ABSTRACT
Plant species inventories provide the foundation for more complex analytical studies and are the basis of monitoring programmes; however, if they are to provide reliable information in the long term, their level of completeness needs to be estimated. This work assessed the completeness of inventories of climbing plants and vascular epiphytes in swamp forest remnants of the Araucanía region of south-central Chile, which has been severely disturbed by agroforestry expansion. We sampled 30 sites using transects, with observations from ground level to a height of 2.3 m up the trees. To assess the potential existence of unrecorded species we drew rarefaction curves based on sample trees and extrapolated them towards one of the most intensely sampled sites. We then calculated the asymptotic species richness with the Chao 1 estimator. The results showed: (1) a total richness of 16 species of epiphytes and 17 species of climbing plants; (2) the rarefaction curve differentiated only two categories of sampling effort (‘rich’ and ‘poor’ sites) as a result of the substantial overlap of the confidence limits at 95%; and (3) the maximum richness estimated by Chao 1 was similar to the richness observed in all the sites. We conclude that greater sampling effort is required to obtain tighter statistical confidence levels in the rarefaction curve; however, from a biological point of view, the sampling effort achieved adequate representation of the species richness at all the sites. Total richness of vascular epiphytes and climbing plants was only slightly below values reported for much larger areas of better-conserved forest in south-central Chile and adjacent areas of Argentina. Finally, we found evidence that forest fragmentation has more severe effects on species richness of vascular epiphytes than on that of climbing plants.
Introduction
Basic inventories are fundamental for knowledge of species diversity in a region and provide the foundation for more complex analytical studies (Funk Citation2006). The species lists generated from these inventories allow: (1) study of the distribution of plant assemblages in the landscape (Götzenberger et al. Citation2012); (2) determine the conservation status of species on a regional scale (UICN Citation2001; Squeo et al. Citation2010); and (3) research into biogeographical (e.g. Swenson et al. Citation2012) or macro-ecological areas of interest (e.g. Cadotte et al. Citation2006).
Species richness is perhaps the measurement most commonly produced from inventories to describe the diversity of a community (e.g. Hortal et al. Citation2007). Currently, measuring the number of species is of great scientific interest because it is the basis of many models in community ecology and an implicit objective of biological conservation studies (Gotelli & Colwell Citation2001; Brooks et al. Citation2004; Colwell et al. Citation2012). However, quantifying the number of species (even of vascular plants) is a difficult task, especially in large areas, because: (1) in practice it is impossible to identify all the species or count all the individuals of every square centimetre of an ecosystem or landscape (Chiarucci & Palemer Citation2005); (2) in nature many species are rare and unlikely to be detected (Magurran & Handerson Citation2003); and (3) logistical limitations require an equilibrium between efficient sampling and the resources required (cost, time and accessibility) for a complete inventory (Hermoso et al. Citation2013).
A reasonable way of overcoming these difficulties is to combine two strategies: first, intensive sampling of assemblages of species sensitive to ecosystem or climate changes, which can be used to infer certain ecosystem processes and as a signal for the rest of the biota (Lambeck Citation1997; Carignan & Villard Citation2002; Zotz & Bader Citation2009; Dominguez et al. Citation2012; Hermoso et al. Citation2013); and second, assessing the quality of the inventory statistically (not by a census) when the sampling effort is unequal or when the expected richness at one or more sites is unknown (Colwell & Coddington Citation1994). To do this, the completeness of the inventory can be assessed by rarefaction curves and asymptotic estimators of species richness (Colwell & Coddington Citation1994). For the first case, algorithms have been proposed that allow the species richness at different sites (or moments) to be compared by interpolation (the traditional way) or extrapolation of the rarefaction curves. The second case is particularly useful when quantitative data are available (Colwell et al. Citation2012; Colwell Citation2013).
In this context, climbing plants and vascular epiphytes are good study models because: (1) they depend almost exclusively on forest trees for their survival (Benzing Citation1990; Schnitzer et al. Citation2015), which can be used to infer certain processes in the ecosystem and as a signal for the rest of the biota; (2) they present differentiated responses to environmental gradients, making them good indicators of the ecological state and the dynamic of the forest ecosystem (Pincheira-Ulbrich Citation2011); (3) they are distributed over a wide latitude, allowing the results to be extrapolated to different areas; and (4) they constitute functional groups that can be clearly differentiated by their morphology, physiology and life history (Schnitzer & Bongers Citation2002; Bartels & Shen Citation2012), meaning that their differentiated representation in the landscape would mark the different functioning of community structures.
Inventories of these assemblages can then become the starting point for a monitoring programme. However, a complete species inventory is required to extract reliable information over the long term on which to base later ecological studies (Hortal et al. Citation2007; Domínguez et al. Citation2012; Rivera Huntiel et al. Citation2012). Inventories are urgently required in many forest regions of Latin America, particularly regions suffering strong pressure from human use, as is the case of the swamp forest of southern South America (Möller & Muñoz-Pedreros Citation2014).
Chilean swamp forest, dominated by trees of the family Myrtaceae, is threatened by agricultural and forestry expansion. These forests are described as azonal hygrophilous formations, i.e. their presence is not determined by the climate but by excess soil moisture (Ramírez et al. Citation1983; San Martín et al. Citation1988; Ramírez et al. Citation1996). Within the broad latitudinal range in which swamp forest is distributed (between 30°S and 41°28′S), one of the areas most deserving of attention is the coast of the Araucanía region of Chile, where a considerable area of swamp forest still exists (c. 7500 ha).
Here we assess the completeness of inventories of climbing plants and vascular epiphytes in swamp forest remnants of the Araucanía region. Three objectives were established for this study: (1) to present a complete inventory of both assemblages; (2) to evaluate the sampling effort in 30 sites by rarefaction curves based on sample trees; and (3) to estimate the maximum species richness expected in these sites. We aimed to lay the basis for understanding how the species richness of both assemblages is affected by the anthropisation of this landscape.
