Abstract
The Blue Lake Fault Zone occurs at a major crustal boundary between Otago Schist and Torlesse greywacke. This zone has been active since the middle Cretaceous (c. 112 Ma), when extensional exhumation of the Otago Schist belt was initiated. The Blue Lake Fault Zone is dominated by a set of normal faults that have caused juxtaposition of rocks of different metamorphic grade, from prehnite-pumpellyite facies turbidites to pervasively recrystallised greenschist facies schists. This metamorphic transition has been thinned from c. 15 km to c. 2 km by normal fault motion on the scale of kilometres. This condensed section is the narrowest such section on the Otago Schist margin. The faults in this condensed section are defined by gouge zones that are hundreds of metres wide and constitute about 30% of the section. Gouge zones were locally reactivated as thin Late Cenozoic reverse faults on the centimetre to metre scale.
Introduction
The boundary between the Mesozoic Otago Schist and Torlesse greywacke terrane to the northeast is a major structural feature in South Island geology, and reflects a discontinuity in crustal structure and thickness (A; Upton et al. Citation2009). The boundary is a complex structural zone that has been periodically tectonically active for more than 100 million years (Gray & Foster Citation2004; Mitchell et al. Citation2009; Upton et al. Citation2009). On a regional scale, the boundary between greywacke and schist along the north-eastern margin of the schist belt appears to be gradational, with progressive increase in metamorphic grade towards the southwest (Bishop Citation1972; Forsyth Citation2001). The present width of the surface expression of this apparent transition varies widely along the schist margin, but is typically of the order 10 km (Bishop Citation1972; Forsyth Citation2001). However, this transition zone becomes as narrow as 2 km wide in the Manuherikia valley of Central Otago (B). This anomalously narrow greywacke-schist transition is the focus of this paper. The surface expression of the major crustal structural boundary between schist and greywacke (A) is defined by two principal structures at the surface: the Hawkdun Fault Zone and the Blue Lake Fault Zone (B, 2). The Hawkdun Fault Zone has the most prominent present topographic expression, with a distinctive fault scarp (>1 km high) on the southwest side of the Hawkdun Range (B). However, the Hawkdun Fault Zone has little lithological change across it, and generally juxtaposes greywacke against greywacke (B, 2). In contrast, the Blue Lake Fault Zone defines at the surface the lithological boundary between the Torlesse greywacke terrane and the Otago Schist (B; MacKenzie & Craw Citation2005).
Figure 1 Locality diagrams for the Blue Lake Fault Zone. A, Location of the area of the Blue Lake Fault Zone of this study, in relation to the crustal discontinuity between Torlesse greywacke and Otago Schist (Upton et al. 2009) in the South Island of New Zealand. B, General geology (partly after Forsyth 2000) of the area surrounding the Manuherikia river gorge, which extends from Falls Dam to the Manuherikia flats near Loop Road. C, D, Sketch cross-sections through active strands of the Blue Lake Fault Zone, at localities marked in B.
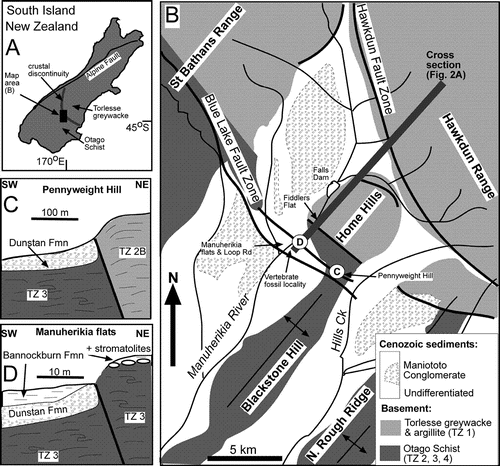
The boundary between greywacke and schist also coincides with a major change in Late Cenozoic and Recent tectonic deformation as a result of the underlying crustal differences (Upton et al. Citation2009). The Otago Schist is currently deforming into a set of northeast-trending antiformal ridges separated by synformal basins (B; Jackson et al. Citation1996; Craw et al. Citation2007). These folds terminate at their north-eastern ends against the northwest-striking Blue Lake and Hawkdun Fault Zones (B). Strands of the Blue Lake Fault have Late Cenozoic and Recent (active) offset (C, 1D; Lindqvist Citation1994; Forsyth Citation2001). The structural change from folded schist to faulted greywacke is linked to the lithological variations at the small to medium scale and to the major crustal discontinuity at the larger scale (Upton et al. Citation2009).
Despite the regional significance of this major structural zone in Central Otago, there is little detailed information on the structure and lithological variations within the zone. This paper reports on observations from a detailed structural section through the Blue Lake Fault Zone, based on a previously undescribed extremely well-exposed river section that cuts across the fault zone from Torlesse greywacke to lower greenschist facies Otago Schist. Parts of the section are overlain by Miocene sediments, and these sediments were deposited during active deformation along the Blue Lake Fault Zone. Some sedimentary facies are directly linked to fault activity (Lindqvist Citation1994; Youngson et al. Citation1998), and some of these sediments contain important vertebrate fossils (Worthy et al. Citation2006). This paper provides some additional structural context for the sediments which contain these significant fossils.
Geological setting
The Mesozoic Otago Schist belt formed during Jurassic collision and metamorphism of Torlesse and Caples Terrane turbiditic complexes that are dominated by greywacke (Mackinnon Citation1983; Mortimer Citation1993; Gray & Foster Citation2004; Little et al. 2009). Metamorphic grade increases from prehnite-pumpellyite facies greywackes to the southwest (Caples Terrane) and northeast (Torlesse Terrane) of the belt to upper greenschist facies, with biotite and garnet, in the core of the belt (Craw Citation1998; Mortimer Citation2000). The metamorphic gradient on the margins of the schist belts includes pumpellyite-actinolite facies semischists and lower greenschist facies (chlorite zone) schists (; Bishop Citation1972).
Figure 2 Cross-sections through the northeast margin of the Otago Schist belt. A, Regional cross-section along a line shown in B, across the Hawkdun and Blue Lake Fault Zones. Different textural zones and metamorphic grades in the basement and the Miocene unconformity (dotted) with preserved remnants of overlying Miocene sediments (thick black lines) are depicted. B, Cross-section through the Blue Lake Fault Zone (as indicated in A), compiled from the Manuherikia gorge river section. Approximate locations of diagrams in this paper are indicated.
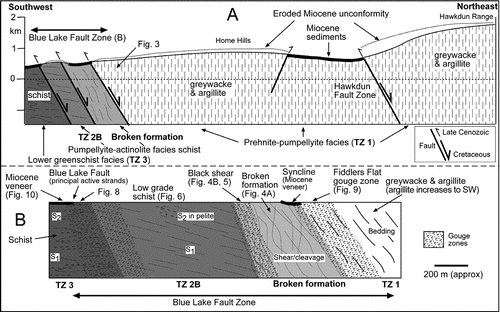
The increase in metamorphic grade on the north-eastern margin of the belt is accompanied by progressive metamorphic recrystallisation and foliation development, and this gradient has been subdivided for regional mapping purposes into textural zones (Bishop Citation1972; Turnbull et al. Citation2001). Prehnite-pumpellyite facies greywackes and associated argillites (Textural Zone 1, TZ 1) are unfoliated and sedimentary textures associated with turbiditic origins are preserved with little recrystallisation other than in the sedimentary matrix (Mackinnon Citation1983). Lowest grade schists (TZ 2A) of pumpellyite-actinolite facies have a weak foliation in greywackes, outcrops are commonly dominated by bedding and metamorphic recrystallisation is minor. More foliated low-grade schists, where foliation dominates rock fabric (TZ 2B), have more extensive metamorphic recrystallisation and sedimentary features are largely obscured. Lower greenschist facies schists (TZ 3) are almost pervasively recrystallised to metamorphic quartz, albite, muscovite, chlorite, epidote, titanite and calcite. TZ 3 schists are pervasively foliated, and locally tightly folded with incipient development of a second foliation parallel to fold axial surfaces (Turnbull et al. Citation2001; MacKenzie & Craw Citation2005)TZ 4 schists, not discussed in this paper, are more recrystallised and coarser grained upper greenschist facies metamorphic rocks (Turnbull et al. Citation2001).
