Abstract
The expansion of open pit mines beyond initial size estimates is a common occurrence in the mining industry, particularly where ore values unexpectedly increase, and where new areas of high-grade ore are identified during mining. The decision to expand a pit should be based not only on expected profits, but also on updated predictions of pit lake water quality based upon the proposed mine geometry. The shape of the pit affects several variables used to generate these predictions, including pit hydrology, wall-rock mineralogy, lake water balance, and physical limnology. A review of pre-expansion geochemical models of the Martha Mine illustrates that changes in the wall-rock composition alone may increase the predicted pH of the pit lake from <5.0 to >6.5. This example underscores the need to update pit lake water quality predictions before open pit mines are expanded in order to best manage environmental risks.
Introduction
Open pit mining involves the large-scale excavation of ore and waste rock which results in an open pit. To ensure the stability of pit walls, the diameter of the pit at the land surface must be many times wider than the width of the active mine face. In order to excavate from successively deeper layers, the diameter of the pit must be continually expanded, and more waste rock must be excavated. Consequently, open pit mines are some of the largest surface features that humans have created. Examples include the Bingham Copper Mine in Utah, USA (width 4 km, depth 1.2 km) and the Chuquicamata Copper Mine in Chile (width 4.5 km, depth 0.8 km).
At some point in the mining process, either the cost of deepening the pit and disposing of waste rock exceeds the financial profit of the processed ore, or the maximum permitted aerial footprint of the mine is achieved. In response, the mining company may decide either to: continue to mine the highest grade ore using underground tunnelling methods, file for a permit to expand the mine footprint via the local regulatory agency, or close the mine.
The closure of open pit mines typically results in the formation of mine pit lakes, especially in high rainfall/low evapotranspiration environments such as New Zealand. During mining, dewatering pumps are used to lower the local water table below the active mining surface, which prevents the open pit from flooding. Upon mine closure, pumping ceases and the water table rebounds towards its pre-mining level. The pit slowly fills with a combination of groundwater, pit wall runoff, and direct precipitation to create a pit lake. Groundwater and pit wall runoff typically react with minerals within the wall-rocks or minerals present on the wall-rock surface to produce acid mine drainage (AMD), neutral mine drainage (NMD), or basic/saline mine drainage (BMD) depending on the local geology (Verburg & Bezuidenhout Citation2009). Collectively, these varieties of mine drainage are referred to as mine impacted water (MIW) (McLemore Citation2008).
Pit lakes have the potential to collect and store large quantities of MIW. In rare cases, groundwater and/or surface water discharging from pit lakes has degraded potential drinking water resources (Savage et al. Citation2000) or negatively impacted wildlife (Hagler Bailly Consulting Citation1996). In less extreme cases, the concentration of dissolved metals in surface water may exceed water quality guidelines (e.g., Australia and New Zealand Environment and Conservation Council (ANZECC) (2000) guidelines) for one or more specific uses (e.g., recreation, irrigation, stock water), which limits the lake's usefulness. However, through careful planning and engineering, several pit lakes exhibit high water quality and have been developed into post-mining resources (McCullough et al. Citation2009). Quantitative geochemical modelling is required to predict post-mining pit lake water quality, both in advance of mining, and when mine plans change, so that mine managers and regulators can evaluate the potential environmental effects.
A common alteration to a mine plan is the expansion of an open pit mine beyond its originally permitted footprint. This expands the mine's operational life and has a direct economic benefit for both mining companies and mining communities. At the same time, pit expansion changes the input parameters used in pit lake water quality models which can modify future pit lake water quality.
This paper reviews the potential effects of mine footprint expansion on an existing pit lake prediction for the Martha Mine in Waihi, New Zealand (). The Martha Mine is a particularly good example because it has twice received approval for open pit expansion (in 1997 and 2010), and it is one of the most well-studied open pit mines in the world, with widely disseminated geo-environmental datasets and pit lake modelling results (Castendyk et al. Citation2005; Castendyk & Webster-Brown, Citation2007a ,Citationb). Building from this example, the paper discusses how various components of a pit lake prediction may be affected by mine expansion, and concludes with recommendations for mine managers, regulators, consultants, and modellers that will aid in the upgrade and evaluation of pit lake predictions.
Fig. 1 Geologic map of the Coromandel Penninsula, North Island, New Zealand, showing the location of the Martha Mine and other epithermal Au-Ag deposits in the Hauraki Goldfield (after Skinner Citation1986).
