Abstract
A dark-green nephrite boulder collected from Pleistocene glacial outwash gravels, West Coast of New Zealand, has a 6.1 cm thick weathering rind composed of an inner pale-green-white bleached zone (3.5 cm) and an outer brown oxidised zone (2.6 cm). The primary mineralogy of the boulder is monomineralic actinolite with small amounts of secondary Al-substituted goethite within the weathering-rind. Outwards from the unweathered nephrite core, SiO2, FeO, MgO, CaO, Na2O and density decrease, while Fe2O3, Al2O3, Cr, H2O+, H2O− and bulk oxidation ratio increase. The generalised weathering reaction can be characterised as: actinolite+H2O+H+=Fe-hydroxide+silicic acid+(Ca2+, Mg2+, Na+)aq. Assuming that alumina was immobile during weathering, isocon analysis indicates a mass decrease of −24% and a volume change of −26% in the bleached zone and a mass decrease of −52% and volume change of −58% in the oxidised zone with 7–84% depletion of all elements except Fe3+ and H2O− which indicates gains of 47–51% and 42–64%, respectively. Using H2O− values to estimate relative porosity, increasing Fe2O3, Al2O3, Cr and oxidation ratio and decreasing SiO2, FeO, CaO, MgO and rock density occur with increasing H2O−. Non-linear trends suggest that higher porosity created by actinolite dissolution results in an exponential increase in the amount of weathering (consistent with dissolution and secondary precipitation). An abrupt change in nephrite density at H2O+ values between ~2.38 and 2.55 wt % is probably related to incipient dissolution and cation loss as well as lower amounts of Fe-hydroxide formation. Depending on the deposition age of the gravels from which the nephrite boulder was derived, the weathering rind may be the result of 360–250 ka of weathering. The intensity of the green colouration of the nephrite reflects total iron content and oxidation ratio (mol.2Fe2O3×100/[2Fe2O3+FeO]). Combined with compositional data of other West Coast nephrites, a colour change from green or dark-green (unweathered) to pale-green-white (weathered) occurs where the oxidation ratio is >7; a change to brown colour occurs where oxidation ratios are >17.
Introduction
Weathering rinds are coloured/oxidation-hydration crusts or layers developed on rock fragments formed by weathering and have been investigated from a large number of rock types, e.g. granite, granodiorite, quartz porphyry, andesite, basalt, sandstone, gneiss and hornfels (see of Oguchi Citation2001 for references). While particular attention has been given to using weathering rind thickness to estimate the ages of Quaternary deposits, other studies have documented mineralogical, chemical, chromatic and mechanical properties of weathering rinds (Coleman & Pierce Citation1981; Coleman Citation1982, Matsukura et al. Citation1994; Oguchi & Matsukura Citation1999; Oguchi Citation2001). Several processes of weathering rind formation have been proposed, e.g. loss of mobile elements and concentration of immobile elements (Coleman Citation1982); dissolution only (Dixon et al. Citation2002); both oxidation and dissolution (Oguchi & Matsukura Citation1999; Oguchi Citation2001 , Citation2004); and biochemical processes, particularly in regard to the formation of iron-rich rock coatings (Etienne Citation2002; Etienne & Dupont Citation2002).
Table 1 EPMA analyses and formulae* of actinolite in nephritic greenstone
The above-mentioned studies are concerned with weathering of polymineralic rocks composed of various mixtures of quartz, feldspars, hydrous and anhydrous mafic silicates and oxides, and obviously involve complex physical and chemical processes related to differences in rates of mineral dissolution and breakdown reactions and in weathering products (Sak et al. Citation2004). The weathering process may be more clearly elucidated if the weathered rock is largely composed of a single mineral, e.g. olivine or pyroxene as in ultramafic rocks such as dunite and pyroxenite, or tremolite-actinolite as in nephrite. While some work has been carried out on the mechanism of olivine dissolution during the weathering of dunite (Velbel & Ranck Citation2008), we are unaware of any geochemical studies of naturally weathered dunite or pyroxenite and there are few on the weathering of nephrite (Tsein & Fang Citation2002; Wilkins et al. Citation2003). In this paper, for the first time, we examine the geochemistry and discuss the mechanism of formation of a conspicuous colour-contrasted weathering rind developed on a boulder of nephrite composed of monomineralic actinolite from New Zealand.
New Zealand nephrite, its distribution and source
In New Zealand, nephrite (Finlayson Citation1909; Turner Citation1935) is termed ‘pounamu’ by the indigenous Maori people and commonly referred to as ‘New Zealand jade’ (Beck Citation1984) or simply as ‘greenstone’ (Chapman Citation1892). In this paper, we use the term ‘nephrite’. In northern Westland, South Island, New Zealand nephrite occurs as rare pebbles and boulders (weighing up to several tons) in: glacial outwash gravels and till; in streams that drain these gravels; in major rivers (mainly the Taramakau and Arahura Rivers and, in minor amounts, the Hokitika River) into which these streams enter; and in tributary streams that drain the nephrite source area of the Pounamu ultramafic rocks, northern part of the Southern Alps () (Johnston Citation1983; Beck Citation1984 , Citation1991; Adams et al. Citation2007).
Fig. 1 Map of the north Westland, South Island of New Zealand (inset map provides location) showing distribution of weathered, slightly weathered and little weathered glacial outwash gravels and till that contain rare nephrite boulders; the distribution of streams that drain the glacial gravels; major rivers (Taramakau, Arahura, Hokitika) in which nephrite boulders have been found; and location of the Pounamu Ultramafics within biotite-garnet zone schist of the Southern Alps from which nephrite is derived (after Warren Citation1967; Nathan et al. Citation2002). Cross section A–B shows glacial outwash gravels and till deposited on Tertiary basement rocks during the Nemona (c. 360 ka), Waimaunga (300–248 ka) and Waimea (190–130 ka) Glacial periods is modified from Suggate & Watters (Citation1991).
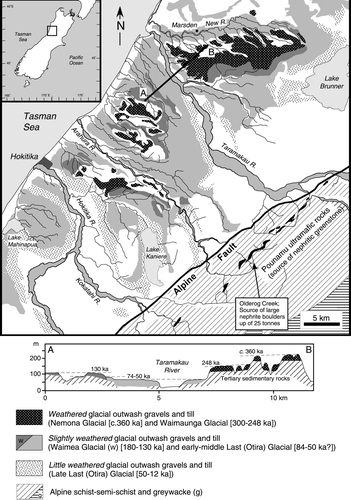
Small in situ occurrences of nephrite or partly nephritised tremolite-actinolite schist in the Southern Alps are rarely developed at the margins of serpentinised ultramafic bodies (the Pounamu Ultramafics; ) and are considered to be of metasomatic origin (Bell & Fraser Citation1906, p. 74; Coleman Citation1966; Cooper Citation1976; Koons Citation1981; Cooper and Reay Citation1983; Ireland et al. Citation1984). The West Coast nephrite has a felted, nephritic microstructure that is interpreted to be the result of late- to post-metamorphic shearing and recrystallisation of tremolite-actinolite rocks (Finlayson Citation1909; Turner Citation1935; Cooper Citation1995) to produce non-schistose nephrite and semi-nephrite. Such boulders are typically dark green but when weathered can exhibit diverse colours of brown, pale green, cream and white conspicuously present as weathering rinds.