The Otago Schist was exhumed in the early to middle Cretaceous as the latter stages of metamorphism gave way to extensional tectonics (Gray & Foster Citation2004; Little et al. 1992). Relative exhumation of rocks of different metamorphic grades and textural zones occurred along normal faults on the margins of the schist belt (Deckert et al. Citation2002; Gray & Foster Citation2004; Mitchell et al. Citation2009). The high-grade core of the schist belt was exhumed relative to the adjacent lower greenschist facies (TZ 3) and lower grade rocks by ca 112 Ma (Gray & Foster Citation2004; Mitchell et al. Citation2009). This was the time of initiation of the northwest-striking fault zones along the north-eastern margin of the schist belt, including the Hawkdun and Blue Lake Fault Zones (Deckert et al. Citation2002; Gray & Foster Citation2004; Mitchell et al. Citation2009).
Minor regional extension continued from late Cretaceous to middle Cenozoic, resulting in basement subsidence and marine planation (Landis et al. Citation2008). Tectonic deformation began again in Central Otago in the Late Cenozoic, related to development of the Alpine Fault (A; Cooper et al. Citation1987; Craw Citation1995). Fluvial sediments related to associated uplift were deposited on the low-relief surface and in channels cut into that surface, as the early to middle Miocene Dunstan Formation (Douglas Citation1986). The Dunstan Formation is dominated by quartz gravels, commonly with placer gold, and fluvial sands, silts, muds and lignite (Douglas Citation1986; Youngson et al. Citation1998). This formation is overlain by, and locally interfingered with, lacustrine sediments of the middle to late Miocene Bannockburn Formation, which were deposited in the regionally extensive lake complex of Lake Manuherikia (Douglas Citation1986). Late Miocene to Pliocene uplift of the Torlesse greywacke mountains along the northwest-striking faults on the northern margin of the schist belt yielded large volumes of greywacke detritus that at least partly filled Lake Manuherikia and formed extensive fan deposits: the Maniototo Conglomerate (B) in the northern part of Central Otago (Douglas Citation1986; Youngson et al. Citation1998).
The Maniototo Conglomerate, Dunstan and Bannockburn Formations, the low-relief unconformity and underlying schist basement are now being folded into broad antiforms and synforms (B, 2) that have been active since the Quaternary (Jackson et al. Citation1996; Craw et al. Citation2007). The basement ranges, uplifted sediments and intervening unconformity are currently rising on folds and faults and shedding debris to form a thin veneer of fan deposits in the intervening basins (B; Youngson et al. Citation1998; Forsyth Citation2001).
Methods
This paper is based primarily on field observations in a rock-walled gorge (20–30 m deep) of the Manuherikia River downstream of Falls Dam (B). The gorge persists through the strath terraces of Fiddlers Flat (B) and ends on the downthrown side of the Blue Lake Fault Zone where broad low-relief alluvial terraces predominate (Manuherikia flats near Loop Road; B). The near-continuous, largely unweathered outcrop along this Manuherikia river section provides an excellent basis for structural observations of four internally coherent structural blocks of basement rocks (; B) and the locally overlying Tertiary sediments. Fault zones, including soft gouge zones, are exposed in many places along this section (B). The continuity of outcrop is interrupted sporadically by minor landsliding of fault rocks and fissile schists, typically on a scale of 10 m laterally.
Table 1 Summary of principal lithological and structural features of basement rocks in the Manuherikia River section (FAS: fold axial surface).
Lithological and structural observations were made through the section, with locations and distances determined with tape measure where practical and by pacing in intervening sections. Observations were necessarily made along the winding river course, and these were then compiled into a summary section drawn perpendicular to the general northwest strike (B). Minerals and textures were examined in standard polished thin sections, with fine-grained mineral confirmation by semiquantitative electron microprobe scanning.
Fault gouges are highly comminuted and fine grained, so determination of gouge protoliths was done geochemically in comparison to unfaulted rocks within the gorge. Fresh rocks (c. 200 g) were sampled for this geochemical analysis from clean exposures. Major elements were analysed by X-ray fluorescence on fused glass disks which were manufactured by melting of rock powder and lithium metaborate flux in platinum crucibles. Analyses were carried out using a Phillips PW2400 spectrometer at the Geology Department, University of Otago. Volatile components were determined by loss on ignition after heating at 1100 °C for 1 hour. Carbonaceous (graphitic) material, which is locally abundant, may not have been completely volatilised by this method. High clay mineral contents of gouges may not have fully dehydrated, resulting in slightly low totals for some analyses. Representative analyses are presented in .
Table 2 Representative whole rock X-ray fluorescence analyses (wt%) of rocks from the Manuherikia gorge section (Turbidite block is TZ 1; BF: broken formation; LOI: loss on ignition). Sample numbers refer to samples curated by University of Otago (OU).
Supplementary data on the clay fraction in fault rocks were derived from X-ray diffraction analyses, carried out on the PANalytical X'Pert PRO MPD Diffractometer at the Department of Geology, University of Otago. The clay fraction was extracted from fault rock samples to derive a set of four samples each. One sample of each set was heated to 150 °C, one was heated to 550 °C and one sample was glycolated for 24 hours for confirmation of clay mineral identifications.
Non-carbonate carbon (or total organic carbon, TOC) and sulphur contents were determined on 3 mg powdered samples. Powders were pre-treated with hot (80 °C) HCl (10%) overnight to dissolve carbonates, then washed and dried. TOC and S data were obtained by oxidation and volatilisation by flash combustion with a Carlo Erber CHNS Elemental Analyser Detection limit is 0.1 wt% for TOC and 0.3 wt% for S. Representative results are presented in , with the associated carbonate and opaque minerals specified.
Table 3 Principal carbonate and opaque minerals in selected samples from the Manuherikia gorge section, with non-carbonate carbon (TOC) and sulphur (S) analyses (both in wt%). (BF: broken formation; DPy: diagenetic pyrite; Dcarb: detrital carbonaceous material; MGraph: metamorphic graphite; MPy: metamorphic pyrite; MPy porph: porphyroblastic metamorphic pyrite.)
