). Marsden et al. (Citation2016) did not observe any urine N loading effects (200 vs 800 kg N ha−1 equivalent) on the N2O emissions factor from simulated sheep urine patches with relatively low N2O emission factors (0.1%–0.2%). In contrast, Di et al. (Citation2016) found that the N2O emission factors from cow urine patches at an N loading rate of 500 kg urine-N ha−1 equivalent resulted in a lower emission factor than an N loading rate of 700 kg urine-N ha−1 equivalent (0.7% vs 1.1%). Some studies that used N fertiliser rather than urine N have also found a lack of a significant effect of the N application rate on the N2O emission factor. This lack of N application rate effect was attributed to factors other than N availability determining the N2O emissions (e.g. dry conditions; Peng et al. Citation2011; and/or low available soil C; Rochette et al. Citation2010; competition for N between vegetation and soil microbes; Kim et al. Citation2013).
Plant species such as plantain that may exhibit diuretic properties have the potential to reduce the urinary-N loading in individual urine patches by increasing the urination frequency of grazing animals. Although the increased urination frequency results in greater coverage of urine-affected soil, total paddock-scale N2O emissions from urine patches are not likely to be higher if the total amount of urinary-N excreted remains the same. In fact, they could be lower if the N2O emission factor reduces with N loading rate. However, there could be increased N2O emissions from any indigenous soil N, due to the increased wetting effect of the increased urination frequency.
(ii) The effect of plant root exudates on the N2O emission factor
There is extensive evidence to show that plant tissues contain a wide variety of chemical compounds that are not involved in primary metabolism. These compounds, termed secondary metabolites, vary according to family and species and play a variety of roles in protecting plants from a range of stresses (e.g. predation and competition, Bennett and Wallsgrove Citation1994; Erickson et al. Citation2000) including suppression or inhibition of soil N transformation processes. Immobilisation of inorganic N forms or inhibition of soil N processes, such as nitrification, has the potential to reduce N2O emissions from animal excreta deposited on land (Di et al. Citation2010; de Klein et al. Citation2011; Cameron et al. Citation2014; Li et al. Citation2014). Abalos et al. (Citation2014) proposed that root exudates in different plant species affect the composition of nitrifying and denitrifying microbial communities, thus influencing N2O emissions.
The suppression of soil nitrification has been observed to occur naturally in some ecosystems where certain plant species release organic molecules from their roots that suppress the function and growth of nitrifying bacteria, a phenomenon termed biological nitrification inhibition (BNI) (Subbarao, Ito, et al. Citation2007; Subbarao, Rondon, et al. Citation2007, Citation2015). These authors detected and quantified biological nitrification inhibitors in root exudates released from plants, with the tropical grass species Brachiaria humidicola showing the highest BNI capacity in root systems: up to 90% decline in soil ammonium oxidation rates within three years of plant establishment. In a field study using tropical grasses with a wide range of BNI capacities, Subbarao et al. (Citation2013) found a negative relationship between the BNI capacity of a species and N2O emissions. Similarly, Byrnes et al. (Citation2017) showed that N2O emissions from urine patches deposited on plots of tropical forage grass with high BNI capacity (Brachiaria humidicola cv. Tully) were three times lower than from urine patches in plots of tropical forage grass with low BNI capacity (Brachiaria hybrid cv. Mulato).
It has been suggested that BNI has evolved as part of some plants’ adaptation mechanisms to conserve and use N efficiently in systems that are naturally limited in mineral N (Subbarao, Rondon, et al. Citation2007; Al-Ansari and Abdulkareem Citation2014) but it appears to be stimulated by high ammonium concentration in the soil (Subbarao et al. Citation2012, Citation2015). Plants that use nitrate as their primary N source did not release BNIs from roots, whereas BNIs were released from plants that use ammonium as their primary N source (Subbarao, Ito, et al. Citation2007; Zakir et al. Citation2008). Research has also found that BNI compounds isolated from plant root exudates of B. humidicola remained effective when added to the soil and incubated for up to 55 days at 20°C (Gopalakrishnan et al. Citation2009). It is expected that the longevity of BNI effectiveness would depend on individual BNI compounds and their concentrations in the soil.
Although early observations of BNI were mostly made on tropical grassland systems, BNI activity was also found in certain temperate forest ecosystems (Smolander et al. Citation2012). It is also likely that some temperate grasses or forbs have BNI capacity in their root systems (Coskun et al. Citation2017; Carlton et al. Citation2018). In a New Zealand study, Bowatte et al. (Citation2018) measured N2O emissions from urine patches receiving 530 kg N ha−1 equivalent in a monoculture experiment with 11 C3 grasses, three forbs and two legumes. Emissions ranged from 0.7 to 3.2 kg N2O-N ha−1 over a 6-week measurement period, with a median value of 1.5 kg N2O-N ha−1. These authors also found that there was a significant difference in soil mineral N among species in the first two weeks after urine application and that soil nitrification potential (SNP) under different species was positively and linearly related to N2O emissions. This would further suggest that plant-induced differences in N transformations in the soil and N transformation process inhibitors, including BNIs, in root exudates from temperate plant species may play an important role in regulating N2O production and emissions.