Materials and methods
Study area
The study area is located on the coast of the Araucanía region of Chile (38°30′–39°30′S, 72°45′–73°30′W) (). It covers an area of 1656 km2, bounded by the Imperial River in the south and the Queule in the north, and lying between the Coastal Range to the east and the Pacific Ocean to the west (Peña-Cortés et al. Citation2014). The climate is oceanic with a Mediterranean influence (Di Castri & Hajek Citation1976), with average annual precipitation of 1200–1600 mm.
Figure 1. Map of the study area with the locations of the 30 sampling sites in the coastal swamp forest remnants of the Araucanía Region, Chile.
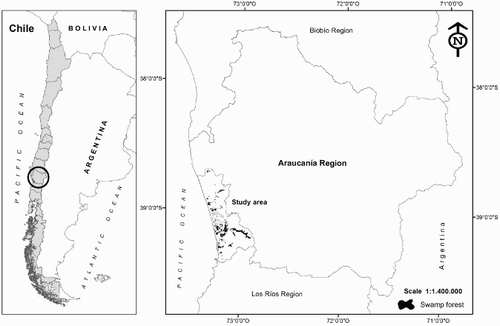
The territory is distributed between numerous indigenous Mapuche communities and private farming/forestry properties. The land is divided into small plots, with high poverty and rural dwelling, largely unchanged since the middle of the 20th century (Gissi Citation2004; Peña-Cortés et al. Citation2009). As a result, historical and current pressure on forest has meant that most of its area is in a degraded state, set in a matrix of anthropic landscape (Peña-Cortés et al. Citation2011; Hauenstein et al. Citation2014).
The forest is a secondary ecosystem (diameter at breast height of trees = 19 ± 11 cm), consisting mainly of endemic species of the Myrtaceae family and dominated by two species: Myrceugenia exsucca O.Berg and Blepharocalyx cruckshanksii (Hook. & Arn.) Nied. This species composition is characteristic of these formations throughout the temperate phytogeographic region (Ramírez et al. Citation1983; Ramírez et al. Citation1996). However, introduced species of European origin such as Rubus constrictus Lefèvre & P.J.Müll. (Rosaceae) and Salix viminalis L. (Salicaceae) can also be found (Hauenstein et al. Citation2014). The forest grows on fluvio-marine and alluvial plains on ground with temporary or permanent flooding, forming intricate and often connected networks. Four hundred and twenty-seven fragments of forest have been identified, covering an area of 7675 ha, which represents 4.6% of the territory (Peña-Cortés et al. Citation2011).
Selection of sampling sites
Before fieldwork began, the study area was divided into six large zones corresponding to the principal hydrographic basins (Budi, Chelle, Toltén, Boldo, Boroa and Queule), to ensure that the distribution of swamp forest in the territory was fully represented. The digital forest template prepared by Peña-Cortés et al. (Citation2011) was superimposed on a satellite image obtained from Google Earth (2015 Google), and a 2.5 km2 grid was projected on to this using the ArcGis 10.1 software with ArcMap (ESRI Citation2011). The grid made it easier to update the forest distribution and eliminate those zones no longer covered by forest (e.g. felled or drained). To capture the maximum floristic diversity in the ecosystems, the forested area was grouped into five size classes: <0.5 ha, 0.5–2 ha, 2–10 ha, 10–50 ha, >50 ha. The seven largest fragments (>50 ha) were chosen subjectively and six fragments were selected at random from each of the other classes, except the 0.5–2 ha class, which contained only five fragments. This produced a total of 30 sampling sites distributed over the whole study area.
Sampling design and data collection
The sampling design was non-random in order to include the largest possible variety of micro-habitats and number of rare species (Dieckman et al. Citation2007; Croft & Crow-Fraser Citation2009). Data were collected from 2011 to 2013 with 30 days spent in the field. Sampling followed a ground-based observations protocol (Flores-Palacios & García-Franco Citation2001), using individual trees as the basic measure of sampling effort. Trees are the quintessential substrate of these species, where the plants are anchored and spend most of their life cycle. Tree selection was by transect sampling, oriented from the edge towards the centre of the fragment (Brower et al. Citation1990). In each transect, a circular quadrat 3 m in diameter was established (7.06 m2), with a distance of at least 10 m between each quadrat. All the quadrats were designed to cover most of the core area.
For logistical reasons (i.e. accessibility, cost and time), we sampled climbing plants and vascular epiphytes in 180 quadrats (see below). The quadrats were established and geo-referenced across the swamp forest with variable sampling intensity which depended on the fragment size (site) and the accumulated species richness recorded in the field. In this way, a minimum of three quadrats were determined for small fragments (<1 ha) and a maximum of 18 quadrats for the largest fragment (936 ha).
Within each quadrat, the abundance of all epiphytes (e.g. fronds) and climbing plants (i.e. stems) was counted systematically from ground level to a height of 2.3 m up the trunks of trees with diameter at breast height (DBH) equal to or greater than 5 cm. This protocol is commonly used in South American temperate forests (see Pincheira-Ulbrich Citation2011). To increase the possibility of observing more species outside the quadrat, only the abundance of new species (on the trees) along the transect line was counted.
It is difficult to directly quantify the abundance (number of individuals) of epiphyte and climbing plant assemblages (e.g. filmy ferns), since an individual may present vegetative reproduction and/or cover extensive areas of the trunk. In this study we therefore used as a substitute the number of fern fronds and the number of creeper stems; and in the case of the epiphyte Fascicularia bicolor (Ruiz & Pav.) Mez, the number of plants (rosettes). These surrogate measures are commonly used criteria in the clonal population study (e.g. Wolf et al. Citation2009; IUCN Citation2010; Mondragón Citation2011).
The height limit for abundance sampling was set by a technical ecological criterion, considering that: (1) the greatest diversity of epiphytes (e.g. filmy ferns) is found in the first few metres of trunk height, particularly in secondary forests, while observation of climbing plants anchored in the earth does not require greater height; and (2) this approach facilitates the systematic inclusion of all the trees in the quadrat (e.g. Muñoz et al. Citation2003; Woda et al. Citation2006; San Martin et al. Citation2008).