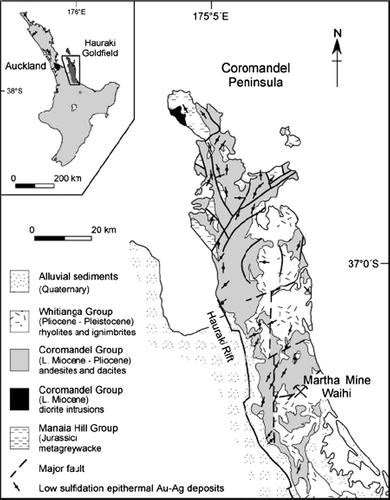
Example from the future Martha Mine pit lake
Geology and history of Martha Mine
The Coromandel Peninsula contains a sequence of late Miocene to early Quaternary subaerial, andesitic to rhyolitic volcanic rocks of the Coromandel Volcanic Zone (Skinner Citation1986; Christie et al. Citation2007) (). Potassium-argon dates suggest that regional volcanism occurred between 18.5 and 2.5 Ma as a result of northwest subduction of the Pacific plate below the Australian–Indian plate along a continental-margin, volcanic arc system (Brathwaite & Skinner Citation1997). Andesitic host rocks at Martha Mine are part of the Waipupu Formation which formed between 7.9 and 6.3 Ma according to K-Ar dates (Brathwaite & Christie Citation1996).
In association with volcanic activity, multiple shallow hydrothermal systems became active which emplaced approximately 50 epithermal Au-Ag deposits and several porphyry copper deposits from Great Barrier Island to the north, to Te Puke to the south, collectively known as the Hauraki Goldfield (Brathwaite et al. Citation1989). Hydrothermal alteration at the Martha low sulfidation epithermal Au-Ag deposit occurred within 1 Ma of volcanic deposition, and was controlled by near-vertical tension fractures that filled with quartz-adularia veins containing Au and Ag ore minerals, electrum and acanthite (Brathwaite & Faure Citation2002). Pyrite is the most abundant sulfide in the deposit, although sphalerite, galena, chalcopyrite, tetrahedrite, molybdenite, and pyrrhotite have also been reported (Christie Citation1982; Brathwaite & McKay Citation1989; Panther et al. Citation1995; Brathwaite & Faure Citation2002). Hydrothermal fluids altered the parent andesite to rocks that contain quartz, adularia, chlorite, illite, pyrite, calcite, albite, and kaolinite (Brathwaite Citation1980; Brathwaite & McKay Citation1989; Jennings et al. Citation1990; Brathwaite & Faure Citation2002).
Castendyk et al. (Citation2005) quantified the wall-rock mineralogy of the pit in 2003 using a novel mineral quantification method, and mapped zones of mineral assemblages in the wall-rocks. They identified two areas of hydrothermally-altered andesite, called ‘fresh-argillic’ and ‘weathered-argillic’, which had average pyrite concentrations of 8 wt% and 6 wt%, respectively. The fresh-argillic rocks contained quartz, kaolinite, chlorite, illite, pyrite, and gypsum, whereas the weathered-argillic rocks contained quartz, illite, chlorite, kaolinite, alunite, and pyrite. Runoff from these zones had a pH of 3.1 and elevated concentrations of iron (18.3 mg/L), aluminium (7.23 mg/L), manganese (1.22 mg/L), zinc (0.199 mg/L), and nickel (0.17 mg/L); characteristic of AMD (Castendyk & Webster-Brown Citation2007a).
The Martha Mine is a world-class epithermal Au mine. Underground mining began in 1878 and continued until 1952. Historic mine shafts extended 600 m below the land surface, and connected over 175 km of horizontal mine tunnels (Newmont, Citation2010). By 1952, the Hauraki Goldfield had produced approximately 1.37 million kg of Au-Ag bullion, with 1.22 million kg of Au-Ag bullion produced from the Martha Mine alone (Brathwaite et al. Citation1989; Brathwaite & Faure Citation2002). Mining recommenced at the Martha Mine in 1988 with the excavation of an open pit. The previous mine owners, Waihi Gold Company, filed for an extension to mine activities in 1997 when the originally permitted surface area footprint had been reached. As part of this permit application, the company provided information on the potential environmental impacts of the proposed expansion, including a detailed prediction of pit lake geochemistry (Geochimica Citation1997). Under new ownership, Newmont Waihi Gold is expanding the width of the pit by excavating a layback in the east wall within the current licensed area from 2010 to 2014. To plan for this expansion, the company updated the pit lake water quality prediction using current pit wall runoff geochemistry, revised geological and wall-rock alteration data resulting from changes in pit geometry, and various mitigation scenarios. The company also received an external peer review of this prediction (Pieter Fransen, Newmont Waihi Gold, pers. comm. 2010).