Luo et al. (Citation2018) found that N2O emissions from urine patches deposited in monoculture plots of plantain were on average 28% lower than from urine patches in monoculture plots of perennial ryegrass. As the plots received the same type and rate of urine, the observed difference in N2O emissions were solely plant-induced. The authors also observed differences in soil water content between the plantain and the ryegrass plots, which could have contributed to the observed difference in N2O emissions. However, they also found differences in N2O emissions between plantain and lucerne (Medicago sativa L.) monoculture plots that had very similar soil water contents. They therefore suggested that BNI was the dominant mechanisms by which plantain reduced N2O emissions. The BNI potential of plantain is further supported by Bowatte et al. (Citation2018) who found that plantain did not fit their observed positive relationship between SNP and N2O emissions as it had a high SNP, yet low N2O emissions. Similarly, a recent study, Carlton et al. (Citation2018) discovered that soil ammonia oxidiser bacteria (AOB) population abundance was lower in plantain soil compared to perennial ryegrass/white clover soil. This resulted in reduced nitrification rates and lower nitrate leaching losses under the plantain soil. Some literature suggests that most of the secondary metabolites produced by plantain are potential BNIs (Dietz et al. Citation2013). These metabolites include the iridoid glycosides aucubin and catalpol and the phenylpropanoid glycoside, acteoside (Gardiner et al. Citation2016; Navarrete et al. Citation2016). Soil incubation experiments with plantain leaf materials, plantain extract or aucubin confirmed that these materials significantly inhibited N mineralisation, nitrification or N2O emissions (Dietz et al. Citation2013; Gardiner et al. Citation2017).
It is also known that glucosinolates (GLSs) are an important group of bioactive phytochemicals and these compounds are naturally abundant in brassica crops (e.g. Kushad et al. Citation1999; Agneta et al. Citation2013). GLSs or their hydrolysis products (GLS-HPs) (e.g. Isothiocyanates, thiocyanates and nitriles) have been shown to inhibit N processes in soil (Bending and Lincoln Citation2000; Reardon et al. Citation2013). Results from Bowatte et al. (Citation2018) showed that N2O emissions from the forage rape (Brassica rapus ssp. Biennis (cv Titan) were lower compared to those from some grass species and legumes. As for plantain above, these authors also found that forage rape did not fit their observed positive relationship between SNP and N2O emissions as it also had a high SNP, yet low N2O emissions. It is therefore suggested that brassica crops that are adapted to temperate climate can produce BNIs in their plant tissues and subsequently release these into the soil. Balvert et al. (Citation2017) showed in a laboratory study that some GLS-HP compounds had an inhibitory effect on nitrification and reduced N2O emissions from urea applied to soil. However, in a field study, the application of GLS-HP compounds to artificial urine patches did not result in significant differences in N2O emissions (Balvert et al. Citation2017).
Root exudates can also affect the availability of soil mineral N, as C in these exudates may temporarily increase microbial immobilisation of N (e.g. Qian et al. Citation1997; Fisk et al. Citation2015). Carbon from root exudates may thus reduce N2O emissions derived from urine deposition due to immobilisation of urine-N. Indeed, a number of studies have demonstrated that excess N in urine patches does not immediately become available for nitrification and denitrification (Carran et al. Citation1982; Whitehead and Bristow Citation1990; Davidson et al. Citation1991; Williams and Haynes Citation1994; Bol et al. Citation2004). Instead, it can be immobilised into organic matter or fixed on clay particles (e.g. Carran et al. Citation1982; Williams and Haynes Citation1994). Davidson et al. (Citation1991) calculated that almost 50% of applied was likely to have been fixed within minutes of being applied. Bowatte et al. (Citation2018) observed that N uptake differed between plant species, but this difference could not explain observed differences in soil mineral N. These authors suggest that a possible explanation is that the degree of immobilisation of N is dependent on the quality and quantity of C inputs through root exudation from different species.
(iii) The effect of plant secondary metabolites in the urine on the N2O emission factor
A recent review suggested that forage plants are also able to affect N2O emissions through changing the animal urine composition after the plants have been consumed (Gardiner et al. Citation2016). Some secondary plant metabolites can be excreted in urine and dung which can subsequently affect soil N cycling processes and N2O emissions. Luo et al. (Citation2015) reported reduced N2O emissions from urine patches derived from animals fed a forage rape (B. napus subsp. oleifera L.) compared to urine from animals fed perennial ryegrass pasture. Although there were differences in the N concentration of the urine, the authors also reported reduced N2O emission factors which accounted for the differences in N loading. A possible explanation for this reduction is that the brassica-derived compounds GLS and GLS-HPs (see above; Balvert et al. Citation2017) were delivered to the soil in the urine (e.g. Ye et al. Citation2002; Munday et al. Citation2008) and inhibited nitrification in the urine patch. However, Hoogendoorn et al. (Citation2016) did not find reduced N2O emissions from the urine of animals consuming brassicas. The reasons for these different findings are unclear but may be related to soil types as the former field and laboratory studies were carried out on free-draining allophanic soil (Luo et al. Citation2015; Balvert et al. Citation2017) and the latter on poorly draining fluvial soil (Hoogendoorn et al. Citation2016). There is an array of potential GLS and GLS hydrolysis compounds that could possibly be in animal urine and can be utilised as BNIs for reducing N2O production in agricultural systems. In New Zealand systems, forage crops that are known to have BNI potential include: leafy turnips, bulb turnips, swedes, kale, forage rape and plantain (de Ruiter et al. Citation2009). However, there is little research that has examined the potential for these feeds and the subsequent effect of the voided urine to reduce soil nitrification and N2O emissions.
Di et al. (Citation2016) reported 29% lower N2O emission factors for urine deposited on fodder beet soil compared to urine deposited on kale soil. In their study they applied fodder beet-specific urine to fodder beet and kale-specific urine to kale. Both urine types had a similar N concentration (ca. 3 g N/L) and the lower emission factors from fodder beet urine could therefore not be explained by a reduced urine N loading rate. Alternative explanations could be differences in urine composition (e.g. secondary metabolites) or differences in plant or root morphology effects on the soil microclimate.
(iv) Plant morphological effects on the N2O emission factor
Plant morphological factors that can affect soil N cycling and N2O emissions include the effect of the root system on a plant’s ability to access water and nutrients, and plant canopy-effects on the dispersion of urine voided by grazing animals.
