Abstract
An important component of quantifying seismic hazard and risk in regions such as Wellington is to characterise both the long-term rate of occurrence of the region's major earthquake-generating active faults, as well as potential interactions between faults (e.g., the potential for earthquake triggering). This paper describes paleoseismic data from two trenches (Ohariu Valley) and a natural streambank exposure (Horokiri valley) c. 24 km apart, which constrain the timing and size of recent surface rupture events on the northeast striking Ohariu Fault c. 6 km northwest of Wellington City. One event is recorded at all three sites and radiocarbon age constraints indicate it correlates with the 1050–1000 cal. years BP event previously identified elsewhere along the fault. A younger, smaller (decimetre-scale) event is recorded in one of the Ohariu Valley trenches, and the timing is constrained by two radiocarbon ages to post-310 cal. years BP. This event may either be a small, primary Ohariu Fault rupture, or a triggered event associated with a large earthquake on a nearby fault. If it is a triggered rupture, then a possible mechanism is dynamic triggering associated with one of the recent large-great earthquakes on the Wellington (post-300 yr), Wairarapa (AD1855), or Awatere (AD1848) Faults. Small rupture events do not necessarily contribute to the recurrence interval classification of the Ministry for the Environment Active Fault Guidelines, but they could be important for sensitive structures crossing the fault and for lifelines crossing multiple active faults.
Introduction
The Ohariu Fault is one of a series of major active dextral strike-slip faults in the Wellington region (A), which together account for c. 70% of the boundary-parallel Pacific-Australian plate motion (Van Dissen & Berryman Citation1996; Little et al. Citation2009). The Ohariu Fault, like many of the Wellington region's active faults, is located near major population centres (A) and is traversed by several major lifelines. It is therefore vitally important to characterise the seismic hazard and risk of these faults, both in terms of strong ground shaking (e.g., Stirling et al. Citation2002) and ground surface rupture hazard (e.g., Kerr et al. Citation2003).
Fig. 1 (A) Location map showing the Ohariu Fault (bold) in relation to other active faults in the Wellington region. The inset shows the major features of the New Zealand plate boundary and the relative Pacific-Australian plate motion vectors along the Hikurangi Trough (labelled in mm/yr), after Nicol & Wallace (Citation2007). Active faults (on-land only; black lines) are from the New Zealand Active Faults Database (http://data.gns.cri.nz/af/index.jsp) (AkF: Akatarawa Fault, AwaF: Awatere Fault, NOF: Northern Ohariu Fault, PkF: Pukerua Fault, SGF: Shepherds Gully Fault, VF: Vernon Fault, WairF: Wairarapa Fault, WF: Wairau Fault, WgtnF: Wellington Fault, W: Wellington City). (B) Detailed map of the Ohariu Fault (black line) and nearby active faults of the Wellington region (white lines), from the same data source as (A). The three sites described in this study are labelled in bold, and the remainder are sites where radiocarbon ages constraining Ohariu Fault surface rupture events have been obtained (Heron et al. Citation1998; Litchfield et al. Citation2004, Citation2006; Cochran et al. Citation2007). Black open circles are the locations of major population centres. Grid references are in New Zealand Map Grid 1949 projection.
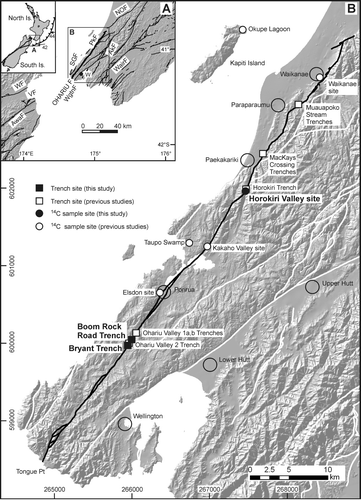
Another important characteristic to consider for seismic hazard and risk studies is the potential for fault interactions. Active faults in the Wellington region are relatively closely spaced; at its southern end, the Ohariu Fault is within 3–4 km of its nearest active neighbours (Shepherds Gully Fault and Wellington-Hutt Valley segment of the Wellington Fault; ). There are also major active faults along-strike to the southwest, namely the dextral strike-slip and oblique-dextral normal faults in Cook Strait (Barnes & Audru Citation1999; Barnes et al. Citation2008), and to the northeast the dextral strike-slip faults comprising the North Island Dextral Fault Belt (Beanland Citation1995; Langridge et al. Citation2007). Thus, there is considerable potential for both static (e.g., Hodgkinson et al. Citation1996; Stein et al. Citation1997) and dynamic (e.g., Hill et al. Citation1993; Gomberg et al. Citation2004) earthquake triggering.
Studies of the potential interaction between faults of the Wellington region has predominantly been through the construction of synthetic seismicity models incorporating static triggering and shadowing (Robinson & Benites Citation1996; Robinson et al. Citation2009), but there has been little documentation of field evidence for triggered fault ruptures. The possible exception is an uncharacteristically small surface rupture event on the Akatarawa Fault (located on A) which has not been dated, but is tentatively interpreted to represent triggered-slip resulting from rupture of the nearby Wellington Fault (Van Dissen et al. Citation2001).
In this study we present results from two new trenches and one natural stream bank exposure along the Ohariu Fault, undertaken to better constrain seismic hazard and risk. Radiocarbon dating of faulted units at all three sites confirms the distribution and timing of a previously recognised rupture event c. 1000 years ago, but one trench also provides strong evidence for a small younger event <300 years ago. We discuss possible mechanisms for the post-300 year BP event, including triggered rupture associated with a large earthquake on one of the nearby active faults. We also discuss the significance of a small event for seismic hazard in the Wellington region.
Ohariu fault: Previous work
The Ohariu Fault is a northeast-striking (030–050°) dextral strike-slip fault with an onland length of c. 70 km, extending from near Waikanae in the north to Tongue Point on the Wellington south coast (). It has been traced offshore for a further c. 20 km as a series of discontinuous ENE-striking traces, which terminate between the offshore Wairau and Cloudy (a splay off the Vernon-Awatere Faults; A) Faults (Barnes et al. Citation2008). It may also continue northward from Waikanae for an additional c. 60 km as the Northern Ohariu Fault (Palmer & Van Dissen Citation2002; A).
The Ohariu Fault slip rate has only been determined onland. A horizontal dextral slip rate of 1–2 mm yr−1 was calculated from nine sites and a vertical slip rate of >0.06 mm yr−1 was calculated from two sites (Heron et al. Citation1998). Likewise, the horizontal single-event surface rupture displacement has been determined from the most recent event offset at seven onland sites. It is 3–5 m, with a mean of 3.7 m (Heron et al. Citation1998).
The timing of the most recent surface rupture event has previously been constrained at three trench sites (Mauapoko Stream, Horokiri, Ohariu Valley; B) and two terrace sites (Waikanae, Kakaho Valley; B) and has been tightly constrained to 1050–1000 cal. years BP (Heron et al. Citation1998; Litchfield et al. Citation2004). Cochran et al. (Citation2007) also identified a coseismic subsidence event in nearby water bodies (Taupo Swamp and Okupe Lagoon on Kapiti Island; B) at approximately this time, which they interpreted to (likely) be the result of an Ohariu Fault earthquake.