Predicted pH prior to the 2010 expansion
Upon mine closure, Newmont Waihi plans to fill the open pit with fresh water diverted from the adjacent Ohinemuri River and to rehabilitate the pit lake into a public recreation area (Waihi Gold Company Citation1997; Ingle Citation2002). Using the 1997 pit design, Woodward Clyde (Citation1997a) estimated a filling period of roughly 5 years before the lake surface achieved a steady-state discharge elevation. Such a lake will ultimately be over 200 m deep with a surface area just over 28.7 ha and a volume of approximately 2.16×107 m3. By combining a water balance and a groundwater inflow model (Woodward Clyde Citation1997a), with a numerical prediction of future lake circulation (Woodward Clyde 1997b), Geochimica (1997) predicted that the pH of the surface layer, or epilimnion, would drop from 7.8 to 7.5 over a 50 yr period, whereas the pH of the middle layer, or hypolimnion, would drop from 6.5 to 6.4 over the same period.
Castendyk & Webster-Brown (2007a, b) developed a revised prediction of lake circulation that included a combination of seasonal stratification and mixing in shallow lake water (i.e., the epilimnion and hypolimnion layers), and permanent stratification in bottom lake water (i.e., the monimolimnion layer). These limnologic conditions were integrated into a new geochimical prediction of lake water quality, one which also allowed for the presence/absence of water-rock reactions with submerged wall-rock. In the absence of these water-rock reactions, they predicted that epilimnion and hypolimnion water pH would drop from initial values of 6.5 and 6.0 respectively to 4.8 over a 55 yr period as a result of the addition of acidic surface runoff from the fresh- and weathered-argillic wall-rock exposed above the lake surface (Model 1, ). Allowing for water-rock reactions further decreased pH by a degree that was dependent upon the reactive surface area of wall-rock minerals (Model 2b multiplied a conservative estimation of mineral surface area by a factor of 10; Model 2c multiplied this area by 100; and Model 2d multiplied this area by 1000; ). It was concluded that the Martha pit lake would exhibit lower water quality than had been initially predicted by Geochemica (1997) as a result of the incremental addition of AMD over time coupled with some degree of water-rock reaction.
Fig. 2 Fifty-five year prediction of Martha lake showing a progressive decrease in lake pH over time (after Castendyk & Webster 2007b). Years −5 to 0 represent lake filling conditions. Layer 1 represents shallow, epilimnion water whereas Layer 2 represents deeper, hypolimnion water. Model 1 considers only mixing reactions between pit wall runoff, groundwater, and direct rainfall. Models 2b, 2c, and 2d consider mixing reactions plus increasing quantities of wall-rock minerals reacting with lake water. ANZECC (Citation2000) guidelines for aesthetic drinking water use and recreational use are provided for comparison.
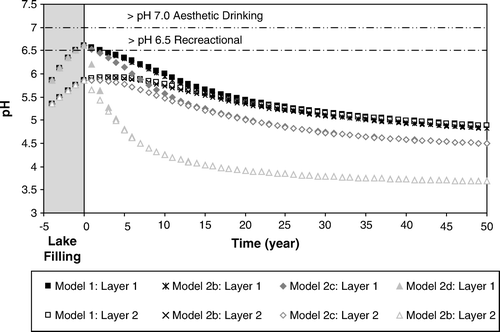
Sensitivity of predicted pH to wall rock modification
As the Martha Mine grows in size, it is possible that zones of fresh- and weathered-argillic wall-rock will be mined out or covered in an effort to improve pit lake water quality thereby reducing the volume of AMD added to the lake. Model 3a in illustrates the effect of removing or covering all fresh- and weathered-argillic wall-rocks throughout the pit in advance of mine closure. This hypothetical action had a profound impact on the water quality of the resulting pit lake, increasing surface water pH from 4.9 to 6.9 after 55 yr (Castendyk & Webster-Brown Citation2006).
Fig. 3 Comparison of 55-year pH predictions for Martha Lake resulting from variable wall-rock compositions (modified from Castendyk & Webster-Brown Citation2006). Model 1 shows the baseline geochemical prediction illustrated in for both the epilimnion (Layer 1) and hypolimnion (Layer 2). Model 3a reflects the removal or covering of fresh- and weathered-argillic rocks throughout the pit prior to lake filling. Model 3b reflects the removal or covering of fresh- and weathered-argillic rocks above the pit lake surface after steady-state lake levels are achieved. These models are based upon wall-rock mineral data reported by Castendyk et al. (Citation2005) and do not represent an updated wall-rock evaluation. ANZECC (Citation2000) guidelines for aesthetic drinking water use and recreational use are provided for comparison.