Sample description and inferred locality
This study was made on a block cut from a weathering rind into the interior of a nephrite boulder supplied by the ‘Jade Factory’ at Hokitika, West Coast, New Zealand. The sample is a 7×3.8×0.9 cm slab cut from the flat face of a halved boulder of about 20 cm diameter. The outer 6.1 cm consists of a weathering rind that encloses a dark-green (Maori ‘kawakawa’ variety) unweathered core. The weathering rind is composed of nine clearly defined coloured layers varying in thickness from 1.2 to 0.2 cm as shown in ; contacts between the layers are irregularly sinuous with a wave amplitude of 2–3 mm.
Fig. 2 Section through weathering rind of a nephrite boulder described in this study. Numbers refer to analyses listed in .
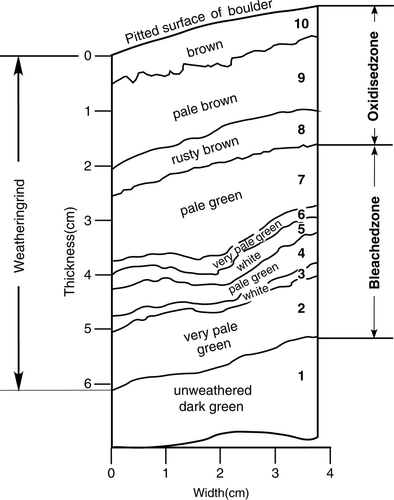
For commercial reasons, the locality of the boulder and details of its occurrence were not provided although it was reported to have been found in the Marsden-New River area of North Westland (). This general locality is well known for finds of conspicuous weathered nephrite boulders, i.e. they are characterised by well-developed brownish weathering rinds (Beck Citation1984 , Citation1991). Surfaces of nephrite boulders dredged from the Taramakau River are characterised by a pale-grey weathering and are assumed to represent boulders derived from younger gravels or reworked boulders from older deposits that have been progressively lowered from high terraces during valley deepening (P. Suggate, pers. comm. 2010). The surface of the nephrite boulder in this study shows no indication of water smoothing, and is presumed to have been derived in situ from a glacial outwash gravel terrace exposure or recently eroded from the gravel.
The thickness of the weathering rind implies that the boulder is most likely derived from the oldest and most weathered glacial outwash gravels of the area (Warren Citation1967; Suggate & Watters Citation1991; Nathan et al. Citation2002) considered to have been deposited during the Nemona and Waimuanga Glacial periods between 380–340 ka (oxygen isotope stage 10) and 300–240 ka (oxygen isotope stage 8), respectively (Suggate Citation1985 , Citation1990). Gravels (boulders and cobbles) forming the highest terrace remnants on the south side of the New River () are dominated by low-grade quartzofeldspathic metasandstone (greywacke) derived from the Southern Alps (Suggate & Waight Citation1999).
Iron pans are sometimes developed at the loess/gravel interface, but more usually they branch and meander through the gravels to considerable depths; this suggests that percolating water moves by lateral flow through the weathered gravels rather than being derived directly through the loess cover (G. Mew, pers. comm. 2010). Weathering varies in response to the degree of development (intensity) of these iron pans, and possibly the timing of their initial formation. Beneath prominent iron pans, the gravels, including older high terrace gravels, may not be prominently weathered. At sites where older gravels are overlain by younger gravels, the older gravels clearly have a more weathered appearance (the matrix more so than the boulders; Suggate & Waight Citation1999). The most strongly weathered deposits are generally found near the surface of oldest gravels forming the high terraces (Suggate & Waight Citation1999) (). It is important to note that weathering of the gravels is not uniform, however, and some greywacke clasts coexisting with unweathered deposits exhibit the unusual phenomena of ‘inside-out weathering’ (Suggate & Watters Citation1991). Gravels and their matrix that form the low, youngest glacial outwash terraces of the late Otira Glaciation () are essentially unweathered.
In pre-European times (pre-1860s), the glacial outwash gravel terraces were covered by dense stands of podocarp/hardwood forest that had developed over the last 11 ka (Vandergoes et al. Citation2005). Virtually none of this forest remains today and the terraces are characterised by a cover of ferns and rushes with the formation of swampy areas called ‘pakahi’, where drainage is poor. On the southern side of New River, the old terraces are mantled with c. 0.6–1.0 m of loess with a silt-loam texture (Young Citation1967) and soils are very poorly drained gley podzols (Mew & Palmer Citation1989).
Petrography and mineralogy of the nephrite
Analytical method
Thin section observation shows that the weathered and unweathered nephrite consists of pale-green amphibole that forms a partly felted mixture of variably sized granular (0.1–0.5 mm diameter), acicular (0.4–0.9 mm length) and fibrous (0.02–0.1 mm length) crystals. There is no directional fabric evident and therefore the rock sample can be classed as a non-schistose semi-nephrite (Turner Citation1935). Electron microprobe analyses indicates that the amphibole is actinolite with XMg of 0.87–0.90 (); it therefore has a composition close to the tremolite-actinolite boundary in terms of Si and Mg/(Mg+Fe2+) content as shown in .
Fig. 3 Actinolite compositions () plotted in terms of Si versus Mg/(Mg+Fe2+). Amphibole nomenclature after Leake et al. (Citation1997).