Basement rock types and structures
Deformed turbidites
The turbidites that dominate the north-eastern part of the section (; A, 2B) have preserved clastic textures and sedimentary structures such as graded bedding, fine laminations, cross-bedding and rare flame structures and load casts. Most of the section has massive (c. 20 m) greywacke beds with only minor, thin-bedded (usually < 50 cm) argillite. The greywacke/argillite proportion increases downstream (southwest) and outcrops become increasingly dominated by thin-bedded (10–40 cm) alternating argillite and greywacke beds interbedded with minor, equally thin-bedded siltstone. The strike of bedding varies considerably from north to west, with moderate to steep dips (A). Sedimentary structures suggest that the sequence youngs towards the southwest, and some packets are overturned. A prominent zone of 10 m scale folds can be traced over approximately 300 m along the Manuherikia River (A, 3B) where the bedding is folded about a northwest-trending fold axis into two close chevron folds with straight limbs and narrow hinge zones. Faults with 1–20 cm brecciated zones mark the contact between 10 m scale greywacke slabs and the bedded turbidite sequence, and similar faults run subparallel to bedding in argillites in tight fold hinge zones (A, 3C).
Figure 3 Section through the folded sequence of TZ 1 turbidites near Fiddlers Flat. A, Cross-sectional view of the wall of the Manuherikia river gorge. B, Lower hemisphere, equal area stereonet of poles to bedding in the folded area in A (arrowed). Open circle is the axis of this fold, with associated great circle. C, Annotated photograph of the dashed box in A, showing complexly folded and faulted bedded turbidites adjacent to two thick greywacke slabs.
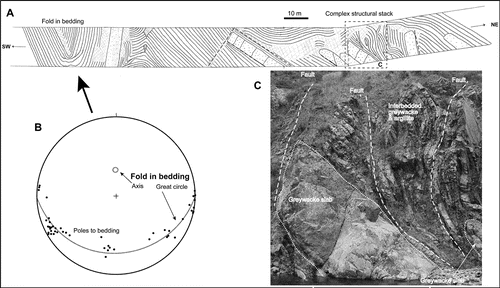
Outcrops of the adjacent structural block (B; ) consist of turbidites with block-in-matrix fabric (A). Some argillites have a weakly developed cleavage, associated with folds of greywacke in dismembered beds (B). These structures are typically called ‘broken formation’ (Nelson Citation1982; Watson & Grey Citation2001; Vannucchi & Bettelli Citation2002), and have been described in Torlesse Terrane turbidites elsewhere (Mackinnon & Howell Citation1984; Silberling et al. Citation1988). The only recognisable metamorphic fabric in most outcrops is a weak pervasive cleavage in some argillaceous rocks. Limited data suggest that bedding strikes are highly variable with moderate to steep dips (A).
Figure 4 Broken formation in the Manuherikia gorge section. A, Sketch made from an oblique photograph, showing the characteristic block-in-matrix fabric that dominates this structural block. Kaolinitised basement rock underlies the Miocene Dunstan Formation at the top of the outcrop. Stereonet inset shows poles to bedding. B, Conglomerate boudin (pale, top) with underlying matrix of sheared argillite (Sh. arg). Matrix contains small boudins of less-sheared argillite (Arg. boudin; margins shown with dashed white lines). Inset stereonet shows poles to argillite shears in the black shear zone of the broken formation.
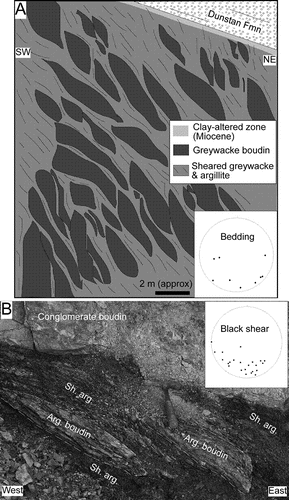
The south-western part of the broken formation block contains abundant sheared argillite with additional metamorphic recrystallisation and shearing, yielding a distinctive black shear zone (B). Two subparallel and intersecting shear sets strike westwards across argillaceous broken formation (B), disrupting the block-in-matrix fabric. The shear fractures are in-filled with black semi-cohesive foliated gouge which also envelopes phacoidal clasts of greywacke and conglomerate with a thin, often sheared and slickensided, veneer. The veneer consists of graphite, muscovite, chlorite, rutile and quartz (optical, electron microprobe and X-ray diffraction confirmation). These metamorphic minerals have been formed and/or recrystallised during deformation to give a complex fabric on boudin margins (). The gouge displays a clear foliation defined by muscovite, chlorite, pervasive foliation-parallel quartz veins and occasional foliation-parallel graphite smears (). Crosscutting quartz veins are also common within this fabric. Total organic carbon (TOC) analyses of this boudin veneer material range from 1.3 to 5.8 wt% ().
Figure 5 Photomicrograph (transmitted light) of the sheared margin of a greywacke boudin in the black shear zone within the broken formation block. Greywacke is at the base, and material added and recrystallised during shearing is at the top. Black material is variably sheared rock with metamorphic graphite, rutile, muscovite and chlorite. White material is quartz (Q) and/or coarse-grained metamorphic muscovite (M). The metamorphic muscovite, chlorite and graphite impart a shear foliation (horizontal) to the rock. This is cut by late-stage quartz veins.
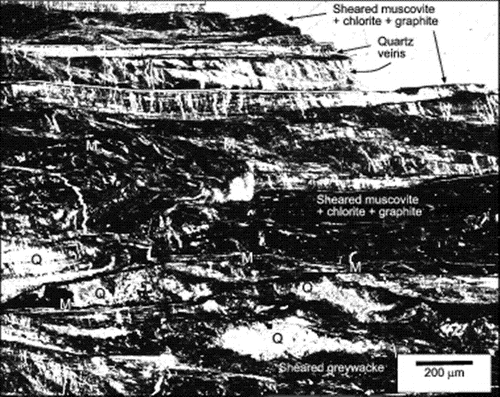
Schist
The low-grade schist structural block (B; ) consists of psammitic and pelitic schists distinguished primarily by the differing proportions of micaceous (muscovite, chlorite) and quartzofeldspathic components (A, 6B). Some pelitic schists are micaceous but also have abundant quartzofeldspathic laminae (A, 6D). The metamorphic fabric has been locally enhanced and disrupted by the development of foliation-parallel metamorphic quartz veins (B). The combination of structural transposition and quartz vein emplacement has resulted in prominent colour-banding in many outcrops, resembling metamorphic segregation (A, 6B, 6D). In addition, numerous post-metamorphic quartz veins cut the foliation, especially in psammitic layers (B). The S1 foliation generally dips gently-to-moderately northwards (C).
Figure 6 Low-grade schist (TZ 2B) outcrops in the Manuherikia gorge section. A, Laminated TZ 2B schist with alternating quartzofeldspathic and micaceous horizons, showing fine-scale transposition of bedding into parallelism with foliation (S1). B, Massive psammitic schist with metamorphic and post-metamorphic quartz veins. C, Stereonet with poles to TZ 2B foliation. D, Post-metamorphic shear zone in a micaceous pelitic schist layer sandwiched between less-deformed psammitic schist (top) and pelitic schist with abundant quartzofeldspathic laminae (bottom).
