Abstract
Ion-microprobe (Sensitive High Resolution Ion MicroProbe or SHRIMP) zircon and monazite U-Th-Pb geochronology of amphibolite-facies orthogneiss and paragneiss units, mostly from the Fraser and Granite Hill Complexes of Westland, New Zealand, has constrained the ages of protolith rocks and metamorphic overprints. Paragneiss zircon (6 samples, 175 analyses) comprise detrital grains of Paleozoic to Archean age and match patterns from metasedimentary (Ordovician) Greenland Group rocks of the Western Province, which are characteristic of early Paleozoic flysch units around the southwest Pacific margin of Gondwana. Orthogneiss zircon (9 samples, 138 analyses) reflect intense Devonian and Cretaceous magmatism associated with emplacement of the Karamea-Paparoa and Hohonu Batholiths within the Western Province. Both paragneiss and orthogneiss monazite analyses (10 samples, 116 analyses) are dominated by Devonian and Cretaceous ages, reflecting thermal pulses related to regional and localised granitoid intrusions. These results indicate that the Fraser and Granite Hill Complex gneisses are metamorphosed parts of the Western Province Buller Terrane with Karamea-Paparoa and Hohonu Batholith intrusive components.
Introduction
The southwest Pacific margin of Gondwana (Eastern Australia, Western Antarctica and New Zealand regions) is characterised by extensive Paleozoic and Mesozoic sedimentary and igneous rocks that record a long period of predominantly east-migrating subduction-related sedimentation, volcanism and pluton intrusion (Gray & Foster Citation2004; Tulloch et al. Citation2009). The opening of the Tasman Sea and the Antarctic Ocean in the late Cretaceous has dispersed these originally contiguous regional elements (Kula et al. Citation2007). In New Zealand, these rocks form outcropping basement in the South Island of New Zealand and collectively form the Western Province and parts of the Median Batholith (Mortimer Citation2004). These rocks have been variably affected by intrusion-related metamorphic events in the Devonian-Carboniferous and Cretaceous, resulting in extensive metamorphic belts and gneisses in Westland and Fiordland (Ireland & Gibson Citation1998; Daczko et al. Citation2009). Also in Westland are high-grade paragneiss and orthogneiss rocks immediately west of the present Australian-Pacific plate boundary that have not been conclusively linked to pre-metamorphic protolith rocks (Rattenbury Citation1987 , Citation1991). These rocks have possibly been emplaced and deformed by one of various extensional (Tulloch & Kimbrough Citation1989; Spell et al. Citation2000; Kula et al. Citation2007), strike-slip and oblique convergent (Klepeis et al. Citation2004; Marcotte et al. Citation2005; Allibone & Tulloch Citation2008) plate boundary tectonic events that have affected the Western Province in the Phanerozoic. The gneisses could be up-faulted, high-grade metamorphic equivalents of nearby known rocks, faulted slices of other known terranes in the South Island or an exotic accreted terrane (Rattenbury Citation1991; Kimbrough et al. Citation1994). This paper reports on a geochronological study of these gneisses using high resolution U-Th-Pb in situ Sensitive High Resolution Ion MicroProbe (SHRIMP) dating of single zircon and monazite crystals to ascertain (1) their protolith ages and (2) the timing of metamorphism of the gneisses, to better constrain their origin and tectonostratigraphic context. This work was initiated in part to provide geochronology for a new geological map of central Westland (Nathan et al. Citation2002).
Geological setting
The western part of the South Island of New Zealand has a long Phanerozoic history of sedimentation, deformation and igneous and metamorphic activity. The area includes the Buller and Takaka basement terranes that form the Western Province. The oldest known rocks are metamorphosed Cambrian volcanogenic arc-related rocks that occur in a small area around Springs Junction (Nathan et al. Citation2002; Rattenbury et al. Citation2006) and more widely further north in northwest Nelson (Rattenbury et al. Citation1998). These predominantly mafic volcanic rocks form the older part of Takaka Terrane and the younger part is dominated by siliceous and calcareous Ordovician to early Devonian platform sedimentary rocks. Most of the basement of western South Island is Buller Terrane (Cooper & Tulloch Citation1992) and is dominated by the quartzo-feldspathic metasedimentary rocks of the Greenland Group of Ordovician age (Cooper Citation1975 , Citation1989). These sandstone and mudstone turbidites are generally weakly metamorphosed (lower-greenschist facies).
While plutonism has occurred intermittently through much of the Paleozoic and Mesozoic in the western South Island, there have been two well-documented periods of significant igneous intrusion. Late Devonian to early Carboniferous activity is recorded in many parts of the area (Allibone et al. Citation2007 , Citation2009a , Citationb; Tulloch et al. Citation2009), particularly in the north associated with emplacement of the Karamea Batholith (Tulloch Citation1983 , Citation1988; Muir et al. Citation1994 , Citation1996a; Rattenbury et al. Citation1998; Tulloch et al. Citation2009). Most of these granite and granodiorite-dominated rocks are grouped into the S-type Karamea Suite with volumetrically minor contributions from the Tobin, Ridge and Paringa Suites (Tulloch Citation1983 , Citation1988; Tulloch et al. Citation2009). Early Cretaceous plutonism has contributed significant volumes of granitic rock in parts of the West Coast, notably in the Paparoa, Victoria and Hohonu Ranges. Most of these are grouped into two geochemically defined suites: the I-type Separation Point Suite and the generally slighter younger I-S type Rahu Suite (Tulloch Citation1983 , Citation1988; Waight et al. Citation1997). Many of these Cretaceous plutons form parts of the Karamea-Paparoa, Hohonu and Median Batholiths (Mortimer Citation2004).
From the early Cretaceous to Recent there has been semi-continuous deposition of clastic sediment, commonly in tectonically active, fault-controlled basins (Nathan et al. Citation1986 , Citation2002). The lower elevation parts of the West Coast area are typically blanketed with late Neogene fluvio-glacial deposits largely derived from the rapidly uplifting Southern Alps (Suggate & Waight Citation1999). Significant periods of tectonism in the Silurian, Devonian, Cretaceous and Neogene in western New Zealand have also contributed to complex structural geometries of the basement and older cover rocks (Tulloch & Kimbrough Citation1989; Allibone & Tulloch Citation2008). Convergent deformation in the Neogene has resulted in uplift of some ranges exposing the crustal basement rocks (Kamp et al. Citation1992; Batt et al. Citation2000 , Citation2004; Little et al. Citation2005).
High-grade gneisses occur in two main areas on the West Coast of the South Island: in the Paparoa Range and along the Alpine Fault (Nathan et al. Citation2002; Cox & Barrell Citation2007). The Paparoa Range gneisses have been attributed to a metamorphic core complex that emerged during early rifting stages that ultimately led to the opening of the Tasman Sea in the late Cretaceous (Tulloch & Kimbrough Citation1989). The gneisses of the Paparoa Range include both metasedimentary and plutonic protoliths (Nathan et al. Citation2002). The paragneisses have geochemical and geochronological similarities to the Greenland Group metasedimentary rocks that form some of the cover rocks in the upper plate of the core complex. The other main area of gneiss exposure, and the focus of this study, mostly occurs between the Alpine and Fraser Faults where gneissic rocks crop out in up-faulted ranges south of Hokitika (Rattenbury Citation1991; Jongens Citation2006) and in the southern Victoria Range ().
Fig. 1 Geological map of Western Province basement granitic, gneissic and metasedimentary rocks and key faults in central Westland (modified after Nathan et al. Citation2002; Cox & Barrell Citation2007) showing sample locations. The 10 km grid is in NZTM2000 coordinates. The inset map shows the New Zealand basement terranes in the central South Island (after Mortimer Citation2004).
