Abstract
Kaiika maxwelli is a new species of archaic fossil penguin from the Kauru Formation (Waipawan–Mangaorapan, Early Eocene) of the southern Canterbury Basin, Waihao River, South Canterbury, New Zealand. Kaiika maxwelli is represented by a well-preserved large and robust humerus, in which the broad m. scapulotricipitalis tendon sulcus, sigmoidal shaft and vestigial supracondylar process are similar to those of the basal penguin Waimanu, but differs from Waimanu and other penguins by having a deeply incised ventral tubercle and a smoothly curved profile of the deltoid crest and head. Humeral length suggests a body length of 1.3 m comparable to that of an emperor penguin, indicating that large penguins lived at a time of global warmth. This is the first significant fossil penguin named from pre-Bortonian (Middle Eocene) strata of the southern Canterbury Basin. Kaiika maxwelli is only the seventh species of fossil penguin reportedly older than Middle Eocene.
Introduction
The global suite of penguin species extends far beyond living taxa, with almost all of the c. 50 identified species belonging to the penguin stem-group, stem-Sphenisciformes. Fossil penguins are known from every Cenozoic epoch and have been collected from every major Gondwanan remnant except India (Jadwiszczak Citation2009). Penguin fossils typically occur as single bones (usually the dense and resistant elements of the wing and leg) and few species are known from skeletons. A taxonomy has developed based on isolated limb bones, particularly the upper wing bone or humerus which is the element detailed in this paper. The catalogue of fossil penguins has been extensively reviewed (e.g. Simpson Citation1946; Citation1971; Citation1972; Citation1975; Marples Citation1952; Fordyce & Jones Citation1990; Jadwiszczak Citation2009), with each successive study essentially confirming a conclusion drawn by Simpson (Citation1946): 69): ‘ … there was a mid-Tertiary radiation of penguins (doubtless rooted in the early Tertiary) which proceeded to an extreme of diversification within the limits of this striking adaptive type, … ’.The transition from volant to obligate-diving lifestyles in the penguin lineage preceded the mid-Tertiary radiation (of Simpson Citation1946) and occurred in the latest Cretaceous or earliest Palaeocene (Slack et al. Citation2006). The ‘root’ of the early stem-penguin radiation is poorly resolved, however, and only six named species have previously been reported from rocks older than the New Zealand Bortonian Stage (mid-Lutetian) – the first 23 million years of the Cenozoic period (65–42 Ma) (correlations throughout follow Cooper Citation2004).
Three of the six named early penguins are Palaeocene (65.0–55.5 Ma). The oldest named fossil penguins are latest Early Palaeocene to Late Palaeocene species from the Waipara Greensand, North Canterbury, New Zealand: Waimanu manneringi Jones et al. 2006 (in Slack et al. Citation2006) and Waimanu tuatahi Ando et al. 2006 (in Slack et al. Citation2006). Waimanu is superficially similar to northern hemisphere wing-propelled diving alcids (living family Alcidae) and to some of the northern hemisphere extinct penguin-like diving pelicaniforms (family Plotopteridae), but is more closely related to Spheniscidae than to any other extant group (Slack et al. Citation2006). The third reported species of Palaeocene penguin, Crossvallia unienwillia Tambussi et al. Citation2005, was recovered from the Cross Valley Formation of Seymour Island, Antarctica (Late Palaeocene; Tambussi et al. Citation2005). In contrast to the informative range of bones known for Waimanu, Crossvallia unienwillia is represented by an incomplete broken humerus and associated fragmentary femur and tibiotarsus of less certain age than for Waimanu. (Seymour Island strata were formed at high southern latitudes and generally lack the calcareous microfossils – foraminifera and coccoliths – elsewhere used widely to correlate temperate- to tropical-latitude sequences). Also from Seymour Island, three penguin species have been reported to range from Early Eocene to Late Eocene within the La Meseta Formation: Palaeeudyptes gunnari Wiman, Citation1905, Anthropornis grandis Wiman, Citation1905 and Delphinornis larseni Ameghino, Citation1905 (Myrcha et al. Citation2002; Jadwiszczak Citation2009).
Here we describe a new fossil penguin, represented by a well-preserved and informative Early Eocene humerus from New Zealand. This is the third-oldest species of New Zealand fossil penguin to be named and is notably better-preserved than the humeri of the older Waimanu tuatahi. The fossil adds to the known taxonomic and structural diversity of early penguins, reinforces the presence of penguins in New Zealand settings at a time of significant global warmth and continues to expand the pivotal New Zealand record of penguins.
Geological setting
Lithology and horizon
Kaiika maxwelli was discovered by PA Maxwell, who found it in a concretion split by hammering; it was collected by Maxwell and RE Fordyce, 25 November 1998 () as an ex situ concretion c. 30 cm in longest axis, from the floodplain on the southern bank of the Waihao River, South Canterbury. The matrix is massive, angular, carbonate-cemented quartz medium sand with occasional quartz pebbles and abundant millimetre-sized plant debris; the concretion has a superficial limonitic skin. Thin sections reveal pervasive secondary calcite cement, vascular plant fragments with fine cellular detail and rare heavy minerals (e.g. zircon, apatite). No mud component or foraminifera are evident, and the matrix is thus judged a poor prospect for any microfossils. The lithology is therefore the key to the stratigraphic horizon, which we identify as lower to middle Kauru Formation based on field observations which show there is no other potential source horizon in the Waihao Valley. The relevant section lies between McCulloch's Bridge (in old literature, McCullough's) and the informally-named ‘Don's hole’ (Fordyce & Maxwell Citation2003: 10), which is close to section line C-C′ shown by Allan (Citation1927): 266, map) and its intersection with the Waimate Fault (sensu Wilson Citation1953). In this section, south of Waimate Hills, the Palaeogene strata are gently anticlinal with shallow dips upstream at McCulloch's Bridge and downstream at ‘Don's hole’ toward the Waimate Fault.