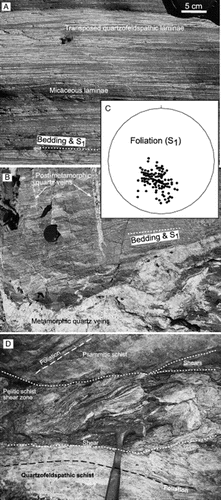
A set of low-grade schist samples (TZ 2B) was analysed for geochemical comparison to turbidites further upstream (). Both sets of analyses show very similar geochemical trends, reflecting the same general lithological variations between greywacke and argillite (A–7D). Rocks with greywacke protoliths have high silica contents (70–80 wt%), and more argillaceous protoliths have progressively lower silica and higher MgO (increasing chlorite) and higher K2O (increasing muscovite) (A, 7B). The most micaceous TZ 2B pelitic schists have higher K2O and lower silica than typical argillites (B). Many of these pelitic schists are prominently enriched in graphite and pyrite and TOC contents () range up to 5.5 wt% and sulphur contents range up to 11.5 wt% for these micaceous schists. This is distinctly elevated relative to background values which were below the detection limit (< 0.3 wt%). Graphite occurs within the metamorphic foliation (S1), and rarely in quartz veins. Pyrite occurs disseminated within the matrix and in syn- to post-metamorphic quartz veins. The elevated pyrite contents of these micaceous schists are also reflected in the high iron contents of the rocks compared to the typical greywacke-argillite range (C). The relatively high amounts of pyrite and graphite in the TZ 2B micaceous schists also results in elevated loss on ignition (C).
Figure 7 Geochemical plots showing the ranges of compositions defined by the primary sedimentary variation between greywacke and argillite in TZ 1 turbidites (black squares). Low-grade schists (TZ 2B; small grey circles) show a similar range, although more extreme pelitic compositions occur. Gouge samples (large open circles) are all derived from argillite. A, Silica–MgO variations. B, Silica–K2O variations. C, Silica–total iron variations. D, Alumina–loss on ignition variations.
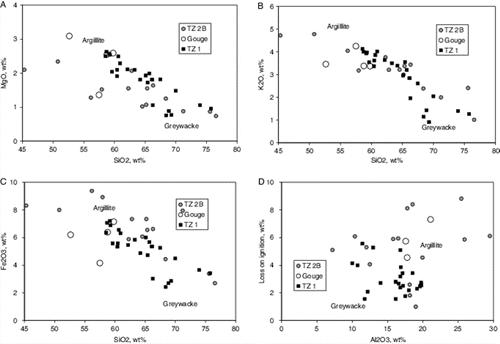
Medium grade schist (TZ 3; ) is exposed at the southwest of the Manuherikia gorge section (B), where the gorge ends at the Manuherikia flats (B). Consequently, outcrop is relatively poor compared to the upstream section. The pervasive foliation (S1) has been extensively deformed on the decimetre to metre scale by recumbent tight to isoclinal folds with shallow-plunging westward trends (A). An S2 fabric is particularly strongly developed on attenuated fold limbs, where it locally dominates the fabric at outcrop (A). Both S1 and S2have metamorphic quartz veins parallel to them (A), and the folded S1 quartz veins are strongly lineated where they have been folded. The S2 fabric is generally flat-lying or dips gently (<20°) to the north or south (A).
Figure 8 Structure of greenschist facies schist (TZ 3) outcrop at Manuherikia flats. A, Foliation S1 is folded, and an incipient fold axial surface foliation (S2) dominates the fabric. Both foliations are accentuated by millimetre-scale quartz veins. Inset stereonet shows orientations of fold axes (squares) and poles to S2 foliation. B, Strongly brecciated and gouge-filled zones cut the outcrop between more intact schist blocks. Location of intact schist in A is indicated with a white box. Inset stereonet shows poles to gouge zones in the boundary between TZ 3 and TZ 2B schists.
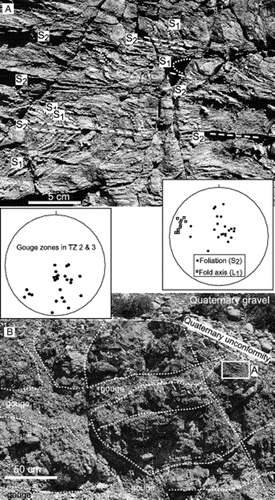
Gouge zones
Fiddlers Flat gouge zone
This is the most prominent and well-exposed gouge zone in the Manuherikia gorge section, and extends over c. 200 m at the boundary between TZ 1 turbidites and the broken formation block (B). The gouges are best developed in the TZ 1 rocks, and exposures of gouges on the broken formation side of the boundary are poor. The gouge zone becomes progressively developed downstream (southwest) in TZ 1 rocks. Isolated bedding-parallel shears and small gouge zones (< 5 m wide) are focused in argillite beds that are locally tectonically thickened (up to 2 m). Apparently randomly oriented small-scale (1–3 m) faults crosscut the bedding with small offsets (< 2 cm) with a normal sense of offset. Fault rocks include fine-grained gouges, coarser grained cataclasites and breccias with clasts up to 5 cm in diameter, and are commonly cemented with calcite and/or ankerite.
In the main gouge zone (A), the fault rocks consist of variably brecciated greywacke and sheared argillite embedded in grey and black incohesive, fine-grained, clay-rich gouge. Gouge bodies up to 2 m across are partially cemented with calcite. The primary mineralogy of black gouge is quartz, muscovite, chlorite, smectite, kaolinite and minor albite (X-ray diffraction identification). Major element data () suggest that the gouge is derived from argillites (A–7D).
Figure 9 Structures within the Fiddlers Flat gouge zone between TZ 1 turbidites and the broken formation block. A, Sketch section through the gouge zone, showing orientations of gouge fabric (dashed lines) and faults (heavy black lines, with inferred sense of motion). B, Sheared argillite (dark grey) and less-deformed greywacke (pale) in a normal fault zone near the south-western edge of the gouge zone (located in A). Both argillite and greywacke are locally coated by calcite (white). C, Zone of complexly folded black and grey gouge (derived from argillite) with abundant secondary calcite impregnation, near centre of gouge zone (located in A). White dashed lines accentuate gouge fabric that has been folded. Southwest-dipping later shears (full white lines) displace sheared argillaceous gouge. One of these later shears has a demonstrable reverse sense of motion (heavy dashed arrow).
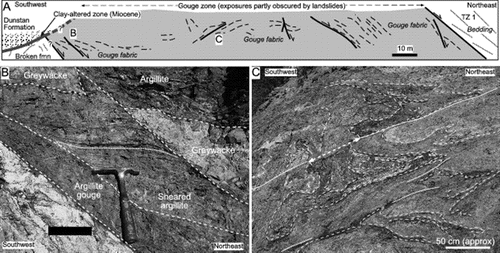
The northeast margin of the gouge zone dips moderately northeast, and bedding within the turbidites has been rotated into approximate parallelism with this margin (A). Sheared rocks, particularly black argillaceous material, define a gouge fabric within the gouge zone, and this fabric has a wide range of orientations (A–9C). Cemented and uncemented gouge is locally deformed into complex folds defined by crushed rock of different shades of black and dark grey (C). The gouge fabric is generally parallel or subparallel to adjacent fault strands that cut through the gouge zone (A). Bending of relict bedding and gouge fabric immediate adjacent to these subsidiary faults is consistent with a normal sense of motion within the gouge zone (A, 9B).
The folded gouge is crosscut and offset by a second generation of narrow southwest-dipping shears (C). The shears consist of thin (< 5 cm) seams of black, fine-grained, incohesive gouge. Displacement along the shears is typically less than a metre, with a reverse sense (C).