When roots are present, root morphology can affect soil structure and hydrology (Gregory Citation2006), both of which influence conditions that govern the reduction of N2O to N2 and diffusion of gases to the soil surface (Chapuis-lardy et al. Citation2007). However, there is little evidence to support plant species indirectly influencing N2O emissions by altering soil moisture content. Dijkstra et al. (Citation2010) observed a significant albeit weak positive relationship between soil moisture content and N2O emissions from soils supporting five different semi-arid grassland species. However, the species composition effect on N2O emissions could not be explained by species composition effects on soil moisture. Root morphology can also affect plant N uptake and thus availability for soil nitrification and denitrification processes (Abalos et al. Citation2014). These authors found that combining two grasses, perennial ryegrass (L. perenne) and rough stalked meadow grass (Poa trivialis), which is a high fertility responsive grass similar to perennial ryegrass (Lambert et al. Citation1986), produced the greatest amount of biomass and also the lowest N2O emissions. They suggested this was due to the complementarity of the root foraging strategies of these two species, where P trivialis may access N hotspots not previously emptied by L. perenne. This combination, together with its very high total root biomass, is thought to increase mineral N uptake, thereby lowering soil nitrate content and subsequent N2O emissions (Abalos et al. Citation2014). Plant species diversity and interactions can also influence N uptake and N2O emissions (Niklaus et al. Citation2016). Increasing plant species richness from 1 to 16 grassland species has been found to reduce N2O emissions in the absence of N fertiliser (Niklaus et al. Citation2016), due to more efficient soil inorganic N uptake. However, this pattern was not observed when the diversity included a large proportion of legumes (Niklaus et al. Citation2016).
Although pasture species with increasing root mass or rooting depth have greater ability to take up N, the winter-activity of pasture species such as Italian ryegrass (Lolium multiflorum Lam.) – i.e. the ability of roots to take up N under cooler conditions – appeared to be more important than specific root architecture (e.g. deep roots) for reducing N leaching losses (Moir et al. Citation2013; Malcolm et al. Citation2014, Citation2015; Woods et al. Citation2016). Woods et al. (Citation2016) found that winter-active Italian ryegrass had the greatest N uptake and lowest N leaching, whereas the opposite was found for the tap-rooted lucerne. The high N leaching losses from lucerne in this study were attributed to poor winter herbage growth and the limited depth of the lysimeters (0.7 m) used for this deep rooting species. Di et al. (Citation2016) and Smith et al. (L.C. Smith pers. comm.) studied the effect of plant winter-activity on N2O emissions from urine patches. Neither study found an effect of Italian ryegrass vs. perennial ryegrass when urine was applied at the same rate, either in autumn or spring. This suggests that any increased N uptake over the cooler autumn/winter season did not influence N2O emissions from urine patches. Nevertheless, reduced N leaching due to increased N uptake by Italian ryegrass pasture will result in a reduction in indirect N2O emissions from water receiving environments such as rivers and estuaries (Ministry for the Environment Citation2018). However, it is unclear whether the increased N uptake may lead to increased N excretion by grazing livestock, ultimately leading to greater direct N2O emissions compared to pasture species where N leaching is greater.
Plant roots may also alter other soil conditions such as soil pH, particularly in the rhizosphere. Legumes generally have a greater effect on rhizosphere pH compared to non-legumes (Maltais-Landry Citation2015; Wang et al. Citation2016). Wang et al. (Citation2016) examined pH changes in the rhizosphere of chickpeas, field peas, wheat and white lupin and observed that only chickpeas acidified its rhizosphere. It was thought that the difference was due to chickpea’s apparent excess uptake of cations over anions during N2 fixation. In contrast, Li et al. (Citation2011) studied wheat and peas, and showed that pH in rhizospheric soil was approximately one unit lower than that in the bulk soil for both plant species. Bowatte et al. (Citation2018) observed a weak relationship (R2 = 0.20) between the bulk soil pH and total N2O emissions from 16 different legume and non-legume pasture species, although the relationship was not influenced by whether a plant species was a legume or not. While legumes generally have a greater effect on rhizosphere pH compared to non-legumes (Maltais-Landry Citation2015), they generally inhabit a smaller soil volume than plant species with extensive adventitious root systems. Therefore, it could be argued that rooting systems of legumes have a limited impact on the pH of the bulk soil, thereby restricting its effect on N2O producing processes.
The size and shape of plant canopies may influence the amount of urine being intercepted by plants, thereby affecting the amount reaching the soil surface (Bowatte et al. Citation2018), or affect the volume and wetted area of urine reaching the soil surface. Research with wheat and pasture plants has shown that plant uptake of foliar-applied liquid urea does occur (Powlson et al. Citation1989; Dawar et al. Citation2012). However, in these studies N application rates were restricted to between 20 and 40 kg N/ha per application; in the case of wheat this was to avoid the risk of foliage burn. Urine deposition by sheep and cattle results in greater N loads, equivalent to between 300 and 1000 kg N/ha (Selbie et al. Citation2015). If it is assumed that the potential uptake by pasture foliage is a maximum of 40 kg N/ha, it is unlikely that plant interception of urine would have a significant effect on urine-derived N2O emissions from the soil surface. Increased dispersion of urine will reduce the N load per soil surface area, thereby increasing the degree of urine derived N by plant roots. For instance, a plant with large, near horizontal leaf structure may act as a ‘splashplate’, spreading urine over a larger soil area compared to an upright narrow-leafed grass species. However, there are currently no published studies that have investigated this.
Conclusions
This review highlights the potential of plant species to reduce N2O emissions. This can either be achieved through reducing the total amount of urinary N or by reducing the N2O emission factor. Plant species that reduce the N concentration of urine but not the total amount of urine N, may not reduce total N2O emissions as the results on the effect of urinary N-loading rate on the N2O emission factor are inconclusive. Recent New Zealand studies suggest that plantain and fodder beet show particular potential for reducing N2O emissions from grazed systems.
None of the existing studies has provided conclusive evidence of the mechanisms of plants to reduce N2O emissions. However, based on the evidence to-date, we suggest that a key mechanism by which plants such as plantain affect N2O emissions is through root exudates that inhibit nitrification and/or increase available C and thus N immobilisation. Further laboratory, field and modelling studies are needed to elucidate the mechanisms involved and to help assess and maximise the GHG reduction potential from plant management options in pasture-based systems.