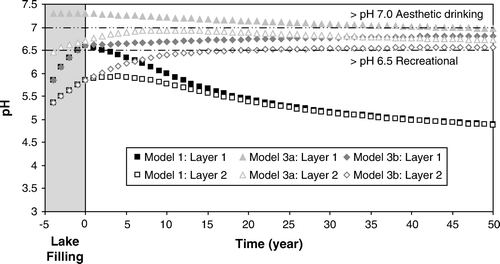
In Model 3b, fresh- and weathered-argillic wall-rocks above the ultimate pit lake surface elevation are either removed or covered once the pit lake floods to its steady-state elevation after 5 yr (). This modification produced an identical pH as Model 1 during lake filling, but increased surface water pH from 6.5 to 6.8 after 50 yr of steady-state hydrologic conditions. Both Model 3a and 3b produced water that is acceptable for recreational use according to the ANZECC (Citation2000) guidelines, which will assist the mining company in achieving its post-closure objectives for the pit lake. Whereas the company plans to develop the pit lake into a recreation area (Waihi Gold Company Citation1997; Ingle Citation2002), the closure criteria for the mine are based upon the water quality of the receiving environment and not the water quality of pit lake (Pieter Fransen, Newmont Waihi Gold, pers. comm. 2010). Model 3b demonstrates that significant improvements to water quality can be generated by focusing mitigation efforts on fresh- and weathered-argillic rocks exposed above the lake surface rather than mitigating these rocks throughout the pit (i.e., Model 3a), thereby reducing mitigation costs. This finding is consistent with recent modelling conducted by the mining company (Pieter Fransen, Newmont Waihi Gold, pers. comm. 2010).
It must be noted that Models 1, 2, and 3 are based on the 1997 predictions of the shape of the pit upon mine closure, hydrology, and water balance combined with the wall-rock mineralogy measured by Castendyk et al. (Citation2005). They do not represent the updated prediction of pit lake water quality conducted by the mining company for the 2010 expansion. It is interesting to note that the mining company's latest remediation results are not significantly different from (Pieter Fransen, Newmont Waihi Gold, pers. comm. 2010). Nevertheless, the pH shift between Model 1 and Model 3 illustrates how changes to one variable used in pit lake predictions (i.e., wall-rock mineralogy) can significantly affect predicted water quality. This finding supports the need for updating pit lake predictions when open pit mines are expanded.
Effects of mine expansion on pit lake predictions
As the Martha Mine example demonstrates, pit lakes are complex geochemical systems and the prediction of future pit lake water quality requires consideration of multiple interrelated components, most notably hydrology, physical limnology, and geochemistry. A recent publication by the Acid Drainage Technology Initiative, Metal Mining Sector (ADTI-MMS), based in the United States, provides one of the first comprehensive texts on pit lake characterisation, predictive modelling, and sustainable development (Castendyk & Eary Citation2009a). These authors used a pyramid structure to illustrate the relationship between each component in a pit lake prediction, as shown in . Mine geology, local climate, and local hydrology represent the initial conditions of any ore deposit, from which all mine decisions and water quality predictions are based. Modifications to components at the bottom of the pyramid influence the resulting water quality prediction at the top.
Fig. 4 Conceptual relationship between the principal components that affect pit lake water quality as considered in the scientific literature (modified from Castendyk & Eary Citation2009b).
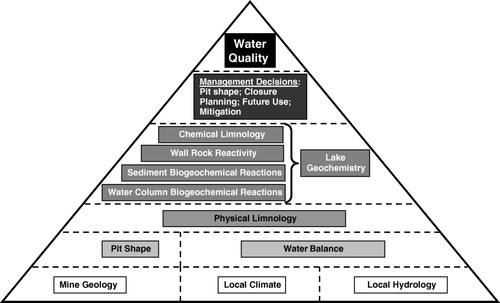
Mine geology and pit shape
By definition, mine expansion involves increasing the dimensions of the existing open pit. Numerical models of hydrology and physical limnology use the dimensions of the open pit specified by a mining company to develop a 1-D, 2-D or 3-D finite-element or finite-difference grid that represents the geometry of the open pit upon mine closure. Grid design is a labour-intensive process for modellers who recognise that inaccuracies in the shape of the grid can generate inaccuracies in the resulting prediction. Many groundwater (e.g., MODFLOW (United States Geological Survey, Reston, Virginia, USA); Harbaugh et al. Citation2000) and limnology (e.g., DYRESM (Centre for Water Research, University of Western Australia, Perth, Australia); Imberger & Patterson Citation1981) modelling programs are written in such a way that makes it very difficult, if not impossible, to modify an existing grid design without causing the model to fail or creating inaccuracies. Consequently, a request by a mining company to update the original hydrologic prediction to account for a larger pen pit can require significant additional work and/or require the modeller to assume that the grid, hydrologic properties, and boundary conditions which applied to the original pit design are appropriate. In some cases, existing models may need to be abandoned and entirely new models created to ensure the most accurate results. This is particularly true when mining companies propose expansion plans more than a decade after initial models were designed, when the institutional knowledge of that model (i.e., the original modeller) is no longer available, and/or when modelling software has been updated in the interim.