Other gouge zones
The boundaries between broken formation and TZ 2B rocks and between TZ 2B and TZ 3 rocks are both defined by gouge zones, but the exposure of these gouge zones is poorer than that at Fiddlers Flat. Deformation associated with these gouge zones appears up to 200 m from the main zones, and is typically focused in weaker rocks in narrow (< 1 m) zones. These zones generally become progressively more common towards the main gouge zones.
Broken formation rocks are juxtaposed against textural zone 2B lithologies, with an abrupt change from broken formation to undisturbed textural zone 2B semischist within 50 m along the Fiddlers Flat section. A distinct fault trace was not observed, but the boundary coincides with the synmetamorphic black shear zone in the broken formation block. Parts of the black shear zone have been redeformed to form steeply dipping zones (metre scale) of grey, incoherent cataclastic rock which is made up of clasts of the foliated gouge. The gouge and clasts are commonly cemented with ankerite, and some cemented zones have been redeformed to yield ankeritic fragments (1–10 cm scale) within the gouge zones. No gouge involving TZ 2B rocks was observed, although micaceous schist layers are locally sheared and disrupted.
The boundary between TZ 2B and TZ 3 schists occurs at the end of the Manuherikia gorge, where outcrop is relatively poor. This boundary is better exposed at Pennyweight Hill, 4 km along strike to the southeast (B), where the bounding gouge zone dips steeply northeast (C). Gouge zones occur over 300–400 m near to this boundary in the Manuherikia gorge (B). Narrow deformation zones in TZ 2B first appear at least 300 m upstream of the boundary between the textural zones, and these are typically confined to micaceous rocks (D). These narrow zones are spaced at 2–10 m intervals and have a wide range of orientations, partly controlled by the foliation orientation which is generally shallow-dipping (c. 30°). However, some of these narrow gouge zones dip steeply northeast with a west to north-westerly strike. The river at the downstream end of the gorge follows one of the prominent west to northwest striking gouge zones for >50 m within TZ 2B rocks, with a 5–20 cm thick shear plane which juxtaposes semi-pelitic against psammitic schist. Bending of foliation and gouge fabric at minor faults within these gouge zones suggest a normal sense of displacement. However, rare thin (centimetre scale) shears cutting through the gouge zones appear to have a reverse sense of motion, based on bending of gouge fabric.
Most outcrops of TZ 3 schists on the Manuherikia flats downstream of the gorge have some degree of brecciation and gouge formation cutting across the flat-lying S2 foliation (B). The gouge zones have a wide range of orientations, generally with a westerly strike and dips ranging from steep to gentle (B). These gouge zones break the outcrops up into relatively intact blocks of schist (0.5–10 m scale), separated by breccia and gouge zones that are also typically 0.5–10 m across (B). Additional narrow gouge zones (centimetre scale) cut across these larger gouge zones (B).
Gouges in TZ 2B and 3 rocks consist of quartz, muscovite, chlorite, smectite, kaolinite and little or no albite. Many gouges show a very weak incipient shear fabric parallel to the strike and dip of the individual gouge zones. The fabric of the matrix anastomoses around clasts ranging in size from 20 µm to 4 cm with a weak preferred orientation. Clasts comprise single crystals to remnant rock fragments. Clasts in TZ 3 gouge are generally more rounded to phacoidal shaped than in textural zone 2B, at least partly because of the greater abundance of metamorphic quartz veins in TZ 3 schists (A). Compositions of gouges in TZ 2B and TZ 3 are similar to the Fiddlers Flat gouge (), and are apparently derived from argillaceous rocks (A–7D). Most TZ 3 gouge is pervasively cemented with ankerite, and ankerite veins extend into nearby fractured host rocks.
Tertiary sediments
Miocene sediments of the Manuherikia Group (Douglas Citation1986; Youngson et al. Citation1998) rest unconformably on some of the rocks of the Blue Lake Fault Zone. Rocks beneath the unconformity are variably altered to clays for up to 20 m below the sediments. The altered rocks and their veneer of sediments, where preserved, provide a useful marker for post-Miocene deformation of the area (A). Miocene sediments and the underlying unconformity are preserved in two places in the Manuherikia gorge: at Fiddlers Flat and on the Manuherikia flats downstream of the Manuherikia gorge (B). Exposures of similar geological features to the latter locality are visible 4 km along-strike at Pennyweight Hill (C).
At Fiddlers Flat, quartz pebble conglomerate, sand and silt of the basal Dunstan Formation overlie the clay-altered unconformity on the broken formation (A, 4A). The sediments are preserved as a shallow syncline whose south-western limb dips c. 25° northeast (A) towards the Fiddlers Flat gouge zone (B). Sediments and clay-altered unconformity are preserved on deformed broken formation gouge (A), but have been largely eroded and/or offset above the TZ 1 part of the gouge zone.
At the Manuherikia flats, the Dunstan Formation was juxtaposed against TZ 3 schist by a strand of the Blue Lake Fault that was active during Miocene sedimentation (D; Lindqvist Citation1994). The plane of this fault strand is not well exposed, but variably sheared and tilted Dunstan Formation sandstones and quartz pebble conglomerates define the downthrown side. On the upthrown side of this fault, the Miocene unconformity dips southwest at 10–20° and is overlain by boulders of TZ 3 schist and also silcrete eroded from the pre-existing Dunstan Formation (Lindqvist Citation1994). Bannockburn Formation lacustrine mudstone fills interstices between boulders, in a layer up to 50 cm thick (D, 10A). Miocene stromatolites have nucleated on many of the cobbles (5–20 cm) of TZ 3 schist and silcrete. Some stromatolites fully coat their core cobble but most have only encrusted three sides, leaving an uncoated portion. The uncoated portions of the stromatolites face in a wide variety of directions, including directly upwards (A, 10B). TZ 3 schist cobbles within stromatolites are variably kaolinitised, similar to the underlying basement.
Figure 10 Miocene unconformity on TZ 3 schist at Manuherikia flats. A, Outcrop photo showing the Bannockburn Formation resting unconformably on kaolinitised schist. Mudstone (M) fills interstices between stromatolites (S). B, Close-up view of stromatolites and mudstone in black box in A. Arrows point in the direction of stromatolite encrustation on schist cobbles. C, Cartoons (not strictly to scale) of a northeast to southwest section across the Blue Lake Fault at the outcrop in A show possible Miocene evolution of the site. In the first panel, stromatolites are in life position on a fault scarp with encrustations growing upwards. The second panel shows dead stromatolites redeposited after continued fault movement.
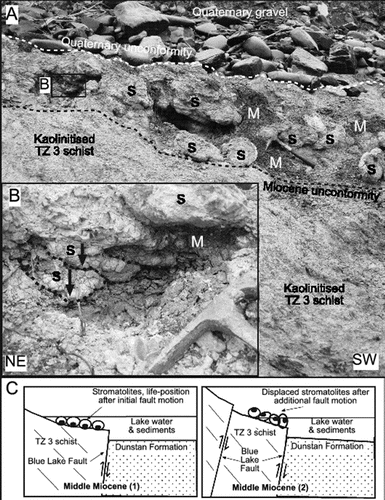
Discussion
Polyphase deformation in the Blue Lake Fault Zone
Rocks in the Blue Lake Fault Zone show evidence for numerous generations of deformation, each with characteristic features. These characteristic features allow for subdivision of the deformation into three main stages in the structural evolution of the area: metamorphic deformation, wide gouge zone deformation and deformation affecting the Miocene unconformity. Not all deformation features within the fault zone can be assigned easily to one of these stages, but the overall development of the fault zone becomes tractable with this simplification. These three stages are summarised in A–11C, with chronological order of deformation stages depicted from bottom to top of the diagram.