Figure 1 Dr Phillip Maxwell with the concretion containing Kaiika maxwelli, floodplain of the Waihao River, 25 November 1998.
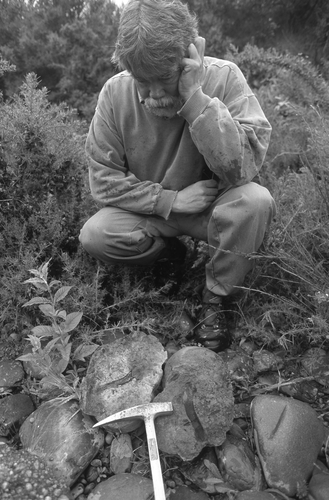
In this summary of the pre-Oligocene sequence, from top to bottom, lithostratigraphic terminology follows Field & Browne (Citation1986). Waihao Greensand is unlikely to be the source horizon as it is a massive muddy calcareous greensand and, in places, a glauconitic, quartzose, very fine sandstone and lacks the lithologies of the penguin concretion. There are concretionary phosphatic and limonitic horizons including the upper contact with the Burnside Mudstone (‘phosphatic band’ of Allan Citation1927: 284). The abundance of glaucony, dearth of coarser terrigenous clastic grains and generally massive bedding indicate that the Waihao Greensand was probably deposited in a middle to outer shelf setting. In the Waihao Valley, the unit is Bortonian (Maxwell Citation1992); it has a basal disconformity with the Kauru Formation. The Kauru Formation in the Lower Waihao Valley has a generally fining-upwards sequence of massive to bedded, sandy, quartz pebble conglomerate, massive to decimetre–centimetre bedded and laminated quartz sandstone and massive to laminated siltstone toward the top. The unit is locally concretionary; plant debris is locally abundant in lower to mid-sequence while glaucony is evident in the middle to upper sequence. Trace fossils are scattered throughout, while molluscan body fossils are rare in lower parts and more common in the middle to upper section. The abundance of terrigenous clastics and sedimentary structures related to traction currents is consistent with an inner to perhaps mid-shelf setting. The age is Waipawan or Mangaorapan (Maxwell Citation2003), but possibly older (see below); we have not seen a basal contact in the Lower Waihao Valley. Outcrops of the Broken River Formation in the sequence are difficult to access; the unit comprises massive to bedded siltstone, sandstone and conglomerate, and lignite seams that are not exposed in the river section but crop out on slopes to the north. In southern Canterbury Basin, the Broken River coal measures are non-marine with no marine body fossils reported (Field & Brown 1986). The age is uncertain.
To consider the Kauru Formation more closely, Field & Browne (Citation1986): 14; see also Field & Browne Citation1989: column J40/c1, 3, 4) reported c. 7 m of Kauru strata in the Lower Waihao Valley and attributed them to the Raupo Concretionary Sandstone member of the Formation. Since Field and Browne's work in the 1980s, floods have exposed more of the Formation although exposures are discontinuous. The age of the Kauru Formation at Waihao and elsewhere in the Canterbury Basin is problematic because of the difficulty of recovering age-diagnostic microfossils and because of uncertain correlation with similar units (e.g. the Tapui Formation of North Otago). Field & Browne (Citation1986) cited upper Dannevirke Series to Bortonian, a range that can be refined for the Lower Waihao because the overlying Waihao Greensand clearly represents the Bortonian Stage (Maxwell Citation1992; Citation2000), later Middle Eocene or later Lutetian–Bartonian (43–37 Ma). Maxwell (Citation2003), citing dinoflagellate determinations by GJ Wilson, gave the age for the Kauru Formation as Waipawan or Mangaorapan. In a discussion of Canterbury Basin invertebrates, Maxwell (Citation2000) noted that Heretaungan molluscan assemblages are generally absent, by implication from the Waihao Valley. Finally, molluscs from the lower Waihao Kauru Formation include species characteristic of the Wangaloan Stage, equivalent to middle Teurian or late Danian, 63–61 Ma (Beu et al. Citation1990, Stilwell Citation2003). It is possible that the lower Kauru Formation here could be older than Waipawan.
Preservation
The humerus, which formed a largely hollow natural mould within the concretion (), was discovered when the concretion was split and was filled with original fossil material only at the proximal and distal ends. Thin sections of the grey-white, friable material at the proximal end showed it to be bone in-filled with calcite. Haversian systems are readily discernable in thin section, and the periosteum and both subperiosteal and compacta zones are distinguishable from one another (Meister Citation1962). Material in-filling the distal end of the humerus was dark-grey to black and well cemented. Thin sections showed the distal-end material to be primarily calcite within an opaque mineral presumed to be pyrite.
Methods
Remaining loose bone was removed manually from the two natural moulds by hand scraping and use of a Chicago Pneumatic Air-scribe under a 10×–63× Zeiss binocular microscope with 200 mm focal length. The well-cemented matrix allowed for good separation, finer than 0.25 mm, at the bone-matrix interface. Each mould was washed with water and then acetone, dried and painted with a thin coat of catalysed polyester resin tinted with opaque ivory pigment. (Thin coats maximise heat loss and minimise distortion, when the resin sets). After c. 1 mm of resin had set, coats of resin and fine fibreglass matting were added. The two blocks were trimmed by saw to within c. 20 mm of the bone, and were then rejoined along the original split made at the discovery. The blocks fitted tightly except for occasional gaps up to 1 mm and at the distal end, where a large hole allowed resin to be introduced.