A second consideration of mine expansion is the modification of wall-rock minerals exposed at the water–rock interface. As shown for the Martha Mine, this can be environmentally advantageous if reactive acid-producing minerals are removed during mine expansion, thereby removing the source of AMD, or if acid-neutralising minerals become exposed. Depending upon the geology of the deposit, expansion could also increase the surface area of un-weathered, un-oxidised, acid-producing minerals exposed in the pit wall, particularly where a mine in a shallow oxidised ore body is expanded into deeper un-oxidised rocks. Fortunately, drilling and geochemical sampling, combined with mineral quantification (see Castendyk et al. Citation2005), can define the future wall-rock mineralogy in advance of mine expansion so that the effects of expansion can be evaluated.
Local hydrology and water balance
In general, excavating deeper requires a mining company to deepen and widen the hydrologic cone of depression surrounding the mine by increasing the pumping rate. During mining, this increases the volume of water that requires treatment before being discharged to the surface environment. Upon mine closure, a larger volume of water must be added to the pit before steady-state hydrologic conditions are achieved. Naturally, this will extend the projected time required to rehabilitate a mine site.
For example, under the 1997 mine plan, the Martha Mine would take approximately 5 yr to fill to a steady-state lake level (Woodward Clyde Citation1997a). Under the 2010 plan, a longer fill time, and/or more water diverted from the Ohinemuri River, may be anticipated to compensate for the additional lake volume. The mine permit does not require Newmont Waihi Gold to fill the pit in a 5 yr period, nor is this expected by regulators. Moreover, the Martha Mine is not being deepened, only widened, such that the pumping rate will not increase. Expansion will not change the rates of groundwater or river water inflow during lake filling. Instead, there will be an increase in the net precipitation rate owing to the increased lake surface area, a decrease in the pit wall runoff rate, and a negligible decrease in the average daily lake filling rate (Pieter Fransen, Newmont Waihi Gold, pers. comm. 2010).
The effects of historic mine tunnels and shafts are often overlooked in groundwater models which sometimes assume homogeneous hydraulic conductivity in wall-rocks. Historic workings can include multiple levels of horizontal tunnels below the open pit which are connected by vertical shafts. With a higher hydraulic conductivity than the surrounding rock, these tunnels are likely to behave as preferential groundwater flow paths capable of transmitting large volumes of groundwater to the pit over a comparatively short time period. For open pit mines that have been previously mined underground, deepening the pit may increase the number of tunnel levels which intersect the pit wall and potentially modify the predicted groundwater input and lake filling rates.
In the case of the Martha Mine, the original underground mine had 15 horizontal levels connected by vertical shafts that extended up to 600 m from the surface (Newmont Citation2010). However, because expansion will not deepen the pit, the number of tunnels intersecting the pit wall will remain the same and the predicted groundwater input rate will not be affected (Pieter Fransen, Newmont Waihi Gold, pers. comm. 2010).
Deepening and widening the cone of depression may also change the chemical composition of water being added to the pit by tapping groundwater from deeper aquifers and/or groundwater originating further from the pit. The addition of new source water with a higher concentration of total dissolved solids (i.e. salinity), or a change in the volume of water added from deeper sources with higher salinity, could significantly modify both the limnologic and geochemical predictions. Finally, lowering the cone of depression can introduce oxygen into the rocks surrounding the mine which, depending on the local geology, may contain un-oxidised rocks with sulfide minerals. This can potentially stimulate the acidification of groundwater and/or wall-rock seepage.
At the Martha Mine, groundwater is pumped from a vertical shaft at the base of the mine. This deep groundwater is warmer and has higher salinity than shallow groundwater adjacent to the pit (Woodward Clyde Citation1997c), indicating that multiple aquifers may be providing water to the mine. The salinity of the deep groundwater inputs affects the limnology of the future pit lake, as discussed below.
In summary, mine expansion typically creates the need for a new water balance analysis for a mine. This water balance must account for changes in groundwater input volumes as the lake fills, changes in the volume of surface runoff added to the pit owing to an enlarged mine footprint, and changes to the direct precipitation added to the pit owing to larger lake surface area.