Acknowledgements
This work was funded by the New Zealand Agricultural Greenhouse Gas Research Centre. We thank Karen Cousins and Wayne Worth (AgResearch) for sourcing and collating the international literature, and Stewart Ledgard and Brendon Welten (AgResearch) for valuable comments on earlier versions of this paper.
Disclosure statement
No potential conflict of interest was reported by the authors.
ORCID
Cecile A. M. de Klein http://orcid.org/0000-0001-7840-0934
Tony J. van der Weerden http://orcid.org/0000-0002-6999-2584
Jiafa Luo http://orcid.org/0000-0001-6198-6887
Keith C. Cameron http://orcid.org/0000-0002-7631-1636
Additional information
Funding
References
- Abalos D, De Deyn GB, Kuyper TW, van Groenigen JW. 2014. Plant species identity surpasses species richness as a key driver of N2O emissions from grassland. Global Change Biology. 20:265–275. doi: 10.1111/gcb.12350
- Agneta R, Möllers C, Rivelli AR. 2013. Horseradish (Armoracia rusticana), a neglected medical and condiment species with a relevant glucosinolate profile: a review. Genetic Resources and Crop Evolution. 60:1923–1943. doi: 10.1007/s10722-013-0010-4
- Al-Ansari AMS, Abdulkareem MA. 2014. Some plant extracts retarde nitrification in soil. Acta Agriculturae Slovenica. 103:5–13. doi: 10.14720/aas.2014.103.1.01
- Baggs E, Philippot L. 2010. Microbial terrestrial pathways to nitrous oxide. In: Smith KA, editor. Nitrous oxide and climate change. London: Earthscan; p. 4–35.
- Balvert SF, Luo J, Schipper LA. 2017. Do glucosinolate hydrolysis products reduce nitrous oxide emissions from urine affected soil? Science of the Total Environment. 603–604:370–380. doi: 10.1016/j.scitotenv.2017.06.089
- Bending GD, Lincoln SD. 2000. Inhibition of soil nitrifying bacteria communities and their activities by glucosinolate hydrolysis products. Soil Biology and Biochemistry. 32:1261–1269. doi: 10.1016/S0038-0717(00)00043-2
- Bennett RN, Wallsgrove RM. 1994. Secondary metabolites in plant defence mechanisms. New Phytologist. 127:617–633. doi: 10.1111/j.1469-8137.1994.tb02968.x
- Bol R, Petersen S, Christofides C, Dittert K, Hansen MN. 2004. Short-term N2O, CO2, NH3 fluxes, and N/C transfers in a Danish grass-clover pasture after simulated urine deposition in autumn. Journal of Plant Nutrition and Soil Science. 167:568–576. doi: 10.1002/jpln.200321334
- Bowatte S, Hoogendoorn CJ, Newton PCD, Liu Y, Brock SC, Theobald PW. 2018. Grassland plant species and cultivar effects on nitrous oxide emissions after urine application. Geoderma. 323:74–82. doi: 10.1016/j.geoderma.2018.03.001
- Box LA, Edwards GR, Bryant RH. 2016. Milk production and urinary nitrogen excretion of dairy cows grazing perennial ryegrass-white clover and pure plantain pastures. Proceedings of the New Zealand Society of Animal Production. 76:18–21.
- Bryant RH, Welten BG, Costall D, Shorten PR, Edwards GR. 2018. Milk yield and urinary-nitrogen excretion of dairy cows grazing forb pasture mixtures designed to reduce nitrate leaching. Livestock Science. 209:46–53. doi: 10.1016/j.livsci.2018.01.009
- Burke F, O’Donovan MA, Murphy JJ, O’Mara FP, Mulligan FJ. 2008. Effect of pasture allowance and supplementation with maize silage and concentrates differing in crude protein concentration on milk production and nitrogen excretion by dairy cows. Livestock Science. 114:325–335. doi: 10.1016/j.livsci.2007.05.019
- Byrnes RC, Nùñez J, Arenas L, Rao I, Trujillo C, Alvarez C, Arango J, Rasche F, Chirinda N. 2017. Biological nitrification inhibition by Brachiaria grasses mitigates soil nitrous oxide emissions from bovine urine patches. Soil Biology and Biochemistry. 107:156–163. doi: 10.1016/j.soilbio.2016.12.029
- Cameron KC, Di HJ, Moir JL. 2014. Dicyandiamide (DCD) effect on nitrous oxide emissions, nitrate leaching and pasture yield in Canterbury, New Zealand. New Zealand Journal of Agricultural Research. 57:251–270. doi: 10.1080/00288233.2013.797914
- Carlton AJ, Cameron KC, Di HJ, Clough TJ, Edwards GR. 2018. Nitrate leaching losses are lower from diverse forages containing plantain than from standard forages under different irrigation. New Zealand Journal of Agricultural Research. doi:10.1080/00288233.2018.1461659.