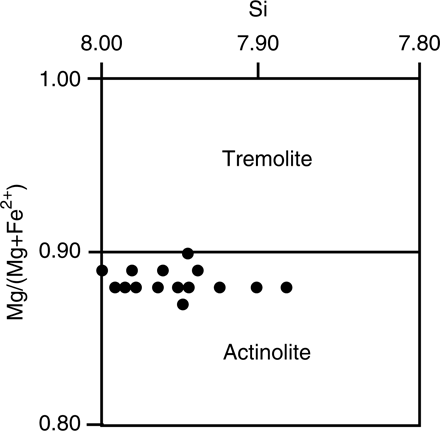
Minor oxides (wt%) present include Al2O3 (0.39–1.23%), Cr2O3 (0.11–0.34%), MnO (0.06–0.24%), NiO (0.09–0.25%), Na2O<0.07% and K2O<0.05%. Recalculation of the actinolite on the basis of 23(O) and 13 cations (for calcic amphiboles with M(4) occupied by Ca and Na) indicates that most of the analyses have no Fe3+. Four analyses of fibrous crystals (analyses 12–15; ) with 0.024 – 0.053 Fe3+ per formula unit are brownish-yellow (plane polarised light) due to iron staining. Two of these analyses (12 and 15) also have the highest Al2O3 of 1.23% and they show the greatest deviation from the amphibole structural formula. It was not possible to obtain a quantitative analysis of the iron films which showed as thin (<1 micron thick) bright areas along actinolite cleavage planes and grain edges on back-scattered electron images. X-ray density dot-mapping indicate that these bright films are Fe-rich and also appeared to have slightly elevated Al. From this, we infer the secondary iron phase to be an Al-substituted iron hydroxide, and most probably goethite (Fitzpatrick & Schwertmann Citation1982).
Geochemistry of the nephrite weathering rind
Analytical methods
Enough material (about 6–8 g) for XRF analyses of major and trace elements, FeO and H2O determination (where H2O+=chemical or combined water; H2O−=absorbed water given off at 110oC), was obtained by using a fine diamond saw to separate ‘blocks’ of each colour layer from 10 slices cut from the nephrite slab. The homogeneity of each colour-layer block was ensured by careful grinding of its two contact surfaces. The samples were oven-dried for 24 hours at 30oC before being powdered and prepared for analysis. Chemical analyses were carried out at the Analytical Facility, Victoria University of Wellington, New Zealand using a Phillips PW 1404 X-ray fluorescence spectrometer on fusion discs (major elements) and pressed powder pellets (trace elements). Ferrous iron was determined using back-titration with potassium dichromate and an Fe(II) and ammonia sulphate solution following dissolution of sample powders in a heated HF/H3PO4 mixture and ammonium vanadate solution (Andrade et al. Citation2001). The amount of absorbed water (H2O−; water released at 110oC over 1 hour heating time) was determined on rock powders that were stored in a dessicator immediately after crushing. The specific gravity (ρ) of small fragments from the different layers was determined using the formula ρ=W a/(W a−W w) where W a is the weight of the sample in air and W w is the weight of the sample immersed in pure water at 25oC.
Chemical variation
Analyses of nine colour bands of the weathering rind and the unweathered nephrite are listed in together with bulk densities. As expected, the rock analyses have actinolite compositions but, in comparison with the Electron probe microanslyser (EPMA) analyses of actinolite grains (), the rock analyses have higher Al2O3 and Fe2O3 which is in agreement with the presence of secondary Fe-hydroxide. Although carefully searched for, chlorite as a possible contributor of Al and Fe3+ was not found. The Cr2O3 content of the unweathered nephrite (0.21 wt %; ) agrees with the mean value of 0.22 wt % in the actinolite (). This confirms the absence of chromite, the presence of which is typically indicated by dark spots in nephrite (Wilkins et al. Citation2003; Tennant et al. Citation2005).
Table 2 XRF analyses* of nephritic greenstone and weathering rind
A notable compositional difference between the nephrite and single actinolite grain analyses is the Na2O content. EPMA analyses of actinolite indicates low Na2O of between 0.03 and 0.07 wt %, whereas sodium in the bulk nephrite is significantly higher and ranges between 0.29 and 0.68 wt %, the highest amount occurring in the unweathered nephrite. Soda values for other west coast nephrite samples are either <0.1 wt % or range between 0.15 and 0.40 wt % (Wilkins et al. Citation2003; Tennant et al. Citation2005). In this case, the difference in Na2O contents of actinolite and bulk nephrite is not considered to be the result of analytical error and so must result from the presence of a discrete Na-bearing solid phase. One possibility is Na-bearing margarite with between 1.3–4.2 wt % Na2O in margarite coexisting with Cr-muscovite in metasomatic corundum-rich rocks derived from the Pounamu ultramafics (Grapes & Palmer Citation1996), although this phase would also increase Al2O3 in the nephrite. Margarite has been confirmed by X-ray diffraction (XRD) in deep blue-green greenstone from the Hokitika River () by Wilkins et al. (Citation2003).
From thin-section observation, Back-scattered electron (BSE) imaging and XRD analysis, the unweathered nephrite of this study is monomineralic actinolite and thus the only possibility of higher Na2O in the bulk nephrite appears to be due to the presence of secondary halite. If sodium is not contributed during laboratory sample preparation, then it should be natural. However, verification of halite of any origin in the nephrite would require Scanning electron microscope (SEM) observation on a freshly broken surface and/or determination of Cl; this was not carried out at the time of analysis. As there was insufficient material available after analysis of the nephrite, we used freshly broken fragments of another relatively unweathered nephrite sample collected from young (Late Last (Otira) Glacial age; ) north Westland terrace gravels (precise locality unspecified) in a leaching experiment to test the possibility of halite in the rock. After oven drying at 105oC, the rock was broken into chips (usually<80 mesh) that were placed in ultrapure water in a Pyrex glass flask within a closed chamber for 1 hr at 30oC. The leachate solution was then filtered with 5B filter paper and immediately analysed for Na (using ICP-AES) and Cl (using Ion Chromatography) at the Department of Earth and Environmental Sciences, Korea University. For quality control, a blank ultrapure water sample was also analysed. The leachate solution contained recognisable concentrations of Na+ (0.56 mg L−1) and Cl− (1.16 mg L−1), corresponding to a molar Na/Cl ratio of 0.74. Although slightly lower than Na/Cl of seawater (0.97), the experimental result suggests that the leached Na and Cl are probably derived from the dissolution of halite within the nephrite. Leaching of Cl together with Na also implies that the possibility of Na-leaching from dissolution of other Na-bearing minerals (e.g. Na-bearing margarite or albite) can be discounted.
Halite has been reported in Alpine Schist by Grapes (Citation1995). BSE observation indicates that it occurs as rare <10 micron diameter grains in microcavities developed within areas of retrograde recrystallisation within the schist, and is inferred to have been precipitated from metamorphic fluids. Under normal circumstances, such halite would be dissolved in water during thin section preparation. This probably accounts for the fact that, apart from the one example observed, halite has not been reported in the Alpine Schist. The unweathered core of the nephrite boulder with the highest Na2O (0.68 wt%; analysis 1 in ) suggests that the halite was precipitated from a metamorphic fluid during greenschist facies conditions of metasomatism/recrystallisation to form the nephrite. However, the additional contribution of sea salt – at least to the weathering rind part of the boulder – from frequent westerly storms that affect the Westland area is also a possibility. Mean cation levels of Na in rainwater in northern Westland are reported by Meder et al. (Citation1991) as 200 µmoles. Rainwater from storm events also contains high amounts of sea-salt-derived cations (Verhoeven et al. Citation1987; Rowe & Fahey Citation1991).