After resin had been poured in, the conjoined blocks were rotated so that the resin covered the join and thus glued the two parts. Further resin was added until the mould was filled. The matrix was then removed from the now resin-filled mould, using an Air-scribe under a binocular microscope. The reconstructed bone shows a distinct but small flash line at the mould junction. Missing bone at the distal end was not reconstructed; resin surface here is the minimum allowed by adjacent bone. Photographs were taken of the bone coated with sublimed ammonium chloride using a Nikon D200 camera and 60 mm Nikon micro lens.
Systematic palaeontology
AVES Linnaeus, 1758 (sensu Gauthier Citation1986)
NEOGNATHAE Pycraft, Citation1900
SPHENISCIFORMES Sharpe, Citation1891 (sensu Clarke et al. Citation2003)
Comment. We follow Clarke et al. (Citation2007) in using the hierarchy above without traditional levels of rank.
Genus Kaiika n. gen.
Type species. Kaiika maxwelli, by monotypy.
Content. Type species only.
Diagnosis. As for the type and only species, Kaiika maxwelli, below.
Etymology. Maori: kai, food; ika, fish; literally, eater of fish. Pronounced with 2 syllables; the i- of ika is as in English ee-, in seek.
Kaiika maxwelli n. sp. & .
Holotype. Left humerus (OU 22402) and an associated second thin fragment of unidentifiable bone; Geology Museum, University of Otago, Dunedin, New Zealand. Only known specimen.
Etymology. Named in honour of Phillip Maxwell (1940)–2007), the prominent New Zealand palaeontologist who discovered the specimen ().
Locality and age. Out of place boulder from the floodplain of the Waihao River, at 44.79°S, 171.02°E, grid reference NZMS260 J40/532998; derived from outcrops sporadically exposed in the Waihao River, South Canterbury, New Zealand. Kauru Formation, Waipawan-Mangaorapan, Early Eocene, as discussed above. Geological Society of New Zealand Fossil Record File locality number J40 /f 223.
Differential diagnosis. Kaiika maxwelli differs from other Sphenisciformes by a deeply excavated ventral tubercle on the humerus and the convex profile (in ventral view) of the deltoid crest, which is more-or-less continuous with the profile of the head but for the barely elevated dorsal tubercle. Differs from penguins other than Waimanu tuatahi in the markedly sigmoidal shaft, with the fossa for the supracoracoideus muscle elongate and positioned in mid-shaft, not distally skewed posteriorly; internal tuberosity or ventral tubercle faces distally rather than obliquely. Differs from Waimanu tuatahi in larger size, relatively wider shaft, presence of a preaxial angle and relatively larger transverse groove. Note that orientation terms are relative to a swimming, and not standing, posture (e.g. Thomas & Fordyce Citation2007).
Description. The left humerus ( & ) is complete and well-preserved but for distal features including parts of the radial and ulnar condyles and sesamoid grooves. Preservational artefacts include: a scrap of adherent thin bone dorsally near the preaxial angle; adjacent, several fine scratches, possibly scavenging traces, traversing the shaft; several post-mortem depressions on the ventral surface of the shaft; and apparent bioerosion (5 small deep grooves) on the posterior rim of the tricipital fossa. Otherwise, muscle scars are well-defined, consistent with the bird having been an adult rather than juvenile. Significant dimensions (in millimetres, to the nearest 0.5 mm) are: total length, 139+ (parallel to shaft, from crest of head to most-distal preserved margin of ulnar condyle); anteroposterior width at level of distal edge of tricipital fossa, 23; anteroposterior width at level of preaxial angle, 22; anteroposterior width at level of proximal edge of supracondylar callus (30 mm distal to the preaxial angle), 21; dorsoventral thickness at level of tricipital fossa, 13; dorsoventral thickness at level of preaxial angle, 10; dorsoventral thickness at level of proximal edge of supracondylar callus, 12.
Figure 2 Left humerus of Kaiika maxwelli (holotype, OU 22402). A, Dorsal; B, posterior; C, ventral; and D, proximal orientations. Note the small adherent fragment of bone in the distal half of the diaphysis, dorsal view.
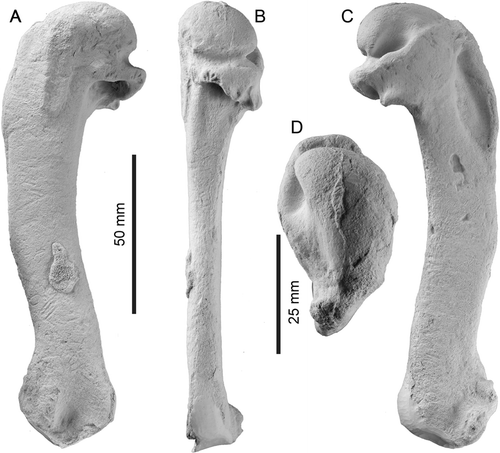
The bone is flattened dorsoventrally with a compressed-oval shaft that is widened anteroposteriorly, but which is rather more gracile than in Oligocene penguins such as Platydyptes and ‘Palaeeudyptes’. The humeral head has a smoothly rounded articular surface (); in proximal view, it is hemispherical with a rounded dorsal margin and a straighter ventral margin. Posteriorly (B, C), the head is bounded by the oblique capital incisura (capsular groove of Marples Citation1952) and a deep transverse groove or sulcus, in turn clearly separated (in posterior view) by a distinct crest that is excavated deeply on the ventral side by the transverse groove.