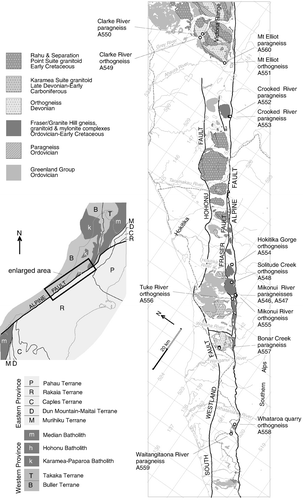
Amphibolite-facies gneisses, associated granitic plutons and enveloping mylonitic rocks south of Hokitika are known as the Fraser Complex (Rattenbury Citation1991). These gneisses include garnet-biotite±kyanite±sillimanite metapelite, migmatitic quartzofeldspathic metasandstone and hornblende±epidote±garnet amphibolite. To the northeast a similar association is known as the Granite Hill Complex (Suggate & Waight Citation1999). The position of the Fraser and Granite Hill Complexes along the current plate boundary has meant a number of potential protoliths can be considered including quartz-rich clastic Buller Terrane (Rattenbury Citation1991), a remnant of the Cambrian-Devonian volcanosedimentary Takaka Terrane, a remnant of the Carboniferous-Cretaceous Median Batholith/Tectonic Zone (Kimbrough et al. Citation1994) or even a completely exotic accreted terrane. The tectonic exhumation of the gneissic rocks could potentially involve localised Cenozoic reverse fault uplift (White & Green Citation1986; Rattenbury Citation1991), major extensional unroofing (Kimbrough et al. Citation1994; Jongens Citation2006) or large-scale strike-slip fault juxtaposition.
Previous geochronology
In situ single grain SHRIMP U-Pb zircon dating of a Victoria Range paragneiss and two Granite Hill Complex paragneisses by Ireland (Citation1992) yielded a range of concordant ages mostly between 1219 and 115 Ma that were interpreted to record Silurian and early Cretaceous metamorphism of an Ordovician sedimentary protolith derived from Archean, Proterozoic and Cambrian rocks. Further work on a Victoria Range paragneiss yielded an ion microprobe monazite U-Pb age of 377±7 Ma and Th-Pb age of 374±7 Ma (Ireland & Gibson Citation1998) that was interpreted to indicate widespread Devonian thermal metamorphism associated with Karamea granite batholith emplacement. A Fraser Complex migmatite U-Pb zircon lower intercept age of 157±21 Ma has been interpreted to date peak metamorphism and the upper intercept of 735±100 Ma, suggesting inheritance of Proterozoic zircon (Kimbrough et al. Citation1994). K-Ar dating of biotite, hornblende and whole rock Fraser Complex gneisses yielded results suggesting some excess argon contamination, but constraining cooling through the biotite K-Ar closure temperature after 44 Ma (Rattenbury Citation1987). Fission track dating of minerals separated from the Fraser Complex indicate ages of c. 9 Ma (zircon) and c. 2 Ma (apatite), reflecting current uplift associated with compression between the Alpine and Fraser Faults (White & Green Citation1986; Kamp et al. Citation1992).
Adjacent late Devonian to early Carboniferous plutonism has been constrained by zircon geochronology. Muir et al. (Citation1996a) documented a cluster of c. 375 Ma ion microprobe ages as well as two younger dates around 330 Ma. Tulloch et al. (Citation2009) obtained ages between 371 and 305 Ma, mostly concentrated around 370 Ma, from ID-TIMS analyses. Early Cretaceous plutonism is also well constrained by ages between 129 and 109 Ma for the Separation Point Suite and 115 and 105 Ma for the Rahu Suite (Muir et al. Citation1994 , Citation1997; Waight et al. Citation1997).
Samples
This study has concentrated on gneissic rocks located close to the Alpine Fault, particularly within the Fraser Complex (). The fifteen samples analysed were collected between the southern Victoria Range and Whataroa, falling into two main categories: six garnet-biotite paragneisses and nine biotite orthogneisses (). The paragneisses contain sillimanite and/or kyanite in places and are inferred to be upper amphibolite facies metapelites. Monazites from two additional samples, one paragneiss and one orthogneiss of the Charleston Metamorphic Complex previously reported by Ireland & Gibson (Citation1998), were analysed to compare our analytical methods and results with previous geochronology on similar samples.
Table 1 Sample locations and lithological descriptions.
Analytical methods
Clean rock crushing and heavy-liquid mineral separation was undertaken at GNS Science, followed by magnetic extraction of zircon and monazite at the Research School of Earth Sciences (RSES) of The Australian National University (ANU). Approximately 100 grains from each sample were transferred onto double-sided adhesive tape under a binocular microscope without attempting to bias the sample selection. Zircon and monazite samples were mounted close to their respective reference materials, SL13 zircon, FC1 zircon and Maffra monazite. All grains were cast in epoxy and polished with a rotary pad and 1 µm diamond paste to expose crystal midsections. Before analysis, the zircon and monazite were characterised with reflected light and transmitted light imaging followed by either Hitachi S-2250N SEM cathodoluminesence (zircon) or Cambridge S360 SEM back-scattered electron (monazite) imaging. This allowed identification of cracks and mineral inclusions, as well as providing images of two-dimensional (2D) growth and recrystallisation textures to guide spot placement. The major growth domains of clean grains from all morphologies were analysed to provide representative age spectra from each sample. Before each SHRIMP analytical session, polished (analytical) surfaces were sequentially cleaned in an ultrasonic bath with petroleum spirit, ethanol, diluted laboratory detergent, 1M HCl (1×quartz distilled) and deionised (18 Mω) H2O before being dried in a 60°C oven. A 100Å Au conductive layer was then evaporated onto the analytical surface and electrically checked for uniform and adequate conductivity before loading into the instrument.
U-Th-Pb ages were determined using the SHRIMP Reverse Geometry (RG) ion microprobe at RSES, ANU. The methods employed here are similar to those described in detail by Muir et al. (Citation1996a), Williams (Citation1998) and Ireland & Gibson (Citation1998) and are summarised as follows. For zircon analysis, a 3 nA mass filtered O2 − primary beam was focused to a c. 30 µm (long axis) elliptical spot and the beam rastered for 120 s to clean the mount surface prior to data acquisition. The magnet was stepped through positive secondary ion peaks of 90Zr2 16O, 204Pb, 206Pb, 207Pb, 208Pb, 238U, 232Th16O and 238U16O, analysed by a single electron multiplier. For monazite analysis the primary beam current was reduced to 1 nA to prevent the ThO+ signal damaging the ion counter, energy filtering was employed to minimise a multi-element isobaric interference on 204Pb and the Zr2O species was replaced with 140Ce31P16O2. Isotope ratios were recorded as measured, without applying any correction for hydride interferences or isotopic mass fractionation. Mass resolution was c. 5500 at the 1% peak height.
Zircon reference material SL13 (Claoué-Long et al. Citation1995; U=238 ppm) was used to calculate U abundances. FC1 zircon (Paces & Miller Citation1993; 1099±1 Ma) and Maffra monazite (Ireland & Gibson Citation1998; 421±1 Ma) reference materials were analysed once every three unknowns and were used for determining empirical quadratic calibrations based on the form 206Pb/U=a(UO/U)2 and 208Pb/Th=a(ThO/Th)2. Decay constants and atomic ratios recommended by the IUGS Subcommission on Geochronology (Steiger & Jäger Citation1977) were used to calculate ages. Analyses with 206Pb/238U ages <800 Ma were corrected for common Pb based on the measured 207Pb/206Pb ratio (207Pb correction method). Analyses were assumed to be a mixture between a concordant radiogenic datum and surface contamination common Pb with a Broken Hill isotopic composition (204Pb/206Pb=0.0625, 207Pb/206Pb=0.962, 208Pb/206Pb=2.23). Most measured data points record a high level of concordance so the common Pb correction was insensitive to the choice of isotopic composition used. Zircon analyses that recorded 206Pb/238U ages >800 Ma are instead listed as 207Pb/206Pb ages corrected for common Pb based on measured 204Pb/206Pb (204Pb correction method).