- Carran RA, Ball P, Theobald PW, Collins MEG. 1982. Soil nitrogen balances in urine-affected areas under two moisture regimes in southland. New Zealand Journal of Experimental Agriculture. 10:377–381. doi: 10.1080/03015521.1982.10427902
- Carulla JE, Kreuzer M, Machmüller A, Hess HD. 2005. Supplementation of Acacia mearnsii tannins decreases methanogenesis and urinary nitrogen in forage-fed sheep. Australian Journal of Agricultural Research. 56:961–970. doi: 10.1071/AR05022
- Castillo AR, Kebreab E, Beever DE, France J. 2000. A review of efficiency of nitrogen utilisation in lactating dairy cows and its relationship to environmental pollution. Journal of Animal and Feed Sciences. 9:1–32. doi: 10.22358/jafs/68025/2000
- Chadwick DR, Cardenas LM, Dhanoa MS, Donovan N, Misselbrook T, Williams JR, Thorman RE, McGeough KL, Watson CJ, Bell M, et al. 2018. The contribution of cattle urine and dung to nitrous oxide emissions: quantification of country specific emission factors and implications for national inventories. Science of the Total Environment. 635:607–617. doi: 10.1016/j.scitotenv.2018.04.152
- Chapuis-lardy L, Wrage N, Metay A, Chotte JL, Bernoux M. 2007. Soils, a sink for N2O? A review. Global Change Biology. 13:1–17. doi: 10.1111/j.1365-2486.2006.01280.x
- Cheng L, Judson HG, Bryant RH, Mowat H, Guinot L, Hague H, Taylor S, Edwards GR. 2017. The effect of feeding cut plantain and perennial ryegrass-white clover pasture on dairy heifer feed and water intake, apparent nutrient digestibility and nitrogen excretion in urine. Animal Feed Science and Technology. 229:43–46. doi: 10.1016/j.anifeedsci.2017.04.023
- Cheng L, McCormick J, Hussein AN, Logan C, Pacheco D, Hodge MC, Edwards GR. 2017. Live weight gain, urinary nitrogen excretion and urination behaviour of dairy heifers grazing pasture, chicory and plantain. The Journal of Agricultural Science. 155:669–678. doi: 10.1017/S0021859616001076
- Coskun D, Britto DT, Shi W, Kronzucker HJ. 2017. Nitrogen transformations in modern agriculture and the role of biological nitrification inhibition. Nature Plants. 3:17074. doi: 10.1038/nplants.2017.74
- Dalley DE, Malcolm BJ, Chakwizira E, de Ruiter JM. 2017. Range of quality characteristics of New Zealand forages and implications for reducing the nitrogen leaching risk from grazing dairy cows. New Zealand Journal of Agricultural Research. 60:319–332. doi: 10.1080/00288233.2017.1345762
- Davidson EA, Hart SC, Shanks CA, Firestone MK. 1991. Measuring gross nitrogen mineralization, and nitrification by 15N isotopic pool dilution in intact soil cores. Journal of Soil Science. 42:335–349. doi: 10.1111/j.1365-2389.1991.tb00413.x
- Dawar K, Zaman M, Rowarth JS, Turnbull MH. 2012. Applying urea with urease inhibitor (N-(n-butyl) thiophosphoric triamide) in fine particle application improves nitrogen uptake in ryegrass (lolium perenne l.). Soil Science and Plant Nutrition. 58:309–318. doi: 10.1080/00380768.2012.680050
- de Klein CAM, Cameron KC, Di HJ, Rys G, Monaghan RM, Sherlock RR. 2011. Repeated annual use of the nitrification inhibitor dicyandiamide (DCD) does not alter its effectiveness in reducing N2O emissions from cow urine. Animal Feed Science and Technology. 166–167:480–491. doi: 10.1016/j.anifeedsci.2011.04.076
- de Klein CAM, Eckard RJ. 2008. Targeted technologies for nitrous oxide abatement from animal agriculture. Australian Journal of Experimental Agriculture. 48:14–20. doi: 10.1071/EA07217
- de Klein CAM, Ledgard SF. 2005. Nitrous oxide emissions from New Zealand agriculture – key sources and mitigation strategies. Nutrient Cycling in Agroecosystems. 72:77–85. doi: 10.1007/s10705-004-7357-z
- de Klein CAM, Luo J, Woodward KB, Styles T, Wise B, Lindsey S, Cox N. 2014. The effect of nitrogen concentration in synthetic cattle urine on nitrous oxide emissions. Agriculture Ecosystems & Environment. 188:85–92. doi: 10.1016/j.agee.2014.02.020
- de Ruiter J, Wilson D, Maley S, Fletcher A, Fraser T, Scott W, Berryman S, Dumbleton A, Nichol W. 2009. Management practices for forage brassicas. Forage brassica development group. p. 70.
- Di HJ, Cameron KC. 2018. Ammonia oxidisers and their inhibition to reduce nitrogen losses in grazed grassland: a review. Journal of the Royal Society of New Zealand. 48:127–142. doi: 10.1080/03036758.2017.1354894
- Di HJ, Cameron KC, Podolyan A, Edwards GE, de Klein CAM, Dynes R, Woods R. 2016. The potential of using alternative pastures, forage crops and gibberellic acid to mitigate nitrous oxide emissions. Journal of Soils and Sediments. 16:2252–2262. doi: 10.1007/s11368-016-1442-1
- Di HJ, Cameron KC, Sherlock RR, Shen JP, He JZ, Winefield CS. 2010. Nitrous oxide emissions from grazed grassland as affected by a nitrification inhibitor, dicyandiamide, and relationships with ammonia oxidising bacteria and archaea. Journal of Soils and Sediments. 10:943–954. doi: 10.1007/s11368-009-0174-x
- Dietz M, Machill S, Hoffmann HC, Schmidtke K. 2013. Inhibitory effects of Plantago lanceolata L. on soil N mineralization. Plant and Soil. 368:445–458. doi: 10.1007/s11104-012-1524-9
- Dijkstra FA, Morgan JA, LeCain DR, Follett RF. 2010. Microbially mediated CH4 consumption and N2O emission is affected by elevated CO2, soil water content, and composition of semi-arid grassland species. Plant and Soil. 329:269–281. doi: 10.1007/s11104-009-0152-5
- Dijkstra J, Oenema O, van Groenigen JW, Spek JW, van Vuuren AM, Bannink A. 2013. Diet effects on urine composition of cattle and N2O emissions. Animal. 7:292–302. doi: 10.1017/S1751731113000578
- Edwards GC, De Ruiter JM, Dalley DE, Pinxterhuis JB, Cameron KC, Bryant RH, Di HJ, Malcolm BJ, Chapman DF. 2014. Urinary nitrogen concentration of cows grazing fodder beet, kale and kale-oat forage systems in winter. Proceedings of the 5th Australian Dairy Science Symposium. p. 144–147.