On the ventral face, the bicipital fossa for the insertion of the pectoral muscle is deep, elongate and narrowly elliptical, rather than parallel-sided. The fossa is bounded anteriorly by a robust deltoid crest which, in ventral view (C), has a gently convex profile that is almost continuous proximally with the curved profile of the head, other than for the slightly raised dorsal tubercle. The latter is a proximodistally elongate oval, barely distinct (in dorsal or ventral view) from the adjacent deltopectoral crest, in contrast to the more elevated tubercle of Oligocene penguins (e.g. Platydyptes, ‘Palaeeudyptes’). A proximal view (D), however, shows that a distinct groove separates the tubercle from the head.
In ventral view, an indistinct ligamental furrow passes anteriorly toward the dorsal tubercle. Distal to the transverse groove, the ventral tubercle (internal tuberosity) is a flat shelf, almost perpendicular to the shaft, on the ventral margin of the tricipital fossa; its distal orientation contrasts with the oblique position of the tubercle in Oligocene penguins. A prominent groove, seen in ventral view, separates the ventral tubercle from the more posterior robust margin of the tricipital fossa. This groove is more obvious in posterior view, where it is seen to rise towards to the capital incisura crest in a line parallel to the humeral shaft. The consistent surface detail on the specimen here indicates that the groove is natural, not a post-mortem artefact.
The tricipital (or pneumotricipital) fossa is large, subcircular, undivided and without a pneumatic foramen. The fossa is about as deep as wide, reaching proximally into the head to about level with the proximal edge of the capital incisura, but not excavating the head deeply. In dorsal view, the tricipital fossa has a prominent, straight, ridged dorsal margin that is developed roughly perpendicular to the shaft, but the margin does not extend obliquely (distally) down the shaft toward the distal end of the supracoracoideus insertion scar. There is therefore no oblique ridge of the sort that, in more crown-ward penguins (e.g. Platydyptes), carries the muscle scar for the posterior coracobrachialis muscle (m. coracobrachialis caudalis) along the posterior edge of the tricipital fossa (insertion for the pectoralis tertius muscle of Marples Citation1952: ). However, just proximal to the dorsal margin of the tricipital fossa, there is a raised tubercle marking the insertion of the posterior coracobrachialis muscle. Further anteriorly on the dorsal face, the supracoracoideus insertion scar is raised, elongate, prominent, parallel with the proximal end of the shaft rather than skewed posteriorly and situated in about the mid-shaft rather than toward the posterior margin. Proximally, it passes into the head without obvious boundary. A shallow subcircular fossa, the secondary tricipital fossa (D Ksepka 2010, personal communication ) is developed on the dorsal face, immediately posterior to the supracoracoideus muscle insertion and distal to the head. The fossa lies anterior to, and grades into, the capital incisura and distally passes smoothly onto the shaft, thus bounding the raised tubercle possibly for the posterior coracobrachialis muscle (above). In Platydyptes, this part of the humerus forms a distinct triangular fossa between the head of the humerus, the robust dorsal margin of the tricipital fossa and the supracoracoideus insertion.
The shaft is gracile, sigmoidal in dorsal or ventral view and does not expand distally. A slight prominence marks the preaxial (or anterior) angle, indicating the proximal border of the origin of the brachialis muscle, a little beyond mid-shaft length. A latissimus dorsi insertion scar is not observed; the generally well-preserved bone surface is consistent with a real absence of the scar, rather than erosion. There is no discernable humeral arterial sulcus traversing the ventral surface of the shaft.
Some distal features are lost or eroded, particularly the trochlear processes and related posterodistal structures. A supracondylar process (an eroded spur on a larger basal callus) is present slightly ventral to the anterior margin and proximal to the radial condyle (E). Such a structure has been reported otherwise for penguins (Clarke et al. Citation2007) only in Waimanu and Perudyptes (termed supracondylar tubercle). The radial condyle is not easily discerned in dorsal or ventral view, but in anterodistal view (E) appears tabular rather than gently subspherical as in most younger penguins. The ulnar condyle lies more posteriorly and ventrally and is less distinctly separated from the radial condyle than in, for example, Platydyptes in which the intercondylar depression is saddle-shaped. The ulnar condyle has a complete semicircular distal profile which is difficult to discern because the ventral face is damaged. In a distal view, the ulnar condyle is elongate anteroposteriorly, and the articular surface is a curved facet rather than a subspherical condyle. In ventral view, the ulnar condyle is bounded by a broad semicircular depression that separates it from the supracondylar process and the indistinct insertion for the entepicondylar ligament. The tangent between the ulnar and radial condyles and the humeral shaft is <45°.
Figure 3 Detailed views of Kaiika maxwelli (holotype, OU 22402); A, dorsal; B, postero-distal; and C, posterior orientations of the proximal end of the humerus; D, dorsal; E, posterior; and F, ventral orientations of the distal end of the humerus. The distal end has been eroded and minimally reconstructed.