Dating of older events has proved problematic and, as a result, so has the constraint of the recurrence interval. Litchfield et al. (Citation2006) dated two events at 5170–4410 and 4810–3260 cal. years BP from a trench at MacKays Crossing (B). Heron et al. (Citation1998) tentatively dated a younger event at 2470–2330 cal. years BP from a trench in the Ohariu Valley (Ohariu Valley 2; B). Cochran et al. (Citation2007) identified three coseismic subsidence events which broadly correspond in time with these poorly dated events. Using statistical analysis of multiple parameters (inter-event times from the MacKays Crossing trench, mean slip rate, single-event displacement, and their uncertainties), Litchfield et al. (Citation2006) calculated a mean recurrence interval of 2200 years, with minimum and maximum 68% and 95% confidence interval limits of 1300 and 3800 years, and 800 and 7000 years, respectively.
Paleoseismology sites and radiocarbon dating
The two trenches presented in this study (Boom Rock Road and Bryant) were located across a relatively straight section of the Ohariu Fault in Ohariu Valley, near three previous trenches (Ohariu Valley 1a, 1b, and 2) of Heron et al. (Citation1998) (B). The trenches were oriented normal to the fault scarp and were 10–15 m long, a maximum of 4–5 m deep, and were benched for safety reasons. The trenches were analysed using standard paleoseismic methods; walls were cleaned with hand tools, a 1 m string grid was constructed, units and faults were marked with coloured flags, and a log drawn at 1:20 scale. Summary trench logs are presented in and , and unit descriptions are presented in the Appendix.
Fig. 2 (A) Oblique aerial view of the Boom Rock Road trench site in the Ohariu Valley (view to the southeast). Yellow arrows denote the surface trace of the Ohariu Fault. (Photo: Lloyd Homer, GNS Science, CN 17409a) (B) Photograph of the northeast wall of the Boom Rock Road trench. Major faults (bold red lines) and the top of the greywacke bedrock (thin black line) are delineated. (C) Summary trench log of the northeast wall of the Boom Rock Road trench (T06/1). Unit numbers are for descriptive purposes only and field descriptions are given in the Appendix. Details of radiocarbon ages are contained in . Brown-coloured units are interpreted to be correlatives across the fault and to be fluvial terrace deposits (see text for further discussion). Grid numbers on the left-hand side and along the base are spaced 1 m apart.
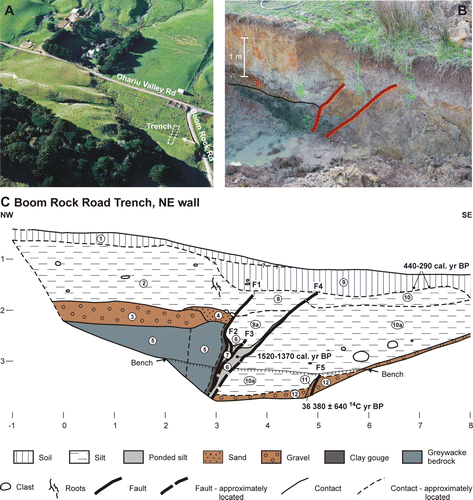
Fig. 3 (A) The Bryant trench site in the Ohariu Valley (view to the southeast). Yellow arrows denote the surface trace of the Ohariu Fault. (B) Photograph of the southwest wall of the Bryant trench showing the major faults (bold red lines, dashed across the bench). (C) Summary trench log of the southwest wall of the Bryant trench (T08/1). Unit numbers, colours, radiocarbon ages, and grid numbers are as described in the caption for C. (D) Photograph of the northeast wall of the Bryant trench. Major faults (bold red lines) and key contacts (thin black lines) are shown. (E) Summary trench log of the northeast wall of the Bryant trench (T08/1). Unit numbers, colours, radiocarbon ages, and grid numbers are as described in the caption for C.
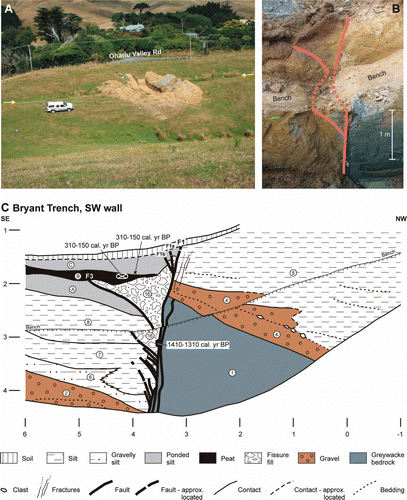
A radiocarbon sample was collected from a natural stream bank exposure of the Ohariu Fault in a small tributary of Horokiri Stream (Horokiri valley site in B), described further below. The collection of the sample was opportunistic during fieldwork for other purposes, and the stream bank exposure was not fully described or analysed.
All radiocarbon samples were analysed by Rafter Radiocarbon Laboratory. The materials dated were plant material, organic silt, and charcoal, as described in the text and in . The calibrations for all of the young (<2000 years BP) radiocarbon ages are as reported by Rafter Radiocarbon Laboratory and are described in . Ages for these samples are quoted in calibrated years before present (cal. years BP) at the 95% confidence interval. The remaining radiocarbon ages are considered too old (>20,000 years) to be reliably calibrated and so are quoted in conventional radiocarbon years before present (14C year BP).
Table 1 Radiocarbon ages
Trench and site descriptions
Boom Rock Road trench (T06/1)
The Boom Rock Road trench (grid reference: NZMS 260 R27/599004) was located between the Ohariu Valley 1 and 2 trenches of Heron et al. (Citation1998), adjacent to (and north of) Boom Rock Road (B, A). The trench was excavated across a c. 1 m high southeast-facing scarp, against which drainage is ponded to form a swamp (A). Unfortunately, because of the high water content in the southeast half (downthrown side) of the trench, the trench partially collapsed (B). Only one wall (northeast) was therefore logged (parts of it not in great detail).
The Boom Rock Road summary trench log is shown in C. The trench exposed massive crushed greywacke (unit 5) on the upthrown (northwest) side of the fault, which is overlain by and/or faulted against gravel, sand, and silt (units 2–4, 6–8, 10–12). These units are all overlain by unfaulted silty subsoil (unit 9) and topsoil (unit 1). The greywacke (unit 5) is interpreted to be Triassic (Rakaia Terrane) Torlesse Supergroup basement, which underlies the hillslopes around the trench site (Begg & Johnston Citation2000). The subhorizontal surface on the greywacke basement is interpreted to be a fluvial strath. The overlying pebble-cobble gravel (unit 3) and sand (unit 4) units, along with their inferred correlative on the downthrown side (unit 12), are therefore interpreted to be fluvial terrace deposits. We interpret the cutting of this strath and its associated deposits to be from Ohariu Stream, which is currently located west of the trench site. The overlying silt units are considered to be either overbank flood deposits from Ohariu Stream and/or from the small tributaries draining the east side of the valley, or possibly loess. In addition, some units such as 6 and 11 (which are both organic-rich and found within the shear zones of the fault) are interpreted to have formed by ponding of drainage against the fault scarp.
