Abstract
At Shag River estuary, North Otago, New Zealand, parts of an Archaic moa-hunter occupation site (AD 1340–1410) lie beneath 50 cm of saltmarsh sediment at a modern elevation of 0.3 m below mean high water. Fossil saltmarsh foraminiferal assemblages in the overlying cored sediment provide high-tidal palaeo-elevation estimates (Modern Analogue Technique) that indicate a gradual rise in relative sea level of 0.59±0.05 m since that time. This part of the moa-hunter site appears to have been located on an unvegetated high-tidal sand flat at the time of occupation. There is no evidence that supports previously hypothesised coseismic compaction of underlying sediment as a cause for the submergence during the time of occupation. Comparison with other saltmarsh foraminiferal records in southern New Zealand suggests that the moa-hunter occupation occurred during the interval of lowest sea level in the last millennium (early part of the Little Ice Age) and that approximately half the subsequent sea-level rise occurred after AD 1900.
Introduction
At least ten archaeological coastal occupation sites from the Archaic moa-hunter period of early human settlement in New Zealand (AD 13–14th century) occur at or below high tide level along the east coast of Otago and Southland (; McFadgen Citation2007).Footnote It is reasonable to assume that all were above mean high water level at the time of occupation and that a subsequent rise in relative sea level has drowned them. McFadgen (Citation2007) has hypothesised that this drowning was caused by coseismic (last rupture on the Akatore Fault, South Otago) compaction of underlying Holocene sediment at each site along 300 km of coast between Waitaki River mouth and Bluff (). He has named this the ‘Murihiku event’ and dated it by bracketing of calibrated radiocarbon ages at six archaeological sites at AD 1340–1410 (McFadgen Citation2007, Fig. 9.11). One of the two most convincing sites of submergence is cited as being the well-documented ‘occupation remains well below high-water mark’ (McFadgen Citation2007, p. 197) at Shag River estuary, North Otago (Haast Citation1875; Anderson et al. Citation1996). McFadgen (Citation2007) also attributes generation of an episode of sand accretion along the Otago coast (McFadgen Citation1985; Hamel Citation2001) to this inferred earthquake event. The maximum age range of this episode has been dated at AD 1340–1650, largely based on its interbedding with dateable Archaic cultural debris at a number of sites (Smith Citation1999; Hamel Citation2001; McFadgen Citation2007).
Figure 1 Location of Shag River estuary study area, North Otago, South Island, New Zealand. A, Location of SR1 core and associated transect of modern foraminiferal samples. B, Coastal Otago and eastern Southland showing location of active Akatore and Settlement faults. C, Tectonic setting of coastal Otago and east Southland on the eastern margin of the 250-km-wide Otago fault-fold belt.
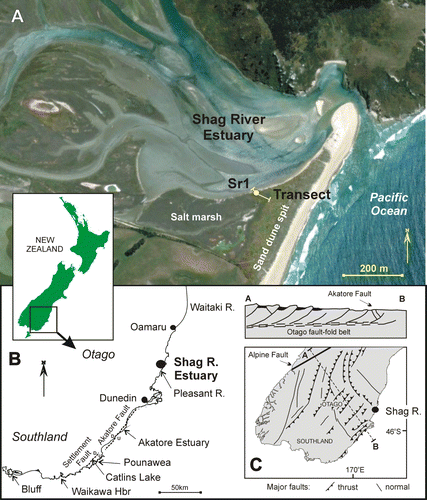
The aim of this study was to test McFadgen's hypothesis that the Archaic moa-hunter cultural layer in the estuary of Shag River was drowned as a result of a coseismic subsidence event that occurred during the short period of occupation of that site (). We proposed to reconstruct the relative sea-level history at the site using the fossil foraminiferal faunas in the overlying sediment and their known high-tidal zonation (e.g. Hayward et al. Citation1999a, Citationb). If the drowning was a result of a Murihiku Event in the 14th century, then the fossil foraminifera should record a significant displacement followed by sediment infill and upward shallowing with a final 20th century record of sea-level rise.