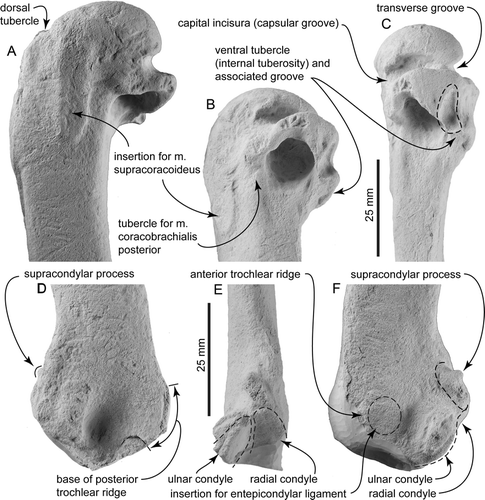
The bases of two trochlear ridges, anterior and posterior, are present at the distal end of the humerus. Not enough details are preserved to say whether, as in Waimanu spp., a medial trochlear process was naturally absent. The sulcus for the scapulotricipitalis muscle is broad and deep. The anterior trochlear ridge is preserved as an elevated callus at the distal end of the humerus that passes back into the insertion for the entepicondylar ligament and then the radial condyle on the ventral surface. The posterior trochlear ridge is represented only by its broken broad base, which has a concave distal border bounded by a remnant of matrix.
Discussion
Significance of humeral characters
In penguins, the humerus is a robust bone that preserves well in fossils. It also happens that the humerus is a structurally complex element with many features related to muscle attachments and bone articulations, and thus wing function and lifestyle. In living penguins, the size and structure of the humerus are reasonably diagnostic at the species level; many fossil species have been based solely or partly on, and their size judged from, the humerus (e.g. Marples Citation1952, Simpson Citation1946; Citation1971, Slack et al. Citation2006). The Waihao humerus thus allows ready comparison with other archaic penguins.
The humerus of Kaiika maxwelli is distinguished from all other penguin humeri by having a deeply excavated and distally facing ventral tubercle (A–C, B) which, in posterior view, is seen to form the ventral-most part of the humeral head. The ventral tubercle (internal tuberosity of Marples Citation1952) is reduced in many stem and all crown penguins, and demarcated in all penguins other than K. maxwelli, by a shallow attachment scar for the caudal coracobrachialis muscle (CBC). The CBC is vestigial in penguins (Schreiweis Citation1982), but helps to depress the wing of volant birds during flight and, consequently, is attached to humeri of flying birds by a deeply incised ventral tubercle. The humerus of K. maxwelli is clearly that of a basal penguin rather than some other bird group, and is too robust to belong to a volant species. The presence of a deeply incised ventral tubercle, and likewise the small supracondylar process, indicates a close association with flying ancestors. It is consistent with the otherwise archaic form of the humerus and a basal position within stem-Sphenisciformes.
Comparison with broadly contemporaneous penguins
Three Palaeocene to Middle Eocene fossil penguin species with humeri have previously been reported: Waimanu tuatahi (60–58 Ma, New Zealand); Crossvallia unienwillia (c. 55 Ma, Seymour Island Antarctica); and Perudyptes devriesi (c. 42 Ma, Peru). Kaiika maxwelli differs sufficiently from all in size, proportions, shape and details of muscle scars to warrant its recognition as a new genus.
The length, median shaft width (at preaxial angle) and median shaft thickness (at preaxial angle) for K. maxwelli are 139, 22 and 12 mm respectively (). The humeral shaft in W. tuatahi is shorter, narrower (median shaft width divided by length) and less flattened (median shaft thickness divided by width) when compared with K. maxwelli. Waimanu tuatahi lacks many of the detailed features preserved in K. maxwelli, precluding closer comparisons; further, the humerus is not known of the older and larger Waimanu manneringi. The P. devriesi humerus is also shorter than in K. maxwelli, but the former is as broad as, and more flattened than, the latter. The proximal margin of the tricipital fossa is well developed in K. maxwelli and is visible in proximal view of the humeral head. The margin is also visible in proximal view for W. tuatahi but not in P. devriesi (Ksepka & Clarke Citation2010: fig.5c) and appears to be missing (damaged) in C. unienwillia (Tambussi et al. Citation2005: c). The relative position of the dorsal tubercle to the apex of the humeral head is most distal for K. maxwelli, more proximal in C. unienwillia (Tambussi et al. Citation2005: a) and nearly level in both W. tuatahi and P. devriesi (Ksepka & Clarke Citation2010: fig. 5b).
The insertion scar of the pectoralis muscle in K. maxwelli is long (23% of total humerus length), broad (breadth 31% of scar length) and deep. In comparison, the m. pectoralis scar is longer, narrower and shallower in W. tuatahi. The pectoralis muscle scar of P. devriesi is shorter and narrower than K. maxwelli (Ksepka & Clarke Citation2010: fig. 5a), and damaged but apparently broad and deep in C. unienwillia (Tambussi et al. Citation2005: a). Waimanu tuatahi, P. devriesi and K. maxwelli each have strongly elevated and elongated supracoracoideus muscle insertion scars, a character earlier identified (Slack et al. Citation2006) as autapomorphic in Waimanu tuatahi. The supracoracoideus muscle insertion scar of W. tuatahi is more strongly developed than K. maxwelli. The preaxial angle in P. devriesi is well developed, but weakly developed in K. maxwelli and C. unienwillia (= angulation of anterior margin; Tambussi et al. Citation2005) and absent in W. tuatahi.
Distally, the K. maxwelli humerus retains an (eroded) supracondylar process, similar to that identified by Clarke et al. (Citation2007) for P. devriesi and W. tuatahi. The sulcus of the scapulotricipitalis muscle is broad and shallow in K. maxwelli and, like W. tuatahi, is not bounded by distinct trochlear ridges. Previously (Slack et al. Citation2006), such a structure was regarded as an autapomorphy originally reported for Waimanu tuatahi. Within Sphenisciformes, only K. maxwelli, W. tuatahi and possibly the poorly preserved C. unienwillia have humeri that lack well-developed trochleae.