Two zones of faulting were exposed (C). The main fault zone is 2 m wide and comprises a series of upward-branching and anastamosing faults (F1–F4). These faults all dip steeply northwest and, where units can be correlated across them (e.g., units 3 with 12 across zone F1 to F5 and unit 6 within the fault zone), show apparent reverse displacements. Two faults (F1 and F4) extend high in the trench into silt units 2, 8, and 10, which are considered partial correlatives of one another. These faults do not appear to offset the subsoil (unit 9), which has an age of 440–290 cal. years BP (BRR-3 in ), indicating the most recent fault rupture occurred before 290 cal. years BP. Faults F2 and F3 branch off F1; F2 rejoins it, whereas F3 terminates within unit 8a. Faults F3 and F4 displace and deform unit 6, which has been dated at 1520–1370 cal. years BP (BRR-1 in ), indicating that there has been at least one fault rupture event since 1520 cal. years BP.
Another fault (F5) was exposed at a low level in the trench (C). Like the main fault zone it is also steeply (80°) northwest-dipping, but shows an apparent normal displacement (e.g., the top of unit 12) thus giving the appearance of a small graben between F5 and the other faults. F5 cuts units 11 and 12, and it could not be traced any higher within unit 10a. Unit 11 does not have a correlative on the northwest side of the trench, so is considered to have either been dragged up from within or beneath the gravel (unit 12), or is from a correlative unit along-strike (i.e., its correlative is not laterally continuous, but is locally preserved on the northwest side of the fault). It has an age of 36 380±640 14C years BP (BRR-2 in ). This is considerably older than the ages from stratigraphically higher units in the trench, suggesting a major unconformity exists. Regardless of exactly where the unconformity occurs in the trench deposits, the c. 1.5 m throw (across F1–F5) on the top of unit 3 = 12 could be the result of several Ohariu Fault rupture events. This interpretation is also supported by the variability in scarp height at the site, suggesting it is the result of multiple events.
Bryant trench (T08/1)
The Bryant trench (grid reference: NZMS 260 R27/595997) was located c. 350 m southwest of the Ohariu Valley 2 trench of Heron et al. (Citation1998) and c. 900 m southwest of the Boom Rock Road trench, adjacent to Ohariu Valley Road (B, A). The trench was excavated across a c. 1 m high southeast-facing scarp against which there is some minor ponding of drainage (A). Both walls were logged in this trench (C, E) and, where possible, the same notation was given to units and faults in both walls. Units A, B, and C in the southwest wall are tentatively correlated with units 15, 17, and 18 in the northeast wall.
Greywacke (unit 1) was exposed on the upthrown side of the fault, and is overlain and/or faulted against gravel, sand, and silt (units 2–9, 13–19, A–C) and soil (units 10 and 11). Peat (unit B), fissure fill (unit 16), and fault pug (units 12 and 13) were also exposed in the trench. The general interpretation of the stratigraphy is similar to the Boom Rock Road trench; i.e., Torlesse Supergroup (Rakaia Terrane; unit 1) basement is overlain by fluvial terrace deposits (units 2, 3, and 4, which are inferred to be correlatives across the fault), resting on a fluvial strath. The terrace deposits are in turn overlain by silt units (5–9), which may be overbank silt or loess, capped on the downthrown side by ponded organic silt (14, 15, 17, 18, 19 A, C) and peat (B) units. Units in the trench dip gently northwest, which may reflect a combination of derivation from the small tributaries draining from the southeast side of the valley (e.g., units 5–9 may be alluvial fan deposits with a primary dip), erosion and infilling of valley and fault topography along the scarp, and possibly tectonic tilting. For the latter, the steep dip and localised warping close to the fault (e.g., only the northwest end of unit 2 appears to be dragged downwards) suggests any rotation could be relatively superficial, reflecting local twisting of a small block along a classic strike-slip mole track structure.
A zone of faulting up to 1 m wide that consists of a main fault plane (F1) and, particularly in the southwest wall, numerous splay faults was exposed in this trench. There are also faults within the greywacke basement (mainly mapped on the northeast wall) but, because only one of these faults (F2) offsets overlying units (and only by a few centimetres), these faults are not discussed further. F1 dips very steeply (85°) southeast and overall the sense of vertical movement is apparently normal (e.g., the tops of correlative fluvial terrace units 4 and 2).
F1 extends high into the trench, either close to (northeast wall), or to (southwest wall) the base of the topsoil. The youngest unit displaced by F1 (unit C (southwest wall) = 18? (northeast wall)) has an age of 310–150 cal. years BP (Bryant 08/7 in ), which is identical (within analysis uncertainties) to the age of the next lowest unit (unit B (SW wall) = 17? (NE wall); Bryant 08/6 in ). Together these ages indicate that the most recent rupture event on F1 was post-310 cal. years BP. F1 and some small splays in the southwest wall (F1a and F1b) also displace the top of the fissure fill (unit 16). This indicates that the fissure was formed before the most recent event on F1. The total vertical offset of the top of unit 16 cannot be measured because it is not present on the upthrown side of F1, but the offset across F1a and F1b is 0.12 m.
The other faults in the trench splay upwards from F1. On the southwest wall there are several splays which terminate upwards within silt units at various depths; notably, several also displace the fissure fill unit 16. The splay with the most displacement is F3, which can be correlated between both walls and is the main fault bounding the southeast side of the fissure fill. F3 terminates upwards at the base of units B (southwest wall) and 17 (northeast wall), which are tentatively correlated. The most recent movement on F3 was therefore before 310–150 cal. years BP. From within the fissure fill (unit 16), an age of 1410–1310 cal. years BP (Bryant 08/8 in ) was obtained from a block considered to be soil, which we interpret was forming prior to the fault rupture event and subsequently fell into the fissure. These two ages indicate that the penultimate rupture event at this site was between 1410 and 150 cal. years BP, and resulted in the formation of a prominent (up to 1.5 m wide and 2.2 m deep) fissure.
The oldest age obtained from this trench is 30,510±320 14C years BP (Bryant 08/4 in ) from an organic silt (unit 3) which appears to have filled a channel cut into gravel unit 2. This suggests that, like the Boom Rock Road trench, there is a major unconformity in the trench stratigraphy and that the total vertical offset on the top of the fluvial terrace deposits (c. 2.1 m) is most likely the result of many Ohariu Fault rupture events.
Horokiri valley site
The Horokiri valley site is a natural stream bank exposure in a small tributary of Horikiri Stream, c. 250 m south of the Horokiri trench of Heron et al. (Citation1998) (). The stream bank exposes the main strand of the Ohariu Fault and a sample of faulted peat (TMG#1 in ) was collected from <2 m below the ground surface. This peat yielded an age of 1290–1180 cal. years BP, which indicates that the fault rupture has occurred since 1290 cal. years BP. However, no other information is available to determine if there are any younger events at this site.
Discussion
The stratigraphy and faulting history recorded in the Boom Rock Road and Bryant trenches are generally consistent with each other and with the previous Ohariu Valley trenches of Heron et al. (Citation1998). The timing of at least one of the fault rupture events is also consistent with that dated at the Horokiri valley site. In this section, we first combine the results from all of the Ohariu Valley trenches and then compare these with the timing of events dated at other sites. We then discuss in some detail the post-300 year event recorded in the Bryant trench and its significance in terms of seismic hazard.
Ohariu Valley trenches
The Ohariu Valley trenches all revealed a fault bounded block of greywacke basement, upthrown to the northwest, overlain by and/or faulted against predominantly alluvial sediments which generally fine upwards. The dip and dip direction of the fault varies between trenches but, overall, the dips are steep indicating that the variability most likely reflects a shallow structure along the predominantly dextral fault.
