Abstract
Crustal seismic reflection data recorded across the Alpine Fault and coastal plain at Whataroa in 1998 are used to derive shallow (4 seconds two-way time or s twt) and deep (14 s twt) seismic reflection images and a simple refraction model. A single 25 km long, 636 channel receiver array recorded energy from 50 kg shots fired at 1 km intervals with intervening shots of 2.5 kg at about 300 m intervals along the profile. Record quality varied from good to poor. Across the Alpine Fault, basement lies at about 300–500 m (0.2–0.5 s twt), and a weak basement reflection may be attributed the Alpine Fault. Basement deepens sharply at about 6 km to the northwest of the fault, and a 3 km section of probably Miocene and younger sediments is imaged to the northwest. The southern boundary of the coastal basin probably corresponds to the South Westland Fault. The deeper section images a distinct band of strong reflectivity at a depth of about 9–10 s twt, the base of which is inferred to be Moho. Depth conversion gives a horizontal Moho across the whole profile at a depth of about 27 km.
Introduction
The Southern Alps of South Island, New Zealand are the result of continental collision along the Pacific–Australian plate boundary. The Alpine Fault marks the western margin of the continental collision zone with lithospheric deformation occurring largely in the Pacific plate to the southeast (Okaya et al. Citation2007). The fault is considered to be the active transcurrent fault boundary linking the opposite dipping subduction zones: the Hikurangi margin to the north and the Fiordland margin to the south. This Southern Alps orogen formed the focus for the South Island Geophysical Trsndect (SIGHT) project in 1996 and 1998, a major international collaborative project aimed at understanding the deformation of the lithosphere at a continental–continental collisional plate boundary () (Davey et al. Citation1998; Okaya et al. Citation2007). As part of this project, active-source multifold crustal seismic reflection data were acquired across the Southern Alps orogen. The data recorded east of the Main Divide have been discussed by Long et al. (Citation2003) and Stern et al. (Citation2007). West of the divide, the profile (Whataroa profile) starts about 5 km southeast of the Alpine Fault and crosses the fault and the adjacent coastal plain to the northwest (), along the line of SIGHT Transect 1 (Van Avendonk et al. 2004). This north-western profile has proved difficult to process due to the very variable response of the subsurface to individual shots. After initial brute stacks were produced in 1999, no further work was done on the data until recently.
Fig. 1 Location of the Whataroa survey shown by the black outline box (also extent of ). SIGHT seismic lines are shown by medium lines labelled with line number; Transect 1 extends from line 1w to line 1e, Transect 2 from line 2w to line 2e. Alpine and Marlborough faults shown by bold lines. Generalised geology. Modified with permission of the American Geophysical Union from in Davey (Citation2005).
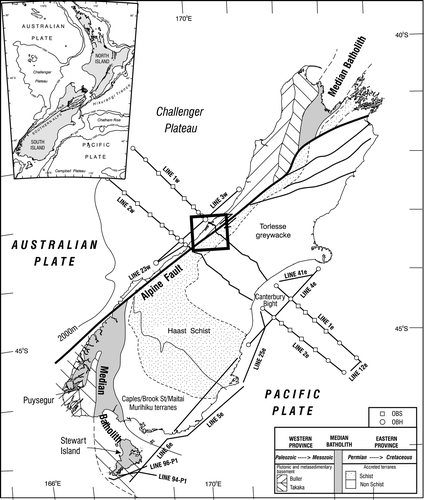
Fig. 2 Simplified topography of the Whataroa region (topographic contours at 100 m, 200 m and thereafter at 200 m intervals). The seismic profile is delineated by the shot positions shown by red circles (sp 221: offset shot; sp 204: start of main part of the line; sp 216: start of dogleg; sp 264: end of line), geophone locations shown by a fine green line , and Common Depth Point (cdp) locations shown in dark blue annotated at 100 cdp intervals. Alpine Fault trace (simplified, light blue) and South Westland Fault trace (dash-dotted line) after Cox & Barrell (Citation2007). Interpreted basement faults are shown by short dashed lines. Drillholes Waiho-1 and Harihari-1 are marked by solid green circles and labelled. Inset: detailed location of seismic line past the bluff. Topographic contours at 100 m intervals are shown by long dash lines; shot points (sp 203–211) by red circles and cdp locations (annotated every 50 cdp) by a black line. Alpine Fault (simplified) is shown by a light blue line, with detailed alternate interpretations (e.g. Cox & Barrell Citation2007) by dashed black lines with question marks.