- Erickson AJ, Ramsewak RS, Smucke AJ, Nair MG. 2000. Nitrification inhibitors from the roots of leucaena leucocephala. Journal of Agricultural and Food Chemistry. 48:6174–6177. doi: 10.1021/jf991382z
- Fisk LM, Barton L, Jones DL, Glanville HC, Murphy DV. 2015. Root exudate carbon mitigates nitrogen loss in a semi-arid soil. Soil Biology and Biochemistry. 88:380–389. doi: 10.1016/j.soilbio.2015.06.011
- Gardiner CA, Clough TJ, Cameron KC, Di HJ, Edwards GR, de Klein CAM. 2016. Potential for forage diet manipulation in New Zealand pasture ecosystems to mitigate ruminant urine derived N2O emissions: a review. New Zealand Journal of Agricultural Research. 59:301–317. doi: 10.1080/00288233.2016.1190386
- Gardiner CA, Clough TJ, Cameron KC, Di HJ, Edwards GR, de Klein CAM. 2017. Potential inhibition of urine patch nitrous oxide emissions by Plantago lanceolata and its metabolite aucubin. New Zealand Journal of Agricultural Research. doi: 10.1080/00288233.2017.1411953
- Gopalakrishnan S, Watanabe T, Pearse SJ, Ito O, Hossain ZA, Subbarao GV. 2009. Biological nitrification inhibition by Brachiaria humidicola roots varies with soil type and inhibits nitrifying bacteria, but not other major soil microorganisms. Soil Science and Plant Nutrition. 55:725–733. doi: 10.1111/j.1747-0765.2009.00398.x
- Gregory PJ. 2006. Roots, rhizosphere and soil: the route to a better understanding of soil science? European Journal of Soil Science. 57:2–12. doi: 10.1111/j.1365-2389.2005.00778.x
- Hoogendoorn CJ, Luo J, Lloyd-West CM, Devantier BP, Lindsey SB, Sun S, Pacheco D, Theobald PW, Judge AA. 2016. Nitrous oxide emission factor for urine from sheep and cattle fed forage rape (Brassica napus L.) or perennial ryegrass/white clover pasture (Lolium perenne L. /Trifolium repens). Agriculture, Ecosystems & Environment. 227:11–13. doi: 10.1016/j.agee.2016.04.029
- Huhtanen P, Nousiainen JI, Rinne M, Kytölä K, Khalili H. 2008. Utilization and partition of dietary nitrogen in dairy cows fed grass silage-based diets. Journal of Dairy Science. 91:3589–3599. doi: 10.3168/jds.2008-1181
- Jarvis SC, Scholefield D, Pain B. 1995. Nitrogen cycling in grazing systems. In: Beacon PE, editor. Nitrogen fertilization in the environment. New York: Marcel Dekker; p. 381–419.
- Kebreab E, France J, Beever DE, Castillo AR. 2001. Nitrogen pollution by dairy cows and its mitigation by dietary manipulation. Nutrient Cycling in Agroecosystems. 60:275–285. doi: 10.1023/A:1012668109662
- Kim D-G, Hernandez-Ramirez G, Giltrap D. 2013. Linear and nonlinear dependence of direct nitrous oxide emissions on fertilizer nitrogen input: a meta-analysis. Agriculture Ecosystems & Environment. 168:53–65. doi: 10.1016/j.agee.2012.02.021
- Krol DJ, Carolan R, Minet E, McGeough KL, Watson CJ, Forrestal PJ, Lanigan GJ, Richards KG. 2016. Improving and disaggregating N2O emission factors for ruminant excreta on temperate pasture soils. Science of the Total Environment. 568:327–338. doi: 10.1016/j.scitotenv.2016.06.016
- Kushad MM, Brown AF, Kurilich AC, Juvick JA, Klein BP, Wallig MA, Jeffery EH. 1999. Variation of glucosinolates in vegetable crops of Brassica oleracea. Journal of Agricultural and Food Chemistry. 47:1541–1548. doi: 10.1021/jf980985s
- Lambert MG, Clark DA, Grant DA, Costall DA. 1986. Influence of fertiliser and grazing on North Island moist hill country 4. Pasture botanical composition. New Zealand Journal of Agricultural Research. 29:1–10. doi: 10.1080/00288233.1986.10417968
- Li J, Shi Y, Luo J, Zaman M, Houlbrooke D, Ding W, Ledgard S, Ghani A. 2014. Use of nitrogen process inhibitors for reducing gaseous nitrogen losses from land-applied farm effluents. Biology and Fertility of Soils. 50:133–145. doi: 10.1007/s00374-013-0842-2
- Li SX, Wang ZH, Stewart BA. 2011. Differences of some leguminous and non-leguminous crops in utilization of soil phosphorus and responses to phosphate fertilizers. Advances in Agronomy. 110:125–249. doi: 10.1016/B978-0-12-385531-2.00003-7
- Liu H, Zhou D. 2014. Mitigation of ammonia and nitrous oxide emissions from pasture treated with urine of sheep fed diets supplemented with sodium chloride. Animal Feed Science and Technology. 192:39–47. doi: 10.1016/j.anifeedsci.2014.03.006
- Luo J, Balvert SF, Wise B, Welten B, Ledgard SF, de Klein CAM, Lindsey S, Judge A. 2018. Using alternative forage species to reduce emissions of the greenhouse gas nitrous oxide from cattle urine deposited onto soil. Science of the Total Environment. 610–611:1271–1280. doi: 10.1016/j.scitotenv.2017.08.186
- Luo J, Ledgard SF, de Klein CAM, Lindsey SB, Kear M. 2008. Effects of dairy farming intensification on nitrous oxide emissions. Plant and Soil. 309:227–237. doi: 10.1007/s11104-007-9444-9
- Luo J, Sun XZ, Pacheco D, Ledgard S, Lindsey S, Hoogendoorn CJ, Wise B, Watkins N. 2015. Nitrous oxide emission factors for urine and dung from sheep fed either fresh forage rape (Brassica napus L.) or fresh perennial rygrass (Lolium perenne L.). Animal. 9:534–543. doi: 10.1017/S1751731114002742
- Luo J, van der Weerden TJ, Hoogendoorn C, de Klein CAM. 2009. Determination of the N2O emission factor for animal dung applied in spring in three regions of New Zealand. Report for MAF Policy, Wellington. p. 46.