The sediments in the trenches have broadly similar ages and all record a major unconformity somewhere in the lower half of the exposed stratigraphy. The oldest ages obtained are >45,000 (Ohariu Valley 1a), >45,000 (Ohariu Valley 1b), 36,380±640 (Boom Rock Road), 29,474±380 (Ohariu Valley 2), and 30,510±320 (Bryant) 14C years BP. These ages suggest that the fluvial terrace deposits resting on the bedrock strath cut were deposited prior to the Last Glacial Coldest Period (28,000–18,000 cal. years BP; Alloway et al. Citation2007). They are probably correlatives of the Rata (c. 40,000–30,000 cal. years BP) and/or possibly Porewa (c. 70,000–55,000 cal. years BP) aggradation terraces described elsewhere in the lower North Island (e.g., Milne Citation1973a, Citationb; Berryman Citation1990; Formento-Trigilio et al. Citation2003; Litchfield & Berryman Citation2005).
The next oldest age in any of the Ohariu Valley trenches is 2470–2330 cal. years BP, from a buried forest layer in Ohariu Valley 2 trench (). The burial of this forest horizon was interpreted by Heron et al. (Citation1998) to be the result of a fault rupture event. A buried forest horizon and a rupture event of this age have not been identified in any of the other trenches in the Ohariu Valley or in the only trench with dated older events, at MacKays Crossing c. 30 km to the northeast (Litchfield et al. Citation2006). Thus, despite the fact that it coincides with the timing of a coseismic subsidence event in nearby water bodies (Cochran et al. Citation2007), the nature of this event is still unknown. Alternatively, the forest burial could have been the result of a non-tectonic event such as a flood or a landslide, or an earthquake event unrelated to rupture of the Ohariu Fault.
Fig. 5 Radiocarbon ages which constrain the timing of recent (post-2500 cal. years BP) events on the Ohariu Fault. The ages presented in this study are shown in bold (BRR = Boom Rock Road, HV = Horokiri valley). Those from the Ohariu 1a, 1b, 2, Kakaho, Horokiri, and Waikanae sites are from Heron et al. (Citation1998) and were recalibrated by Litchfield et al. (Citation2004). Those from the Muaupoko site are from Litchfield et al. (Citation2004). The age from the Elsdon site is from Grant-Taylor (Citation1974), calibrated by Rafter Radiocarbon Laboratory as described in the text. The dashed line and arrows indicate whether the sample is a minimum or maximum age constraint. The shaded grey boxes are (top to bottom) the best estimate for the timing of the small, possibly triggered, surface rupture event identified in the Bryant Trench (310–150 cal. years BP), the most recent major surface rupturing event along the Ohariu Fault (1050–1000 cal. years BP), and the possible event recorded by the buried forest in the Ohariu 2 Trench (post-2470 cal. years BP).
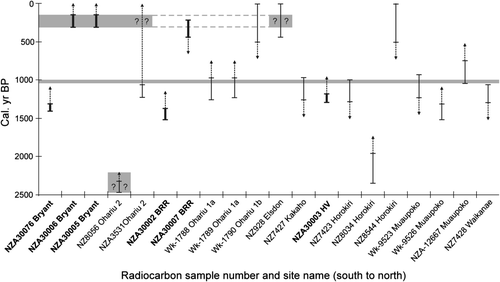
The remaining ages in the Ohariu Valley trenches are all <2000 cal. years BP and all constrain the timing of young fault rupture events (). In the Ohariu Valley 1a and 1b trenches, the most recent event was bracketed between 1130 and 150 cal. years BP. In the Boom Rock Road trench, we have bracketed the most recent event to between 1520 and 290 cal. years BP. Heron et al. (Citation1998) were not able to constrain the timing of the most recent event in the Ohariu Valley 2 trench. They dismissed a radiocarbon age of 1220–1060 cal. years BP () from beneath three colluvial wedges as being too young; we discuss this age further below. In the Bryant trench we dated two fault rupture events, one between 1410 and 310 cal. years BP and a younger one post-310 cal. years BP.
We interpret the most recent surface rupture events in the Ohariu Valley 1a, Ohariu Valley 1b, and Boom Rock Road trenches and the penultimate event on the Bryant trench to be the same event (). Furthermore, we consider it to be the same event as one dated in several trenches further northeast along the Ohariu Fault (Heron et al. Citation1998; Litchfield et al. Citation2004) and also the event in which the peat at the Horokiri valley site was faulted (). Litchfield et al. (Citation2004) combined all the available ages to tightly constrain the timing of this event to 1050–1000 cal. years BP. The new ages presented above are consistent with the timing of this event () but do not provide any tighter age constraints.
Post-300 year event
Given that previous study of the Ohariu Fault has estimated the last surface rupture event at c. 1000 cal. years BP, the young event recorded in the Bryant trench was unexpected. Reassessing the previously published data, however, it is possible that there are at least two other locations where a young rupture event has also been recorded on the Ohariu Fault but was overlooked or dismissed. As noted above, the Ohariu Valley 2 Trench (c. 360 m northeast of the Bryant Trench) exposed three colluvial wedges interpreted to represent fault rupture events but an age of 1220–1060 cal. years BP from beneath these wedges was dismissed as being too young (Heron et al. Citation1998). This was partly justified by the fact that the sample comprised roots, and thus may have been from trees significantly younger than the unit in which they were collected (Heron et al. Citation1998). However, we note that it is stratigraphically consistent with (i.e., younger than) the age of the buried forest horizon below it. The three wedges are also relatively small and only the lower one is significantly faulted suggesting that, similar to the post-300 year event in the Bryant trench, the two most recent events recorded in the Ohariu Valley 2 trench were probably small.
Grant-Taylor (Citation1974) reported a radiocarbon age of 251 or 196±55 14C year BP from a faulted charcoal-bearing alluvial fan near Porirua (Elsdon site on B). Rafter Radiocarbon Laboratory have recalculated this age to 246±47 14C years BP and provided a calibrated age of 437–0 cal. years BP (). Williams (Citation1975) described this site as being 200 m west of the Ohariu Fault, and suggested that “the charcoal layer is presumed to have been displaced by secondary faulting”. Although the interpretation of the young events at both of these sites remains speculative, it is intriguing that three sites along the southern half of the fault may record a young event (i.e., post-300 year BP); this certainly warrants further investigation.
We consider that there are two possible explanations for the young rupture event identified in the Bryant trench (and possibly the other two sites). Either (1) there is an Ohariu Fault earthquake event that is younger than the ‘most recent event’ constrained by Heron et al. (Citation1998) and Litchfield et al. (Citation2004) at 1050–1000 cal. years BP, or (2) the post-300 year event is a sympathetic or triggered rupture associated with the rupture of another nearby fault. We do not think that the observed displacements can be explained as a secondary effect as, unlike the Boom Rock Road trench, the sediments on the downthrown side of the fault in the Bryant Trench are stiff to hard and therefore not as susceptible to compaction due to strong ground shaking.