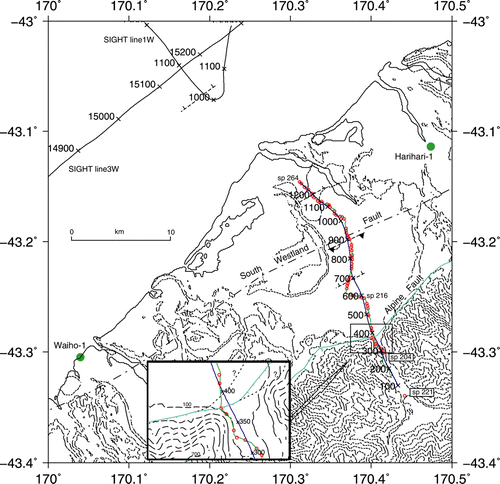
The South Island () comprises two main continental fragments: the Western Province (largely a fragment of Gondwana continental crust) (Cooper Citation1989) and the Eastern Province (a sequence of island-arc and subduction-related metasedimentary terranes accreted onto the Western Province crust of Gondwana margin (e.g. Mortimer Citation2004). The Alpine Fault has offset these geological terranes by about 460 km from the northeast to the southwest (Carter & Norris Citation1976; Sutherland Citation1999) and has juxtaposed the Eastern and Western Provinces in the Whataroa region. The Whataroa seismic profile extends across the Alpine Fault from the Eastern Province (Pacific Plate) to Western Province (Australian Plate) rocks. Plate boundary convergence along the Alpine Fault has ramped the rocks of the Eastern Province up over the Western Province such that metamorphic rocks of increasingly high grade lie against the mylonites and cataclasites forming the Alpine Fault zone. The Alpine Fault dips at the surface at about 45° and 40–60° at depths of about 15–30 km (e.g. Cooper & Norris Citation1994; Stern et al. Citation2007). Northwest, the seismic profile is underlain by basement inferred to be dominantly formed of Ordovician Greenland Group quartz-rich metasediments of the Buller terrane and gneissic intrusions of Palaeozoic and Cretaceous granitoids. These basement rocks are, in general, overlain by up to 4 km of Tertiary–Quaternary sediments (Nathan et al. Citation1986).
During the Last Glacial Maximum (LGM), ice extended from the Whataroa valley onto the coastal plain. The Whataroa glacier occupied a deep trough which, upon withdrawal of the ice, was filled by late-glacial and post-glacial alluvium (Cox & Barrell Citation2007). The base of late Quaternary sediments represents an ice-erosion surface dating from about 18 ka, when the retreat of the LGM ice began (Cox & Barrell Citation2007). The surface trace and movement of the Alpine Fault near the surface is partitioned into alternating segments of strike-slip movement and thrust movement, with segmentation extending to about 3–4 km depth (Norris & Cooper Citation1995). Measured dips at or near the thrust segments vary from 30° to 50° (Norris & Cooper Citation1995). The seismic profile crosses the Alpine Fault at the inferred change from one segment type to the other, although interpretations are hampered as observed geological data are limited (Cox & Barrell Citation2007; Norris et al. Citation2010).
Seismic reflection investigations on land in the region, largely by the oil industry, are sparse. New Zealand Petroleum Company Ltd. (Citation1971a), PR 523) recorded seismic profiles across the coastal plain northwest from Whataroa (lines 4, 4a) that show discontinuous reflections to depths of about 0.5 seconds two-way travel time (s twt) in the southeast that deepen steadily to about 2.5 s twt at the northwest end of their profile. A more extensive body of seismic data exists offshore where a simple three-layer sedimentary sequence is imaged (e.g. Sircombe & Kamp Citation1998). Transect 1 of the SIGHT project (Van Avendonk et al. 2004) across the South Island and offshore partly coincides with the Whataroa seismic profile, where it recorded a 2.5 km thick sedimentary basin under the north-western part of the coastal plain, underlain by basement with seismic velocity of 5 km s−1 and greater. Crustal seismic velocities reached 5.8 km s−1 by 10 km depth, increasing to 6.3 km s−1 by about 20 km depth. A thick lower crust with seismic velocities of about 7 km s−1 extended to Moho at about 29 km under the range front and 26 km at the coast. Brikke (Citation2007) modelled the first-break refraction data for Transect 1 across the Alpine Fault and coastal plain and interpreted a 2 km thick 3.4 km s−1 layer within the coastal plain basin.
This paper presents reflection and refraction models derived from the SIGHT Whataroa seismic reflection data and discusses the constraints that these data place on the dip and character of the Alpine Fault; the structure and relationship of the coastal plain sedimentary basin to the Alpine Fault and the South Westland Fault and other data in the region; and the depth of Moho and the constraints this provides on the deformation of the Australian plate by the overthrust Pacific Plate from the southeast. Furthermore, in view of the current interest in drilling the Alpine Fault at depth under the auspices of the International Continental Drilling Program (ICDP) (Townend et al. Citation2009), these data provide some constraints on both the location of the fault at Whataroa and the acquisition parameters necessary to adequately image the fault subsurface seismically.