Comparison with younger penguins
In the literature, 49 species of fossil penguins with humeri have been reported of which most belong in the stem-Sphenisciformes (44 species). In addition to characters discussed immediately above, the Kaiika maxwelli humerus retains the primitive state for six characters diagnostic for the penguin crown group (Spheniscidae) (Clarke et al. 200;, Ksepka & Clarke Citation2010), further supporting placement as a stem-penguin. The articular surface of K. maxwelli is oriented ventrally and the tricipital fossa is a single excavation, features that are observed in taxa as young as Archaeospheniscus lowei Marples, Citation1952 (Late Oligocene, New Zealand; Ksepka & Clarke Citation2010). The lack of distal widening in K. maxwelli is characteristic of Palaeogene taxa and the sigmoidal shape of the humerus is observed in Waimanu tuatahi, Perudyptes devriesi, four Late Eocene species from Seymour Island, Antarctica (Anthropornis grandis Wiman, Citation1905, A. nordenskjoeldi Wiman, Citation1905, Palaeeudyptes gunnari Wiman, Citation1905 and P. klewkowskii Myrcha et al., Citation1990) and Archaeospheniscus lowei (e.g. Ksepka & Clarke Citation2010). The radial and ulnar condyles form a tangent that is less than 45° to the midline of the humeral shaft and the ulnar condyle is projected and rounded; such features all represent primitive character states for Sphenisciformes (Marples Citation1952; Ksepka et al. Citation2006) .
Comparison with Plotopteridae
Plotopterids are extinct diving penguin-like pelicans known from Japan and North America, with three of the six named species within Plotopteridae based on humeri. Broad similarities between plotopterid and spheniscid humeri (e.g. sigmoidal shape, well-developed humeral head incised by a tricipital fossa and the possession of a deltoid crest) have been explained either as convergent evolution (Olson Citation1980; Olson & Hasegawa Citation1996) or as close phylogenetic relationship (Mayr Citation2005). Some features of K. maxwelli are reminiscent of those in the plotopterids Copepteryx hexeris Olson & Hasegawa, Citation1996, Tonsala hildegardae Olson, Citation1980, and an unnamed plotopterid identified as specimen UWBM 86871 (Burke Museum of Natural History and Culture) (Mayr Citation2009). Like K. maxwelli and Waimanu tuatahi, all three plotopterid specimens have a shallow secondary tricipital fossa that is not bounded by a ridge of bone along the posterior edge (Olson Citation1980: a; Olson & Hasegawa Citation1996: fig. 5a; Mayr Citation2009: fig.7.4a). Furthermore, the transverse grooves of K. maxwelli, Copepteryx hexeris and Tonsala hildegardae appear deeply incised (Olson Citation1980: b; Olson & Hasegawa Citation1996: fig. 5b) with the groove of the former two taxa terminating into a capital incisura crest (Olson & Hasegawa Citation1996: fig. 4b). Tonsala hildegardae features a broad preaxial angle, suggesting a reduction of the biceps muscle seen in crown-Spheniscidae (Schreiweis Citation1982).
Palaeobiology
Caution is appropriate when inferring aspects of palaeobiology from a single bone. Nevertheless, some inferences can be made. Simpson (Citation1975); see also Jadwiszczak Citation2001) discussed the use of fossil penguin bones as a proxy for original body size based on extrapolation from sizes of limb bones, especially the humerus, femur and tarsometatarsus, in living species. Such extrapolation assumes that fossil species had proportions similar to those of living species, but no fossil species have yet been described from skeletons that are complete enough to confirm proportions.
Using the multipliers of Simpson (Citation1946): 75) and assuming body proportions as in living penguins, the humerus of Kaiika maxwelli at 139+ mm indicates a total body length of approximately 1.3 m (and body height 5–10% less, in the range 1.1–1.2 m). Furthermore, humerus length allows prediction of femoral and tarsometatarsi lengths (calculations based on Simpson Citation1946), and also estimation of a body mass for K. maxwelli of 35+ kg (calculations based on Jadwiszczak Citation2001). For comparison, a modern emperor penguin (Aptenodytes forsteri Gray, 1844 ) has a maximum total body length of 1.2 m and can weigh up to 30 kg (Stonehouse Citation1975). The one adult emperor penguin skeleton available for study (University of Canterbury, Department of Zoology, 1054) has a left humerus 135.5 mm long with length from head of humerus to distal apex of ulnar condyle 129.0 mm; the latter dimension in K. maxwelli is 139+. These comparisons support the notion that K. maxwelli was slightly taller and heavier than an emperor penguin. To compare K. maxwelli with another early penguin from New Zealand, Waimanu tuatahi has a humerus 107 mm long, indicating a body length of about 1.0 m and body height in the range 0.8–0.9 m (calculations based on Jadwiszczak Citation2001). Some caution is appropriate here, for the tarsometatarsus in Waimanu tuatahi is relatively longer than expected given the length of the humerus (Slack et al. Citation2006), indicating that body proportions probably differed from those of later penguins.