If the young event is a primary Ohariu Fault rupture (i.e., explanation (1) above), then it was likely a smaller magnitude earthquake than the c. 1000 cal. years BP earthquake. Although we could not measure the total throw (nor dextral slip displacement), the vertical offset of the top of the fissure fill unit 16 across faults F1a and F2a is only a few centimetres to a few tens of centimetres. This is consistent with the observation that the fissure is still relatively intact, suggesting there was also no significant strike-slip displacement. In comparison, the penultimate event resulted in the creation of the prominent fissure and ponding against the scarp, suggesting that the displacement was of the order of metres. If the post-300 year decimetre-scale displacement was the result of an earthquake on the Ohariu Fault, then this earthquake was probably of moderate magnitude and just above the threshold to produce ground surface displacement. The possible occurrence of a moderate-sized earthquake on the Ohariu Fault could suggest that it does not always behave characteristically (Schwartz & Coppersmith Citation1984), but may instead exhibit some other behaviour such as Gutenberg-Richter (e.g., Wesnousky Citation1994; Stirling et al. Citation1996; Hamiel et al. Citation2009).
The second explanation is that the post-300 year event was a triggered or sympathetic rupture associated with the rupture of another nearby fault. The small displacement described above may also support this explanation and we explore the potential and mechanisms for triggering in the following section.
Triggered rupture?
If the post-300 year BP event does represent a triggered rupture of the Ohariu Fault during primary rupture along a nearby fault, it is interesting to speculate about possible candidate faults. shows a recent compilation of the timing of fault ruptures on a number of central New Zealand active faults (Van Dissen et al. Citation2009). This figure shows that there are at least three faults which have ruptured in the last c. 300 years: the Wellington-Hutt Valley segment of the Wellington Fault (Van Dissen et al. Citation1992; Langridge et al. Citation2009), the Awatere Fault (AD1848; Grapes et al. Citation1998; Benson et al. Citation2001; Mason & Little Citation2006), and the Wairarapa Fault (AD1855; Grapes & Downes Citation1997; Rodgers & Little Citation2006; Little et al. Citation2009). Regarding the latter two historical events, the Ohariu Valley appears to have been settled at around AD1860 when the Bryant and Best families built homesteads there. It is therefore likely that a small rupture on the Ohariu Fault in 1848 or 1855 would not have been witnessed by Europeans; accordingly, Eiby (Citation1980) and Grapes and Downes (Citation1997) do not report damage or rupture anywhere along the Ohariu Fault as a result of either of these events. This suggests that c. AD1860 is probably a minimum age for this event ().
Fig. 6 Comparison of the timing of Ohariu Fault surface rupture events with those on other major central New Zealand active faults. Modified from Van Dissen et al. (Citation2009), compiling data from Little et al. (Citation2009), McSaveney et al. (Citation2006), Cochran et al. (Citation2007) (Wairarapa Fault), Langridge et al. (Citation2009) (Wellington Fault), Barnes et al. (Citation2008), Pondard et al. (Citation2007) (Wairau Fault), and Benson et al. (Citation2001) and Mason et al. (Citation2006) (Awatere Fault). Trapezoidal shapes are schematic interpretations of the best estimate (2σ) probability distribution for the timing of events which are constrained by few radiocarbon dates. Note that the post-300 year Ohariu Fault event identified in this study coincides with the timing of events on the Wairarapa, Wellington, and Awatere Faults.
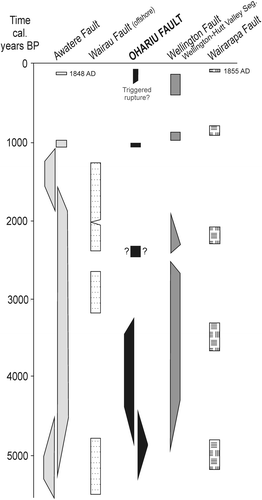
We do not know the mechanism (e.g., static or dynamic triggering) which could have triggered a small rupture on the Ohariu Fault. However, synthetic seismicity modelling suggests that static triggering is unlikely; the Wellington and Wairarapa Faults are parallel strike-slip faults which would tend to unload the Ohariu Fault as a result of their rupture (Robinson & Benites Citation1996). Preliminary modelling of the new Cook Strait fault dataset (Barnes et al. Citation2008) also suggests that the Awatere Fault does not cause static triggering of the Ohariu Fault (personal communication from R. Robinson, 2009). This may be because the Awatere Fault is too far away from the Ohariu Fault and/or that loading is instead transferred onto the intervening Cook Strait faults (Cloudy and Vernon; Barnes et al. Citation2008).
Dynamic triggering is generally considered to be the result of oscillatory dynamic deformations associated with radiating seismic waves (e.g., Gomberg et al. Citation2001), but the details of this process are not well understood. One of the first well-documented historical examples is the 1992 Mw7.3 Landers earthquake, which triggered seismicity up to 1250 km from the mainshock (Hill et al. Citation1993; Anderson et al. Citation1994). As well as the primary rupture on five major dextral faults (e.g., Sieh et al. Citation1993), the Landers earthquake also triggered minor ground surface rupture on numerous other Southern California faults (Hart et al. Citation1993; Bodin et al. Citation1994; Rymer Citation2000). These triggered ruptures were small (≤ 10 cm offset), and occurred on short, isolated zones up to 80 km away from the primary ruptures.
A historical New Zealand earthquake which resulted in triggered ground surface rupture is the 1987 Mw6.3 Edgecumbe earthquake, which ruptured five faults over an area c. 15×7 km (Beanland et al. Citation1989). The largest displacements (≤ 2.5 m vertical) occurred on the Edgecumbe Fault, which is considered to be the primary fault rupture. Rupture on some of the other faults such as the Rotoitipakau Fault Zone are interpreted to be triggered slip, and displacements were significantly smaller than previous ‘primary’ ruptures identified in trenches on those faults (Berryman et al. Citation1998).
Based on such examples, we suggest that if the small post-300 year event on the Ohariu Fault is a sympathetic or triggered rupture then a possible mechanism is dynamic triggering associated with one or more of the large recent earthquakes on the Wellington, Wairarapa, or Awatere Faults. Intriguingly, Grapes and Downes (Citation1997) do report some possible evidence during the AD1855 Wairarapa Earthquake for rupture on the Awatere and Wellington Faults, suggesting it is possible that this large magnitude (Mw8.1–8.2) earthquake caused some dynamic triggering on other faults.
Implications for seismic hazard
Regardless of whether the small post-300 year event on the Ohariu Fault is a triggered rupture or not, it is important to consider the significance of such events in terms of seismic hazard and risk for the Wellington region. The Ministry for the Environment Active Fault guidelines for development of land on or close to active faults (Kerr et al. Citation2003) are primarily designed to assess levels of life safety risk, particularly that induced by building collapse as a result of fault ground surface rupture. As such, the recurrence interval classes (the basis for assessing this risk) were defined based upon metre-scale fault ruptures (i.e., those ruptures that are large enough to be considered likely to pose a life safety, collapse, hazard to structures sited across the rupture). As rupture displacement get smaller, life safety hazard diminishes. Therefore, small events such as the decimetre-offset observed in the Bryant Trench would not alter the recurrence interval classification for the Ohariu Fault, which is currently assessed as Recurrence Interval Class II with medium to low confidence of classification (Litchfield et al. Citation2006).