The compositions of nephrite colour bands are plotted against distance outwards from the unweathered core in Aand B. With respect to the unweathered nephrite values, there is an overall decrease in SiO2, MgO, CaO, FeO, Na2O and K2O (not plotted) from core to rim and an increase in Al2O3, Fe2O3, Cr, Zn, H2O+ and H2O−. Nickel, Sc and V show no consistent core to rim variation, as do TiO2 and MnO (B; ). The presence of P2O5 (0.02–0.04 wt %; ) in the outer part of the weathering rind suggests that it may have been introduced from groundwater. Oxidation ratio (expressed as mol.2Fe2O3×100/(2Fe2O3+FeO)) increases from core (5.9) to rim (19.6). Except for an increase in Fe2O3 and H2O of up to 1.4 wt %, the oxide net change is less than 0.5 wt% compared with the unweathered nephrite composition. Bulk density decreases towards the rim from 3.03 g cm−3 in the unweathered core to values of less than 2.83 g cm−3 in the brown oxidised zone of the rind. Conversely, increasing amounts of absorbed water (H2O−) towards the rim is interpreted as the effect of increasing microporosity.
Fig. 4 (A) Plots of unweathered nephrite and weathering rind compositions with respect to colour zone thickness indicated in and . Values are numbered as in (analyses 1–10) and are normalised to 100 wt %. (B) Plots of unweathered nephrite and weathering rind compositions, oxidation ratios and bulk densities with respect to colour zone thickness indicated in and . Values are numbered as in (analyses 1–10).
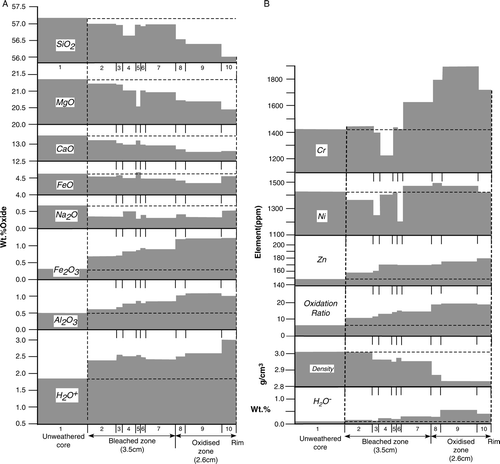
Based on colour and elemental variation, the nephrite weathering rind can be subdivided into an inner pale-green or bleached zone and an outer brownish or oxidation/hydration zone with the highest Fe2O3, Al2O3, Cr2O3, H2O and lowest density (, 4A, B; ). Similar bleached and oxidised zones of weathering rinds developed on andesite are described by Oguchi (Citation2001).
Discussion
Weathering process and products
In general, under a surface weathering environment with circum-neutral pH (5–8) and oxidising conditions, alkalis and alkaline earths (e.g. Na, K, Ca, Mg) with high ionic potentials are sufficiently moderately mobile to be effectively leached from silicate (or carbonate) minerals in rocks to form mobile cations in water (Davis & DeWiest Citation1966; Perel'man Citation1967; Whittacker & Muntus Citation1970). In contrast, Fe, Al, Ti and Cr are typically immobile (insoluble) under such conditions; thus, they should remain and therefore become enriched in weathered rocks.
Oxide/element trends of the nephrite weathering rind shown in A and B suggest leaching of Mg, Ca and Na and concomitant increase in Al, Cr and oxidation of iron with hydration during weathering. These trends reflect dissolution of actinolite, preferentially along grain boundaries, cleavage planes and other dislocations (Zhang et al. Citation1993). This results in increased micoporosity with removal of mobile ions (Ca, Mg and Si) (Schott et al. Citation1981) and the relative increase of less mobile leached elements (Fe3+, Al and Cr) that are precipitated as thin films of Fe-hydroxide within the partly leached areas of the actinolite (which form the ‘walls’ of moisture diffusion paths). As pointed out by Wilkins et al. (Citation2003), the fibrous habit of nephritic actinolite is likely to increase its susceptibility to weathering. Under surface conditions it is metastable with respect to fully crystalline tremolite-actinolite; inwards diffusion of moisture along access channels in the disordered crystal structure can also occur.
Oxidation of Fe2+ to form goethite under aerated conditions according to the reaction
Higher Al2O3 in the oxidised brown zone (A, ) cannot be explained by the presence of gibbsite or kaolinite, and it is suggested that Al substitutes for Fe3+ in goethite. Similarly, the outward increase in Cr (B, 5) suggests substitution of Cr for Fe3+ in goethite (Schwertmann et al. Citation1989) and/or adsorption of Cr (possibly as the aqueous oxyanion HCrO4 −) with goethite (Grossl et al. Citation1997).
Fig. 5 Isocon diagrams for unweathered composition and average compositions of bleached zone (upper diagrams) and oxidized zone (lower diagrams) of weathering rind of nephrite boulder. An isoconcentration line (isocon) is drawn assuming constant Al2O3 during weathering. Oxides are plotted in wt % (analyses in normalised to 100 wt %) and elements in ppm (scaled together with minor oxides in right-side enlargement diagrams). Total iron as FeO is shown as FeO(T). Displacement of the data points from the isocon indicates relative gains (above the isocon) and losses (below the isocon); i.e. the change in concentration of a component relative to its concentration prior to weathering assuming constant Al2O3 (see text).
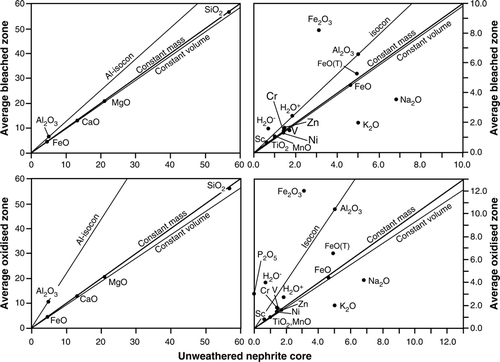
In order to evaluate chemical gains and losses during weathering in relation to possible mass-volume change, the average composition of the 6 bleached zone layers (analyses 2–7; ) and the 3 oxidised zone layers (analyses 8–10; ) of the weathering rind are compared with the unweathered nephrite composition (analysis 1; ) using isoconcentration (isocon) diagrams (Grant Citation1986 , Citation2005) as shown in .