- Malcolm BJ, Cameron KC, Di HJ, Edwards GR, Moir JL. 2014. The effect of four different pasture species compositions on nitrate leaching losses under high N loading. Soil Use and Management. 30:58–68. doi: 10.1111/sum.12101
- Malcolm BJ, Moir JL, Cameron KC, Di HJ, Edwards GR. 2015. Influence of plant growth and root architecture of Italian ryegrass (Lolium multiflorum) and tall fescue (Festuca arundinacea) on N recovery during winter. Grass and Forage Science. 70:600–610. doi: 10.1111/gfs.12157
- Maljanen M, Komulainen VM, Hytönen J, Martikainen PJ, Laine J. 2004. Carbon dioxide, nitrous oxide and methane dynamics in boreal organic agricultural soils with different soil characteristics. Soil Biology and Biochemistry. 36:1801–1808. doi: 10.1016/j.soilbio.2004.05.003
- Maltais-Landry G. 2015. Legumes have a greater effect on rhizosphere properties (pH, organic acids and enzyme activity) but a smaller impact on soil P compared to other cover crops. Plant and Soil. 394:139–154. doi: 10.1007/s11104-015-2518-1
- Marsden KA, Jones DL, Chadwick DR. 2016. Disentangling the effect of sheep urine patch size and nitrogen loading rate on cumulative N2O emissions. Animal Production Science. 56:265–275. doi: 10.1071/AN15613
- Marsden KA, Jones DL, Chadwick DR. 2017. DMPP is ineffective at mitigating N2O emissions from sheep urine patches in a UK grassland under summer conditions. Agriculture, Ecosystems and Environment. 246:1–11. doi: 10.1016/j.agee.2017.05.017
- Ministry for the Environment. 2018. New Zealand’s greenhouse gas inventory 1990–2016. Fulfilling reporting requirements under the United Nations Framework Convention on Climate Change and the Kyoto Protocol. New Zealand Government. http://www.mfe.govt.nz/sites/default/files/media/Climate%20Change/National%20GHG%20Inventory%20Report%201990-2016-final.pdf.
- Misselbrook TH, Powell JM, Broderick GA, Grabber JH. 2005. Dietary manipulation in dairy cattle: laboratory experiments to assess the influence on ammonia emissions. Journal of Dairy Science. 88:1765–1777. doi: 10.3168/jds.S0022-0302(05)72851-4
- Moir JL, Edwards GR, Berry LN. 2013. Nitrogen uptake and leaching loss of thirteen temperate grass species under high N loading. Grass and Forage Science. 68:313–325. doi: 10.1111/j.1365-2494.2012.00905.x
- Mulligan FJ, Dillon P, Callan JJ, Rath M, O’Mara FP. 2004. Supplementary concentrate type affects nitrogen excretion of grazing dairy cows. Journal of Dairy Science. 87:3451–3460. doi: 10.3168/jds.S0022-0302(04)73480-3
- Munday R, Mhawech-Fauceglia P, Munday CM, Paonessa JD, Tang L, Munday JS, Lister C, Wilson P, Fahey JW, Davis W, Zhang Y. 2008. Inhibition of urinary bladder carcinogenesis bybroccoli sprouts. Cancer Research. 68:1593–1600. doi: 10.1158/0008-5472.CAN-07-5009
- Navarrete S, Kemp PD, Pain SJ, Back PJ. 2016. Bioactive compounds, aucubin and acteoside, in plantain (Plantago lanceolata L.) and their effect on in vitro rumen fermentation. Animal Feed Science and Technology. 222:158–167. doi: 10.1016/j.anifeedsci.2016.10.008
- Nielsen NM, Kristensen T, Nørgaard P, Hansen H. 2003. The effect of low protein supplementation to dairy cows grazing clover grass during half of the day. Livestock Production Science. 81:293–306. doi: 10.1016/S0301-6226(02)00229-4
- Niklaus PA, Le Roux X, Poly F, Buchmann N, Scherer-Lorenzen M, Weigelt A, Barnard RL. 2016. Plant species diversity affects soil–atmosphere fluxes of methane and nitrous oxide. Oecologia. 181:919–930. doi: 10.1007/s00442-016-3611-8
- O’Connell CA, Judson HG, Barrell GK. 2016. Sustained diuretic effect of platain when ingested by sheep. Proceedings of the New Zealand Society of Animal Production. 76:14–17.