This is not to say, however, that the seismic hazard and risk from such events can be ignored. Decimetre-scale rupture would significantly damage sensitive structures built directly across the fault (e.g., brittle pipelines). The possibility of triggered ruptures in the Wellington region is also an important consideration for lifelines which cross multiple active faults. For example, following a large earthquake it may be necessary to consider repairs to sections of lifelines not just at the primary fault rupture but also at ruptures that may have been triggered on other active faults. From a design perspective, the results of this study suggest that it may be prudent to design lifelines with some minimum level of resistance at all active fault crossings, regardless of the recurrence interval of metre-scale displacements for those faults.
Conclusions
Two new trenches and a natural streambank exposure of the Ohariu Fault have provided further constraints on the timing of recent ground surface rupture events. The timing of the most recent event in the Boom Rock Road trench (Ohariu Valley) coincides with the timing of the penultimate event in the Bryant trench (Ohariu Valley) and the timing of faulting of peat in the streambank exposure (Horokiri valley). It is also correlated with the 1050–1000 cal. years BP event constrained from five other sites along the fault (Heron et al. Citation1998; Litchfield et al. Citation2004).
The presence of a major unconformity in the lower half of the stratigraphy in the Boom Rock Road and Bryant trenches is consistent with previous trenches in Ohariu Valley (Heron et al. Citation1998), and generally precludes the dating of older (pre-2000 cal. years BP) events. Radiocarbon dates from near the base of all these trenches indicate the fluvial terrace deposits resting on the strath cut into greywacke basement are likely correlatives of Rata (c. 40,000–30,000 cal. years BP) and/or possibly Porewa (c. 70,000–55,000 cal. years BP) terraces described elsewhere in the lower North Island.
A young (post-310 cal. years BP) event in the Bryant trench was unexpected but, upon further investigation, possibly correlates with young events previously described at two other locations on the Ohariu Fault (Grant-Taylor Citation1974; Williams Citation1975; Heron et al. Citation1998). This event may either be a small primary (surface rupture threshold) Ohariu Fault event, or a triggered rupture associated with a large earthquake on other nearby faults of the Wellington region. If it is a triggered rupture, then a possible mechanism is dynamic triggering associated with one or more large recent earthquakes on the Wellington (post-300 yr), Wairarapa (AD1855), or Awatere (AD1848) faults.
Regardless of the mechanism of the post-300 year event, a decimetre-scale rupture would not change the recurrence interval classification of the Ohariu Fault but may be important for structures built across the fault. The possibility of triggered rupture may also be important for lifelines crossing multiple active faults.
Acknowledgements
We thank Greater Wellington and the It's Our Fault Project for facilitation of paleoseismic investigation of the trenches and funding from FRST Project CO5X0402 Geological Hazards and Society. John Begg, Mark Stirling, and two anonymous journal reviewers provided constructive reviews.
References
- Alloway , BV , Lowe , DJ , Barrell , DJA , Newnham , RM , Almond , PC , Augustinus , PC , Bertler , NAN , Carter , L , Litchfield , NJ , McGlone , MS , Shulmeister , J , Vandergoes , MJ , Williams , PW and NZ-INTIMATE members . 2007 . Towards a climate event stratigraphy for New Zealand over the past 30 000 years (NZ-INTIMATE project) . Journal of Quaternary Science , 22 : 9 – 35 .
- Anderson , JG , Brune , JN , Louie , JN , Zeng , Y , Savage , M , Yu , G , Chen , Q and dePolo , D . 1994 . Seismicity in the Western Great Basin apparently triggered by the Landers, California, Earthquake, 28 June 1992 . Bulletin of the Seismological Society of America , 84 : 863 – 891 .
- Barnes , PM and Audru , J-C . 1999 . Quaternary faulting in the offshore Flaxbourne and Wairarapa Basins, southern Cook Strait, New Zealand . New Zealand Journal of Geology and Geophysics , 42 : 349 – 367 .
- Barnes PM Pondard N Lamarche G Mountjoy J Van Dissen R Litchfield N 2008 It's Our Fault: active faults and earthquake sources in Cook Strait NIWA Client Report WLG2008–56
- Beanland S 1995 The North Island Dextral Fault Belt, Hikurangi Subduction Margin, New Zealand PhD thesis 341 Victoria Univ. of Wellington Wellington
- Beanland , S , Berryman , KR and Blick , GH . 1989 . Geological investigations of the 1987 Edgecumbe earthquake, New Zealand . New Zealand Journal of Geology and Geophysics , 32 : 73 – 91 .
- Begg JG Johnston MR comp. 2000 Geology of the Wellington area Institute of Geological and Nuclear Sciences 1:250 000 geological map 10
- Benson , AM , Little , TA , Van Dissen , RJ , Hill , N and Townsend , DB . 2001 . Late Quaternary paleoseismic history and surface rupture characteristics of the eastern Awatere strike-slip fault . Geological Society of America Bulletin , 113 : 1079 – 1091 .
- Berryman , K . 1990 . Late Quaternary movement on the Wellington Fault in the Upper Hutt area . New Zealand Journal of Geology and Geophysics , 33 : 257 – 270 .
- Berryman , K , Beanland , S and Wesnousky , S . 1998 . Paleoseismicity of the Rotoitipakau Fault Zone, a complex normal fault in the Taupo Volcanic Zone, New Zealand . New Zealand Journal of Geology and Geophysics , 41 : 449 – 465 .
- Bodin , P , Bilham , R , Behr , J , Gomberg , J and Hudnut , KW . 1994 . Slip triggered on Southern California Faults by the 1992 Joshua Tree, Landers, and Big Bear Earthquakes . Bulletin of the Seismological Society of America , 84 : 806 – 816 .
- Cochran , U , Hannah , M , Harper , M , Van Dissen , R , Berryman , K and Begg , J . 2007 . Detection of large, Holocene earthquakes using diatom analysis of coastal sedimentary sequences, Wellington, New Zealand . Quaternary Science Reviews , 26 : 1129 – 1147 .
- Eiby GA 1980 The Marlborough Earthquakes of 1848. New Zealand Department of Scientific and Industrial Research, Wellington DSIR Bulletin 225
- Formento-Trigilio , ML , Burbank , DW , Nicol , A , Shulmeister , J and Reiser , U . 2003 . River response to an active fold-and-thrust belt in a convergent margin setting, North Island, New Zealand . Geomorphology , 49 : 125 – 152 .
- Gomberg , J , Reasenberg , PA , Bodin , P and Harris , RA . 2001 . Earthquake triggering by seismic waves following the Landers and Hector Mine earthquakes . Nature , 411 : 462 – 466 .
- Gomberg , J , Bodin , P , Larson , K and Dragert , H . 2004 . Earthquake nucleation by transient deformations caused by the M = 7.9 Denali, Alaska, earthquake . Nature , 427 : 621 – 624 .
- Grant-Taylor TL 1974 Appendix 1 Working report: Geology of the Wellington urban area. In: Grant-Taylor TL, Adams RD, Hatherton T, Milne JDG, Northey RD, Stephenson WR Microzoning for earthquake effects in Wellington, New Zealand New Zealand Department of Scientific and Industrial Research Bulletin 213 13 22
- Grapes , RH and Downes , G . 1997 . The 1855 Wairarapa, New Zealand, earthquake: analysis of historical data . Bulletin of the New Zealand National Society for Earthquake Engineering , 30 : 271 – 368 .