From reaction 2, and assuming no addition of Al to the weathering rind from groundwater, it can be reasonably assumed that Al2O3 behaved as an immobile element during actinolite dissolution and an Al-isocon was constructed (). In the bleached zone, the isocon may also be defined by H2O+ and Sc ().
The slope of the isocon is 1.32 for the bleached zone indicating a mass decrease of −24% and a volume change of −26%; in the oxidised zone the slope increases to 2.08 indicating a mass decrease of −52% and a volume change of −58%. With respect to the alumina isocon, apart from Fe2O3 and H2O− there is loss of all oxides/elements in the weathering rind zones. In the bleached zone, losses (%) are Zn (7), Cr (18), Ti and Mn (10), Ni (14), V (32), Na2O (57) and K2O (70); in the oxidised zone losses are H2O+ (30), V (32), Sc (33), Cr (35), Zn (56), Na2O (74) and K2O (83).
The main oxide components of actinolite, SiO2, MgO, CaO and FeO plot on the constant mass lines, and their loss (reaction 2) was coupled with the percent decrease in mass. It can be noted that element losses such as Cr, Zn in the bleached zone and Cr, H2O+ in the oxidised zone, assuming constant Al2O3, are less than that of the main oxide components of c. 24 and 52%, respectively; this accounts for their apparent outwards increase in the weathering rind shown in A and B. The increase in Fe2O3 (51% in bleached zone; 47% in oxidised zone) is probably mainly due to oxidation of FeO; because total iron (as FeO) shows a reduction with respect to the alumina isocon (), introduction of additional Fe3+ from iron-bearing groundwater that formed iron-pans in the nephrite-bearing terrace gravels may have occurred. The gain in H2O− (42% in bleached zone; 64% in oxidised zone) reflects increasing microporosity as a result of actinolite dissolution with a decrease of average density ratios of the unweathered and weathered nephrite from 0.980 (bleached zone) to 0.937 (oxidised zone) ().
The dissolution-precipitation process of nephrite weathering described above can be mainly related to two independent variables: groundwater/rainwater (moisture) acidity and dissolved oxygen content. For nephrite weathering-rind development, low acidity water with sufficiently high oxygen content favours the development of brown hydroxylated ferric oxide, whereas higher acidity promotes the dissolution of iron to leave a pale-green-white weathered zone (Wilkins et al. Citation2003).
The formation of the nephrite weathering rind can also be related to periods of wetting and drying and to short-term seasonal and long-term climatic variations that have gradually advanced the alteration front into the boulder since its deposition (see below). During periods of drying, dissolved ions will be transported by competing processes of advection within the moisture that transports ions to the boulder surface and by diffusion within the moisture which tends to smooth out any accumulations of ions (Pel at al. Citation2004). Whichever process dominates will depend on the rate of drying that would be seasonally/climatically variable. The bleached zones (light green and white) of the nephrite weathering rind represent the extent of dissolution of high solubility alkaline earth metals, silica and intergranular halite as water infiltrates into a certain depth of the boulder, resulting in an increase in pore water alkalinity. However, outwards movement of the leached elements should occur with diffusive outwards flow of pore water accompanying evaporitic moisture loss during drying. The fine-scale colour zoning within the bleached zone of the weathering rind therefore reflects the effects of long-term (over an inferred period of perhaps 360–250 ka; ) repeated wetting/drying, variable depths of water penetration and consequent variable element outwards migration distances due to advection and diffusion.
In , H2O− values of the weathering rind layers () are used as a measure of relative rock porosity. The increasing H2O−, Fe2O3, Al2O3, H2O+, Cr and oxidation ratio and SiO2, FeO, CaO, MgO and density decrease is shown in A and B. As described above, these trends are explained by leaching of Si, Ca and Mg, which causes higher porosity, allowing entry of H2O and precipitation of secondary Al and Cr-substituted Fe-hydroxide according to reaction (2). Decreasing FeO is caused by oxidation to Fe2O3. The non-linear increase/decrease of oxide, Cr and density trends with increasing H2O− suggests that increasing porosity created by actinolite dissolution may be related to an exponential increase in the degree of weathering (dissolution of actinolite and secondary Fe-hydroxide precipitation). A non-linear relationship between porosity and the diffusion coefficient has also been found in weathering rinds developed on andesite pebbles (Oguchi Citation2004). In the case of weathering rinds on andesite pebbles, however, the extent (i.e. weathering-rind thickness) and rate of weathering are related to the original rock porosity rather than to increased porosity due to mineral dissolution as suggested here for the nephrite boulder.
Fig. 6 Plots of wt % SiO2, FeO, MgO, CaO, Fe2O3, Al2O3, ppm Cr, bulk oxidation ratio and density versus H2O– for unweathered (open square) and weathered (filled circles) nephrite. Analyses in normalised to 100 wt %.
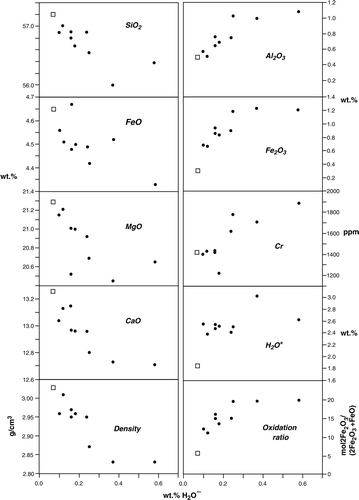
A plot of H2O+ versus density shown in correlates with the respective trends in , but also highlights a marked change in density at H2O+ values between c. 2.38 and 2.55 wt %. A change in rock density is likely related to incipient dissolution and cation loss as well as lower amounts of Fe-hydroxide formation. Although evident by a colour change from dark- to pale-green (), the volume of rock that marks the abrupt change in density (0.14 g cm−3) with little change in H2O+ (0.17 wt %) has a thickness of about 3.5 cm (). This represents the zone of maximum (although not necessarily the youngest) inwards extent of the less oxidised, bleached part of the weathering rind.
Relationship of nephrite weathering and climate
The West Coast area of the South Island of New Zealand currently has a superhumid mesothermal climate (Hessel Citation1982) with an annual rainfall of 2500–4000 mm a−1 that has promoted dense podocarp/hardwood forest growth. Most precipitation comes from the west. Rainwater from air masses moving eastwards across the Southern Ocean and Tasman Sea has a pH of 5.7–6.2, implying its general chemical equilibrium with atmospheric CO2. Bacterial degradation of forest-floor litter to humic acids results in lower groundwater pH values of 3.6–4.7 (Hawke & Powell Citation1995) than for shallow groundwaters of forest-free alluvial flats. In the latter case, the pH may have a similar range to rainwater of between 5.7 and 6.6 and has a dissolved oxygen content of 45–64% with respect to saturation conditions (Baker Citation2004).