Epiphyte identification followed the criteria established in the publications of Diem & Lichtenstein (Citation1959), Marticorena & Rodríguez (Citation1995), Rodríguez et al. (Citation2009) and Larsen et al. (Citation2014), while climbing plant identification was based on Martínez (Citation1985) and Marticorena et al. (Citation2010). Some specimens were identified in the herbarium of Universidad de Concepción (CONC), Chile. The nomenclature was based on The International Plant Names Index (Citation2008).
Standardisation of the sampling effort
To assess the sampling effort at the sites, rarefaction curves were drawn for each forest. In this approach, we used sample-based incidence data as a function of trees sampled, rescaling (extrapolating) the number of species towards one of the most intensely sampled sites, which is called the ‘reference sample’ equal to 75 trees (sensu Colwell et al. Citation2012). The null hypothesis, tested by comparison of two sites with different sample sizes (n trees), was that the species richness in the smaller sample does not differ from that in the larger sample (Gotelli & Colwell Citation2011). The calculations followed equation 18 of Colwell et al. (Citation2012) independently for the assemblages of vascular epiphytes and climbing plants. Using this technique (extrapolation) prevents loss of the information contained in large assemblages as has occurred traditionally, because the species richness was interpolated towards the smaller sample (see Colwell et al. Citation2012). The 95% confidence intervals were obtained analytically with equation 19 of Colwell et al. (Citation2012) considering a variance not conditioned by the observed species richness, so these limits do not converge on zero. This supposes that some species in the assemblage sampled are not detected when all the sampling units (i.e. trees) in a particular site are pooled (Colwell Citation2013). The estimated richness was considered statistically different when there is non-overlap with the confidence intervals (Colwell et al. Citation2012). All calculations were performed using EstimateS 9.10 software (Colwell Citation2013).
Expected (minimum) richness
The extrapolated rarefaction curve calculated with the unconditional ‘open’ confidence intervals supposes that some species in the assemblage sampled remain undetected, even when asymptote is achieved (Colwell Citation2013). To assess this, the expected species richness in each of the sites was calculated using equation 2 of Chao 1 which includes an adjustment factor for when the number of sample units (i.e. number of trees) is small (Colwell Citation2013). The calculations were done independently for the assemblages of vascular epiphytes and climbing plants using the EstimateS 9.10 software (Colwell Citation2013). Chao 1 calculates the (asymptotic) species richness as a function of the number of singletons (species represented by a single individual) and doubletons (represented by exactly two individuals) observed in a group of samples (n trees in a site). The underlying principle is that the abundance of very rare species (which emerges from the sampled trees pooled on a site) can be used to estimate the frequency of undetected species (Gotelli & Colwell Citation2011). Asymptote is reached when there is no singleton or exactly one singleton and no doubletons (Colwell Citation2013). This implies that an inventory has been achieved of all the species in a determined site (i.e. fragment of forest), under the supposition that the samples represent the site properly. Chao 1 is a robust, non-parametric estimator, which does not require any supposition of the location of the samples in space, or of the model for the distribution of species abundances (Gotelli & Colwell Citation2001, Citation2011). In some cases, when the coefficient of variation of the abundance is greater than 0.5, Chao 1 is inaccurate; in these cases, the index was calculated with the classic formula following the recommendation of Anne Chao (equation 2 in Colwell Citation2013).
The 95% confidence intervals were calculated in the EstimateS 9.10 software with the logarithmic transformation proposed by Chao (Citation1987). Equations 13 or 14 presented in Colwell (Citation2013) were used for this purpose, depending on whether equation 1 or 2 from Chao 1 was used. To compare the estimated richness between the different sites, the sampling units were resampled at random with replacement by random numbers obtained from the strong-hash-driven cryptographic algorithm with 100 randomisations. This allowed unconditional confidence limits to be obtained which do not converge to zero at the right-hand end of the curve (Colwell et al. Citation2012, Citation2013).
Results
Richness and composition of flora
The sampling protocol resulted in 914 sample units ( = 30 ± 18 trees in 30 sites) on which 33 species were identified for the coastal swamp forest of the Araucanía region. Of these, 17 were climbing plants (including two introduced species) and 16 were vascular epiphytes. The climbing plants were represented by 15 families, while the epiphytes came from six families. Of the latter assemblage the filmy ferns family, Hymenophyllaceae, presented the highest representation with 10 species.
The most abundant climbing plants were Luzuriaga radicans Ruiz & Pav. and Cissus striata Ruiz & Pav., with 1228 and 759 stems, respectively. These species also had the highest incidence, since they were found on 18.3% and 24% of the trees, respectively (). The most abundant epiphytes were Hymenophyllum plicatum Kaulf. and Hymenophyllum caudiculatum Mart. with 10,381 and 7956 fronds, respectively (). Hymenophyllum plicatum also presented the highest incidence with 20.4% of the trees. Other important species were Asplenium dareoides Cav., Hymenophyllum krauseanum Phil., Hymenophyllum caudiculatum and Sarmienta scandens Pers. with incidence ranging between 10.2% and 13.6%.
Table 1. Vascular epiphytes and climbing plants recorded on 914 trees in coastal swamp forest remnants of the Araucanía region, Chile.
The results showed that most species (n = 22) are low frequency (incidence <5%), despite the high abundances registered in the forest. The situation is more critical when low incidence is combined with reduced abundance, as with Hymenophyllum secundum Hook. & Grev. and the climbing plant Hydrangea serratifolia F.Phil.
Standardisation of the sampling effort
The standardisation of the sampling effort by extrapolation of the rarefaction curve from each reference sample (number of trees in each site) to a number of 75 trees showed that the variability of the expected species richness between the sites and within each assemblage resulted in the identification of only two categories of site (‘rich’ and ‘poor’), for both vascular epiphytes and climbing plants, since the limits for 95% confidence overlap substantially within each category (; Table A1.1). This implies that if we could record the species richness on the same number of trees (i.e. 75) in every site, we would distinguish only two groups of species richness (using a conservative approach).