Seismic data
The Whataroa seismic reflection profile () coincided with SIGHT Transect 1 as the access across the Alpine Fault (along the upper Whataroa River) was easier for deploying geophones and for shot hole drilling trucks. Access limitations resulted in a major dogleg in the line of the profile at Whataroa () that coincided with a shallow, high seismic velocity, basement block. The dogleg and the wiggly nature and variable spacing of the shot points resulted in a variable coverage for the shallow seismic section. Seismic data were acquired using a 636 channel, telemetered digital recording system with a length of over 25 km. Shots were dynamite in drilled shotholes; nominal 24 m deep for 50 kg shots and 6 m deep for 2.5 kg shots. The 50 kg shots were at 1 km intervals with two smaller shot in between, giving a nominal shot spacing of 250−350 m. A long offset shot (50 kg in about 5 m water depth) was set off in the river about 2 km to the south of the south-eastern end of the recording array, near the Perth–Whataroa river confluence (sp 221, ). This shot was fired on global positioning system (GPS) time signals that were also recorded at the recording truck. Shot and recorder positions were recorded using GPS at the conclusion of the experiment.
Processing of reflection data
The shot gathers are characterised by a very variable shot coupling giving rise to a wide range in signal quality, some records having little or no primary energy after the near-surface reflections and first arrivals. lists the processing sequence used. Shot records were edited for noisy traces, amplitude corrected for spherical divergence, refraction statics and floating statics computed and survey data inserted. A wiggly-line geometry was defined with 20 m common depth point (cdp) bins (geophone separation was a nominal 40 m) that were 2400 m wide to accommodate the positional wiggles along the seismic profile.
Table 1 Seismic processing sequence
The major dogleg midway along the profile results in a gap in the shallow processed section. Ground roll and air wave energy contaminate the data. A narrow-band airwave filter was used. FK velocity filtering and low-cut (10 Hz LC) filtering were tested to reduce ground roll, and a simple low-cut filter was chosen. The processing then followed two routes: one for the shallow section (0–4 s twt) where all shot gathers were used but were restricted to offset of±3000 m, and one for the total crustal section (0–14 s twt) where only the large shot data were used.
Shallow section
The shallow seismic section was initially processed in two parts: the south-eastern end of the profile across the Alpine Fault as reflections from the Alpine Fault would only be imaged by shots located over the fault, i.e. the first 8–10 shots; and the whole profile. The data were subsampled to give shot gathers 12 s twt long and with traces out to±3000 m offsets. The variable signal characteristics from shot to shot () with the strong ground roll on some shots (B) and high-frequency (40–50 Hz) ringing in the upper 1 s of data (e.g. Fig. 3A) degraded the processed section. Pre-stack deconvolution testing gave no discernable improvement of the shot gathers. After cdp sort, several runs of integrated common velocity stack and common velocity analysis were carried out to derive stacking velocities. Post-stack deconvolution was tested but only minor improvements were achieved. The stacked data is single or very low fold across the Alpine Fault sector. The steep dip expected for an Alpine Fault reflection indicates that dip move out or prestack migration of the data is required to correctly position the reflection events for stacking; the fold is too small however, particularly at the southeast end of the profile, for these processes to be used.
Fig. 3 Examples of raw shot gathers showing: (A) shallow ringing; (B) strong ground roll; (C) good data and high-frequency (40 Hz) event at 2 s twt (also on A).
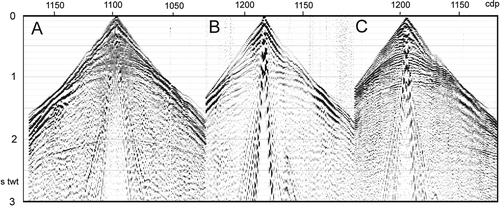
Preliminary stacks were carried out using a variety of stacking velocities (based on refraction velocities and on CVA analysis) but only one weak event was imaged below a poor basement reflection (0.3 s twt) across most of this section (), dipping at about 25° to the southeast from about 1.0 s twt (2 km) at cdp 260 to 0.65 s twt (1.1 km) at cdp 380. Towards the northwest end of the total profile, the reflection at about 2 s twt had a marked sharp change in frequency response from a dominant frequency of 20 Hz to one of 40 Hz between about cdp 1000 to 1125 (C). This is not shot related as it occurred at approximately the same position as several shots. This degrades the final image as sharp spatial variable filtering is needed to bring out the event in the final section (A).
Fig. 4 Final shallow seismic section for the southern part of the profile across the Alpine Fault region. A possible reflection from the thrust segment of the Alpine Fault is marked by an arrow. Alpine Fault surface trace on is at cdp 380.