Analytical uncertainties for individual spots are reported as 1 σ within-spot errors. To condense a weighted mean 206Pb/238U or 208Pb/232Th age for selected samples, analyses were culled to selectively remove outliers. Outliers were statistically and/or geologically identified based on U-Th-Pb ages that were products of inheritance, local recrystallisation or Pb disturbance (e.g., around cracks and inclusions). A population of acceptable analyses from each sample was then pooled to calculate crystallisation ages using the ExcelTM macro Isoplot (Ludwig Citation2003). Weighted mean 95% confidence limit uncertainties were calculated from the inverse square of the assigned within-spot errors summed in quadrature with the 2 σ external reproducibility of the U/Pb or Th/Pb calibration standard that ranged from ±0.66% to ±0.76% for zircon and ±0.94% to ±1.05% for monazite.
Results
Summaries of U-Th-Pb results from individual spot analyses of paragneiss zircon (6 samples, 175 analyses), orthogneiss zircon (9 samples, 138 analyses) and combined paragneiss and orthogneiss monazite (10 samples, 116 analyses) can be found in , and , respectively. Spot analyses used for weighted mean 206Pb/238U or 208Pb/232Th age calculations of selected samples are marked in bold. Uncorrected 238U/206Pb – 207Pb/206Pb ratios are presented as Tera-Wasserberg Concordia diagrams for all zircon analyses in and all monazite analyses in A–J. Comparisons between monazite 206Pb/238U and 208Pb/232Th ages are presented in K–T. Probability density plots of sample age distributions are presented in . Representative grain images and summary histograms depicting paragneiss zircon, orthogneiss zircon and combined paragneiss and orthogneiss monazite age structures are presented in and 6.
Fig. 2 Tera-Wasserberg concordia diagrams presenting uncorrected 238U/206Pb–207Pb/206Pb ratios of all zircon analyses.
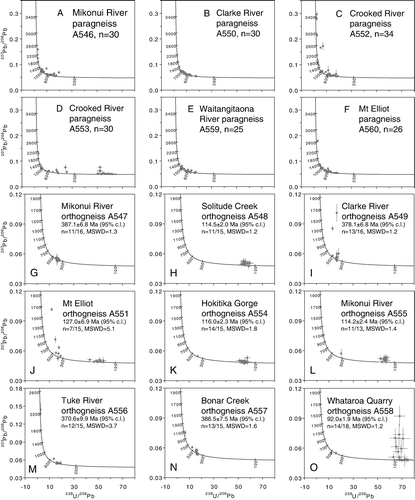
Fig. 3 Tera-Wasserberg concordia diagrams presenting uncorrected 238U/206Pb–207Pb/206Pb ratios of all monazite analyses (A–J). Correlations between 206Pb/238U and 208Pb/232Th ages for all monazite analyses (K–T).
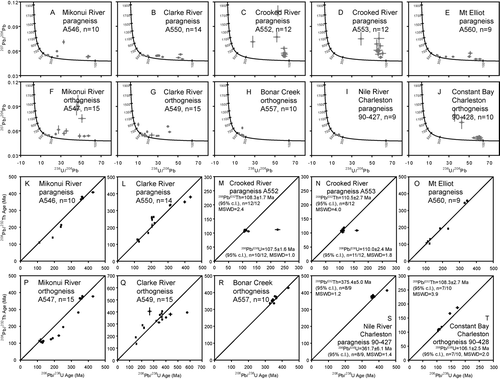
Fig. 4 Probability density plots for all samples. Orthogneiss ages >600 Ma omitted for clarity. For all plots 238U/206Pb ages <800 Ma are corrected for common Pb based on 207Pb. 207Pb/206Pb ages >800 Ma are corrected for common Pb based on 204Pb.
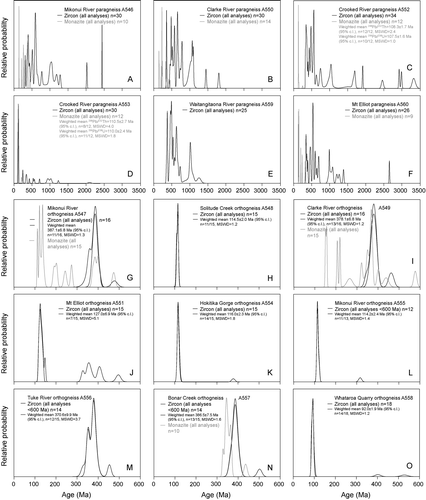
Fig. 5 Zircon cathodoluminesence (CL) and monazite back-scattered electron (BSE) grain images and SHRIMP analytical spot ages for representative (A) paragneiss A546; (C) orthogneiss A551; and (E) orthogneiss A552. Excellent agreement exists between monazite 206Pb/238U (*) and 208Pb/232Th (‘) ages. Cumulative histogram of (B) paragneiss zircon, (D) orthogneiss zircon and (F) combined paragneiss and orthogneiss monazite age structures. For all histograms 238U/206Pb ages <800 Ma are corrected for common Pb based on 207Pb. 207Pb/206Pb ages >800 Ma are corrected for common Pb based on 204Pb.
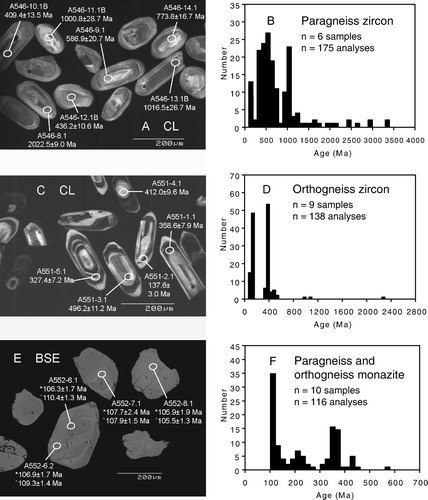
Table 2 U-Th-Pb geochronology results from analysis of paragneiss zircon. f206 = fraction of common 206Pb of the total measured Pb. Total ratios are given as measured and are uncorrected for common Pb. Correction method 1 is based on 207Pb (with 238U/206Pb ages <800 Ma). Correction method 2 is based on 204Pb (with 207Pb/206Pb ages >800 Ma).
Table 3 U-Th-Pb geochronology results from analysis of orthogneiss zircon. f206 = fraction of common 206Pb of the total measured Pb. Total ratios are given as measured and are uncorrected for common Pb. Correction method 1 is based on 207Pb (with 238U/206Pb ages <800 Ma). Correction method 2 is based on 204Pb (with 207Pb/206Pb ages >800 Ma). Spot analyses used for weighted mean 206Pb/238U age calculations of selected samples are marked in bold.
Table 4 U-Th-Pb geochronology results from analysis of paragneiss and orthogneiss monazite. f206 or f208 = fraction of common 206Pb or 208Pb of the total measured Pb. Total ratios are given as measured and are uncorrected for common Pb. Correction is based on 207Pb. Spot analyses used for weighted mean 206Pb/238U or 208Pb/232Th age calculations of selected samples are marked in bold.