Figure 2. Extrapolation of the rarefaction curve from the reference sample for vascular epiphytes (A) and climbing plants (B) up to 75 samples (trees) in 30 swamp forest sites. The unbroken and broken lines represent groups of rich and poor sites, respectively, which present no statistical difference in standardised richness at 95% confidence. The circles represent the curves that achieve asymptote. To avoid saturating the image, the confidence limits of the rarefaction curves are not shown (see Table A1.1).
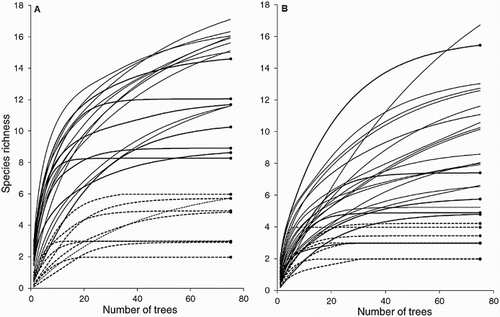
In general, the sites rich in epiphytes (observed) are associated with larger fragments while at the other extreme both assemblages become poorer, although we note that in the case of epiphytes there is a superposition zone of the curves for the rich and poor sites between 1 and 3.9 ha. Climbing plants present three peaks for richness (86–156, 50 and 5.5 ha) with 10 species in each, while epiphytes present a peak for the largest sites (156 and 936 ha) with 16 species (see Table A1.1).
The projection of the rarefaction curve suggests that the richness of approximately half the sites (15 for epiphytes and 13 for climbing plants) would achieve asymptote, and that greater sampling effort is required for climbing plants than for epiphytes to achieve this statistical asymptote, since in most of the sites, the number of undiscovered species would be larger for climbing plants. Unusual values can also be observed. For example, if we observe only the upper confidence limits in all the sites and stop at the 33 ha site (see Table A1.1), we would probably find up to 15 more species of epiphyte ( = 6.36 ± 5.08 species considering all the sites), while in climbing plants the expected maximum number of additional species would be 24 in the 0.13 ha site (
= 7.03 ± 5.39 species considering all the sites) (Table A1.1). These richness values are not possible in the field, for two reasons: (1) there are no more species in the region (see ‘Discussion’); and (2) in small fragments practically all the trees at the site were inspected.
Expected (minimum) richness
The estimated asymptotic richness presented small differences between the observed value and the value estimated by Chao 1, of less than one species for epiphytes ( = 0.51 ± 0.47) and close to one species for climbing plants (
= 1.10 ± 1.01) (). The Chao 1 result was less than the observed richness of epiphytes in most sites (except the sites of 156 and 11 ha) while the richness of climbing plants remained within the confidence limits, except for some rich (e.g. 133, 86 and 33 ha) and poor sites (e.g. 0.8 and 0.13 ha) where the number of species observed was higher (). This implies that the inventory of epiphytes and climbing plants was representative of the expected minimum richness, especially for epiphytes where in practical terms no other species would be expected. For the climbing plants assemblage on the other hand it is likely that two or three more species might be found in medium to large sites (e.g. 936, 50, 40 and 22 ha). The results show that when the assemblages are compared site to site, there is significantly greater species richness for epiphytes (except for the site of 86 ha) than for climbing plants from the 7.1 ha site and larger (P ≤ 0.05, see Table A1.1). This pattern is significant and is the opposite of that observed in the 5.5 ha site and smaller, where climbing plants are more important (P ≤ 0.05, except for the sites of 3.4 and 1.9 ha).
Figure 3. Estimated asymptotic richness for assemblages of vascular epiphytes (A) and climbing plants (B) in 30 swamp forest sites (ha). The dots represent the Chao 1 estimator and the bars the 95% confidence intervals (see Table A1.1).
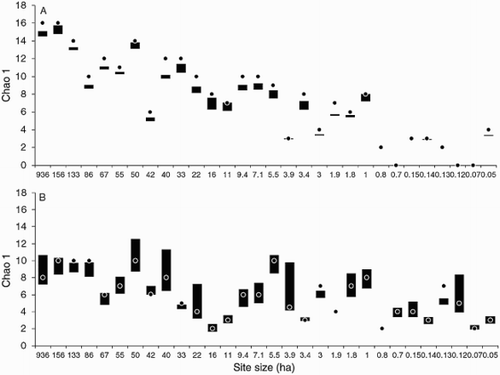
The variability in species richness along the fragment size gradient was clearly differentiated between assemblages (). In the case of epiphytes, the estimated richness reached its maximum in the largest sites (936 and 156 ha), diminishing with the fragment size gradient. There were even sites with 95% confidence intervals for species richness that did not match the intervals of any other site; this means that the number of species is unique and distinguishable from other sites (e.g. 133 and 42 ha, see Table A1.1). In the case of climbing plants, the variation in the species richness is less clear (the overlap between sites is bigger), with five maxima of 10 species observed. No significant differences were found in species richness between the majority of the large fragments (936, 156, 133, 86 and 50 ha), some medium (8, 1.8 and 1 ha) and one small (0.12 ha) ().
Discussion
Chilean coastal swamp forest remnants were surprisingly rich in both vascular epiphytes (16 species) and climbing plants (15 native species) (). These values are almost as high as those reported from much more extensive areas of better-conserved forest in the Chilean coast ranges further south (Smith-Ramirez et al. Citation2005) and in the Argentine Andes (Ezcurra & Brion Citation2005). Chilean swamp forest may therefore have an important role in conserving these groups of plants, despite its degraded state and survival mostly as second-growth stands.
Likewise, several of the sites sampled in the present study appear to be as rich in species as mature forests of other forest types in the Chilean coast ranges, which have a more diverse structure. This can be seen in Jiménez-Castillo et al. (Citation2007), who reported the presence of five and seven species of climbing plants in two sites, and Pincheira-Ulbrich et al. (Citation2012) who recorded a maximum of seven climbing plants and 12 epiphytes (for a review, see Pincheira-Ulbrich Citation2011). Nevertheless, it must be remembered that no simple, direct comparison can be made of this study with the few existing inventories of temperate forests which include climbing plants and vascular epiphytes, because different methodologies have been used and often different objectives pursued (e.g. Pincheira-Ulbrich Citation2011). Moreover, and especially, because the other studies do not include explicit statistical assessments of their completeness or the effect of the sampling effort, as has been strongly suggested in the literature (Gotelli & Colwell Citation2001; Jiménez-Valverde & Hortal Citation2003; Colwell et al. Citation2012; Rivera Huntiel et al. Citation2012).