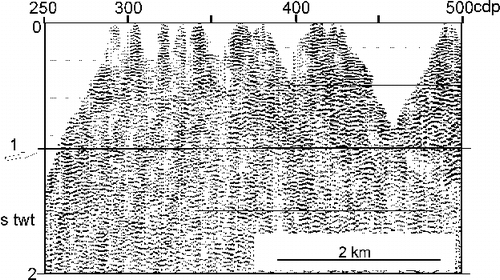
Fig. 5 (A) Final shallow seismic section and (B) interpreted shallow section with correlation to the geology recorded at Harihari-1 and Waiko-1 drill holes (located in ) and to offshore seismic profile SIGHT line 1W (); ‘bluff’: where the seismic line lies at base of a bluff ( inset); ‘Alpine fault’: vertical line as mapped on ; horizontal dashed line shows the possible extent of surface trace location. Interpreted seismic units delineated by short dashed lines. Possible fault strands of South Westland Fault marked by vertical dashed lines.
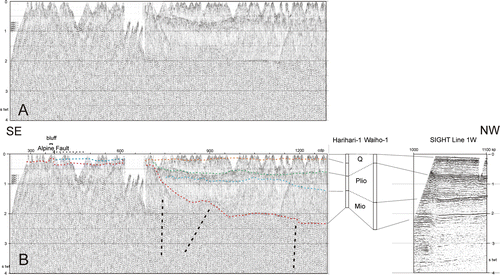
Deep section (crust and upper mantle)
Inspection of the shot gathers showed no primary coherent energy on the small shot data below about 3 s twt; only data from the large shots were therefore used. Some of these shots showed little primary energy. Shot gathers with good primary coherent energy at depth (> 5 s twt) for the full 636 channel (25 km) array were therefore selected by eye. A low-fold normal move out (NMO) corrected stack of variable angle reflections was performed for the whole array using stacking velocities based on the shallow-section analysis and on refraction data for SIGHT Transect 1 (Van Avendonk et al. 2004). The time section () was depth corrected using the same velocity field and is shown in .
Fig. 6 Deep seismic section with interpretation and correlation to the geology recorded at drill holes and to offshore seismic profile SIGHT line 1W (). Moho and mantle reflections interpreted on the reflection data are consistent with refraction data (van Avendonk et al. 2004; Melhuish et al. Citation2005); ‘Alpine fault’: vertical line as mapped on ; horizontal dashed line shows the possible extent of surface trace location.
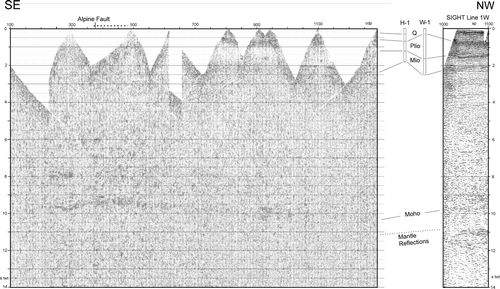
Fig. 7 Depth-converted deep seismic section (based on stacking velocities for the shallow section and wide angle velocities; Van Avendonk et al. 2004). The refraction model (P-wave velocity contours) of Van Avendonk et al. (Citation2004) is overlain on the section. Horizontal scale shows 216–240 km to reference the Van Avendonk et al. (Citation2004) model, 2–25 km to reference to the shallow refraction model in ; and cdp 200–1200 to reference the seismic reflection images in –6.
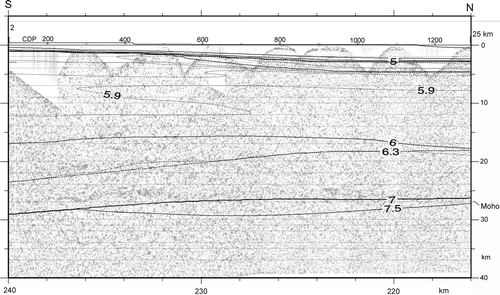
Forward modelling of refraction and Alpine Fault reflection data
A simple velocity model for the sediments and basement was derived by forward modelling of first break refraction arrivals from a limited selection of gathers from the large shots, using the ray-tracing code of Zelt & Smith (Citation1992), to aid the interpretation of the reflection sections. The profile was modelled in two parts: southeast and northwest of the profile dogleg. Shot gathers that gave good clear first break arrivals and were well distributed along the segment were selected for modelling. Shot positions were as measured from an origin just south of the river shot; receiver positions were as measured from their respective shots. The deepest layer in the northwest was derived from travel times for shots at the south-eastern end of the seismic line. The two models were linked using long offset data from shots on the other segment (). Modelling proceeded from the upper layer to deeper layers. Seismic velocities (P-wave only) that were constant with depth but variable along a layer were assumed and arrivals fitted to about 50 ms.