Physical limnology
Modellers use physical limnology models to define stratification (i.e. the depth and frequency of vertical mixing events) within pit lakes. These models require an accurate description of the 3-D geometry of the lake upon mine closure plus a detailed water balance that includes the volume, temperature, and salinity of surface water and groundwater inputs/outputs. As previously stated, changes to the size and shape of the pit will directly affect limnologic predictions.
There is a perception that pit lakes have a greater potential to permanently stratify than natural lakes because of their unique shape. The ratio of maximum depth to surface area, expressed by Hutchinson (Citation1957) as relative depth, tends to be higher in pit lakes than in natural lakes, which suggests that pit lakes require more wind energy to undergo complete vertical circulation than natural lakes with equal surface area (Doyle & Runnels Citation1997). However, recent observations of permanently stratified lakes have found little correlation between lake morphology and circulation patterns (Castendyk & Webster-Brown Citation2007b; Boehrer & Schultz, Citation2009). Instead, the density of water from various sources (e.g., pit wall runoff, shallow groundwater, deep groundwater, river water, and rain water), and the volumetric contribution of each source to the lake over time, appear to have a greater influence on the establishment and maintenance of permanent stratification than pit lake morphology. Therefore, simple predictions of future circulation involving relative depth or other depth-to-surface-area ratios can provide unreliable predictions of future circulation. Numerical limnologic models have shown strong correlations between predicted and observed circulation in existing pit lakes, and can provide a more accurate prediction of future limnology than morphology-based predictions alone. Such models require an accurate description of the pit shape upon mine closure.
For the Martha Mine, Castendyk & Webster-Brown (Citation2007b) showed that groundwater pumped from the bottom of the pit has a significantly greater density than surface waters (i.e. pit wall runoff, river water, and direct precipitation) on account of its elevated salinity. By incorporating this observation into a limnologic model, they predicted that the discharge of dense groundwater to the lake bottom during lake filling would lead to permanent stratification in the Martha pit lake. This may be advantageous should the mining company wish to consider utilising the permanently isolated monimolimnion water to sequester metals adsorbed onto suspended particulates settling downward from shallow water layers—a process that has been successfully engineered at the permanently stratified Island Copper Pit Lake in Canada (Pelletier et al. Citation2009).
In addition, Ivey et al. (Citation2004) demonstrated that it is important to consider the depth of groundwater inputs and outputs to a lake as these discharge zones can also influence the physical limnology. For this reason, updated limnologic models should account for new groundwater discharge zones (e.g., historic workings and major fractures) which may become exposed during mine expansion.
Recommendations
Significant modifications to the width and depth of an open pit mine can affect the exposed wall-rock mineralogy, groundwater inputs, lake water balance, and future physical limnology of a pit lake, and thereby modify future water quality. The example from the Martha Mine demonstrates the sensitivity of an existing water quality prediction to changes in wall-rock mineralogy alone.
Updated geochemical predictions that account for changes in pit shape, geology, hydrology, and limnology can be powerful tools for informing management decisions. These models can provide ‘what if’ scenarios that allow mine managers to identify cost-effective strategies that meet closure objectives for pit lake water quality. To maximise the potential of the predictions requires an active approach to pit lake modelling on the part of a mining company whereby each component of the initial prediction is updated as major changes to the mine shape are proposed and implemented. Mining companies, regulators, consultants, and modellers should be mindful of the potential for future expansions to mining activities when initial modelling is undertaken, even if this appears unlikely at the time. Mine managers should appreciate that modifications to the mine shape are difficult to integrate into pre-existing models and may require new models to be generated. Moreover, managers need to be aware that adjustments to the geochemical component of a pre-existing prediction alone may lead to inaccuracies between predicted and observed water quality.
For their part, modellers need to clearly and explicitly state simplifying assumptions used to generate predictions as these can generate errors between predicted and observed water quality. Like all predictive models, the accuracy of pit lake water quality predictions is dependent upon the accuracy of the input data used to construct the prediction ().
At the same time, environmental regulators and the general public need to have realistic expectations of the accuracy of pit lake predictions. Models are simplified representations of the natural world which require modellers to make simplifying assumptions about complex natural systems (Anderson & Woessner Citation1992). Therefore, it is unrealistic to expect that predicted water chemistry will match observed water chemistry with 100% accuracy. Instead, regulators and the public should expect pit lake predictions to provide a likely range of water quality values that will exist in the future (Eary & Castendyk Citation2009).