The transect-based sampling protocol and intensity of sampling (914 trees) of our study enabled detection of species that had not previously been recorded in these forests. Examples include the filmy ferns Hymenophyllum cuneatum Kunze and Hymenophyllum secundum, and the climbing plants Griselinia racemosa (Phil.) Taub. and Hydrangea serratifolia. These can now be added to the earlier inventory carried out in the same study area (Hauenstein et al. Citation2014), which identified 14 epiphytes and nine climbing plants using Braun-Blanquet’s methodology. The use of transects in this method allows environmental gradients to be captured more efficiently than with plots, even though the specific area is smaller; in this study at least this would appear to be a good way of identifying rare species, an important aspect for biological conservation (Croft & Crow-Fraser Citation2009; Dieckman et al. Citation2007).
The sampling protocol used here is a non-random method, implying that the results of a sample may not be as representative of population parameters as a randomised protocol. However, the advantage of subjective sampling is that it allows the use of scientific foreknowledge of the study site, and it has proved to be more efficient in identifying rare species (it facilitates the search for species in field); the decision on the type of sampling will therefore depend on the purpose of study (Kenkel et al. Citation1989; Diekmann et al. Citation2007).
The rarefaction curves extrapolated to 75 trees per site showed that the variability of the expected richness can be reduced to two groups: rich sites and poor sites. However, it is important to note that in approximately half of the sites this curve does not stabilise and presents a wide variance, meaning that a greater sampling effort would be required. Nevertheless, even if it were possible to increase the sampling effort, it would be very difficult to find more species in small fragments of forest (<1 ha), because there are limitations in the availability of substrate (trees) and because in the field practically all the trees in these fragments were inspected and there are simply no more species. At the other extreme of fragment size, and considering the local richness and the data provided by other works (e.g. Pincheira-Ulbrich Citation2011), the large fragments where asymptote was not reached (e.g. 936, 156 and 133 ha) might not hold any more species since the maximum species richness for the region has already been reached (see Smith-Ramirez et al. Citation2005). More attention may need to be paid to the proportion of rare species in the medium to large sites, since recording rare species requires a greater capture effort for the rarefaction curve to reach asymptote (Thompson & Whitters Citation2003).
Despite the potential limitations of our sampling method, the Chao 1 index, used to assess undetected species, resulted in a better-fitting richness with narrower confidence intervals than estimates reached by rarefaction. The advantage of this estimator is that when representative sampling has been done of the study community, for example using the scientific judgement of the researcher (Wallington & Moore Citation2005), and the abundance data have been obtained, the effects of under-sampling environmental gradients and spatial auto-correlation do not appear to have any consequences in this non-parametric estimator (O’Hara Citation2005; Hortal et al. Citation2006; Colwell Citation2013).
Species richness estimators depend on having a sufficiently large sample size; for Chao 1 this means that the proportion of singletons must be less than 50% (Colwell Citation2013) as occurs in our study (see Table A1.1). Although Chao 1 was derived for estimating the lower limit of species richness (Chao Citation1984; Colwell et al. Citation2012), in the opinion of Chao & Shen (Citation2006), based on the works of Shen et al. (Citation2003) and Chao et al. (Citation2006), for many sets of biological data, Chao 1 could justifiably be used as an estimator of the total species richness, not just the lower limit. Consequently, we believe that an appropriate level of inventory completeness was achieved.
Our species richness data suggest that assemblages of vascular epiphytes gradually become impoverished in small forest fragments. Climbing plants on the other hand appear to be less affected by habitat reduction and are more likely to be found in small fragments, while epiphytes tend to disappear (see Table A1.1). This may be explained by the stronger edge effect in small sites and/or reduction of the core area of forest fragments which change micro-environmental patterns (at habitat scale, see Murcia Citation1995) and influence the distribution of these assemblages (e.g. Pincheira-Ulbrich et al. Citation2012). Alternatively, following the classical theory of island biogeography, the mechanism for maintaining this diversity in the landscape would be based on a dynamic of colonisation and extinction where small fragments would hold relatively few species due to their higher extinction rates, while large fragments maintain greater species richness and lower extinction rates (MacArthur & Wilson Citation1967). Future studies need to be conducted to determine the relative importance of the processes at habitat and landscape scale that explain changes in diversity.
In temperate forests it has in fact been shown that more humid environments provide better micro-habitat conditions for epiphytic ferns (Hymenophyllaceae), while climbing plants prefer sites with more direct sunlight (Muñoz et al. Citation2003; Woda et al. Citation2006; San Martín et al. Citation2008; Parra et al. Citation2009). In general, micro-climatic and forest structural changes appear to correlate more directly with vascular epiphytes than with climbing plants (e.g. Gianoli et al. Citation2010; Reyes et al. Citation2010; Saldaña et al. Citation2014). This reflects the vulnerability of epiphytes to desiccation, because of their limited access to water (Zotz & Bader Citation2009).
Conclusions
Our assessment of the effect of sampling effort on the inventory of climbing plants and vascular epiphytes in Chilean swamp forest led us to conclude that complete inventories were obtained. The Chao 1 index resulted in a better-fitting richness with narrower confidence intervals than estimates reached by rarefaction. Use of this index is therefore recommended when prior information of ecosystem and regional richness is available, and when the proportion of singletons is adequately captured by the sampling design. We found evidence that forest fragmentation has more severe effects on species richness of vascular epiphytes than on that of climbing plants.
The swamp forest of the Araucanía region maintains a particularly high richness of climbers and vascular epiphytes, comparable to larger, better-conserved areas of forest in the same region. This was unexpected, considering that this ecosystem is dominated by just two tree species, and that remaining stands are mostly second-growth and under strong anthropogenic pressure.