There is good agreement between the monazite age determination of Charleston Metamorphic Complex samples from this study () and those made by Ireland & Gibson (Citation1998). Nile River paragneiss sample 90-427 records well-defined late Devonian weighted mean 206Pb/238U and 208Pb/232Th ages of 361.7±6.1 Ma (n=8/9, MSWD=1.4) and 375.4±5.0 Ma (n=8/9, MSWD=1.2; I,S). These are identical or slightly older than respective published ages of 361±6 Ma and 367±6 Ma for this sample. The Constant Bay orthogneiss sample 90-428 records poorly defined middle Cretaceous weighed mean 206Pb/238U and 208Pb/232Th ages of 106.1±2.5 Ma (n=7/10, MSWD=2.0) and 108.3±2.7 Ma (n=7/10, MSWD=3.9; J,T) that are marginally younger than previously reported ages of 111±2 and 112±2 Ma.
Paragneiss zircon grain morphologies are similar among all samples. Crystals are typically anhedral, rounded and 100–300 µm in length (e.g., sample A546, A). The majority of zircon domains consist of either magmatic oscillatory growth structures or metamorphic recrystallisation textures, or occasionally as mixtures of both. Inherited cores and thin overgrowth rims are abundant, while particular zonation patterns cannot typically be correlated with specific age populations. Composite detrital age structures are similar across all six samples (A–F, 4A–F) and all data are combined as a histogram in B. The youngest 206Pb/238U ages for most samples are Carboniferous to Devonian (313.2±7.1 Ma, A546-12.1; 327.1±4.7 Ma, 552-21.1; 340.5±10.0 Ma, A560-A4.1A; 355.7±5.0 Ma, A550-18.1; 362.7±10.9 Ma, A559-A3.1) while A553 from Crooked River has 13 Cretaceous ages between 103.4±3.2 Ma (A553-A5.1) and 126.1±2.0 Ma (A553-22.1) as well as two apparently Triassic ages (D, 4D). Over the six samples, a total of 37 analyses provide ages<400 Ma and are mostly from grains with metamorphic recrystallisation textures and low Th/U ratios (Th/U<0.1, n=20; Th/U=0.1–0.3, n=12; Th/U<0.3, n=5). Across all paragneiss samples, large numbers of 206Pb/238U age components are consistently recognised between 300–500 Ma (n=46 or 26.3% of all analyses), 500–600 Ma (n=27 or 15.4%) and 60–800 Ma (n=30 or 17.1%). Following a distinctive gap in ages between 800 and 900 Ma a major spike in 207Pb/206Pb ages occurs between 900 and 1100 Ma (n=33 or 18.9%) followed by scattered data through the rest of the Proterozoic 1100–2500 Ma (n=18 or 10.3%) and into the Archean as old as 3348.0±46.9 Ma (A552-4.1; n=5 or 2.9%).
Orthogneiss zircon grain morphologies are also similar among all samples. Crystals are typically euhedral with prismatic habits, 100–350 µm in length (e.g., sample A551, Fig. 5C). Most crystals preserve large domains displaying clear igneous oscillatory growth patterns. Metamorphic recrystallisation textures are common but typically exist as a replacement to oscillatory growth structures. Inherited cores are relatively uncommon and overgrowth rims are typically confined to growth terminations. Zircon crystallisation ages across all samples are generally characterised as part of either major Devonian or Cretaceous peaks (D). Three well-spread samples from Mikonui River, Bonar Creek and Clarke River have relatively well defined middle–late Devonian ages: A547 (387.1±6.8 Ma, n=11/16, MSWD=1.3, G, 4G), A557 (386.5±7.5 Ma, n=13/15, MSWD=1.6, N, 4N) and A549 (378.1±6.8 Ma, n=13/16, MSWD=1.2, I, 4I). The slightly younger late Devonian A556 from Tuke River (370.6±9.9 Ma, n=12/15, M, 4M) records a high MSWD of 3.7. Three closely spaced samples between Hokitika Gorge and Mikonui River have well-defined middle Cretaceous ages that agree within error: A554 (116.0±2.3 Ma, n=14/15, MSWD=1.8, K, 4K), A548 (114.5±2.0 Ma, n=11/15, MSWD=1.2, H, 4H) and A555 (114.2±2.4 Ma, n=11/13, MSWD=1.4, L, 4L). The notably younger sample A558 from Whataroa Quarry has a well-defined late Cretaceous age of 92.0±1.9 Ma (n=14/18, MSWD=1.2, O, 4O).
From the total 138 analyses of these nine orthogneiss samples, 24 were culled for pooling of weighted mean ages making an average rejection count of less than three data per sample. The variability displayed by these analyses within individual samples probably represents some combination of unaccounted analytical scatter and real geological age heterogeneity. The latter is clearly demonstrated by the five analyses of inherited zircon with ages>500 Ma in four separate samples between Whataroa Quarry and Mikonui River: 502.8±10.8 Ma, A557-2.1; 516.5±15.1 Ma, A558-A-5.1; 987.9±52.6 Ma, A557-A-4.1; 1077.8±10.5 Ma, A555-A-1.1; and 2267.3±61.9 Ma, A556-8.1. Minor spot overlap onto these zones could produce variability around the main peaks. Zircon age variability within a single orthogneiss sample is well demonstrated by sample A551 from Mt Elliot, which contains a mix of Paleozoic and Mesozoic ages (J, 4J, 6) that can be clearly related to the texture of zircon analysed. For example, in C inherited cores record late Cambrian (496.2±11.2 Ma, A551-3.1) and early Devonian ages (412.0±9.6 Ma, A551-4.1), magmatic oscillatory growth domains record early Carboniferous ages (358.6±7.9 Ma, 551-1.1; 327.4±7.2 Ma, 551-5.1) and a zone of metamorphic recrystallisation records an early Cretaceous age (137.6±3.0 Ma, A551-2.1). A total of seven early Cretaceous analyses in A551 represent a relatively poorly defined population with a weighted mean age of 127.0±6.9 Ma (MSWD=5.1).
Paragneiss and orthogneiss monazite share similar age distributions so have been combined in . Grains sourced from both paragneiss and orthogneiss samples are typically 100–400 µm in length, lack any zoning growth structure and range from rounded to wedge-shaped but are rarely prismatic (e.g., sample A552, E). 206Pb/238U and 208Pb/232Th ages from individual analyses and sample-weighted means typically agree (Table 4, K–T). Across all samples monazite crystallisation ages are mainly Devonian or Cretaceous (F). The two paragneiss samples from Crooked River have four combined middle Cretaceous ages that all lie within error: A553 (206Pb/238U=110.0±2.4 Ma, n=11/12, MSWD=1.8; 208Pb/232Th=110.5±2.7 Ma, n=8/12, MSWD=4.0; Figs 3D, 3N, 4D) and A552 (206Pb/238U=107.5±1.6 Ma, n=10/12, MSWD=1.0; 208Pb/232Th=108.3±1.7 Ma, n=12/12, MSWD=2.4; C, 3M, 4C). Three paragneiss samples (A546, A, 3K, 4A; A550, B, 3L, 4B; A560, E, 3O, 4F) and three orthogneiss samples (A547, F, 3P, 4G; A549, G, 3Q, 4I; A557, H, 3R, 4N) all have highly mixed 206Pb/238U monazite ages (n=73 analyses) with Cretaceous (n=15), Triassic (n=12), Jurassic (n=7), Permian (n=2), Carboniferous (n=18), Devonian (n=13), Silurian (n=5) and Neoproterozoic (n=1) age components. It is likely that mixtures of Devonian and Cretaceous zone s have caused Triassic and Jurassic ages because they do not combine to yield statistically defined ages.