Acknowledgements
We wish to thank Newmont Waihi Gold (NWG) for permission to study the Martha Mine and for permission to publish our findings. In particular, we are grateful for the comments provided by Pieter Fransen, Environmental Manager NWG, which greatly improved the paper. We give special thanks to Jeff Mauk at The University of Auckland, and to an anonymous reviewer for their constructive editorial feedback. Finally, we wish to thank Fulbright New Zealand, The University of Auckland, and The State University of New York, College at Oneonta, for supporting this research.
References
- Anderson , MP and Woessner , WW . 1992 . Applied groundwater modeling: simulation of flow and advective transport , San Diego : Academic Press .
- ANZECC 2000 Australian and New Zealand guidelines for fresh and marine water quality Wellington , Ministry for the Environment .
- Boehrer B , Schultz M 2009 . Stratification and circulation of pit lakes . In : Castendyk DN , Eary LE . Mine pit lakes: characteristics, predictive modeling, and sustainability . Littleton, Colorado , Society for Mining, Metallurgy, and Exploration . 45 59 .
- Brathwaite , RL . 1980 . Petrology of hydrothermally altered rocks from the Waihi mines , Wellington : New Zealand Geological Survey. Report M80 .
- Brathwaite RL , Christie AB , Skinner DNB 1989 . The Hauraki Goldfield – regional setting, mineralization and recent exploration . In : Kear , D Mineral deposits of New Zealand. Australasian Institute of Mining and Metallurgy. Monograph 13 . Parville , Victoria, , Australia , 45 56 .
- Brathwaite RL , McKay D 1989 . Geology and exploration of the Martha Hill gold–silver deposit In Kear , D. Mineral Deposits of New Zealand. Australasian Institute of Mining and Metallurgy, Monograph 13 . Parville , Victoria, , Australia , 83 88 .
- Brathwaite , RL and Christie , AB . 1996 . Geology of the Waihi area. Institute of Geological & Nuclear Sciences Scale 1:50,000 Geological Map 21 , Lower Hutt, , New Zealand : Institute of Geological & Nuclear Sciences .
- Brathwaite RL , Skinner , DNB 1997 . The Coromandel epithermal gold–silver province: a result of collision of the Northland and Colville volcanic arcs in northern New Zealand . Proceedings, New Zealand Minerals & Mining Conference, Auckland, New Zealand . 111 117 .
- Brathwaite , RL and Faure , K . 2002 . The Waihi epithermal gold–silver-base metal sulfide-quartz vein system, New Zealand: temperature and salinity controls on electrum and sulfide deposition . Economic Geology , 97 : 269 – 290 .
- Castendyk , DN , Mauk , JL and Webster , JG . 2005 . A mineral quantification method for wall rocks at open pit mines, and application to the Martha Au-Ag mine, Waihi, New Zealand . Applied Geochemistry , 20 : 135 – 156 .
- Castendyk DN , Webster-Brown JG 2006 . Geochemical prediction and remediation options for the proposed Martha Mine Pit Lake, New Zealand . In : Barnhisel R . Proceedings, 7th International Conference on Acid Rock Drainage . Lexington, Kentucky , American Society for Mining and Reclamation . 306 324 .
- Castendyk , D and Webster-Brown , J . 2007a . Sensitivity analysis in pit lake prediction, Martha Mine, New Zealand 2: geochemistry, water–rock reactions, and surface adsorption . Chemical Geology , 244 : 56 – 73 .
- Castendyk , D and Webster-Brown , J . 2007b . Sensitivity analysis in pit lake prediction, Martha Mine, New Zealand 1: relationship between turnover and input water density . Chemical Geology , 244 : 42 – 55 .
- Castendyk DN , Eary LE . 2009a . Mine pit lakes: characteristics, predictive modeling, and sustainability . Littleton, Colorado , Society for Mining, Metallurgy, and Exploration .
- Castendyk DN , Eary LE 2009b . The nature and global distribution of pit lakes . In : Castendyk DN , Eary LE . Mine pit lakes: characteristics, predictive modeling, and sustainability . Littleton, Colorado , Society for Mining, Metallurgy, and Exploration . 1 11 .
- Christie AB 1982 . Fluid inclusions, stable isotopes and geochemistry of porphyry copper and epithermal vein deposits of the Hauraki Gold–Silver Province, New Zealand . Unpublished PhD thesis , Victoria University of Wellington , Wellington .
- Christie , AB , Simpson , MP , Brathwaite , RL , Mauk , JL and Simmons , SF . 2007 . Epithermal Au-Ag and related deposits of the Hauraki Goldfield, Coromandel Volcanic Zone, New Zealand . Economic Geology , 102 : 785 – 816 .
- Doyle , GA and Runnells , DD . 1997 . Physical limnology of existing mine pit lakes . Mining Engineering , 49 : 76 – 80 .