Acknowledgements
Elías Andrade, Erwin Vázquez, Enrique Hauenstein, Ulises Sambrano, María Jesús Vargas, Martina Pincheira, Alvaro Montaña, Erna Chaparro, Elías Painevilo and Rodrigo Cid for their collaboration in the field. Enrique Hauenstein, Diego Alarcón, Liliana Casaa, Cristian Larsen, Jonathan Urrutia and the CONC herbarium for their generous help in identifying rare species. Daniel Rozas and Elías Andrade for their dedicated work in preparing the cartography and database. To Chris Lusk, Kevin Burns, Amanda Taylor and two anonymous reviewers for their generous contribution. To the small landowners, administrators and estate owners for allowing us access to their farms. The research was conducted within the framework of Project 075/2011 ‘Plantas trepadoras y epifitas vasculares en bosques pantanosos del borde costero de la Araucanía; determinación de especies y áreas de conservación’.
Associate Editor: Dr Chris Lusk.
Disclosure statement
No potential conflict of interest was reported by the authors.
ORCiD
Jimmy Pincheira-Ulbrich http://orcid.org/0000-0001-9841-7426
Francisco Aguilera-Benavente http://orcid.org/0000-0001-5710-2057
Additional information
Funding
References
- Bartel S, Chen H. 2012. Mechanisms regulating epiphytic plant diversity. Crit Rev Plant Sci. 31:391–400. doi: 10.1080/07352689.2012.680349
- Benzing D. 1990. Vascular epiphytes. New York, NY: Cambridge University Press.
- Brooks T, da Fonseca G, Rodrigues A. 2004. Species, data, and conservation planning. Conservation Biol. 18:1682–1688. doi: 10.1111/j.1523-1739.2004.00457.x
- Brower J, Zar J, Von Ende C. 1990. Field and laboratory methods for general ecology. Dubuque: Brown Company Publishers.
- Cadotte M, Murray B, Lovett–Doust J. 2006. Ecological patterns and biological invasions: using regional species inventories in macroecology. Biol Invasions. 8:809–821. doi: 10.1007/s10530-005-3839-4
- Carignan V, Villard M. 2002. Selecting indicator species to monitor ecological integrity: a review. Environmental Monit Assess. 78:45–61. doi: 10.1023/A:1016136723584
- Chao A. 1984. Non-parametric estimation of the number of classes in a population. Scand J Stat. 11:265–70.
- Chao A. 1987. Estimating the population size for capture-recapture data with unequal catchability. Biometrics. 43:783–91. doi: 10.2307/2531532
- Chao A, Shen T. 2006. Species Prediction and Diversity Estimation (SPADE) [Software and User’s Guide]. [cited 2016 July 24]. Available from: http://chao.stat.nthu.edu.tw/wordpress/wp-content/uploads/software/SPADE_UserGuide.pdf
- Chao A, Shen T, Hwang W. 2006. Application of Laplace’s boundary-mode approximations to estimate species and shared species richness. Aust NZ J Stat. 48:117–128. doi: 10.1111/j.1467-842X.2006.00430.x
- Chiarucci A, Palmer M. 2005. The inventory and estimation of plant species richness. Encyclopaedia of Life Support Systems (EOLSS). Developed under the Auspices of the UNESCO. Oxford: EOLSS Publishers.
- Colwell R. 2013. EstimateS: Statistical estimation of species richness and shared species from samples. Version 9. User's Guide and application. [cited 2016 July 24]. Available from: http://purl.oclc.org/estimates
- Colwell R, Chao A, Gotelli N, Lin S, Mao Ch, Chazdon R, Longino J. 2012. Models and estimators linking individual-based and sample-based rarefaction, extrapolation and comparison of assemblages. J Plant Ecol. 5:3–21. doi: 10.1093/jpe/rtr044
- Colwell R, Coddington J. 1994. Estimating terrestrial biodiversity through extrapolation. Philos T Roy Soc B: Biol Sci. 345:101–18. doi: 10.1098/rstb.1994.0091
- Croft M, Crow-Fraser P. 2009. Non-random sampling and its role in habitat conservation: a comparison of three wetland macrophyte sampling protocols. Biodivers Conserv. 18:2283–2306. doi: 10.1007/s10531-009-9588-4
- Di Castri F, Hajek E. 1976. Bioclimatología de Chile. Santiago de Chile: Editorial Universidad Católica de Chile.
- Dieckman M, Kühne A, Isermann M. 2007. Random vs non-random sampling: effects on patterns of species abundance, species richness and vegetation-environment relationships. Folia Geobot. 42:179–190. doi: 10.1007/BF02893884
- Diem J, Lichtenstein J. 1959. Las Himenofiláceas del área argentino-chilena del sud. Darwiniana. 11:611–760.
- Domínguez F, Rebelo A, Bitmann R. 2012. How plant inventories improve future monitoring. Biodiversity Conservation. 21:1937–1951. doi: 10.1007/s10531-012-0286-2
- ESRI. 2011. ArcGIS desktop: release 10. Redlands, CA: Environmental Systems Research Institute.
- Ezcurra C, Brion C. 2005. Plantas del Nahuel Huapi. Catálogo de flora vascular del Parque Nacional Nahuel Huapi. Bariloche, Argentina: Universidad Nacional del Comahue.
- Flores-Palacios A, García-Franco J. 2001. Sampling methods for vascular epiphytes: their effectiveness in recording species richness and frequency. Selvyana. 22:181–191.