Fig. 8 Refraction model: (A) true scale and (B) details. Data from shots labelled at the top of (B) were used in the modelling. Refraction velocities for each layer are annotated on the section. Velocities were varied smoothly along layers but were kept constant vertically within a layer.
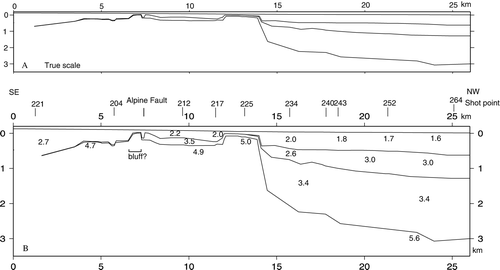
The south-eastern model comprises a single thin sedimentary layer (Vp=2.2–2.7 km s−1) over basement (4.7 km s−1) southeast of the mapped Alpine Fault trace, with two thin layers (Vp1=2.2 km s−1 and Vp2=3.5 km s−1) over basement immediately to the northwest (). The receiver array across the mapped Alpine Fault trace lies at the edge of the Whataroa valley and is close to a basement bluff (, inset); the shallow basement modelled here is probably unrelated to the Alpine Fault. Due to the wiggly track of the profile, the shot-receiver geometry varies significantly with respect to the bluff making a simple basement model difficult to derive there.
The north-western model shows a three-layer sedimentary section that rapidly increases in thickness to the northwest to about 2 km (). This offset in basement at the southeast of the basin is only partly directly imaged but is well constrained to the northwest and southeast and must be steep. The upper layer seismic velocity ranges from about 2.0 km s−1 in the southeast to 1.6 km s−1 near the coast. The intermediate layer ranges from about 2.6 km s−1 in the southeast to 3.0 km s−1 in the northwest. The lower sedimentary layer has a well-defined seismic velocity of 3.4 km s−1 at the top of the layer. The base of the layer and a basement velocity (5.6 km s−1) are defined by long offset arrivals from shots from the south end of the profile, and thus poorly defined. The basement velocity is similar to SIGHT Transect 1, but plausible variations in this velocity would change the thickness of layer 3.
The structure at the junction of the two profiles is derived from travel times for long offset data.
The first arrival data for these regions clearly and consistently show a decrease in travel time across this region corresponding to an offset in structure: uplifted at the north-western end of the south-eastern profile i.e. the start of the dogleg and down-dropped at the south-eastern end of the north-western segment, of about the same vertical offset. They may be recording the same feature (basement offset) oriented northwest across the basement plain because of the dogleg in the seismic line. However, the north-western offset coincides with the deepening of the sedimentary basin under the coastal plain to the northwest, and the interpretation of a shallow basement region on the southeast margin of the coastal basin is preferred.
Discussion
The seismic data recorded by the SIGHT98 Whataroa survey had a primary objective of imaging the north-western side of the Southern Alps orogen. In particular it was hoped that the data would image the Alpine Fault that has a strong reflection signature at depth (Davey et al. Citation1995; Kleffman et al. Citation1998; Okaya et al. Citation2007) and deformation of the crust of the Australian plate to the west of the plate boundary. The results are only partially successful as the dataset has a number of characteristics that degrade the quality of the recorded data produced, resulting in poor-quality images. These include the variable geometry of the profile (irregular track, major offset in mid-profile, wide and variable shot spacing), variable thickness near surface layer and the variable coupling and response of the earth for individual shots. Data quality tended to deteriorate for shots towards the north-western end of the profile, probably due to the increased sediment thickness and unconsolidated sediments in the low-lying coastal plain absorbing energy.
The Alpine Fault does not coincide with a strong reflector at the shallow depths imaged by these data. Geological data (Cox & Barrell Citation2007; Norris et al. Citation2010) indicate that the seismic profile crosses an intersection of the thrust and strike-slip segments of the Alpine Fault. A weak reflector is imaged dipping southeast at about 25° (), suggesting that the seismic profile obliquely crosses the thrust segment of the Alpine Fault. However, the reflection is deep and its extrapolation to the surface would lie too far to the north for it to be consistent with the documented surface trace of the Alpine Fault; it therefore must lie within its footwall. Alternatively, it may be documenting an abandoned section of the thrust following the ‘out-of-sequence’ imbrication model of Norris & Cooper (Citation1995), fig. 15).
At the Alpine Fault trace, the modelled shallow seismic basement is a local effect resulting from the close proximity of the seismic line to basement outcrop (as noted earlier). Basement (Vp>4.5 km s−1) lies at approximately the same depth (300–500 m) where the seismic line follows the centre of the Whataroa valley and onto the coastal plain. However, the sedimentary section to the northwest has a thin higher velocity (Vp=3.5 km s−1) layer immediately above basement, suggesting a structural discontinuity with the southeast. This velocity is similar to the lower sedimentary layer in the coastal plain basin to the north, in which case it may be of Mid–Late Miocene age (see below). The similar depth to basement along this part of the seismic profile suggests low uplift rates across the Alpine Fault, since deposition of the overlying sediments is to the southeast of the fault.