An inferred derivation of the weathered nephrite boulder from old (c. 360–248 ka) glacial outwash gravels in north Westland indicates that it has undergone a period of weathering that spans climatic and environmental changes associated with several glacial and interglacial periods (). Dense forest cover developed under warm-moist conditions since the beginning of the Holocene interglacial c. 11 ka, as presumably also during previous interglacial periods (Moar & Suggate Citation1996; Vandergoes et al. Citation2005). In contrast, during the Last (Otira) Glaciation (84–12 ka) and during previous glacial periods, cold-dry tundra-like conditions established montane-subalpine vegetation on outwash gravels and till.
For a given environmental setting and clast morphology, weathering is expected to be less intense under dry (glacial) conditions than humid (interglacial) conditions. Water-soluble organic acids in groundwater, especially high-molecular-weight organics such as fulvic and humic acids, are known to increase the weathering rates of amphiboles. In turn, this is related to the quantity of O in the organic ligand groups (e.g. R-OH and R-OOH) involved that controls the strength of metal-ligand complexing at a given pH and temperature (e.g. Zhang & Bloom Citation1999). Thus, groundwater in contact with the nephrite boulder is likely to contain higher amounts of dissolved organic acid and therefore have a lower oxygen content and pH when forest cover is dominant, i.e. during interglacial conditions. This would promote dissolution of iron as Fe2+ resulting in the pale-green/cream/white colouration such as in the inner part of the weathering rind. Groundwater levels in the West Coast terrace gravels are also likely to be at a higher level during moist interglacial periods (as they are now) than during drier glacial periods.
On the other hand, with a sufficiently high dissolved oxygen content i.e. >1–2 mg L−1 (possibly due to lower dissolved organic acids in the groundwater because of reduced or no forest cover during periods of glacial advance, lower rainfall and therefore lower recharge of surface oxygenated water), conditions of low acidity would promote the formation of iron hydroxide resulting in the development of the brown outer part of the nephrite weathering rind. Chromium has a high solubility in oxygenated surface waters (Fendorf Citation1995), and its marked concentration in the outer brown oxidised part of the nephrite weathering rind (B), substituted in or absorbed on goethite, is consistent with this.
Nephrite colour
The intensity of green colouration of nephrite is mainly a function of total iron content (Finlayson Citation1909; Wilkins et al. Citation2003). However, the oxidation ratio (mol.2Fe2O3×100/[2Fe2O3+FeO]) is also important and this increases with weathering, irrespective of the colour that is developed (Wilkins et al. Citation2003). For the nephrite of this study, the change from dark-green to pale-green-white colouration occurs within the interval between total iron contents of 4.9 to 5.2 wt % and a corresponding change in oxidation ratios from 5.9 to 11.3 (). The change from pale-green-white to brown colouration occurs at a total iron content of 5.5–5.6 wt % and between oxidation ratios of 16.2 and 19.6 ().
The nephrite analyses of this study are compared with other unweathered and weathered nephrite samples with similar XMg values from the West Coast area in a plot of total iron versus oxidation ratio (). The combined data show that the intensity of green colouration of unweathered nephritic greenstone increases with increasing total iron (up to 5 wt %) and increasing oxidation ratio (up to 7). With high total iron contents of >6 wt % (oxidation ratio <3.5), unweathered nephrite is black due to the presence of ultrafine magnetite (Tennant et al. Citation2005). A change from green/dark-green to unweathered pale-green and weathered pale-green-white nephrite occurs where the oxidation ratio is >7 over a range of total iron between 3.4 and 5.0 wt %. Between oxidation ratios of 7 and 17 (total iron between 3.6 and 6.4 wt %), weathered nephrite is pale-green to white. When oxidation ratios are >17 (total iron between 4.6 and 6.4 wt %), nephrite is brown due to the presence of secondary iron-hydroxide ().
Fig. 8 Plot of variously coloured north Westland nephrite compositions from this study (open circles), Wilkins et al. (Citation2003) (filled circles) and unpublished analyses by R. Grapes (open squares) in terms of oxidation ratio (mol.2Fe2O3×100/[2Fe2O3+FeO]) versus total iron (wt % Fe2O3+FeO) content. Tie lines connect compositions of weathering rinds developed on unweathered nephrite.
![Fig. 8 Plot of variously coloured north Westland nephrite compositions from this study (open circles), Wilkins et al. (Citation2003) (filled circles) and unpublished analyses by R. Grapes (open squares) in terms of oxidation ratio (mol.2Fe2O3×100/[2Fe2O3+FeO]) versus total iron (wt % Fe2O3+FeO) content. Tie lines connect compositions of weathering rinds developed on unweathered nephrite.](/cms/asset/569b7ae6-7875-448b-aa5b-158560c229b6/tnzg_a_514929_o_f0008g.gif)
Conclusions
The conclusions of this study are as follows.
-
A 6.1 cm thick weathering rind on a boulder of dark-green monomineralic actinolite semi-nephrite from north Westland, South Island of New Zealand, is characterised by an outer brown, oxidised/hydration zone (2.6 cm) and an inner, finely banded pale-green to white bleached zone (3.5 cm). These changes are accompanied by decreasing SiO2, FeO, MgO, CaO, Na2O and density and increasing Al2O3, Fe2O3, H2O+, H2O−, bulk oxidation ratio, Cr and Zn outwards from the unweathered nephrite core through the weathering rind.
-
Weathering involves interrelated processes of dissolution, leaching, precipitation of Fe-hydroxide, oxidation and hydration. Assuming that alumina was immobile during weathering, the compositional variation described above involved 7–83% loss (mobile) of all elements with the exception of Fe3+ and H2O−. The latter show increases of 47–51% and 42–64%, respectively, a mass decrease of −24% and volume change of −26% in the bleached zone and a mass decrease of −52% and volume change of −58% in the oxidised zone of the nephrite weathering rind.
-
Fine-scale colour zoning of the weathering rind reflects the effects of seasonal/longer term climatic wetting/drying events possibly over a period of 360–250 ka, variable water penetration depths into the nephrite boulder and consequent variable element outward migration distances due to advection and diffusion in the pore fluid. Non-linear oxide, Cr and density changes with increasing H2O− (used as a measure of porosity) across the weathering rind suggest that increasing porosity created by actinolite dissolution results in an exponential increase in the amount of weathering. A marked change in density at H2O+ values between c. 2.38 and 2.55 wt % is likely related to incipient dissolution and cation loss as well as lower amounts of Fe-hydroxide formation in the bleached zone of the weathering rind.
-
Colour changes of nephrite are mainly determined by total iron content and oxidation ratio (mol.2Fe2O3×100/[2Fe2O3+FeO]). The change from green/dark-green to pale-green-white colour occurs when oxidation ratios are >7. The change from pale-green-white to brown occurs when oxidation ratios are >17.