Figure 11 Summary of the structural evolution of the Otago Schist margin at the Manuherikia gorge section. A, Present active structures. B, Cretaceous structures. C, Original metamorphic transition in C. The thick metamorphic section C became extremely condensed by normal faults to the present narrow section in B, which was reactivated as in A.
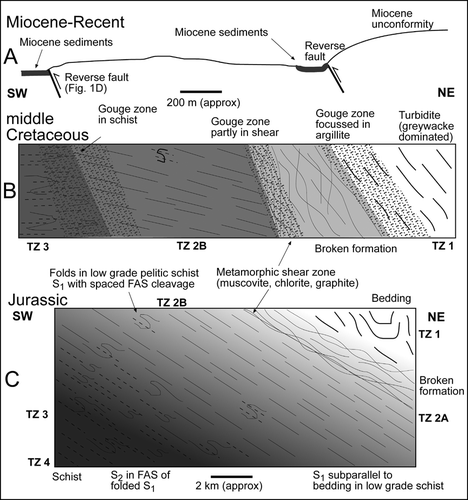
Deformation that accompanied or immediately post-dated metamorphism is different for each of the different structural blocks defined in the section (; B). This deformation is of a regional nature and therefore unrelated to the development of the Blue Lake Fault Zone, but is discussed here in order to distinguish it from structures related to the Blue Lake Fault Zone. In TZ 1, synmetamorphic deformation involved folding of bedding, with localised development of brittle faults and sheared zones (A–3C). Fault zones are typically dominated by brittle lithic breccias, rather than soft gouges. The main observable large-scale fold in the TZ 1 turbidites (A, 3B) is steeply north-plunging, and is geometrically and rheologically unrelated to the large northwest-striking gouge zones further to the southwest in the section (B).
In TZ 2B and TZ 3, development of foliation(s) was a regional scale effect of metamorphic deformation under ductile deformation conditions. This ductile deformation occurred at considerable depth, ranging up to greenschist facies conditions of c. 350°C and>15 km depth (C; Mortimer Citation2000). The broken formation formed under metamorphic conditions intermediately between TZ 1 and TZ 2B & 3, and involved minor metamorphic cleavage development and abundant shearing and brecciation. The black shear within the broken formation block shows the extreme extent of the broken formation style of deformation, with formation of foliated gouge and localised metamorphic foliation on boudin margins (). This metamorphic foliation involved crystallisation and recrystallisation of muscovite, chlorite and graphite () to a much greater extent than elsewhere in the broken formation zone.
The above-described metamorphism and metamorphic deformation prepared the host rocks for development of post-metamorphic structures that make up the Blue Lake Fault Zone. The Late Cenozoic component of this deformation was small, as the Miocene unconformity is largely preserved over the general area (A). The Blue Lake Fault Zone is dominated by gouge zones that were formed before the Miocene unconformity developed, as outlined in the next section.
Cretaceous extension and the condensed metamorphic section
The gouge zones that make up about 30% of the 2 km section from greywacke to schist (B) represent major fault movement that resulted in juxtaposition of the different structural blocks. In contrast, each structural block is internally broadly homogeneous. The structural and metamorphic transition from TZ 1 to TZ 3 that presumably existed in some form (e.g. C) has been extensively disrupted and tectonically thinned to c. 15% of its original width (B). Textural zone 2A rocks, abundant elsewhere on the northeast schist margin (Bishop Citation1972; Forsyth Citation2001), have apparently been completely excised from the Manuherikia gorge section. This requires kilometre-scale relative movement on the faults represented by the gouge zones. Similar fault disruptions of metamorphic gradations are common throughout the Otago Schist (Craw Citation1998; Mortimer Citation2000), but the Blue Lake Fault Zone is the most thinned and disrupted such section in Otago.
The gouge zones have a broad northwest strike and moderate to steep northeast-wards dip (A, 2B, 9A). The northeast side (footwall) of each of the zones is relatively lower grade and therefore has been relatively downthrown. Hence, these gouge zones primarily represent normal faults. Evidence for normal sense of motion is common in the gouge zones (e.g. A, 9B), although the gouge zones are so internally complex and chaotic (e.g. B, 9C) that obtaining consistent motion senses from outcrops is difficult. The gouges are soft and incohesive and contain clay minerals (kaolinite and smectite) that have altered from metamorphic minerals: mainly muscovite, chlorite and albite. Hence, the gouges are clearly post-metamorphic in origin and have formed at shallower crustal levels than any of the host metamorphic rocks.
On a regional scale, the most prominent tectonic event in the Otago Schist that involved post-metamorphic normal faults with kilometre-scale offset occurred in the middle Cretaceous (Deckert et al. Citation2002; Gray & Foster Citation2004; Mitchell et al. Citation2009). This event resulted in exhumation of the core of the schist belt via normal faults on the margin of the belt, and was active on the northeast side of the belt c. 112 Ma (Gray & Foster Citation2004; Mitchell et al. Citation2009; Tulloch et al. Citation2010). The major deformation in the Blue Lake Fault Zone, which formed the gouge zones, was probably part of that regional event. Middle Cretaceous sediments associated with these normal faults are locally preserved on the schist margin, including a thick pile (Kyeburn Formation) c. 20 km east of the Manuherikia section (Bishop & Laird Citation1976; Mitchell et al. Citation2009). Normal fault movement persisted in Central Otago to at least 96 Ma (Barker et al. Citation2010).
Late Cenozoic fault reactivation
Deformation of the Miocene unconformity has been minor and highly localised. Offset on faults has been on the 1–100 m scale, and the results of this deformation are largely visible as the present relief of the area surrounding the Manuherikia gorge (A, 11A). The gorge itself has been cut because of the ongoing uplift on the south-easternmost strands of the Blue Lake Fault (C, 1D, 11A) that define the boundary between the schist gorge and the sediment-coated Manuherikia flats immediately downstream (B, 2B).
The faults responsible for the offset of the Miocene unconformity are narrow (centimetre to metre scale) and are hosted in the wide gouge zones of the pre-existing normal faults. Hence, the Miocene–Recent component of movement on the Blue Lake Fault Zone is localised reactivation of pre-existing gouge zones. The contrast between these two different types of fault zones can be seen in C, where centimetre-scale shears with reverse motion (Miocene–Recent) cut across the wide, multiply-deformed Cretaceous gouge zone. Cenozoic reactivation has occurred in this Fiddlers Flat gouge zone to the extent that the syncline in Miocene sediments has been truncated on its north-eastern limb and a greywacke hill (>50 m high) has been uplifted on that side (A). Reactivation in the gouge zone between TZ 2B and TZ 3 at the edge of the Manuherikia flats has resulted in only minor offset (<10 m) of the Miocene unconformity (D, 11A).