Table 1 Pooled mean radiocarbon ages for combinations of suitable samples spanning the full stratigraphic and geographic extent of the Shag River moa-hunter archaeological site (25 different excavated cultural layers). All mud snail (Amphibola crenata), flax (Phormium tenax), moa bone and poorly preserved fishbone samples gave highly inconsistent dates with those around them and were excluded as unreliable (from Anderson et al. Citation1996; Petchey & Higham Citation2000).
Late Holocene faults of coastal Otago
Coastal Otago and eastern Southland lie near the eastern edge of the 250-km-wide Otago fault-fold belt, where the continental collision zone along the Australian–Pacific plate boundary reaches its greatest breadth (e.g. Norris et al. Citation1990). This belt is characterised by a number of northeast-striking folds and associated reverse faults inferred to extend up from a basal detachment at c. 10–15 km depth (Beanland & Berryman Citation1989). There are no known active (last 10 ky) faults near the coast in North Otago, but there is evidence of late Holocene displacement on the Akatore and Settlement faults in coastal South Otago (). The 65-km-long Akatore Fault has a maximum of c. 120 m of Quaternary uplift (Litchfield & Norris Citation2000). Detailed studies of the onshore section have identified two rupture events within the Holocene (Litchfield & Norris Citation2000; Litchfield & Lian Citation2004). The youngest rupture is well constrained by 14 radiocarbon ages at AD 800–950 (Litchfield & Norris Citation2000), although McFadgen (Citation2007) has reinterpreted the constraining dates and extended the possible age to lie between AD 900 and 1450. In an independent study Hayward et al. (Citation2007) recorded a sudden subsidence event on the downthrown side of the Akatore Fault in Akatore estuary with the drowned saltmarsh peat having a date of AD 630–900, supporting the earlier age for the last movement on this fault.
Less than 50 km south of the Akatore Fault, the Settlement Fault () appears to have a topographically young surface trace that suggests late Quaternary movement (Bishop & Turnbull Citation1996) and it may be responsible for the existence and orientation of Catlins Lake estuary. A core at the head of Catlins Lake records 40 cm of sudden subsidence at a level 20 cm above a piece of wood dated at AD 650–890 (Hayward et al. Citation2007). This was inferred to be caused by coseismic displacement or compaction during last movement on the Settlement Fault at about the same time as the last rupture on the Akatore Fault (Hayward et al. Citation2007).
Late Holocene sea level, coastal Otago
Foraminiferal studies on a saltmarsh core at Pounawea, Catlins Lake estuary, indicate c. 0.4 m of progressive relative sea-level rise since c. AD 1500 with 0.28±0.05 m of rise since AD 1900 (Gehrels et al. Citation2008). Similar rates of gradual 20th century sea-level rise were recorded in saltmarsh cores from Catlins Lake and Akatore estuary, South Otago (Hayward et al. Citation2007), at stratigraphic levels above the evidence for the sudden subsidence inferred to be coseismic. From saltmarsh foraminiferal faunas in peat cores on compacted basement at Waikawa Harbour, east Southland (), Figueira et al. (Citation2009) inferred c. 0.5 m of sea-level rise since c. AD 1400 with 0.3 m of that during the 20th century. Hannah (Citation2004) analysed tide-gauge records around New Zealand and, after corrections for various inconsistencies, deduced the Lyttelton (Christchurch) tide-gauge recorded 0.21±0.01 m rise of mean sea level during the 20th century.