- Funk V. 2006. Floras: a model for biodiversity studies or a thing of the past? Taxon. 55:581–588. doi: 10.2307/25065635
- Gianoli E, Saldaña A, Jiménez-Castillo M, Valladares F. 2010. Distribution and abundance of vines along the light gradient in a southern temperate rain forest. J Veg Sci. 21:66–73. doi: 10.1111/j.1654-1103.2009.01124.x
- Gissi N. 2004. Segregación espacial mapuche en la ciudad: ¿Negación o revitalización identitaria. Revista de Urbanismo, N°9, Departamento de Urbanismo, Universidad de Chile. Santiago de Chile. [cited 2016 July 24]. Available from: http://web.uchile.cl/vignette/revistaurbanismo/CDA/urb_complex/0,1311,SCID%253D6418%2526ISID%253D315%2526IDG%253D2%2526ACT%253D0%2526PRT%253D6416,00.html
- Gotelli N, Colwell R. 2001. Quantifying biodiversity: procedures and pitfalls in the measurement and comparison of species richness. Ecol Lett. 4:379–391. doi: 10.1046/j.1461-0248.2001.00230.x
- Gotelli N, Colwell R. 2011. Frontiers in measuring biodiversity. New York: Oxford University Press. Chapter 4, Estimating species richness; p. 39–54.
- Götzenberger L, de Bello F, Brathen K, Davison J, Dubuis A, Guisan A, Leps J, Lindborg R, Moora M, Partel M, et al. 2012. Ecological assembly rules in plant communities—approaches, patterns and prospects. Biol Rev. 87:111–127. doi: 10.1111/j.1469-185X.2011.00187.x
- Hauenstein E, Peña-Cortés F, Bertrán C, Tapia J, Vargas-Chacoff L, Urrutia O. 2014. Composición florística y evaluación de la degradación del bosque pantanoso costero de temu-pitra en la Región de La Araucanía, Chile. Gayana Bot. 71:43–57. doi: 10.4067/S0717-66432014000100008
- Hermoso V, Kennard M, Linke S. 2013. Data acquisition for conservation assessments: is the effort worth it? Plos One. 8:e59662. doi:10.1371/journal.pone.0059662
- Hortal J, Borges P, Gaspar C. 2006. Evaluating the performance of species richness estimators: sensitivity to sample grain size. J Anim Ecol. 75:274–287. doi: 10.1111/j.1365-2656.2006.01048.x
- Hortal J, Lobo J, Jiménez-Valverde A. 2007. Limitations of biodiversity databases: case study on seed-plant diversity in Tenerife, Canary Islands. Conserv Biol. 21:853–863. doi: 10.1111/j.1523-1739.2007.00686.x
- IPNI. 2008. The international plant names index [cited 2016 July 24]. Available from: http://www.ipni.org/
- IUCN. 2010. Standards and petitions subcommittee. Guidelines for Using the IUCN Red List Categories and Criteria. Version 8.1. Prepared by the Standards and Petitions Subcommittee in March 2010. [cited 2016 July 24]; Available from: http://www.plants2020.net/document/0175/
- Jiménez-Castillo M, Wiser S, Lusk C. 2007. Elevation parallels of latitudinal variation in the proportion of lianas in woody floras. J Biogeogr. 34:163–168. doi: 10.1111/j.1365-2699.2006.01570.x
- Jiménez-Valverde A, Hortal J. 2003. Las curvas de acumulación de especies y la necesidad de evaluar la calidad de los inventarios biológicos. Revista Ibérica de Aracnología. 8:151–161.
- Kenkel N, Juhász-Nagy P, Podani J. 1989. On sampling procedures in population and community ecology. Vegetatio. 83:195–207. doi: 10.1007/BF00031692
- Lambeck R. 1997. Focal species: a multi-species umbrella for nature conservation. Conserv Biol. 11:849–856. doi: 10.1046/j.1523-1739.1997.96319.x
- Larsen C, Ponce M, Scataglini A. 2014. Revisión de las especies de Hymenophyllum (Hymenophyllaceae) del sur de Argentina y Chile. Gayana Bot. 70:274–329.
- MacArthur R, Wilson E. 1967. The theory of island biogeography. Princeton: Princeton University Press.
- Magurran A, Handerson P. 2003. Explaining the excess of rare species in natural species abundance distributions. Nature. 422:714–716. doi: 10.1038/nature01547
- Marticorena A, Alarcón D, Abello L, Atala C. 2010. Plantas trepadoras, epifitas y parásitas nativas de Chile: Guía de Campo. Concepción: Ediciones Corporación Chilena de la Madera.
- Marticorena C, Rodríguez R. 1995. Flora de Chile. Vol I Pteridophyta-Gymnospermae. Concepción: Universidad de Concepción.
- Martínez O. 1985. Plantas trepadoras del bosque chileno. Valdivia: Editorial Alborada.
- Möller P, Muñoz-Pedreros A. 2014. Legal protection assessment of different inland wetlands in Chile. Rev Chil Hist Nat. 87:1–13. doi: 10.1186/s40693-014-0023-1
- Mondragón D. 2011. Guidelines for collecting demographic data for population dynamics studies on vascular epiphytes. J Torrey Bot Soc. 138:327–335. doi: 10.3159/TORREY-D-11-00025.1
- Muñoz A, Chacon P, Perez F, Barnert E, Armesto J. 2003. Diversity and host tree preferences of vascular epiphytes and vines in a temperate rainforest in southern Chile. Aust J Bot. 51:381–391. doi: 10.1071/BT02070
- Murcia C. 1995. Edge effects in fragmented forests: implications for conservation. Trends Ecol Evol. 10:58–62. doi: 10.1016/S0169-5347(00)88977-6
- O’Hara R. 2005. Species richness estimators: how many species can dance on the head of a pin? J Anim Ecol. 74:375–386. doi: 10.1111/j.1365-2656.2005.00940.x
- Parra M, Acuña K, Corcuera L, Saldaña A. 2009. Vertical distribution of Hymenophyllaceae species among host tree microhabitats in a temperate rain forest in Southern Chile. J Veg Sci. 20:588–595. doi: 10.1111/j.1654-1103.2009.01078.x
- Peña-Cortés F, Escalona-Ulloa M, Rebolledo G, Pincheira-Ulbrich J, Torres Álvarez O. 2009. Efecto de los cambios globales sobre la salud humana y la seguridad alimentaria. Buenos Aires: Published by RED CYTED 406RT0285. Efecto del cambio en el uso del suelo en la economía local: Una perspectiva histórica en el borde costero de La Araucanía, Sur de Chile; p. 184–197.