- Eary LE , Castendyk DN 2009 . The state of the art of pit lake research . In : Castendyk DN , Eary LE . Mine pit lakes: characteristics, predictive modeling, and sustainability . Littleton, Colorado , Society for Mining, Metallurgy, and Exploration . 275 289 .
- Geochimica 1997 . Hydrogeochemistry of the pit lake, extended project, Martha Hill Mine, Waihi, New Zealand . Unpublished report. Waihi, Waihi Gold Company .
- Hagler Bailly Consulting 1996 . Supplemental injury assessment report: Clark Fork River NPL sites NRDA, lethal injuries to snow geese, Berkeley pit, Butte, MT. Helena . Montana , Montana Natural Resource Damage Assessment Litigation Program .
- Harbaugh AW , Banta ER , Hill MC , McDonald MG 2000 . MODFLOW-2000, the US Geological Survey modular ground-water model – User guide to modularization concepts and the ground-water flow process . United States , United States Geological Survey. Open-File Report 00-92 : 121 p.
- Hutchinson , GE . 1957 . A treatise on limnology I: geography, physics, and chemistry , New York : John Wiley & Sons .
- Imberger J , Patterson JC 1981 . A dynamic reservoir simulation model – DYRESM:5 . In : Fischer H. . Transport models for inland and coastal waters . New York , Academic Press . 310 361 .
- Ingle DA 2002 . Newmont Waihi Operations – an overview . Proceedings, Australasian Institute of Mining and Metallurgy Conference, 1–4 September 2002, Auckland, New Zealand 105 109 .
- Ivey GN , Oldham CE , Huber A , Wake G 2004 . Hydrodynamic processes in a Western Australian coal mine pit lake . In: Proceedings of Pit Lakes 2004, Reno, Nevada. United States, United States Environmental Protection Agency. United States Environmental Protection Agency Report EPA/C-06/002 .
- Jennings K , Browne PRL , Clarke DS , Brathwaite RL 1990 . Aspects of hydrothermal alteration at the Waihi epithermal Au-Ag deposit, New Zealand . Proceedings, 12th New Zealand Geothermal Workshop, 1990, University of Auckland, New Zealand . 237 242 .
- McCullough C , Hunt D , Evans L 2009 . Sustainable development of open pit mines; creating beneficial end uses . In : Castendyk DN , Eary LE . Mine pit lakes: characteristics, predictive modeling, and sustainability . Littleton, Colorado , Society for Mining, Metallurgy, and Exploration . 249 267 .
- McLemore VT . 2008 . Basics of metal mining influenced water . Littleton, Colorado , Society for Mining, Metallurgy, and Exploration .
- Newmont 2010 . History of Martha. Newmont Waihi Gold . www.marthamine.co.nz/orig_martha.html (accessed 22 March 2010) .
- Panther CA , Mauk JL , Arehart GB 1995 . A petrographic and oxygen isotope study of banded epithermal veins from the Martha Hill Au-Ag Mine, Waihi, New Zealand . In : Mauk JL , St George JD . Proceedings, 1995 PACRIM Congress . Carlton, , Australia , Australasian Institute of Mining and Metallurgy . 447 452 .
- Pelletier CA , Wen M , Poling GW 2009 . Flooding pit lakes with surface water . In : Castendyk DN , Eary LE . Mine pit lakes: characteristics, predictive modeling, and sustainability . Littleton, Colorado , Society for Mining, Metallurgy, and Exploration . 187 202 .
- Savage , KS , Bird , DK and Ashley , RP . 2000 . Legacy of the California gold rush: environmental geochemistry of arsenic in the Southern Mother Lode Gold District . International Geology Review , 42 : 385 – 415 .
- Skinner , DNB . 1986 . Neogene volcanism of the Hauraki volcanic region . Royal Society of New Zealand Bulletin , 23 : 21 – 47 .
- Verburg R , Bezuidenhout N . 2009 . The global acid rock drainage guide . International Network for Acid Prevention, Melbourne . www.gardguide.com (accessed 1 November 2009) .
- Waihi Gold Company 1997 . Martha Mine extended project: assessment of environmental effects . Unpublished report . Waihi , Waihi Gold Company .
- Woodward Clyde 1997a . Pit lake water balance and preliminary lake outlet design, Martha Mine Extension . Unpublished report . Waihi , Waihi Gold Company .
- Woodward Clyde 1977b . Density stratification and mixing in the proposed Waihi pit lake . Unpublished report . Waihi , Waihi Gold Company .
- Woodward Clyde 1997c . Mine dewatering study, Martha Mine Extension . Unpublished report . Waihi , Waihi Gold Company .