Shag River estuary study site
This estuary occurs at the mouth of Shag (Waihemo) River on the south side of Shag Pt in North Otago, New Zealand (45°28.8′S, 170°49′E). The river has a catchment of c. 140 km2 with the mouth of its valley nearly blocked by a 600-m-long sand spit (). The tidal estuary extends c. 2.5 km up the valley with its margins lined by high-tidal saltmarsh with its greatest development on the landward side of the sand spit. Uplifted Late Quaternary river and marine terraces occur near the estuary (Paterson Citation1941) and indicate a local setting of ‘minor tectonic uplift’ (Forsyth Citation2001). The only known young fault in the area is the Waihemo Fault where the youngest movement has been dated at >14.1±1.3 ka (Curran et al. Citation2011). There is no known evidence of any Holocene tectonic movement in the area. The archaeological remains (shell and bone middens, ovens, charcoal and artefacts) of an Archaic moa-hunter occupation site occur at shallow depth on the older, landward half of the sand spit and extend beneath the adjoining saltmarsh in places (Haast Citation1875; Hutton Citation1876; Teviotdale Citation1924; Anderson et al. Citation1996). The remains of ovens below high tide mark were commented upon by Haast (Citation1875) and Teviotdale (Citation1924), but disputed by Hutton (Citation1876).
McFadgen (Citation1996) produced a detailed topographic map of the spit and analysed its geomorphological history with respect to the extent of the much-studied archaeological site. He concluded that a younger, higher line of sand dunes had subsequently grown up seaward of the occupation site. He surveyed the elevation of two intertidal ovens and reported that they were approximately midway between mean sea level (MSL) and high-water level (McFadgen Citation1996, Citation2007).
The mean spring tidal range at Shag River estuary is c. 1.7 m and the extreme spring (lowest and highest astronomical tide or LAT and HAT) tidal range is c. 2 m. In our study we surveyed the elevation of an intertidally exposed charcoal-rich occupation layer on the side of the estuary at 0.45 m below mean high water springs (MHWS; c. 0.6 m below HAT). Our study site was on the eroding, estuarine edge of a firm flat area of high-tidal saltwort (Sarcocornia australis)-dominated saltmarsh () adjacent to the older sand dunes in the northwest corner of the mapped archaeological site (near site of ovens shown on Fig. 2.2 in McFadgen Citation1996). A 2.5-m-deep study core (SR1) was taken at the estuary edge of the saltmarsh at an elevation of 0.05 m above MHWS (0.1 m below HAT) and as close as possible (within 2 m) to the only exposure in the sloping intertidal beach of the charcoal-rich cultural layer.
Figure 2 Shag River estuary core site SR1 and the lower end of the modern analogue transect were located on the eroding estuarine edge of the high tidal Sarcocornia saltmarsh. The moa-hunter occupation layer, dated at AD 1340–1410 (McFadgen Citation2007), is exposed beneath the thin sand cover of the beach and encountered at 50 cm downcore in SR1.
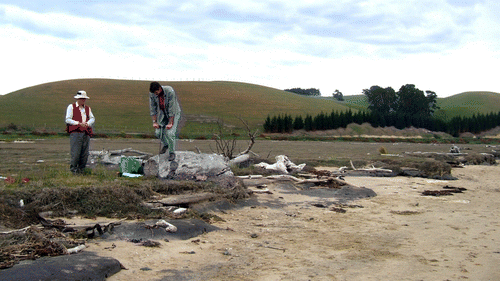
Materials and methods
Field sampling
Nine samples were collected from a 58-m-long transect through the saltmarsh at the study site (). The samples extend from close to HAT down through the vegetated high-tidal zone, to mid-tidal sand on the side of a Shag River estuary channel (). At each station a 10 ml sample was obtained at 1–2 cm depth along the transect using a short length of polyvinyl chloride (PVC) tubing (3.6 cm inside diameter) to take a plug of sediment.
A 250-cm-long core (SR1) was taken on the transect near the estuarine edge of the saltmarsh () using an Eijkelkamp Gouge Auger. Seventeen 10 ml samples were taken at selected intervals down the core for foraminiferal studies.