Discussion
Paragneiss protoliths and metamorphism
Previous studies have recognised the strong similarity between detrital zircon ion microprobe U-Pb age spectra derived from Western Province paragneisses and Greenland Group metasediment (Ireland Citation1992; Ireland & Gibson Citation1998). Both spectra are characterised by major peaks at 500–600 Ma (Delamerian-Ross orogen) and 1000–1200 Ma (Grenville orogen) with other minor contributions from Proterozoic and Archean protoliths. An Ordovician deposition age has been suggested for the Greenland Group metasedimentary rock (Cooper Citation1975 , Citation1989). The results of this study are consistent with that scenario given the large numbers of Cambrian (n=17) and Ordovician (n=13) detrital zircons obtained from samples A546, A550, A552, A559 and A560. The small number of Silurian analyses (n=4) may simply be associated with Pb loss during the younger metamorphic events. The common detrital zircon U-Pb age spectra present in the Fraser and Granite Hill Complex paragneisses indicate the Greenland Group is a suitable protolith for these Western Province paragneisses (Ireland Citation1992; Ireland & Gibson Citation1998). This interpretation supports earlier suggestions that Western Province paragneisses are derived from the Greenland Group, based on field relationships (Laird Citation1967), and whole-rock Sm-Nd isotopic compositions (Pickett & Wasserburg Citation1989). The Greenland Group origin for the gneisses was further supported by recognition of intervening major extensional detachment faults in the Paparoa Range that have juxtaposed the contrasting metamorphic grade rocks (Tulloch & Kimbrough Citation1989). The results of zircon geochronology from all six paragneiss samples in this study are similar to these earlier results. Additionally, each paragneiss sample locality is within 10 km of sub-greenschist facies Ordovician Greenland Group rocks (), indicating the close proximity of the metasedimentary protolith in all cases.
Clear similarities have also been previously recognised between Greenland Group detrital zircon age patterns and those of other sedimentary rocks and inherited zircon age spectra in granites from the Lachlan Fold Belt in southeast Australia (Chen & Williams Citation1990; Ireland Citation1992) and the Kanmantoo group of South Australia (Gibson & Ireland Citation1996; Ireland et al. Citation1998). These rocks were possibly derived from a provenance of similar source terrains along the Paleozoic Gondwana margin. No Precambrian rocks are recognised in New Zealand (Kimbrough & Tulloch Citation1989). However, the consistency of detrital zircon age patterns across all samples analysed indicates that the source terranes must have included a component of Cambrian, Proterozoic and Archaean material. Potential source rocks of these ages exist in Australia and Antarctica (Ireland Citation1992). Alternatively, the ancient zircons may be sourced from eroding granites derived from remelting of older, lower crustal rocks (Ireland Citation1992) or may have been sourced from some completely different reservoir, now eroded or concealed by younger rocks.
Over all six paragneiss samples, the 37 zircon analyses with U-Pb ages<400 Ma have consistently low Th/U (< 0.3). These grains are interpreted as products of metamorphic recrystallisation and minor igneous zircon growth in response to Devonian, Carboniferous and Cretaceous metamorphism and localised melting. Thermobarometry of paragneiss samples from the Charleston Metamorphic Group near Charleston (Kimbrough & Tulloch Citation1989) and in the Paparoa Range (White Citation1994) indicate peak temperatures of 600–700°C and pressures of 4±1 kbar. Monazite ion probe geochronology on Victoria Range and Charleston paragneisses (Ireland & Gibson Citation1998) indicate this upper amphibolite facies metamorphism occurred during or slightly after the intrusion of Devonian granitoids (Tulloch et al. Citation2009). Analysis of the Charleston orthogneiss monazite demonstrated that this pervasive, regional, Devonian metamorphism was followed by more localised metamorphism during emplacement of Cretaceous granitoids (Ireland & Gibson Citation1998).
Paragneiss sample A553 from Crooked River contains metamorphic Cretaceous zircons (n=13) with ages ranging from 103.4±3.2 to 126.1±2.0 Ma and low Th/U ratios (from 0.02 to 0.36). The zircon age is anomalous compared to other samples, including A552 less than 500 m away. The timing of this metamorphism is better defined by weighted mean monazite ages (206Pb/238U=110.0±2.4 Ma, 208Pb/232Th=110.5±2.7 Ma) and is within error of the emplacement ages of Rahu Suite granitoids from the nearby Hohonu Batholith (; Waight et al. Citation1997). Paragneiss sample A552 contains slightly younger Cretaceous monazite weighted mean ages of 206Pb/238U=107.5±1.6 Ma and 208Pb/232Th=108.3±1.7 Ma. However, in this sample, Cretaceous metamorphism was probably less intense as monazite ages are not accompanied by Cretaceous zircon growth and recrystallisation. Metamorphic zircon ages in paragneiss samples A546, A550, A559 and 560 are Silurian (n=2), Devonian (n=9) or Carboniferous (n=5), whereas monazite ages for samples A546, A550 and A560 vary widely between the Silurian and Cretaceous. This indicates that these samples contain a mixed sample of zoned crystals. No monazite ages older than Silurian were obtained from any paragneiss sample indicating that, if present, any older detrital monazite grains were reset during later metamorphism. It is noteworthy that a detrital monazite pattern similar to the Gondwana zircon signature was found by Ireland & Gibson (Citation1998) in a sillimanite-grade paragneiss in Fiordland.
Permian or Triassic detrital zircons are absent from all paragneiss U-Pb age spectra, ruling out a Rakaia Terrane greywacke Eastern Province protolith (Ireland Citation1992; Wysoczanski et al. Citation1997; Cawood et al. Citation1999; Pickard et al. Citation2000; Wandres et al. Citation2004 , Citation2005; Adams et al. Citation2007), whose metamorphosed equivalents – the Alpine Schist – occur southeast of the Alpine Fault ().
Orthogneiss protoliths and metamorphism
Four widely spaced orthogneiss samples A547, A557, A549 and A556 have middle–late Devonian U-Pb zircon ages of 387.1±6.8, 386.5±7.5, 378.1±6.8 and 370.6±9.9 Ma, respectively. These are interpreted as intrusion ages that are slightly older than, or contemporaneous with, the ~370 Ma emplacement of the Karamea Batholith (Muir et al. Citation1994 , Citation1996a; Tulloch et al. Citation2009). Karamea or other Devonian granitoids are mapped within several kilometres of each of these samples (). Petrogenesis of the Karamea Batholith has previously been demonstrated to be the result of mixing between primitive primary magmas and Greenland Group metasediments in varying proportions (Muir et al. Citation1996b). This interpretation is supported here by the presence of a minor component of inherited zircon in each sample, with ages that correspond to those identified in Greenland Group detrital zircon age spectra during the Ordovician (455.0±9.9 Ma, A549-9.1; 467.7±10.1 Ma, A549-10.1; 475.2±13.8 Ma, A547-A-4.1), Cambrian (502.8±10.8 Ma, A557-2.1), Neoproterozoic (987.9±52.6 Ma, A557-A-4.1) and Paleoproterozoic (2267.3±61.9 Ma, A556-8.1).