- Peña-Cortés F, Limpert C, Andrade E, Hauenstein E, Tapia J, Bertrán C, Vargas-Chacoff L. 2014. Dinámica geomorfológica de la costa de La Araucanía. Rev Geogr Norte GD. 58:241–260. doi: 10.4067/S0718-34022014000200013
- Peña-Cortés F, Pincheira-Ulbrich J, Bertrán C, Tapia J, Hauenstein E, Fernández E, Rozas D. 2011. A study of the geographic distribution of swamp forest in the coastal zone of the Araucanía Region, Chile. Appl Geogr. 31:545–555. doi: 10.1016/j.apgeog.2010.11.008
- Pincheira-Ulbrich J. 2011. Patrones de diversidad de plantas trepadoras y epifitas vasculares en el bosque lluvioso valdiviano de Sudamérica: Una síntesis entre los años 2000 y 2010. Phyton-Int J Exp Bot. 80:9–18.
- Pincheira-Ulbrich J, Rau JR, Smith-Ramirez C. 2012. Diversidad de plantas trepadoras y epifitas vasculares en un paisaje agroforestal del sur de Chile: una comparación entre fragmentos de bosque nativo. B Soc Argent Bot. 47:411–426.
- Ramírez C, Ferriere A, Figueroa H. 1983. Estudio fitosociológico de los bosques pantanosos templados del sur de Chile. Rev Chil Hist Nat. 56:11–26.
- Ramírez C, San Martín C, San Martín J. 1996. Ecología de los Bosques nativos de Chile. Santiago de Chile: Editorial Universitaria. Estructura florística de los bosques pantanosos de Chile sur-central; p. 215–234.
- Reyes F, Zanetti S, Espinosa A, Alvear M. 2010. Biochemical properties in vascular epiphytes substrate from a temperate forest of Chile. Rev Cienc Suelo Nutr Vegetal. 10:126–138.
- Rivera-Huntiel A, Bustamante R, Marin V, Medel R. 2012. Effects of sampling completeness on the structure of plant-pollinator networks. Ecology. 93:1593–1603. doi: 10.1890/11-1803.1
- Rodríguez R, Alarcón D, Espejo J. 2009. Helechos nativos del centro y sur de Chile: Guía de campo. Concepción: Editorial Corporación Chilena de la Madera.
- Saldaña A, Parra M, Flores-Bavestrello A, Corcuera L, Bravo L. 2014. Effects of forest successional status on microenvironmental conditions, diversity, and distribution of filmy fern species in a temperate rainforest. Plant Spec Biol. 29:253–262. doi: 10.1111/1442-1984.12020
- San Martín J, Espinosa A, Zanetti S, Hauenstein E, Ojeda N, Arraigada C. 2008. Composición y estructura de la vegetación epifita vascular en un bosque primario de Olivillo (Aextoxicom punctatum R. et P.) en el sur de Chile. Ecología Austral. 18:1–11.
- San Martín J, Troncoso A, Ramírez C. 1988. Estudio fitosociológico de los bosques pantanosos nativos de la Cordillera de la Costa en Chile central. Bosque. 9:17–33. doi: 10.4206/bosque.1988.v9n1-03
- Schnitzer S, Bongers F. 2002. The ecology of lianas and their role in forests. Trends Ecol Evol. 17:223–230. doi: 10.1016/S0169-5347(02)02491-6
- Schnitzer S, Bongers F, Burham R, Putz F. 2015. Ecology of lianas. Chichester: John Wiley and Sons.
- Shen T, Chao A, Lin C. 2003. Predicting the number of new species in further taxonomic sampling. Ecology. 84:798–804. doi: 10.1890/0012-9658(2003)084[0798:PTNONS]2.0.CO;2
- Smith-Ramírez C, Pliscoff P, Teillier S, Barrera E. 2005. Historia, biodiversidad y ecología de los bosques costeros de Chile. Santiago de Chile: Editorial Universitaria. Patrones de riqueza y distribución de la flora vascular en la Cordillera de la Costa de Valdivia Osorno, y Llanquihue; p. 253–277.
- Squeo F, Estades C, Bahamonde N, Cavieres L, Rojas G, Benoit I, Parada E, Fuentes A, Avilés R, Palma A, et al. 2010. Revisión de la clasificación de especies en categorías de amenaza en Chile. Rev Chil Hist Nat. 83:511–529. doi: 10.4067/S0716-078X2010000400006
- Swenson N, Enquist B, Pither J, Kerkhoff A, Boyle B, Weiser M, Elser J, Fagan W, Forero-Montaña J, Fyllas N, et al. 2012. The biogeography and filtering of woody plant functional diversity in North and South America. Global Ecol Biogeogr. 21:798–808. doi: 10.1111/j.1466-8238.2011.00727.x
- Thompson G, Whitters P. 2003. Effect of species richness and relative abundance on the shape of the species accumulation curve. Austral Ecol. 28:355–360. doi: 10.1046/j.1442-9993.2003.01294.x
- UICN. 2001. Categorías y Criterios de la Lista Roja de la UICN: Versión 3.1. Comisión de Supervivencia de Especies de la UICN. Gland, Suiza and Cambridge, Reino Unido. [cited 2016 July 24]. Available from: http://www.sinia.cl/1292/articles-35077_pdf_1.pdf
- Wallington T, Moore S. 2005. Ecology, value and objectivity: advancing the debate. Bioscience. 55:873–878. doi: 10.1641/0006-3568(2005)055[0873:EVAOAT]2.0.CO;2
- Woda C, Huber A, Dohrenbusch A. 2006. Vegetación epífita y captación de neblina en bosques siempreverdes en la Cordillera Pelada, sur de Chile. Bosque. 27:231–240. doi: 10.4067/S0717-92002006000300002
- Wolf J, Gradstein S, Nadkarni N. 2009. A protocol for sampling vascular epiphyte richness and abundance. J Trop Ecol. 25:107–121. doi: 10.1017/S0266467408005786
- Zotz G, Bader M. 2009. Progress in Botany 70. Berlin: Springer. Chapter 7, Epiphytic plants in a changing world-global: change effects on vascular and non-vascular epiphytes; p. 147–170.