The reflectivity of the interface at this depth is small, in contrast to the inferred fault interface at greater (c. 20 km) depth (Stern et al. Citation2007). For future work, the fault appears to give a detectable signature on shot gathers and a well-designed seismic experiment should be able to image the fault well.
The coastal sedimentary basin is formed of three layers which are generally well defined by the seismic reflection and refraction data. These layers can be correlated with the sequences interpreted by Sircombe & Kemp (1998) for offshore data ( and 6). The uppermost layer has a seismic velocity that varies from 2.0 km s−1 in the southeast, where the basin sharply deepens, to 1.6 km s−1 at the north-western end. It increases from 400 m in the southeast to 700 down thrown to the northwest at about sp 1010m in the northwest (refraction). The lower velocity indicates a less well-consolidated sediment. A Quaternary (Pleistocene and younger) age is inferred for sediments from comparison with the Harihari-1 and Waiho-1 drill cores (New Zealand Petroleum Company Ltd. Citation1971b , Citation1972). It also probably corresponds to the top two layers of Sircombe & Kamp (Citation1998) where seismic velocities of 1.55−2.1 km s−1 were obtained. Layer two has a seismic velocity of 2.6–3.0 km s−1 and increases in thickness from 400 m in the southeast to 600 m thick at the north-western end. This would correspond to 2.5 km s−1 derived by Sircombe & Kamp (Citation1998); its age is likely Pliocene. Layer three has a seismic velocity of 3.4 km s−1 (possibly higher at depth) and is about 2000 m thick. Its south-eastern margin is not imaged but it is constrained to start at about 13–14 km along profile (cdp 670–770) and is probably fault controlled. Its age is probably Miocene and it is probably underlain by mid-Miocene limestone and Greenland Group basement. The seismic velocity is again higher than the velocity reported by Sircombe & Kamp (Citation1998) of 3.1 km s−1.
The character of the basement event changes sharply at about cdp 1000 and 1150, from a dominantly 20 Hz to a 40 Hz signal and back again (C). A possible cause could be near-surface conditions absorbing low-frequency energy. A more likely cause, however, is a thin strong reflector of a suitable thickness to reinforce high-frequency reflections (e.g. as noted by Bannister et al. Citation2008). This would be consistent with a thin patch of limestone on basement (as found in the Harihari-1 and Waiho-1 drillholes). The higher velocities onshore for the lower two layers may reflect closer proximity to source. A direct tie into offshore seismic data e.g. SIGHT lines 3W and 1W (Melhuish et al. Citation2005; Van Avendonk et al. 2004) is not possible; however, these lines also show three main layers separated by strong reflecting units at 0.7 s twt, 1.5 s twt and 2.0 s twt at their south-eastern end (SP 1001; cdp 130 line 1w). A reasonable correlation with the Whataroa profile and the drillhole results is shown in and 6.
The probably faulted southeast margin of the basin has a vertical offset of about 2000 m (). The South Westland Fault traverses the region, running subparallel with the coast and range front and is marked by a sharp down fault of basement to give 2000–3300 m of sediments towards the coast (Nathan et al. Citation1986; Cox & Barrell Citation2007). Its location is not well controlled in the Whataroa region and the seismic model suggests that its main strand runs closer to the Alpine Fault () than inferred by Cox & Barrell (Citation2007). The seismic reflection and refraction models ( and 8) also define other offsets in basement of about 800 m at cdp 900 and 500m at cdp 1200 ( and 5). These may be documenting normal or thrust faulting. The South Westland Fault therefore may be a fault zone with multiple strands resulting from the convergence on the leading edge of the Australian plate. Further offshore, the seismic data on SIGHT line1W (a) shows another basement offset down thrown to the northwest at about sp 1010.
The deep reflection section () shows several bands of subhorizontal reflectivity in the crust and upper mantle. At the south-eastern end of the profile, bands of reflectivity occur at 6–7 s twt, 7.5–8 s twt, 9–10 s twt and 11–11.5 s twt. The strongest and most continuous band of reflectivity is at 9–10 s twt and is inferred to mark the lowermost crust and Moho. It is traceable across the whole profile, but is greatly diminished in strength at its north-western end. The bands of mid-crustal reflectivity are only clear on the south-eastern part of the profile. The change in strength is attributed to poor coupling and absorption of energy in the shallow sediments under the north-western part of the profile.