Acknowledgements
Expert information from Drs Pat Suggate (GNS Science, Lower Hutt) and Geoff Mew (Landcare Research, Lower Hutt) on weathering, soils and vegetation in North Westland is greatly appreciated. We also thank two anonymous reviewers for their comments and suggestions that resulted in clarification of text and diagrams and Nick Mortimer for suggested changes, advice and editorial handling.
References
- Adams , CJ , Beck , RJ and Campbell , HJ . 2007 . Characterisation and origin of New Zealand nephrite jade and its strontium isotope signature . Lithos , 97 : 307 – 322 .
- Andrade , S , Hypolito , R , Ulbrich , HHGJ and Sliva , ML . 2001 . Iron (II) oxide determination in rocks and minerals . Chemical Geology , 182 : 85 – 89 .
- Baker T 2004 . Groundwater quality and farm nitrogen management on the West Coast, South Island, New Zealand . Unpublished MSc. Thesis in Physical Geography , Victoria University of Wellington , New Zealand
- Beck RJ 1984 . New Zealand Jade, the Story of Greenstone , 2nd ed AH and AW Reed , Wellington, , New Zealand .
- Beck RJ 1991 . Jade in the South Pacific . Kervern , R (Consulting Editor), Jade . New York : Van Nostrand Reinhold , 229 257 .
- Bell JM , Fraser C 1906 . The Geology of the Hokitika Sheet. North Westland Quadrangle . New Zealand Geological Survey Bulletin 1 .
- Chapman , FR . 1892 . On the working of greenstone or nephrite by the Maoris . Transactions of the New Zealand Institute , 14 : 479 – 539 .
- Coleman , RG . 1966 . New Zealand serpentinites and associated metasomatic rocks . New Zealand Geological Survey Bulletin , 70 : 102p
- Coleman , SM . 1982 . Chemical weathering of basalts and andesites: evidence from weathering rinds. U.S . Geological Survey Professional Paper , 1246 : 1 – 51 .
- Coleman , SM and Pierce , KL . 1981 . Weathering rinds in andesitic and basaltic stones as a Quaternary age indicator, western United States. U.S . Geological Survey Professional Paper , 1210 : 1 – 56 .
- Cooper , AF . 1976 . Concentrically zoned ultramafic pods from the Haast Schist Zone, South Island, New Zealand . New Zealand Journal of Geology and Geophysics , 19 : 603 – 623 .
- Cooper , AF . 1995 . Nephrite and metagabbro in the Haast Schist at Muddy Creek, northwest Otago, New Zealand . New Zealand Journal of Geology and Geophysics , 38 : 325 – 332 .
- Cooper , AF and Reay , A . 1983 . Lithology, field relations, and structure of the Pounamu ultramafics from the Whitcombe and Hokitika rivers, Westland, New Zealand . New Zealand Journal of Geology and Geophysics , 2 : 359 – 379 .
- Davis SN , DeWiest RJM 1966 . Hydrology . John Wiley , New York , 463p .
- Dixon , JC , Thorn , CE , Darmody , RG and Campbell , SW . 2002 . Weathering rinds and rock coatings from an arctic alpine environment, northern Scandinavia . Geological Society of America Bulletin , 114 : 226 – 238 .
- Etienne , S . 2002 . The role of biological weathering in periglacial areas: a study of weathering rinds in south Iceland . Geomorphology , 47 : 75 – 86 .
- Etienne , S and Dupont , J . 2002 . Fungal weathering of basaltic rock in a cold oceanic environment (Iceland): comparison between experimental and field observations . Earth Surface Processes and Landforms , 27 : 737 – 748 .
- Fendorf , SE . 1995 . Surface reactions of chromium in soils and waters . Geoderma , 67 : 55 – 71 .
- Finlayson , AM . 1909 . The nephrite and magnesian rocks of the South Island of New Zealand . Quarterly Journal of the Geological Society , 65 : 351 – 381 .
- Fitzpatrick , R and Schwertmann , U . 1982 . Al-substituted goethite – an indicator of pedogenic and other weathering environments in South Africa . Geoderma , 27 : 335 – 347 .
- Grant , JA . 1986 . The isocon diagram – a simple solution to Gresen's equation for metasomatic alteration . Economic Geology , 81 : 1976 – 1982 .
- Grant , JA . 2005 . Isocon analysis: A brief review of the method and applications . Physics and Chemistry of the Earth , 30 : 997 – 1004 .
- Grapes , RH . 1995 . Uplift and exhumation of Alpine Schist, Southern Alps, New Zealand: thermobarometric constraints . New Zealand Journal of Geology and Geophysics , 38 : 525 – 533 .
- Grapes , R and Palmer , K . 1996 . (Ruby-sapphire)-chromian mica-tourmaline rocks from Westland, New Zealand . Journal of Petrology , 37 : 293 – 315 .
- Grossl , PR , Eick , M , Sparks , DL , Goldberg , S and Ainsworth , CC . 1997 . Arsenate and chromate retention mechanisms of goethite. 2. Kinetic evaluation using a pressure-jump relaxation technique . Environmental Science Technology , 31 : 321 – 326 .
- Hawke , DJ and Powell , HKJ . 1995 . Soil solution chemistry at a Westland petrel breeding colony, New Zealand: Paleoecological implications . Australian Journal of Soil Research , 33 : 915 – 924 .
- Hessel JWD 1982 . The climate and weather of Westland . New Zealand Meteorological Service Miscellaneous Publication 115 , Ministry of Transport , Wellington .
- Ireland , TR , Reay , A and Cooper , AF . 1984 . The Pounamu Ultramafic Belt in the Diedrich Range, Westland, New Zealand . New Zealand Journal of Geology and Geophysics , 27 : 247 – 256 .
- Johnston MR 1983 . Nephrite in the Pounamu Formation, McArthur Crags, Olderog Creek, Arahura Valley, Westland . Industrial Rocks and Minerals, v.8 , New Zealand Geological Survey , Department of Industrial and Scientific Research , Lower Hutt 5 8 .
- Koons , PO . 1981 . A study of natural and experimental metasomatic assemblages in an ultramafic-quartzo-feldspathic metasomatic system from the Haast Schists, South Island, New Zealand . Contributions to Mineralogy and Petrology , 78 : 189 – 195 .
- Leake BE , and 21 other authors 1997 . Nomenclature of amphiboles: Report of the subcommittee on amphiboles of the International Mineralogical Association, Commission on New Minerals and Mineral Names . The Canadian Mineralogist 35 : 219 246 .