There is no preserved evidence for post-Miocene reactivation in other gouge zones in the Manuherikia gorge, but late-stage centimetre-thick shears are common in these gouges and some appear to have reverse sense of motion. However, Mackie et al. (Citation2009) report Cretaceous compressional structures in the schist of North Rough Ridge (B), so it is not possible to link reverse-fault reactivation features to Miocene–Recent deformation without observing an offset of Miocene features. The Miocene unconformity appears to have been warped over the Manuherikia gorge between the two known reactivated fault strands (A). It is possible that post-Miocene folding of the schist basement and overlying unconformity has occurred between these fault strands, similar to that which is occurring in the antiformal schist ranges such as Blackstone Hill and North Rough Ridge to the south (B; Jackson et al. Citation1996). Small-scale reactivation steps in gouge zones throughout the gorge may have facilitated this broad folding. However, warping of the Miocene unconformity near the Blue Lake Fault Zone has a northwest trend, rather than the northeast trend of antiformal ranges to the south (B).
The Miocene reactivation of normal faults was significant for the stromatolites located at the contact of TZ 3 schist and Miocene sediments (D, 10A, 10B). The stromatolites owe their existence to the formation of a favourable habitat on the Miocene lake margin by the initial development of a small (metre-scale) bedrock scarp by fault reactivation in TZ 3 schist (Lindqvist Citation1994). Their photosynthetic growth at that time presumably resulted in encrustations on the tops and sides of cobbles (C, panel 1). Their current positions are therefore not life positions (A, 10B), and some form of disruption has occurred at this site. The stromatolites have interstitial mudstone of the Bannockburn Formation, so the disruption must have occurred during Miocene deposition of that sediment. A possible disruption event, related to ongoing fault reactivation, is depicted in C (panel 2). With increasing tectonic relief on the lake margin, the stromatolites were uplifted relative to the water level of the lake, which would have led to the demise of the colony. The dead stromatolites were probably redeposited by mass flow or surficial creep on the steepening slope.
Lithological controls on deformation
Throughout the structural evolution of the Manuherikia gorge section, there has been strong lithological control on deformation style. In particular, argillites and pelitic schists derived from argillites have been important for localising faults, starting under metamorphic conditions. Greywacke and psammitic schists have formed more resistant blocks of rock at a range of scales, around which the argillites have deformed and thickened (C). This effect is most pronounced in the broken formation block, where strain during metamorphism was so strongly focused into argillites (B) that greywacke and conglomerate boudins did not develop any cleavage. Focusing of synmetamorphic deformation into argillite reached an extreme in the broken formation with development of the black shear zones (B, 5), and this may have been facilitated and accentuated by simultaneous formation and recrystallisation of micaceous minerals and graphite.
Argillites have also controlled the location and development of the Cretaceous gouge zones, the most important faults in this section. Geochemical analyses show that gouge is derived from argillaceous material (A–7D). The Fiddlers Flat gouge zone occurs mainly in TZ 1 turbidites where argillite is relatively common, rather than in a greywacke-dominated part of the section (A, 11B). Likewise, the gouge zone between TZ 2B and TZ 3 is preferentially concentrated in pelitic schists on the TZ 2B side of the fault (C). The TZ 3 schists are so fissile that this distinction becomes less significant in schists of that metamorphic grade (B).
Once deformation zones developed in argillites and pelitic schists, later reactivation was controlled by these zones of weakened rocks. The synmetamorphic black shear in the broken formation helped to localise the post-metamorphic fault which juxtaposes the broken formation against TZ 2B rocks (B, 11C). The effects of this reactivation are observable over at least 100 m in the argillaceous black shear in the broken formation, but there is little evidence for deformation along this fault boundary in the psammitic schists of TZ 2B in the hanging wall. Likewise, Miocene–Recent reverse faulting has been focused into argillaceous gouge zones of the Cretaceous normal faults (A, 11B). While the regional-scale control on the location of the Blue Lake Fault Zone was the major crustal boundary between Torlesse greywacke and Otago Schist (A; Upton et al. Citation2009), on a more local scale it was the argillites and pelitic schists in the rock sequence that controlled the location (and subsequent reactivation) of specific fault strands.
Conclusions
Three major northwest-striking fault strands of the Blue Lake Fault Zone are well exposed in a gorge of the Manuherikia River in Central Otago. These faults juxtapose slices of basement rocks of increasing metamorphic grade, from largely unmetamorphosed Torlesse turbidites to greenschist facies schist. The turbidite slice (prehnite-pumpellyite facies; TZ 1) consists mainly of well-bedded greywacke with minor argillite. The proportion of argillite increases up-section, and these argillaceous rocks have controlled formation of a fault with a prominent gouge zone (200 m wide) at Fiddlers Flat. This fault juxtaposes the turbidite sequence against broken formation (probable pumpellyite-actinolite facies).
The broken formation consists of uncleaved greywacke and conglomerate boudins (1–20 m scale) in a matrix dominated by sheared and weakly cleaved argillite. More extreme shearing in this broken formation has yielded a black argillaceous shear zone with a fabric containing recrystallised muscovite, chlorite and graphite. This black shear zone has, in turn, localised a later set of clay-bearing gouges constituting a fault zone that juxtaposed the broken formation against low-grade schists (pumpellyite-actinolite facies, TZ 2B). These schists are dominated by a pervasive foliation that is subparallel to transposed bedding. More pelitic schists within this slice are locally folded with development of a spaced axial surface cleavage. Some of these pelitic schists contain more abundant metamorphic pyrite and graphite than argillites at lower grade. However, the TZ 2B schists are generally geochemically similar to the low-grade turbidites. TZ 2B psammitic schists show few effects of the fault juxtaposition to the broken formation block. However, pelitic schists at the other side of the TZ 2B structural block have controlled development of a wide (300 m) gouge zone where these schists were juxtaposed against micaceous greenschist facies schists (TZ 3). The TZ 3 schists are also extensively deformed, with abundant gouge zones subparallel to and crosscutting the pervasive foliation.
The three main gouge zones make up about 30% of the narrow (2 km wide) structural transition from low- to high-grade rocks. This structural section has been strongly thinned to c. 15% of its original metamorphic width by normal faulting, and is now the narrowest such section on the Otago Schist margin. These normal faults were initiated during middle Cretaceous tectonic unroofing of the Otago Schist. The resultant Blue Lake Fault Zone is part of the surface manifestation of a major crustal boundary between Otago Schist and Torlesse greywacke that formed in the Cretaceous.
The Blue Lake Fault Zone was reactivated in the Miocene, in narrow (centimetre- to metre-scale) faults with metre-scale reverse offsets. These offsets locally affected the deposition of fluvial and lacustrine sediments of the Manuherikia Group, and facilitated growth of stromatolites on a palaeo-lake shore at a fault scarp in basement schists. Ongoing deformation caused stromatolite death and redeposition in this setting. Miocene–Recent deformation has caused, and continues to cause, minor folding and faulting (1–100 m scale) of the basement topography and overlying Miocene sediments.
Acknowledgements
Funding for this research was provided by the Foundation for Research, Science and Technology with additional support from the University of Otago. This paper has benefited from discussions with Daphne Lee and Alan Cooper. Thanks are due to Damian Walls and Brent Pooley for laboratory assistance and to Tim Blackler, James McCarthy and Zoe Reid Lindroos for field assistance. Two journal referees provided constructive reviews that substantially improved the presentation of the paper.
References
- Barker , SLL , Sibson , RH , Palin , JM , FitzGerald , JD , Reddy , S , Warr , LN and van der Pluijm , BA . 2010 . Cretaceous age, composition, and microstructure of pseudotachylyte in the Otago Schist, New Zealand . New Zealand Journal of Geology and Geophysics , 53 : 15 – 29 .