Orthogneiss sample A557 from Bonar Creek has an intrusive U-Pb zircon age of 386.5±7.5 Ma. Subsequent metamorphism is recorded by nine monazites with Devonian and Carboniferous U-Pb and Th-Pb ages. This rock intrudes more strongly foliated orthogneiss nearby (Jongens Citation2006), indicating older gneissic plutons are present in this area. One Silurian monazite (A557-8.1) probably represents an inherited monazite from a Greenland Group source that was not reset during igneous emplacement or metamorphic overprinting of the orthogneiss granitoid protolith. U-Pb zircon ages of 387.1±6.8 and 378.1±6.8 Ma were obtained from orthogneiss samples A547 and A549 respectively, interpreted to date the time of intrusion. Both samples also contain a minor component of inherited zircon. Thirty monazite analyses from these rocks yield highly mixed U-Pb and Th-Pb ages. Fifty-two monazite analyses are equal to or younger than the inferred intrusive ages. These are as young as Cretaceous and are interpreted to date subsequent metamorphism. Eight monazite ages are older than the inferred timing of intrusion and possibly represent a component of inherited monazite. Monazite inheritance is rare in granitic systems (Rapp et al. Citation1987); it has however been documented in leucogranites as well as high-grade metamorphic rocks (e.g., Copeland et al. Citation1988; Parrish Citation1990; Kingsbury et al. Citation1993; Harrison et al. Citation1995; Vry et al. Citation1996; Simpson et al. Citation2000). The Charleston Orthogneiss sample 90-427 records weighted mean monazite U-Pb and Th-Pb ages of 361.7±6.1 and 375.4±5.0 Ma, respectively, that are clearly associated with intrusion of Devonian granitoids (Ireland & Gibson Citation1998).
Three orthogneiss samples A554, A548 and A555 have well-defined middle Cretaceous U-Pb zircon intrusive ages of 116.0±2.3, 114.5±2.0 and 114.2±2.4 Ma that overlap the age ranges of Separation Point and Rahu Suites. The nearest mapped Cretaceous plutons are in the Hohonu Batholith, c. 10 km to the northeast (Nathan et al. Citation2002). Samples A554 and A555 also contain minor amounts of inherited Carboniferous, Devonian and Mesoproterozoic zircon, indicating their protolith incorporated some older crust such as Paleozoic granitoids or Greenland Group metasediments. The Charleston Orthogneiss sample 90-428 has weighted mean monazite U-Pb and Th-Pb ages of 106.1±2.5 and 108.3±2.7 Ma that are probably associated with intrusion of the Separation Point or Rahu Suites (Ireland & Gibson Citation1998). The younger sample A558 has a well-defined but previously unrecognised late Cretaceous age of 92.0±1.9 Ma (n=14/18, MSWD=1.2, O, 4O) that falls between intrusive ages of the Rahu Suite and the younger Hohonu Batholith A-type French Creek Granite at c. 82 Ma (Waight et al. Citation1997).
The ages of the orthogneiss samples are generally similar to the emplacement ages of known igneous intrusions in the region. Of interest is the lack of ages in the range 160–130 Ma. The late Jurassic to early Cretaceous age of metamorphism inferred for a Fraser Complex migmatite (Kimbrough et al. Citation1994) would appear to be the result of combining multiple components of Cretaceous and Devonian age with a mixed population of detrital zircons. The lack of zircons in this age range is noteworthy because rocks of this age are a major component of the Median Batholith (Muir et al. Citation1998; Allibone & Tulloch Citation2004; Allibone et al. Citation2007 , Citation2009a). However, although these older Mesozoic ages are not associated with the Fraser Complex, Separation Point and Rahu Suite ages are common.
Regional trends
The influence of Cretaceous plutonism and metamorphism is widespread throughout the area sampled (). The more northern paragneiss and orthogneiss samples occur close to large areas of mapped Cretaceous plutonism in the Hohonu Batholith (Waight et al. Citation1997) and the southern Victoria Range (Nathan et al. Citation2002). The more southern samples are typically located close to the Alpine and/or Fraser Faults, where Cretaceous plutonism is not well documented. Only the Bonar Creek sample A557 appears to lack Cretaceous monazites. Samples with Cretaceous zircons generally contain inherited Devonian and older zircons also, suggesting thermal metamorphism was localised and intense.
Summary and conclusions
SHRIMP zircon U-Pb and monazite U-Pb and Th-Pb ages have been obtained from 15 gneiss samples of the Fraser Complex and similar rocks in the region between the southern Victoria Range and Whataroa. Ages are similar to those previously obtained from metamorphic rocks of the Western Province. Paragneiss samples have patterns of Paleozoic, Proterozoic and Archean detrital zircons ages, indicating that they are likely to be metamorphosed Greenland Group of Ordovician depositional age. The similar detrital zircon age spectra in the paragneisses and Greenland Group metasedimentary rocks indicate a Gondwana margin provenance. Orthogneiss samples are dominated by Devonian and Cretaceous emplacement zircon ages that are associated with recognised episodes of intensive plutonism within the Western Province. Metamorphism during the Devonian and Cretaceous is indicated by monazite geochronology.
Detrital zircon age spectra and periods of magmatism and metamorphism correspond closely to events previously defined in the Western Province. There is no evidence the Fraser Complex is an exotic terrane, nor any strong evidence of either plutonism or metamorphism between the Carboniferous (c. 310 Ma) and early Cretaceous (c. 130 Ma). There is no indication of the presence of Eastern Province protoliths in these rocks. Similarly, the absence of ages between 160 and 130 Ma suggests Median Batholith material of this age is absent. Results of this study indicate that the metasedimentary components of the Fraser and Granite Hill Complexes are part of the Buller Terrane and are intruded by middle Paleozoic and early Cretaceous plutons that dominate the Karamea-Paparoa and Hohonu Batholiths.
Acknowledgements
The field assistance of Marilyn Vogel and Cal Rattenbury is gratefully acknowledged. Marilyn Vogel undertook much of the mineral separation and preliminary electron microprobe analysis. Preliminary SHRIMP analysis was undertaken by Rattenbury and Ireland in 2001 and later by Hiess between 2003 and 2006 as a Summer Research Scholar and PhD student at RSES, ANU. Andy Tulloch and Andrew Allibone are thanked for their helpful reviews that improved the manuscript.
References
- Adams , CJ , Campbell , HJ and Griffin , WL . 2007 . Provenance comparisons of Permian to Jurassic tectonostratigraphic terranes in New Zealand: perspectives from detrital zircon age patterns . Geological Magazine , 144 : 701 – 729 .
- Allibone , AH and Tulloch , AJ . 2004 . Geology of the plutonic basement rocks of Stewart Island, New Zealand . New Zealand Journal of Geology and Geophysics , 47 : 233 – 256 .
- Allibone , AH and Tulloch , AJ . 2008 . Early Cretaceous dextral transpressional deformation within the Median Batholith, Steward Island, New Zealand . New Zealand Journal of Geology and Geophysics , 51 : 115 – 134 .
- Allibone , AH , Turnbull , IM , Tulloch , AJ and Cooper , AF . 2007 . Plutonic rocks of the Median Batholith in southwest Fiordland, New Zealand: field relations geochemistry, and correlation . New Zealand Journal of Geology and Geophysics , 50 : 283 – 314 .
- Allibone , AH , Jongens , R , Scott , JM , Tulloch , AJ , Turnbull , IM , Cooper , AF , Powell , NG , Ladley , EB , King , RP and Rattenbury , M . 2009a . Plutonic rocks of the Median Batholith in eastern and central Fiordland, New Zealand: field relations, geochemistry, correlation and nomenclature . New Zealand Journal of Geology and Geophysics , 52 : 101 – 148 .
- Allibone , AH , Jongens , R , Turnbull , IM , Milan , LA , Daczko , NR , De Paoli , MC and Tulloch , AJ . 2009b . Plutonic rocks of western Fiordland, New Zealand: field relations, geochemistry, correlation, and nomenclature . New Zealand Journal of Geology and Geophysics , 52 : 379 – 415 .