The offshore SIGHT data (line 1W and 3W) also show a double band of reflectivity (), attributed to lower crust/Moho and an intra-mantle reflection band attributed to a palaeosubduction zone (Davey Citation2005; Melhuish et al. Citation2005). The data presented here indicate that this double-reflection band extends into the plate boundary region. The depth-converted seismic profile () shows that Moho is horizontal across the whole 25 km long profile. Comparison with the wide-angle veloci ty model for SIGHT Transect 1 (Van Avendonk et al. 2004) shows, in general, a very close correspondence of reflection Moho with refraction Moho (), but the models diverge under the Alpine Fault where the refraction model starts to dips under the Southern Alps. This may be a real difference but may alternatively be the result of smoothing in the tomographic modelling used by Van Avendonk et al. (Citation2004). The imaging of a horizontal Moho under the Alpine Fault supports arguments for a strong Australian plate (e.g. Scherwath et al. Citation2003)
Conclusions
The Whataroa seismic profile has delineated a deep (2.5–3 km) sedimentary basin under the coastal plain of Westland, near Whataroa. Three main sedimentary layers have been defined with seismic velocities of 1.6–2.0 km s−1, 2.6−3.0 km s−1 and 3.4 km s−1. Their thicknesses are: 400–700 m for the upper layer, 400–600 m for the middle layer and 1200–1800 m for the deepest layer. These layers can be correlated to onshore drillholes (Harihari-1 and Waihi-1) and offshore seismic data in the region, and are inferred to have ages of Holocene–Pleistocene, Pliocene and Mid–Late Miocene, respectively.
The crustal seismic section images a band of strong reflectivity corresponding to the lower crust and Moho. Moho is horizontal along the whole length of the profile; this is an unexpected result in view of the loading of the Australian Plate by the overthrust Pacific Plate from the east and the inferred foreland basin immediately offshore. A strong Australian Plate is inferred. A weak southeast-dipping reflector may correspond to an abandoned thrust segment of the Alpine Fault that extends well to the northwest of the range front or a deep splay fault in the footwall. Although there is no offset in basement across the range front, the addition of a higher velocity sediment layer to the northwest may indicate a strike-slip segment of the fault trace. Imaging the Alpine Fault trace within a few kilometres of the surface will be crucial as site survey data for planning the proposed ICDP drilling of the fault. The present survey design was deficient in several ways for achieving good images of the fault, but shows that images are possible with a well-designed experiment with attention to simple geometry with close shot and receiver spacing.
Acknowledgements
The support and enthusiasm of field crew (in particular A. Melhuish and D. Okaya) and the GECO-Western acquisition party is acknowledged. A. Melhuish carried out preliminary processing of the seismic data. Thoughtful reviews of the manuscript by S. Cox, T. Stern and D. Barrell were greatly appreciated. The research was supported by the New Zealand Foundation for Research, Science and Technology, grant C05514.
References
- Bannister S , Davey FJ , Woodward DJ 2008 . A lower crustal “bright spot” under the Bay of Plenty, North Island, New Zealand . Tectonophysics : doi: 10.1016/j.tecto.2008.09.044 .
- Brikke N 2007 . Seismic velocity structure of the shallow part of the Alpine fault and gravity study of the basement features in the Whataroa River flood plain, central Westland, South Island . Unpublished Graduate Diploma thesis, Victoria University of Wellington, Wellington, New Zealand .
- Carter , RM and Norris , RJ . 1976 . Cainozoic history of southern New Zealand: an accord between geological observations and plate tectonic predictions . Earth and Planetary Science letters , 31 : 85 – 94 .
- Cooper , AF and Norris , RJ . 1994 . Anatomy, structural evolution and slip rate of a plate boundary thrust: The Alpine Fault at Gaunt Creek, Westland, New Zealand. Geological Society of America . Bulletin , 106 : 627 – 633 .
- Cooper , RA . 1989 . Early Paleozoic terranes of New Zealand . Journal of the Royal Society , 19 ( 1 ) : 73 – 112 .
- Cox SC , Barrell DJA 2007 . Geology of the Aoraki area . Institute of Geological and Nuclear Sciences 1:250,000 geological map 15, 1 sheet+71 pages (compilers) GNS Science , Lower Hutt, , New Zealand .
- Davey FJ 2005 . A Mesozoic crustal suture on the Gondwana margin in the New Zealand region . Tectonics , 24 4 : TC4006 , doi: 1029/2004TC001719 .
- Davey FJ , Henyey T , Kleffmann S , Melhuish A , Okaya D , Stern T , Woodward DJ 1995 . A possible crustal seismic reflection from the Alpine Fault (Note) . New Zealand Journal of Geology and Geophysics the South Island Geophysical Transect (SIGHT) project working group 38 : 601 604 .