- Grapes , R , Little , T and Downes , G . 1998 . Rupturing of the Awatere Fault during the 1848 October 16 Marlborough earthquake, New Zealand: historical and present day evidence . New Zealand Journal of Geology and Geophysics , 41 : 387 – 389 .
- Hamiel , Y , Amit , R , Begin , ZB , Marco , S , Katz , O , Salamon , A , Zilberman , E and Porat , N . 2009 . The seismicity along the Dead Sea Fault during the last 60,000 years . Bulletin of the Seismological Society of America , 99 : 2020 – 2026 .
- Hart , EW , Bryant , WA and Treiman , JA . 1993 . Surface faulting associated with the June 1992 Landers Earthquake, California . California Geology , 16 : 10 – 16 .
- Heron , D , Van Dissen , R and Sawa , M . 1998 . Late Quaternary movement on the Ohariu Fault, Tongue Point to MacKays Crossing, North Island, New Zealand . New Zealand Journal of Geology and Geophysics , 41 : 419 – 439 .
- Hill , DP , Reasenberg , PA , Michael , A , Arabaz , WJ , Beroza , G , Brunbaugh , D , Brune , JN , Castro , R , Davis , S , dePolo , D , Johnston , MJS , Jones , L , Keller , R , Malone , S , Munguia , L , Nava , S , Pechmann , JC , Sanford , A , Simpson , RW , Smith , RB , Stark , M , Stickney , A , Vidal , A , Walter , S , Wong , V and Zollweg , J . 1993 . Seismicity remotely triggered by the Magnitude 7.3 Landers, California, Earthquake . Science , 260 : 1617 – 1623 .
- Hodgkinson , KM , Stein , RS and King , GCP . 1996 . The 1954 Rainbow Mountain-Fairview Peak-Dixie Valley earthquakes: a triggered normal faulting sequence . Journal of Geophysical Research , 101 ( B11 ) : 25459 – 25471 .
- Kerr J Nathan S Van Dissen R Webb P Brunsdon D King A 2003 Planning for development of land on or close to active faults Institute of Geological & Nuclear Sciences Client Report 2002/124
- Langridge , RM , Berryman , KR and Van Dissen , RJ . 2007 . Late Holocene paleoseismicity of the Pahiatua section of the Wellington Fault, New Zealand . New Zealand Journal of Geology and Geophysics , 50 : 205 – 226 .
- Langridge RM Van Dissen R Villamor P Little T 2009 It's Our Fault – Wellington Fault paleoearthquake investigations: final report. GNS Science Consultancy Report 2008/344
- Litchfield , NJ and Berryman , KR . 2005 . Correlation of fluvial terraces within the Hikurangi Margin, New Zealand: implications for climate and baselevel controls . Geomorphology , 68 : 291 – 313 .
- Litchfield , N , Van Dissen , R , Langridge , R , Heron , D and Prentice , C . 2004 . Timing of the most recent surface rupture event on the Ohariu Fault near Paraparaumu. New Zealand . New Zealand Journal of Geology and Geophysics , 47 : 123 – 127 .
- Litchfield , N , Van Dissen , R , Heron , D and Rhoades , D . 2006 . Constraints on the timing of the three most recent surface rupture events and recurrence interval for the Ohariu Fault: trenching results from MacKays Crossing, Wellington, New Zealand . New Zealand Journal of Geology and Geophysics , 49 : 57 – 61 .
- Little , TA , Van Dissen , R , Schermer , E and Carne , R . 2009 . Late Holocene surface ruptures on the southern Wairarapa fault, New Zealand: link between earthquakes and the raising of beach ridges on a rocky coast . Lithosphere , 1 : 4 – 28 .
- McCormac , FG , Hogg , AG , Blackwell , PG , Buck , CE , Higham , TFG and Reimer , PJ . 2004 . CHCal04 southern hemisphere calibration, 0-11.0 cal kyr BP . Radiocarbon , 46 : 1087 – 1092 .
- McSaveney , MJ , Graham , IJ , Begg , JG , Beu , AG , Hull , AG , Kim , K and Zondervan , A . 2006 . Late Holocene uplift of beach ridges at Turakirae Head, south Wellington coast, New Zealand . New Zealand Journal of Geology and Geophysics , 49 : 337 – 358 .
- Mason , DPM and Little , TA . 2006 . Refined slip distribution and moment magnitude of the 1848 Marlborough earthquake, Awatere Fault, New Zealand . New Zealand Journal of Geology and Geophysics , 49 : 375 – 382 .
- Mason , DPM , Little , TA and Van Dissen , RJ . 2006 . Refinements to the paleoseismic chronology of the eastern Awatere Fault from trenches near Upcot Saddle, Marlborough, New Zealand . New Zealand Journal of Geology and Geophysics , 49 : 383 – 397 .
- Milne J.D.G. 1973a Upper Quaternary Geology of the Rangitikei Drainage Basin, North Island, New Zealand PhD Thesis. Massey University Palmerston North New Zealand
- Milne J.D.G. 1973b Map and Sections of River Terraces in the Rangitikei Basin, North Island, New Zealand New Zealand Soil Survey Report 4 Department of Scientific and Industrial Research Wellington, , New Zealand
- Nicol , A and Wallace , LM . 2007 . Temporal stability of deformation rates: Comparison of geological and geodetic observations, Hikurangi subduction margin, New Zealand . Earth and Planetary Science Letters , 258 : 397 – 413 .
- Palmer A Van Dissen R 2002 Northern Ohariu Fault: Earthquake hazard assessment of a newly discovered active strike-slip fault in Horowhenua Unpublished report prepared for Earthquake Comission Research Foundation Project 97/263. EQC Research Paper 3619 (http://www.eqc.govt.nz/research/researchpapers.aspx/)
- Pondard N Barnes P Lamarche G Mountjoy J 2007 Active faulting and seismic hazard in cook strait using high-resolution seismic data Joint Geological Society of NewZealand and NewZealand Geophysical Society Joint Annual Conference, Tauranga, Programme and Abstracts. Geological Society of NewZealand Miscellaneous Publication 123A 132
- Robinson , R and Benites , R . 1996 . Synthetic seismicity models for the Wellington Region, New Zealand: Implications for the temporal distribution of large events . Journal of Geophysical Research : 27,833–27 , 101 : 844
- Robinson R Van Dissen R Litchfield N 2009 It's Our Fault-Synthetic Seismicity of the Wellington Region: Final Report. GNS Science Consultancy Report 2009/192
- Rodgers DW Little TA 2006 World's largest coseismic strike-slip offset: the 1855 rupture of the Wairarapa Fault, New Zealand, and implications for displacement/length scaling of continental earthquakes Journal of Geophysical Research 111 B12 doi:10.1029/2005JB004065
- Rymer , MJ . 2000 . Triggered surface slips in the Coachella Valley area associated with the 1992 Joshua Tree and Landers, California, Earthquakes . Bulletin of the Seismological Society of America , 90 : 832 – 848 .
- Schwartz , DP and Coppersmith , KJ . 1984 . Fault behaviour and characteristic earthquakes: examples from the Wasatch and San Andreas fault zones . Journal of Geophysical Research , 89 ( B7 ) : 5681 – 5698 .