- Batt , GE , Braun , J , Kohn , BP and McDougall , I . 2000 . Thermochronological analysis of the dynamics of the Southern Alps, New Zealand . Geological Society of America Bulletin , 112 : 250 – 266 .
- Batt GE , Baldwin SL , Cottam M , Fitzgerald P , Brandon MT 2004 . Cenozoic Plate Boundary Evolution in the South Island of New Zealand: New Zealand Thermochronolocal constraints . Tectonics 23: TC4001 10.1029/2003TC001527 .
- Cawood , PA , Nemchin , AA , Leverenz , A , Saeed , A and Ballance , PF . 1999 . U/Pb dating of detrital zircons: implications for the provenance record of Gondwana margin terranes . Geological Society of America Bulletin , 111 : 1107 – 1119 .
- Chen , YD and Williams , IS . 1990 . Zircon inheritence in mafic inclusions from Bega batholith granites, southeastern Australia: an ion microprobe study . Journal of Geophysical Research , 95 : 787 – 796 .
- Claoué–Long , JC , Compston , W , Roberts , J and Fanning , CM . 1995 . “ Two Carboniferous ages: a comparison of SHRIMP zircon dating with conventional zircon ages and 40Ar/39Ar analysis ” . In Geochronology, Time Scales and Global Stratigraphic Correlation , Edited by: Berggren , WA , Kent , DV , Aubry , MP and Hardenbol , J . 3 – 21 . SEPM Special Publication .
- Cooper , RA . 1975 . Age of the Greenland and Waiuta Groups, South Island, New Zealand . New Zealand Journal of Geology and Geophysics , 17 : 955 – 962 .
- Cooper , RA . 1989 . Early Paleozoic terranes of New Zealand . Journal of the Royal Society of New Zealand , 19 : 73 – 112 .
- Cooper , RA and Tulloch , AJ . 1992 . Early Paleozoic terranes in New Zealand and their relationship to the Lachlan Fold Belt . Tectonophysics , 214 : 129 – 44 .
- Copeland , P , Parrish , RR and Harrison , TM . 1988 . Identification of inherited radiogenic Pb in monazite and its implication for U–Pb systematics . Nature , 333 : 760 – 763 .
- Cox SC , Barrell DJA 2007 . Geology of the Aoraki Area . Institute of Geological and Nuclear Sciences 1:250 000 Geological Map QMAP15 . 1 map sheet and 71p .
- Daczko , NR , Milan , LA and Halpin , JA . 2009 . Metastable persistence of pelitic metamorphic assemblages at the root of a Cretaceous magmatic arc – Fiordland, New Zealand . Journal of Metamorphic Geology , 27 : 233 – 247 .
- Gibson , GM and Ireland , TR . 1996 . Extension of Delamerian (Ross) orogen into western New Zealand: Evidence from zircon ages and implications for crustal growth along the Pacific margin of Gondwana . Geology , 24 : 1087 – 1090 .
- Gray , DR and Foster , DA . 2004 . Tectonic evolution of the Lachlan Orogen, southeast Australia: historical review, data synthesis and modern perspectives . Australian Journal of Earth Sciences , 51 : 773 – 817 .
- Harrison , TM , McKeegan , KD and LeFort , P . 1995 . Detection of inherited monazite in the Manaslu leucogranite by 208Pb/232Th ion microprobe dating: crystallisation age and tectonic implications . Earth and Planetary Science Letters , 133 : 271 – 282 .
- Ireland , TR . 1992 . Crustal evolution of New Zealand: Evidence from age distributions of detrital zircons in Western Province paragneisses and Torlesse greywacke . Geochimica et Cosmochimica Acta , 56 : 911 – 920 .
- Ireland , TR and Gibson , GM . 1998 . SHRIMP monazite and zircon geochronology of high-grade metamorphism in New Zealand . Journal of Metamorphic Geology , 16 : 149 – 167 .
- Ireland , TR , Flöttmann , T , Fanning , CM , Gibson , GM and Preiss , WV . 1998 . Development of the early Paleozoic Pacific margin of Gondwana from detrital-zircon ages across the Delamerian orogen . Geology , 26 : 243 – 246 .
- Jongens , R . 2006 . Gneissic rocks of the Bonar Range, central Westland, New Zealand: short communication . New Zealand Journal of Geology and Geophysics , 49 : 281 – 286 .
- Kamp , PJJ , Green , PF and Tippett , JM . 1992 . Tectonic architecture of the mountain front-foreland basin transition, South Island, New Zealand, assessed by fission track analysis . Tectonics , 11 : 98 – 113 .
- Kimbrough , DL and Tulloch , AJ . 1989 . Early Cretaceous age of orthogneiss from the Charleston Metamorphic Group, New Zealand . Earth and Planetary Science Letters , 95 : 130 – 140 .
- Kimbrough , DL , Tulloch , AJ and Rattenbury , MS . 1994 . Late Jurassic-Early Cretaceous metamorphic age of Fraser Complex migmatite, Westland, New Zealand . New Zealand Journal of Geology and Geophysics , 37 : 137 – 142 .
- Kingsbury , JA , Miller , CF , Wooden , JL and Harrison , TM . 1993 . Monazite paragenesis and systematics in rocks of the eastern Mojave Desert, California, U.S.A.: implications for thermochronometry . Chemical Geology , 110 : 147 – 167 .
- Klepeis , KA , Clarke , GL , Gehrels , G and Vervoort , J . 2004 . Processes controlling vertical coupling and decoupling between upper and lower crust of orogens: results from Fiordland, New Zealand . Journal of Structural Geology , 26 : 765 – 791 .
- Kula , J , Tulloch , A , Spell , TL and Wells , ML . 2007 . Two-stage rifting of Zealandia–Australia–Antarctica: Evidence from 40Ar/39Ar thermochronometry of the Sisters shear zone, Stewart Island, New Zealand . Geology , 35 : 411 – 414 .
- Laird , MG . 1967 . Field relations of the Constant Gneiss and Greenland Group in the Central Paparoa Range, West Coast, South Island . New Zealand Journal of Geology and Geophysics , 10 : 247 – 256 .
- Little , TA , Cox , S , Vry , JK and Batt , GE . 2005 . Variations in exhumation level and uplift-rate related to oblique slip ramp geometry, Alpine Fault, Central Southern Alps, New Zealand . Geological Society of America Bulletin , 117 : 707 – 723 .
- Ludwig , KR . 2003 . Isoplot 3.00 User's Manual: A Geochronological Toolkit for Microsoft Excel, Berkeley Geochronological Center, Special Publication No. 4, Rev . May , 30 : 70p
- Marcotte , SB , Klepeis , KA , Clarke , GL , Gehrels , G and Hollis , JA . 2005 . Intra-arc transpression in the lower crust and its relationship to magmatism in a Mesozoic magmatic arc . Tectonophysics , 407 : 135 – 163 .
- Mortimer , N . 2004 . New Zealand's Geological Foundations . Gondwana Research , 7 : 261 – 272 .
- Muir , RJ , Ireland , TR , Weaver , SD and Bradshaw , JD . 1994 . Ion microprobe U-Pb zircon geochronology of granitic magmatism in the Western Province of the South Island, New Zealand . Chemical Geology (Isotope Geoscience Section) , 113 ( 1 ) : 171 – 189 .
- Muir , RJ , Ireland , TR , Weaver , SD and Bradshaw , JD . 1996a . Ion microprobe dating of Paleozoic granitoids: Devonian magmatism in New Zealand and correlations with Australia and Antarctica . Chemical Geology (Isotope Geoscience Section) , 127 : 191 – 210 .