- Davey , FJ , Henyey , T , Holbrook , WS , Okaya , D , Stern , TA , Melhuish , A , Henrys , S , Eberhart-Phillips , D , McEvilly , T , Urhammer , R , Anderson , H , Wu , F , Jiracek , G , Wannermaker , P , Caldwell , G and Christensen , N . 1998 . Preliminary results from a geophysical study across a modern continent-continent collisional plate boundary— the Southern Alps, New Zealand . Tectonophysics , 288 : 221 – 235 .
- Kleffmann , S , Davey , F , Melhuish , A , Okaya , D and Stern , T . 1998 . Crustal structure in central South Island from the Lake Pukaki seismic experiment . New Zealand Journal of Geology and Geophysics , 41 : 39 – 49 .
- Long , DT , Cox , SC , Bannister , SC , Gerstenberger , MC and Okaya , D . 2003 . Upper crustal structure beneath the eastern Southern Alps and the Mackenzie Basin, New Zealand, derived from seismic reflection data . New Zealand Journal of Geology and Geophysics , 46 ( 1 ) : 21 – 39 .
- Melhuish A , Holbrook WS , Davey FJ , Okaya DA , Stern T 2005 . Crustal and upper mantle seismic structure of the Australian Plate, South Island, New Zealand . Tectonophysics , 395 , 113 135 , doi: 10.1016/j.tecto2004.09.005 .
- Mortimer , N . 2004 . New Zealand's Geological Foundations . Gondwana Research , 7 ( 1 ) : 261 – 272 .
- Nathan S , Anderson HJ , Cook RJ , Herzer RH , Hoskins RH , Raine JI , Smale D 1986 . Cretaceous and Cenozoic sedimentary basins of the West Coast region, South Island, New Zealand. New Zealand Geological Survey basin studies 1 . New Zealand Geological Survey , Lower Hutt .
- New Zealand Petroleum Company Ltd 1971a . New Zealand Ministry of Commerce Open-File Petroleum Report 523 .
- New Zealand Petroleum Company Ltd . 1971b . Well completion report, Harihari-1. New Zealand Ministry of Commerce Open-File Petroleum Report 528 .
- New Zealand Petroleum Company Ltd . 1972 . Well completion report, Waiho-1. New Zealand Ministry of Commerce Open-File Petroleum Report 529 .
- Norris , RJ and Cooper , AF . 1995 . Origin of small-scale segmentation and transpressional thrusting along the Alpine fault, New Zealand . Geological Society of America Bulletin , 107 : 231 – 240 .
- Norris RJ , Cooper AF , Read SE 2010 . http://www.otago.ac.nz/geology/research/structural_geology/alpinefault/07.html (accessed 5 January 2010)
- Okaya D , Stern T , Davey F 2007 . A Continental Plate Boundary: Tectonics at South Island, New Zealand Geophysical Monograph Series 175, American Geophysical Union, Washington, D.C.
- Scherwath M , Stern T , Davey FJ , Okaya D , Holbrook WS , Davies R , Kleffmann S 2003 . Lithospheric structure across oblique continental collision in New Zealand from wide-angle P wave modelling . Journal of Geophysical Research , 108 B12 , 2566 , doi: 10.1029/2002JB002286 .
- Sircombe , KN and Kamp , PJJ . 1998 . The South Westland Basin: seismic stratigraphy, basin geometry and evolution of a foreland basin within the Southern Alps collision zone, New Zealand . Tectonophysics , 300 ( 1/4 ) : 359 – 387 .
- Stern , T , Okaya , D , Kleffmann , S , Scherwath , M , Henrys , S and Davey , F . 2007 . “ Geophysical exploration and dynamics of the Alpine fault zone ” . In A Continental Plate Boundary: Tectonics at South Island, New Zealand, Geophysical Monograph Series 175 , Edited by: Okaya , D , Stern , T and Davey , F . Washington, D.C : American Geophysical Union .
- Sutherland , R . 1999 . Cenozoic bending of New Zealand basement terranes and Alpine Fault displacement: A brief review . New Zealand Journal Geology and Geophysics , 42 : 295 – 301 .
- Townend J , Sutherland R , Toy V 2009 . Deep Fault Drilling Project—Alpine Fault, New Zealand . Scientific Drilling , 8 , doi: 10.2204/iodp.sd.8.12.2009 .
- Van Avendonk HJA , Holbrook WS , Okaya D , Austin JK , Davey F , Stern T 2004 . Continental crust under compression: A seismic refraction study of SIGHT Transect I, South Island, New Zealand . Journal of Geophysical Research , 109 B6 , B06302 , doi: 10.1029/2003JB002790 .
- Zelt , CA and Smith , RB . 1992 . Seismic traveltime inversion for 2-D crustal velocity structure . Geophysical Journal International , 108 : 16 – 34 .