- Peng Q, Qi Y, Dong Y, Xiao S, He Y. 2011. Soil nitrous oxide emissions from a typical semiarid temperate steppe in inner Mongolia: effects of mineral nitrogen fertilizer levels and forms. Plant and Soil. 342:345–357. doi: 10.1007/s11104-010-0699-1
- Powlson DS, Poulton PR, Møller NE, Hewitt MV, Penny A, Jenkinson DS. 1989. Uptake of foliar-applied urea by winter wheat (Triticum aestivum): the influence of application time and the use of a new 15N technique. Journal of the Science of Food and Agriculture. 48:429–440. doi: 10.1002/jsfa.2740480405
- Qian JH, Doran JW, Walters DT. 1997. Maize plant contributions to root zone available carbon and microbial transformations of nitrogen. Soil Biology and Biochemistry. 29:1451–1462. doi: 10.1016/S0038-0717(97)00043-6
- Ramírez-Restrepo CA, Barry TN. 2005. Alternative temperate forages containing secondary compounds for improving sustainable productivity in grazing ruminants. Animal Feed Science and Technology. 120:179–201. doi: 10.1016/j.anifeedsci.2005.01.015
- Reardon CL, Strauss SL, Mazzola M. 2013. Changes in available nitrogen and nematode abundance in response to Brassica seed meal amendment of orchard soil. Soil Biology and Biochemistry. 57:22–29. doi: 10.1016/j.soilbio.2012.10.011
- Rochette P, Tremblay N, Fallon E, Angers DA, Chantigny MH, MacDonald JD, Bertrand N, Parent LÉ. 2010. N2o emissions from an irrigated and non-irrigated organic soil in eastern Canada as influenced by N fertilizer addition. European Journal of Soil Science. 61:186–196. doi: 10.1111/j.1365-2389.2009.01222.x
- Selbie DR, Buckthought LE, Shepherd MA. 2015. The challenge of the urine patch for managing nitrogen in grazed pasture systems. Advances in Agronomy. 129:229–292. doi: 10.1016/bs.agron.2014.09.004
- Smolander A, Kanerva S, Adamczyk B, Kitunen V. 2012. Nitrogen transformationsin boreal forest soils – does composition of plant secondary compounds giveany explanations. Plant and Soil. 350:1–26. doi: 10.1007/s11104-011-0895-7
- Steinshamn H, Höglind M, Garmo TH, Thuen E, Brenøe UT. 2006. Feed nitrogen conversion in lactating dairy cows on pasture as affected by concentrate supplementation. Animal Feed Science and Technology. 131:25–41. doi: 10.1016/j.anifeedsci.2006.02.004
- Subbarao GV, Ito O, Sahrawat KL, Berry WL, Nakahara K, Ishikawa T, Watanabe T, Suenaga K, Rondon M, Rao IM. 2007. Scope and stragegies for regulation of nitrification in agricultural systems – challenges and opportunities. Critical Reviews in Plant Sciences. 25:303–335. doi: 10.1080/07352680600794232
- Subbarao GV, Rao IM, Nakahara K, Sahrawat L, Ando Y, Kawashima T. 2013. Potential for biological nitrification inhibition to reduce nitrification and N2O emissions in pasture crop-livestock systems. Animal. 7:322–332. doi: 10.1017/S1751731113000761
- Subbarao GV, Rondon M, Ito O, Ishikawa T, Rao IM, Nakahara K, Lascano C, Berry WL. 2007. Biological nitrification inhibition - is it a widespread phenomenon? Plant and Soil. 294:5–18. doi: 10.1007/s11104-006-9159-3
- Subbarao GV, Sahrawat KL, Nakahara K, Ishikawa T, Kishii M, Rao IM, Hash CT, George TS, Srinivasa Rao P, Nardi P, et al. 2012. Biological nitrificatin inhibition – a novel strategy to regulate nitrification in agricultural systems. Advances in Agronomy. 114:249–302. doi: 10.1016/B978-0-12-394275-3.00001-8
- Subbarao GV, Yoshihashi T, Worthington M, Nakahara K, Ando Y, Sahrawat KL, Rao IM, Lata JC, Kishii M, Braun HJ. 2015. Suppression of soil nitrification by plants. Plant Science. 233:155–164. doi: 10.1016/j.plantsci.2015.01.012
- Totty VK, Greenwood SL, Bryant RH, Edwards GR. 2013. Nitrogen partitioning and milk production of dairy cows grazing simple and diverse pastures. Journal of Dairy Science. 96:141–149. doi: 10.3168/jds.2012-5504
- van der Weerden TJ, Styles TM. 2012. Reducing nitrous oxide emissions from grazed winter forage crops. Proceedings of the New Zealand Grassland Association. 74:57–62.
- van Groenigen JW, Palermo V, Kool DM, Kuikman PJ. 2006. Inhibition of denitrification and N2O emission by urine-derived benzoic and hippuric acid. Soil Biology and Biochemistry. 38:2499–2502. doi: 10.1016/j.soilbio.2006.02.023
- Wang X, Tang C, Severi J, Butterly CR, Baldock JA. 2016. Rhizosphere priming effect on soil organic carbon decomposition under plant species differing in soil acidification and root exudation. New Phytologist. 211:864–873. doi: 10.1111/nph.13966
- Waghorn G, Griffin A, Bryant M, Dalley D. 2018. Digestion and nitrogen excretion by Holstein-Friesian cows fed grasses with lucerne or lucerne and plantain. Animal Production Science. 59:1070–1080. doi: 10.1071/AN18105
- Whitehead DC. 1995. Grassland nitrogen. Wallingford: CAB International.
- Whitehead DC, Bristow AW. 1990. Transformations of nitrogen following the application of 15N-labelled cattle urine to an established grass sward. The Journal of Applied Ecology. 27:667–678. doi: 10.2307/2404310
- Williams PH, Haynes RJ. 1994. Comparison of initial wetting pattern, nutrient concentrations in soil solution and the fate of 15N-labelled urine in sheep and cattle patch areas of pasture soil. Plant and Soil. 162:49–59. doi: 10.1007/BF01416089
- Woods RR, Cameron KC, Edwards GR, Di HJ, Clough TJ, Nicholson F. 2016. Effects of forage type and gibberellic acid on nitrate leaching losses. Soil Use and Management. 32:565–572. doi: 10.1111/sum.12297
- Ye L, Dinkova-Kostova A, Wade KL, Talalay P. 2002. Quantitative determination of dithiocarbamates in human plasma, serum, erythrocytes and urine: pharmacokinetics of broccoli sprout isothiocyanates in humans. Clinica Chimica Acta. 316:43–53. doi: 10.1016/S0009-8981(01)00727-6
- Zakir HAKM, Subbarao GV, Pearse SJ, Gopalakrishnan S, Ito O, Ishikawa T, Kawano N, Nakahara K, Yoshihashi T, Ono H, Yoshida M. 2008. Detection, isolation and characterization of a root-exuded compound, methyl 3-(4-hydroxphenyl) propionate, responsible for biological nitrification inhibition by sorghum (Sorghum bicolar). New Phytologist. 180:442–451. doi: 10.1111/j.1469-8137.2008.02576.x