If the age of Kaiika maxwelli is Waipawan–Mangaorapan and not older, then the species lived at a time of marked warmth during the Palaeocene/Eocene Thermal Maximum or the Early Eocene Climatic Optimum (Bijl et al. Citation2009; Hollis et al. Citation2009). Zealandia (proto-New Zealand) straddled 55°S at this time, and the surrounding coastal waters reportedly experienced temperatures now found at sub-tropical and tropical latitudes (exceeding 30°C; Hollis et al. Citation2009). Those living penguins (species of Spheniscus) that forage in modern-day warm oceans reach a maximum length of 0.6 m and require heat-loss strategies to tolerate hot onshore environments (Frost et al. Citation1975; Stonehouse Citation1975). In contrast, K. maxwelli was approximately the size of an emperor penguin, a species currently restricted to cool settings of coastal Antarctica. The relatively low surface to volume ratio of emperor penguins, and by inference K. maxwelli, suggests that the latter species would have suffered considerable heat stress unless it had evolved thermal-coping mechanisms; we would expect that the latter appeared very early in penguin history. Alternatively, K. maxwelli may have been a vagrant from deeper southern latitudes, as occasionally happens with Antarctic penguin species in New Zealand. Such occasions are rare however; given biases against fossilisation, this situation is considerably less likely.
Finally, Clarke et al. (Citation2007) suggested that large body size in stem-Sphenisciformes evolved once, before the Middle Eocene. Kaiika maxwelli is sufficiently large and of the right age to support the earlier Palaeogene origins of large body size. More complete material of Kaiika maxwelli would allow phylogenetic analyses to establish relationships with large Palaeogene Antarctic and South American penguins.
Acknowledgements
We thank the late Andrew Grebneff for help with preparation; Tatsuro Ando and Daniel Ksepka for discussions on fossil penguins and for helpful and constructive reviews of the manuscript; and Gabriel Aguirre and Felix Marx for reviewing the manuscript. The Albrey, Campbell, Cochran and Studholme families kindly granted access. DBT was supported by research funds from the Department of Geology, University of Otago. REF acknowledges the many rewarding field trips in the Waihao Valley and beyond with the late Phillip A. Maxwell – a profound philosopher of palaeontology and stratigraphy in New Zealand in the later 20th century.
References
- Allan , RS . 1927 . The geology and palaeontology of the Lower Waihao Basin, South Canterbury, New Zealand . Transactions of the New Zealand Institute , 57 : 265 – 309 .
- Ameghino , F . 1905 . Enumeracion de los impennes fosiles de la Patagonia e Isla Seymour . Anales del Museo nacional de historia natural, Buenos Aires , 6 : 97 – 167 .
- Beu , AG , Maxwell , PA and Brazier , RC . 1990 . Cenozoic Mollusca of New Zealand . New Zealand Geological Survey Paleontological Bulletin , 58 : 1 – 518 .
- Bijl , PK , Schouten , S , Sluijs , A , Reichart , G-J , Zachos , JC and Brinkhuis , H . 2009 . Early Palaeogene temperature evolution of the southwest Pacific Ocean . Nature , 461 : 776 – 779 .
- Clarke , JA , Olivero , EB and Puerta , P . 2003 . Description of the earliest fossil penguin from South America and first Paleogene vertebrate locality of Tierra del Fuego, Argentina . American Museum Novitates , 3423 : 1 – 18 .
- Clarke , JA , Ksepka , DT , Stucchi , M , Urbina , M , Giannini , N , Bertelli , S , Narváez , Y and Boyd , CA . 2007 . Paleogene equatorial penguins challenge the proposed relationship between biogeography, diversity, and Cenozoic climate change . Proceedings of the National Academy of Sciences of the United States , 104 : 11545 – 11550 .
- Cooper RA 2004 . The New Zealand geological timescale . Institute of Geological and Nuclear Sciences Monograph 22 : 1 284 .
- Field , BD and Browne , GH . 1986 . Lithostratigraphy of Cretaceous and Tertiary rocks, southern Canterbury, New Zealand . New Zealand Geological Survey Record , 14 : 1 – 55 .
- Field , BD and Browne , GH . 1989 . Cretaceous and Cenozoic sedimentary basins and geological evolution of the Canterbury Region, South Island, New Zealand . New Zealand Geological Survey Basin Studies , 2 : 1 – 94 .
- Fordyce , RE and Jones , CM . 1990 . “ The history of penguins, and new fossil penguin material from New Zealand ” . In Penguin Biology , Edited by: Davis , LS and Darby , JD . 419 – 446 . San Diego : Academic Press .
- Fordyce , RE and Maxwell , PA . 2003 . Canterbury basin paleontology and stratigraphy. Geological Society of New Zealand Annual Conference 2003 field trip guides . Geological Society of New Zealand Miscellaneous Publications , 116B : FT8-1 – 18 .
- Frost , PGH , Siegfried , WR and Greenwood , PJ . 1975 . Arteriovenous heat exchange systems in jackass penguin Spheniscus demersus . Journal of Zoology , 175 : 231 – 241 .
- Gauthier , J . 1986 . Saurischian monophyly and the origin of birds . Memoirs of the California Academy of Sciences , 8 : 185 – 197 .
- Gauthier , J . 1986 . Saurischian monophyly and the origin of birds . Memoirs of the California Academy of Sciences , 8 : 185 – 197 .
- Hollis CJ , Handley L , Crouch EM , Morgans HEG , Baker JA , Creech J , Collins KS , Gibbs SJ , Huber M , Schouten S and others 2009 . Tropical sea temperatures in the high-latitude South Pacific during the Eocene . Geology 37 : 99 102 .
- Jadwiszczak , P . 2001 . Body size of Eocene Antarctic penguins . Polish Polar Research , 22 : 147 – 158 .
- Jadwiszczak , P . 2009 . Penguin past: The current state of knowledge . Polish Polar Research , 30 : 3 – 28 .