- Muir , RJ , Weaver , SD , Bradshaw , JD , Eby , GN , Evans , JA and Ireland , TR . 1996b . Geochemistry of the Karamea Batholith, New Zealand and comparisons with the Lachlan Fold Belt granites of SE Australia . Lithos , 39 : 1 – 20 .
- Muir , RJ , Ireland , TR , Weaver , SD , Bradshaw , JD , Waight , TE , Jongens , R and Eby , GN . 1997 . SHRIMP U-Pb geochronology of Cretaceous magmatism in northwest Nelson-Westland, South Island, New Zealand . New Zealand Journal of Geology and Geophysics , 40 : 453 – 463 .
- Muir , RJ , Ireland , TR , Weaver , SD , Bradshaw , JD , Evans , JA , Eby , GN and Shelley , D . 1998 . Geochronology and geochemistry of a Mesozoic magmatic arc system, Fiordland, New Zealand . Journal of the Geological Society of London , 155 : 1037 – 1053 .
- Nathan S , Anderson HJ , Cook RA , Herzer RH , Hoskins RH , Raine JI , Smale D 1986 . Cretaceous and Cenozoic sedimentary basins of the West Coast region, South Island, New Zealand . New Zealand Geological Survey Basin Studies 1 . 4 map sheets and 99p .
- Nathan S , Rattenbury MS , Suggate RP 2002 . Geology of the Greymouth Area . Institute of Geological and Nuclear Sciences 1:250,000 Geological Map QMAP12 . 1 map sheet and 58p .
- Paces , JB and Miller , JD . 1993 . Precise U-Pb age of Duluth Complex and related mafic intrusions, northeastern Minnesota: Geochronological insights to physical, petrogenetic, paleomagnetic, and tectonomagnetic processes associated with the 1.1 Ga midcontinent rift system . Journal of Geophysical Research , 98 ( B8 ) : 13997 – 14013 .
- Parrish , RR . 1990 . U-Pb dating of monazite and its applications to geological problems . Canadian Journal of Earth Sciences , 27 : 1431 – 1454 .
- Pickard , AL , Adams , CJ and Barley , ME . 2000 . Provenance of New Zealand Late Permian to Cretaceous depocentres at the Australian margin of Gondwanaland: evidence from detrital zircon SHRIMP dating . Australian Journal of Earth Sciences , 47 : 987 – 1007 .
- Pickett , DA and Wasserburg , GJ . 1989 . Neodynium and strontium isotopic characteristics of New Zealand granitoids and related rocks . Contributions to Mineralogy and Petrology , 103 : 131 – 142 .
- Rapp , RP , Ryerson , FJ and Miller , CF . 1987 . Experimental evidence bearing on the stability of monazite during crustal anatexis . Geophysical Research Letters , 14 : 307 – 310 .
- Rattenbury , MS . 1987 . Timing of mylonitisation west of the Alpine Fault, central Westland, New Zealand . New Zealand Journal of Geology and Geophysics , 30 : 287 – 297 .
- Rattenbury , MS . 1991 . The Fraser Complex: high-grade metamorphic, igneous and mylonitic rocks in central Westland, New Zealand . New Zealand Journal of Geology and Geophysics , 34 : 23 – 33 .
- Rattenbury MS , Cooper RA , Johnston MR 1998 . Geology of the Nelson Area . Institute of Geological and Nuclear Sciences 1:250 000 Geological Map QMAP9 . 1 map sheet and 67p .
- Rattenbury MS , Townsend D , Johnston MR 2006 . Geology of the Kaikoura Area . Institute of Geological and Nuclear Sciences 1:250,000 Geological Map QMAP13 . 1 map sheet and 70p .
- Simpson , RL , Parrish , RR , Searle , MP and Waters , DJ . 2000 . Two episodes of monazite crystallisation during metamorphism and crustal melting in the Everest region of the Nepalese Himalaya . Geology , 28 : 403 – 406 .
- Spell , TL , McDougall , I and Tulloch , AJ . 2000 . Thermochronologic constraints on the breakup of the Pacific Gondwana margin: The Paparoa metamorphic core complex, South Island, New Zealand . Tectonics , 19 : 433 – 451 .
- Steiger , RH and Jäger , J . 1977 . Subcommission on geochronology: convention on the use of decay constants in geo- and cosmochronology . Earth and Planetary Science Letters , 36 : 359 – 362 .
- Suggate RP , Waight T 1999 . Geology of the Kumara-Moana Area . Institute of Geological and Nuclear Sciences 1:50,000 Geological Map GM24 . 1 map sheet and 124p .
- Tulloch , AJ . 1983 . Granitoid rocks of New Zealand – a brief review . Geological Society of America Memoir , 159 : 5 – 20 .
- Tulloch , AJ . 1988 . Batholiths, plutons and suites: nomenclature for granitoid rocks of Westland-Nelson . New Zealand Journal of Geology and Geophysics , 31 : 505 – 509 .
- Tulloch , AJ and Kimbrough , DL . 1989 . The Paparoa metamorphic core complex, New Zealand; Cretaceous extension associated with fragmentation of the Pacific margin of Gondwana . Tectonics , 8 : 1217 – 1235 .
- Tulloch , AJ , Ramezani , J , Kimbrough , DL , Faure , K and Allibone , AH . 2009 . U-Pb geochronology of mid-Paleozoic plutonism in western New Zealand: Implications for S-type granite generation and growth of the east Gondwana margin . Geological Society of America Bulletin , 121 : 1236 – 1261 .
- Vry , J , Compston , W and Cartwright , I . 1996 . SHRIMP II dating of zircons and monazites: reassessing the timing of high-grade metamorphism and fluid flow in the Reynolds Range, northern Arunta Block, Australia . Journal of Metamorphic Geology , 14 : 335 – 350 .
- Waight , TE , Weaver , SD , Ireland , TR , Maas , R , Muir , RJ and Shelley , D . 1997 . Field characteristics, petrography, and geochronology of the Hohonu Batholith and the adjacent Granite Hill Complex. North Westland, New Zealand . New Zealand Journal of Geology and Geophysics , 40 : 1 – 17 .
- Wandres , AM , Bradshaw , JD , Weaver , SD , Maas , R , Ireland , TR and Eby , GN . 2004 . Provenance of the sedimentary Rakaia sub-terrane, Torlesse Terrane, South Island, New Zealand: the use of igneous clast compositions to define the source . Sedimentary Geology , 168 : 193 – 226 .
- Wandres , AM , Bradshaw , JD and Ireland , T . 2005 . The Paleozoic-Mesozoic recycling of the Rakaia Terrane, South Island, New Zealand: sandstone clast and sandstone petrology, geochemistry, and geochronology . New Zealand Journal of Geology and Geophysics , 48 : 229 – 245 .
- White , PJ . 1994 . Thermobarometry of the Charleston Metamorphic Group and implications for the evolution of the Paparoa Metamorphic Core Complex, New Zealand . New Zealand Journal of Geology and Geophysics , 37 : 201 – 211 .
- White , SH and Green , PF . 1986 . Tectonic development of the Alpine fault zone, New Zealand: A fission-track study . Geology , 14 : 124 – 127 .
- Williams , IS . 1998 . U-Th-Pb geochronology by ion microprobe . Reviews in Economic Geology , 7 : 1 – 35 .
- Wysoczanski , RJ , Gibson , GM and Ireland , TR . 1997 . Detrital zircon age patterns and provenance in Late Paleozoic Early Mesozoic New Zealand; terranes and development of the Paleo-Pacific Gondwana margin . Geology : 939 , 25 : 942