- Bishop , DG . 1972 . Progressive metamorphism from prehnite–pumpellyite to greenschist facies in the Dansey Pass area, Otago, New Zealand . Geological Society of America Bulletin , 83 : 3177 – 3198 .
- Bishop , DG and Laird , MG . 1976 . Stratigraphy and environment of deposition of the Kyeburn Formation (Cretaceous), a wedge of coarse terrestrial sediments in Central Otago . Journal of the Royal Society of New Zealand , 6 : 55 – 71 .
- Cooper , AF , Barreiro , BA , Kimbrough , DL and Mattinson , JM . 1987 . Lamprophyre dike intrusion and the age of the Alpine fault, New Zealand . Geology , 15 : 941 – 944 .
- Craw , D . 1995 . Reinterpretation of the erosion profile across the southern portion of the Southern Alps, Mt Aspiring area, Otago, New Zealand . New Zealand Journal of Geology and Geophysics , 38 : 501 – 507 .
- Craw , D . 1998 . Structural boundaries and biotite and garnet ‘isograds’ in the Otago and Alpine Schists, New Zealand . Journal of Metamorphic Geology , 16 : 395 – 402 .
- Craw , D , Burridge , C and Waters , J . 2007 . Geological and biological evidence for drainage reorientation during uplift of alluvial basins, central Otago, New Zealand . New Zealand Journal of Geology and Geophysics , 50 : 367 – 376 .
- Deckert , H , Ring , U and Mortimer , N . 2002 . Tectonic significance of Cretaceous bivergent extensional shear zones in the Torlesse accretionary wedge, central Otago Schist, New Zealand . New Zealand Journal of Geology and Geophysics , 45 : 537 – 547 .
- Douglas BJ 1986. Lignite resources of Central Otago. N.Z. Energy Research and Development Committee, University of Auckland, Auckland, New Zealand, Publication P104: 368 pp.
- Forsyth PJ 2001. Geology of the Waitaki area. Institute of Geological and Nuclear Sciences 1:250 000 Geological Map 19. 1 sheet + 64 p.
- Gray , DR and Foster , DA . 2004 . 40Ar/39Ar thermochronologic constraints on deformation, metamorphism and cooling/exhumation of a Mesozoic accretionary wedge, Otago Schist, New Zealand . Tectonophysics , 385 : 181 – 210 .
- Jackson , J , Norris , RJ and Youngson , JH . 1996 . The structural evolution of active fault and fold systems in Central Otago, New Zealand: evidence revealed by drainage patterns . Journal of Structural Geology , 18 : 217 – 234 .
- Landis , CA , Campbell , HJ , Begg , JG , Mildenhall , DC , Paterson , AM and Trewick , SA . 2008 . The Waipounamu Erosion Surface: questioning the antiquity of New Zealand land surface and terrestrial fauna and flora . Geological Magazine , 145 : 173 – 197 .
- Lindqvist JK 1994. Lacustrine stromatolites and oncoids: Manuherikia Group (Miocene), New Zealand. In: Bertrand–Safari J, Monty C eds. Phanerozoic Stromatolites 11. Kluwer Academic Publishers. Pp. 227–254.
- Little , T , Mortimer , N and McWilliams , M . 1999 . An episodic Cretaceous cooling model for the Otago–Marlborough Schist, New Zealand, based on 40Ar/39Ar white mica ages . New Zealand Journal of Geology and Geophysics , 42 : 305 – 325 .
- MacKenzie , DJ and Craw , D . 2005 . Structural and lithological continuity and discontinuity in the Otago Schist, Central Otago, New Zealand . New Zealand Journal of Geology and Geophysics , 48 : 279 – 293 .
- Mackie , C , MacKenzie , DJ and Craw , D . 2009 . Structural and lithological controls on gold mineralization at Oturehua on the northeastern margin of the Otago Schist, New Zealand . New Zealand Journal of Geology and Geophysics , 52 : 43 – 47 .
- Mackinnon , TC . 1983 . Origin of Torlesse and related rocks, South Island, New Zealand . Geological Society of America Bulletin , 94 : 967 – 985 .
- Mackinnon , TC and Howell , DG. 1984 . Turbidite facies in an ancient subduction complex: Torlesse Terrane, New Zealand . Geo-Marine Letters , 3 : 211 – 216 .
- Mitchell , M , Craw , D , Landis , CA and Frew , R . 2009 . Stratigraphy, provenance, and diagenesis of the Cretaceous Horse Range Formation, east Otago, New Zealand . New Zealand Journal of Geology and Geophysics , 52 : 171 – 183 .
- Mortimer , N . 1993 . Jurassic tectonic history of the Otago Schist, New Zealand . Tectonics , 12 : 237 – 244 .
- Mortimer , N . 2000 . Metamorphic discontinuities in orogenic belts: example of the garnet–biotite–albite zone in the Otago Schist, New Zealand . International Journal of Earth Sciences , 89 : 295 – 306 .
- Nelson , D . 1982 . A suggestion for the origin of mesoscopic fabric in accretionary melange, based on features observed in the Crystalls Beach Complex, South Island, New Zealand . Geological Society of America Bulletin , 93 : 625 – 634 .
- Silberling , NJ , Nichols , KM , Bradshaw , JD and Blome , CD . 1988 . Limestone and chert in tectonic blocks from the Esk Head subterrane, South Island, New Zealand . Geological Society of America Bulletin , 100 : 1213 – 1223 .
- Tulloch AJ , Ramezani J , Mortimer N , Mortensen J , van den Bogaard P , Maas R 2010 . Cretaceous felsic volcanism in New Zealand and Lord Howe Rise (Zealandia) as a precursor to final Gondwana breakup . Geological Society of London Special Paper 321 : 89 – 118 .
- Turnbull , IM , Mortimer , N and Craw , D . 2001 . Textural zones in the Haast Schist – a reappraisal . New Zealand Journal of Geology and Geophysics , 44 : 171 – 183 .
- Upton P , Koons PO , Craw D , Henderson CM , Enlow R 2009 . Along-strike differences in the Southern Alps of New Zealand: Consequences of inherited variation in rheology . Tectonics 28: TC2007 . doi: DOI:10.1029/2008TC002353
- Vannucchi , P and Bettelli , G . 2002 . Mechanisms of subduction accretion as implied from the broken formations in the Apennines, Italy . Geology , 30 : 835 – 838 .
- Watson , JM and Grey , DR . 2001 . Character, extent and significance of broken formation for the Tabberabbera Zone, central Lachlan Orogen . Australian Journal of Earth Sciences , 48 : 943 – 954 .
- Worthy , TH , Tennyson , AJD , Archer , M , Musser , AM , Hand , SJ , Jones , C , Douglas , BJ , McNamara , JA and Beck , RMD . 2006 . Miocene mammal reveals a Mesozoic ghost lineage on insular New Zealand, southwest Pacific . Proceedings of the National Academy of Sciences , 103 : 19419 – 19423 .
- Youngson , JH , Craw , D , Landis , CA and Schmitt , KR . 1998 . Redefinition and interpretation of late Miocene–Pleistocene terrestrial stratigraphy, Central Otago, New Zealand . New Zealand Journal of Geology and Geophysics , 41 : 51 – 68 .