- Ksepka , DT and Clarke , JA . 2010 . The basal penguin (Aves: Sphenisciformes) Perudyptes devriesi and a phylogenetic evaluation of the penguin fossil record . Bulletin of the American Museum of Natural History , 337 : 1 – 77 .
- Ksepka , DT , Bertelli , S and Giannini , NP . 2006 . The phylogeny of the living and fossil Sphenisciformes (penguins) . Cladistics , 22 : 412 – 441 .
- Marples , BJ . 1952 . Early Tertiary penguins of New Zealand . New Zealand Geological Survey Paleontological Bulletin , 20 : 1 – 66 .
- Maxwell , PA . 1992 . Eocene Mollusca from the vicinity of McCulloch's Bridge, Waihao River, South Canterbury, New Zealand: paleoecology and systematics . New Zealand Geological Survey Paleontological Bulletin , 65 : 1 – 280 .
- Maxwell , PA . 2000 . Early Paleogene climates in the southwest Pacific: Evidence from marine Mollusca and other invertebrates . Geologiska Föreningens i Stockholm Förhandlingar , 122 : 104 – 105 .
- Maxwell , PA . 2003 . The Volutid genera Athleta and Lyria (Mollusca: Gastropoda) in the New Zealand Cenozoic . Journal of the Royal Society of New Zealand , 33 : 363 – 394 .
- Mayr , G . 2005 . Tertiary plotopterids (Aves, Plotopteridae) and a novel hypothesis on the phylogenetic relationships of penguins (Spheniscidae) . Journal of Zoological Systematics and Evolutionary Research , 43 : 61 – 71 .
- Mayr , G . 2009 . Paleogene fossil birds , 262 Berlin : Springer .
- Meister , W . 1962 . Histological structure of the long bones of penguins . The Anatomical Record , 143 : 377 – 387 .
- Myrcha , A , Tatur , A and del Valle , R . 1990 . A new species of fossil penguin from Seymour Island, West Antarctica . Alcheringa , 14 : 195 – 205 .
- Myrcha , A , Jadwiszczak , P , Tambussi , CP , Noriega , JI , Gaździcki , A , Tatur , A and del Valle , R . 2002 . Taxonomic revision of Eocene Antarctic penguins based on tarsometatarsal morphology . Polish Polar Research , 23 : 5 – 46 .
- Olson , SL . 1980 . A new genus of penguin-like pelecaniform bird from the Oligocene of Washington (Pelecaniformes: Plotopteridae). In: Campbell KE Jr ed. Papers in avian paleontology honoring Hildegarde Howard. Contributions in Science . Natural History Museum of Los Angeles County , 330 : 51 – 57 .
- Olson , SL and Hasegawa , Y . 1996 . A new genus and two new species of gigantic Plotopteridae from Japan (Aves: Pelecaniformes) . Journal of Vertebrate Paleontology , 16 : 742 – 751 .
- Pycraft , WP . 1900 . Part II: on the morphology and phylogeny of the Palaeognathae (Ratitae and Crypturi) and Neognathae (Carinatae) . Transactions of the Zoological Society of London , 15 : 149 – 290 .
- Schreiweis , DO . 1982 . A comparative study of the apendicular musculature of penguins (Aves: Sphenisciformes) . Smithsonian Contributions to Zoology , 341 : 1 – 46 .
- Sharpe , RB . 1891 . A review of recent attempts to classify birds . Proceedings of the Second International Ornithological Congress , 2 : 1 – 90 .
- Simpson , GG . 1946 . Fossil penguins . Bulletin of the American Museum of Natural History , 87 : 7 – 99 .
- Simpson , GG . 1971 . A review of the pre-Pliocene penguins of New Zealand . Bulletin of the American Museum of Natural History , 144 : 319 – 378 .
- Simpson , GG . 1972 . Pliocene penguins from North Canterbury, New Zealand . Records of the Canterbury Museum , 9 : 159 – 182 .
- Simpson , GG . 1975 . “ Fossil penguins ” . In The Biology of Penguins , Edited by: Stonehouse , B . 19 – 41 . London : Macmillan Press .
- Slack , KE , Jones , CM , Ando , T , Harrison , GL , Fordyce , RE , Arnason , U and Penny , D . 2006 . Early penguin fossils, plus mitochondrial genomes, calibrate avian evolution . Molecular Biology and Evolution , 23 : 1144 – 1155 .
- Stilwell , JD . 2003 . Patterns of biodiversity and faunal rebound following the K-T boundary extinction event in Austral Palaeocene molluscan faunas. Palaeogeography, Palaeoclimatology . Palaeoecology , 195 : 319 – 356 .
- Stonehouse , B . 1975 . “ Introduction: The Spheniscidae ” . In The Biology of Penguins , Edited by: Stonehouse , B . 321 – 336 . London : Macmillan Press .
- Tambussi , CP , Reguero , MA , Marenssi , SA and Santillana , SN . 2005 . Crossvallia unienwillia, a new Spheniscidae (Sphenisciformes, Aves) from the Late Paleocene of Antarctica . Geobios , 38 : 667 – 675 .
- Thomas , DB and Fordyce , RE . 2007 . The heterothermic loophole exploited by penguins . Australian Journal of Zoology , 55 : 317 – 321 .
- Wilson , DD . 1953 . Geology of the catchment of Waimate Stream, South Canterbury, New Zealand . New Zealand Journal of Science and Technology , B35 : 14 – 21 .
- Wiman , C . 1905 . ber die altertiären Vertebrata der Seymourinsel. Wissenschaftliche Ergebnisse der Schwedischen Südpolar? . Expedition 1901–1903 , 3 : 1 – 37 .