- Matsukura Y , Kimata M , Yokoyama S 1994 . Formation of weathering rinds on andesite blocks under the influence of volcanic gases around the active crater of Aso Volcano, Japan . Rock Weathering and Landform Evolution Robinson DA , Williams RBG John Wiley & Sons : Chichester : 89 98 .
- Meder , R , Hunter , KA and Peake , BM . 1991 . Trans-Tasman tropospheric transport of acid rain . Journal of the Royal Society of New Zealand , 21 : 137 – 142 .
- Mew , G and Palmer , RWP . 1989 . Comparison of soil groups in North and South Westland, and implications for land use . Journal of the Royal Society of New Zealand , 19 : 405 – 418 .
- Moar , NT and Suggate , RP . 1996 . Vegetation history from the Kaihinu (Last) Interglacial to the present, West Coast, South Island, New Zealand . Quaternary Science Reviews , 15 : 521 – 547 .
- Nathan S , Rattenbury MS , Suggate RP (compilers) 2002 . Geology of the Greymouth area. Institute of Geological and Nuclear Sciences 1: 250 000 geological map 12. 1 sheet+58 p . Lower Hutt, , New Zealand . Institute of Geological and Nuclear Sciences Limited .
- Oguchi , CT . 2001 . Formation of weathering rinds in andesite . Earth Surface Processes and Landforms , 26 : 847 – 858 .
- Oguchi , CT . 2004 . A porosity-related diffusion model of weathering-rind development . Catena , 58 : 65 – 75 .
- Oguchi , CT and Matsukura , Y . 1999 . Effect of porosity on the increase in weathering-rind thickness of andesite gravel . Engineering Geology , 55 : 77 – 89 .
- Pel , L , Huinink , H , Kopinga , K , van Hees , RPJ and Adan , OCG . 2004 . Efforescence pathway diagram: understanding salt weathering . Construction and Building Materials , 18 : 309 – 313 .
- Perel'man AI 1967 . Geochemistry of Epigenesis . Plenum Press , New York , 266 p.
- Rowe , LK and Fahey , BD . 1991 . Hydrology and water chemistry change after harvesting small, indigenous forest catchments, Westland, New Zealand. In: Sediment and Stream Water Quality in a Changing Environment: Trends and Explanation. Proceedings of the Vienna Symposium, August 1991 . IAHS Pub. , 203 : 259 – 266 .
- Sada , E , Kumazawa , H , Makino , K and Cho , H-M . 1990 . Size control in the formation of extremely fine goethite particles by oxidation of alkaline suspensions of ferrous hydroxide . Chemical Engineering Communications , 91 : 225 – 234 .
- Sak , PB , Fisher , DM , Gardner , TW , Murphy , K and Grantley , SL . 2004 . Rates of weathering rind formation on Costa Rican basalt . Geochimica et Cosmochimica Acta , 68 : 1453 – 1472 .
- Schott , J , Berner , RA and Sjöberg , EL . 1981 . Mechanism of pyroxene and amphibole weathering. I. Experimental studies of iron-free minerals . Geochemica et Cosmochimica Acta , 45 : 2123 – 2135 .
- Schwertmann , U , Gasser , U and Sticher , H . 1989 . Chromium-for-iron substitution in synthetic goethite . Geochemica et Cosmochimica Acta , 53 : 1293 – 1297 .
- Suggate RP 1985 . The glacial/interglacial sequence of north Westland, New Zealand . New Zealand Geological Survey record 7 .
- Suggate , RP . 1990 . Late Pliocene and Quaternary glaciations in New Zealand . Quaternary Science Reviews , 9 : 175 – 197 .
- Suggate , RP and Watters , WA . 1991 . “Inside-out weathering” of boulders in glacial outwash gravel . New Zealand Journal of Geology and Geophysics , 34 : 93 – 97 .
- Suggate , RP and Waight , TE . 1999 . Geology of the Kumara-Moana area . Institute of Geological and Nuclear Sciences, Geological Map 24; Lower Hutt, New Zealand, Institute of Geological and Nuclear Sciences, scale , 1 50,000
- Tennant , WC , Claridge , RFC , McCammon , CA , Smirnov , AI and Beck , RJ . 2005 . Structural studies of New Zealand pounamu using Mössbauer spectrospcopy and paramagnetic resonance . Journal of the Royal Society of New Zealand , 35 : 385 – 398 .
- Tsein , H-H and Fang , J-N . 2002 . Mineralogy and alteration of Chinese archaic jade artifacts . Western Pacific Earth Sciences , 2 : 239 – 250 .
- Turner , FJ . 1935 . Geological investigations of the nephrites, serpentinites and related “greenstones” used by the Maori of Otago and South Canterbury . Transactions of the Royal Society of New Zealand , 65 : 187 – 210 .
- Vandergoes , MJ , Newnham , RM , Preusser , F , Hendy , CH , Lowell , TV , Fitzsimons , SJ , Hogg , AG , Kasper , HU and Schlüchter , C . 2005 . Regional insolation forcing of late Quaternary climate change in the Southern Hemisphere . Nature , 436 : 242 – 245 .
- Velbel , MA and Ranck , JM . 2008 . Etch pits on naturally altered olivine from dunites of the Appalachian Blue Ridge Mountains, North Carolina, USA . Mineralogical Magazine , 72 : 145 – 148 .
- Verhoeven , R , Herrmann , R , Eden , R and Klemm , O . 1987 . A comparison of the chemical composition of fog and rainwater collected in the Fichtelgebirge, Federal Republic of Germany, and from the South Island of New Zealand . Theoretical Applied Climatology , 38 : 210 – 221 .
- Warren G 1967 . Sheet 17, Hokitika (1st Ed.) Geological Map of New Zealand 1:250,000 . Department of Scientific and Industrial Research , Wellington, , New Zealand .
- Whittacker , EJW and Muntus , R . 1970 . Ionic radii for use in geochemistry . Geochimica et Cosmochimica Acta , 34 : 945 – 956 .
- Wilkins , CJ , Tennant , WC , Williamson , BE and McCammon , CA . 2003 . Spectroscopic and related evidence on the coloring and constitution of New Zealand jade . American Mineralogist , 88 : 1336 – 1344 .
- Young , DJ . 1967 . Loess deposits of the West Coast of the South Island, New Zealand . New Zealand Journal of Geology and Geophysics , 10 : 647 – 658 .
- Zhang , H and Bloom , PR . 1999 . Dissolution kinetics of hornblende in organic acid solutions . Soil Science Society of America Journal , 63 : 815 – 822 .
- Zhang , H , Bloom , PR and Nater , EA . 1993 . Change in surface area and dissolution rates during hornblende dissolution at pH 4.0 . Geochimica et Cosmochimica Acta , 57 : 1681 – 1689 .