- Sieh , K , Jones , L , Haukkson , E , Hudnut , K , Eberhart-Phillips , D , Heaton , T , Hough , S , Hutton , K , Kanamori , H , Lilje , A , Lindvall , S , McGill , SF , Mori , J , Rubin , C , Spotila , JA , Stock , J , Thio , HK , Treiman , J , Wernicke , B and Zacharariasen , J . 1993 . Near-field investigations of the Landers Earthquake sequence, April to July 1992 . Science , 260 : 171 – 176 .
- Stein , RS , Barka , AA and Deitrich , JH . 1997 . Progressive failure on the North Anatolian fault since 1939 by earthquake stress triggering . Geophysical Journal International , 128 : 594 – 604 .
- Stirling , M , Wesnousky , SG and Shimazaki , K . 1996 . Fault trace complexity, cumulative slip, and the shape of the magnitude-frequency distribution for strike-slip faults: a global survey . Geophysical Journal International , 124 : 833 – 868 .
- Stirling , MW , McVerry , GH and Berryman , KR . 2002 . A new Seismic Hazard Model for New Zealand . Bulletin of the Seismological Society of America , 92 : 1878 – 1903 .
- Van Dissen , RJ and Berryman , KR . 1996 . Surface rupture earthquakes over the last ∼1000 years in the Wellington region, New Zealand, and implications for ground shaking hazard . Journal of Geophysical Research , 101 : 5999 – 6019 .
- Van Dissen , RJ , Berryman , KR , Pettinga , JR and Hill , NL . 1992 . Paleoseismicity of the Wellington – Hutt Valley Segment of the Wellington Fault, North Island, New Zealand . New Zealand Journal of Geology and Geophysics , 35 : 165 – 176 .
- Van Dissen RJ Begg JC Robinson R 2001 The Akatarawa Fault: a newly discovered active fault in the Wellington region, and implications for increased hazard on the Wellington fault New Zealand Society for Earthquake Engineering Conference Paper No. 4.06.01
- Van Dissen R Berryman K King A Webb T Brackley H Barnes P Beavan J Benites R Barker P Carne R Cochran U Dellow G Fry B Hemphill-Haley M Francois-Holden C Lamarche G Langridge R Litchfield N Little T McVerry G Ninis D Palmer N Perrin N Pondard N Semmens S Robinson R Villamor P Wallace L Wilson K 2009 It's Our Fault: better defining the earthquake risk in Wellington – results to date and a look to the future New Zealand Society of Earthquake Engineering Conference Paper no. 48 8
- Wesnousky , SG . 1994 . The Gutenberg-Richter or Characteristic earthquake distribution, which is it? . Bulletin of the Seismological Society of America , 84 : 1940 – 1959 .
- Williams , DN . 1975 . Ohariu Fault at Porirua, Wellington, New Zealand . New Zealand Journal of Geology and Geophysics , 18 : 659 – 665 .
Appendix: Trench unit descriptions
Boom Rock Road trench (T06/1) ()
-
Chocolate brown friable silt with roots = topsoil.
-
Mottled orange and creamy-grey massive silt, grading down to fine sand at base, with sparse greywacke cobbles ≤15 cm. Vertical root casts. SW wall has two distinct bands of iron staining at the top, and in the middle of the silt = subsoil.
-
Grey with orange iron staining, poorly sorted, pebble to cobble gravel. Subangular to subrounded greywacke clasts ≤ 20 cm. Silty sandy gritty matrix.
-
Creamy-grey interbedded silty fine sand and fine grit. Bedded on a cm scale. Not visible on SW wall – gravel extends to fault.
-
Dark grey massive crushed greywacke = cataclasite. 10 cm wide gouge zone along the fault. Contains angular blocks of greywacke and quartz ≤ 5 cm, and at least one subrounded greywacke boulder.
-
Grey-brown organic-rich silt.
-
Greenish-grey clayey silt.
-
Light grey, massive clayey silt. Grey colour due to soil gleying.
8a. Orange mottled silt.
-
Medium grey-brown massive flaky silt with moderately developed crumb soil structure. Abundant roots.
-
Light-grey, massive silty clay. Grey colour due to soil gleying
10a Light grey-brown, with prominent orange mottles, clayey silt. In places fine sandy clayey silt, especially on top of the gravel. Contains a stone line and the concentration of clasts increases within 30 cm of underlying gravel. May correlate with unit 2.
-
Subvertically banded chocolate-brown organic silt.
-
Same description as unit 3; may be the same unit.
Bryant trench (T08/1)-both walls (C, E)
-
Massive blue-grey fine sandstone. Top 60 cm oxidised to light brown to orange brown = Torlesse.
-
Compact fresh, to slightly to moderately weathered, sandy, pebbly fine to coarse cobble gravel with sparse boulders. Maximum clast size 25–30 cm, typically <15 cm. Blue-grey, oxidised to orange-brown towards the fault. Massive to very poorly bedded. Predominantly subrounded = fluvial gravel.
-
Firm to slightly compact, massive silty fine sand at base grading up to cm-bedded, blue-grey, silty fine sand and dark brownish grey organic-rich silt. The top 10 cm is cm-bedded oxidised orange stiff clay (between 9 and 12 m).
-
Gravelly silt to silty gravel, pebble sized, with rare cobbles. Cm to dm-bedded, moderately bedded. Clasts typically sub-angular, very firm, light brown to light orange brown. The largest clasts are subrounded and probably derived from a correlative of unit 2.
-
Silt with cm-thick stringers of silty gravel. Clasts subangular, light brown to light orange-brown, with the intensity of light grey and orange mottling increasing toward the top. Very firm. More gravel at the base (i.e., fines up).
-
Moderately to poorly dm-bedded fine to medium gravely silt to silt with gravel stringers. Firm, light brown to light orange-brown. Subangular. Maximum clast size 10 cm, typically 3 cm.
-
Clay, grading to silty clay at the base. Massive, firm, with rare cobbles. Light orange-brown mottled throughout.
-
Clayey silt, massive, light orange-grey. Notable preservation of roots throughout.
-
Silty clay, orange-brown, massive, mottled.
-
Soil horizon in unit 9. Less mottled and pronounced crumb structure.
-
Grey-brown soil A horizon. Lots of roots, soil crumb structure.
-
Dark grey laminated fault pug.
-
Crushed faulted and pugged unit 1.
-
Light grey, soft silt, contains some roots.
-
Slightly firm, light grey silt with orange mottles (slightly mottled) with pronounced roots.
-
Chaotic mixture of blocks and blobs of units 8, 14, 15, 5. Light grey to light orange-brown, orange mottling.
-
Slightly firm, light grey with orange mottles clayey silt. Some big roots but less fine roots compared with unit 15.
-
Light grey-brown silt with crumb soil structure. May have been soil on unit 17.
-
Medium grey-brown silt with crumb soil structure.
Bryant trench (T08/1) – units in southwest wall only (C)
-
Slightly firm light grey, slightly orange mottled silt with pronounced roots. Probably analogous to unit 15 in the NE wall.
-
Chocolate brown organic rich silt and peat. Possibly analogous to unit 17 in the NE wall.
-
Grey-brown silt, organic rich, with charcoal pieces. Possibly analogous to unit 18 in NE wall. Base appears to contain cm-size blocks of mixed unit 16.