Foraminiferal processing
Within 24 hours of collection, all transect samples and those from the upper 10 cm of the core were soaked in a solution of Rose Bengal and ethanol to stain for protoplasm and identify ‘live’ specimens. The stain was washed out after 24 hours. Both transect and core samples were washed over a 63 µm sieve to remove the mud. The retained sand fraction was dried and micro-split to an amount containing approximately 100–200 unstained foraminiferal specimens, all of which were identified (Appendix) and counted. Even when the entire sample was completely picked, some samples had fewer than 100 specimens. For the purposes of this study a census of >80 dead specimens per sample has sufficient accuracy to identify clearly major differences in the relative abundances of the few dominant species (Patterson & Fishbein Citation1989). Two transects and six core samples were non-fossiliferous. In addition, one transect and four core samples contained far fewer than 34 foraminifera; these were excluded from the modern analogue analyses.
Nomenclature and data repositories
To distinguish between depths downcore and all other elevations we have used centimetres for SR1 sediment and metres for all others. Abbreviations used for standard tidal levels are given in .
Table 2 Abbreviations of terms used for tidal levels in New Zealand.
The core has been registered in the New Zealand Fossil Record File database as J43/f192. Washed foraminiferal samples and picked faunas are held in the collection of the Geology Department, University of Auckland. Foraminiferal census count data are available online in the supplementary data.
Estimating palaeotidal elevations
Modern analogue technique (MAT) was used to estimate palaeotidal elevations based on foraminiferal census counts (e.g. Hayward et al. Citation2004). This method assumes that the foraminifera had the same tidal-elevation-related zonation throughout the past 600 years as the modern day. The modern analogue dataset needs to be reasonably large as the palaeo-elevation estimate we derived is based on the elevation of the five most similar modern analogue faunas (using a squared chord dissimilarity coefficient). It also needs to contain samples with similar faunal compositions to those in the study core samples. The modern analogue set used here comprised 133 census counts of modern faunas from New Zealand saltmarsh transects selected to reflect the composition of faunas in core SR1 (i.e. high relative abundances of Jadammina macrescens, Trochammina inflata, Trochamminita salsa). It comprised data from Shag River estuary (7 samples, this study), Pounawea (30 samples, Southall et al. Citation2006), Akatore estuary (7 samples, Hayward et al. Citation2007), Mokomoko Inlet (17 samples, unpubl. data), Pauatahanui Inlet, Wellington (26 samples, Hayward et al. Citation1999a), Miranda and Kaipara Harbour, Auckland (9 and 37 samples, Hayward et al. Citation1999a). Because tidal ranges vary around New Zealand, the tidal elevations for samples in each modern analogue transect were converted to proportions of the local LAT to HAT range for use. The derived palaeo-elevation estimates in terms of the proportional tidal range were reconverted to the actual range for Shag River estuary (extreme astronomical tidal range: 2 m).
Transect of modern analogue foraminiferal faunas
Only seven of the Shag River estuary transect samples had sufficiently rich foraminiferal faunas (>34 specimens) to be useful in later elevational estimates. The lowest fauna (c. MSL) from unvegetated sand was dominated by calcareous Elphidium excavatum and Ammonia aoteana (34%, 14% of benthic foraminifera) that typically live in this environment. The remainder comprised a diverse suite of abraded foraminifera (e.g. Elphidium charlottense, Notorotalia spp., Zeaflorilus parri) that live in high-energy sand environments outside estuaries (Hayward et al. Citation1999b).
Five of the remaining samples with rich faunas came from the MHW–MHWS zone in Sarcocornia-dominated saltmarsh. The faunas comprised almost entirely agglutinated species co-dominated by Trochammina inflata (39–76%) and Jadammina macrescens (16–54%). The fauna with the highest elevation (between MHWS and HAT) was dominated by T. inflata (98%; ).
Figure 3 Relative abundance (and 95% confidence limits) of main saltmarsh foraminiferal taxa plotted against standardised proportional tidal elevation (LAT to HAT=1) for samples from the Shag River estuary transect [square symbol] and other samples [diamond symbol] in our modern analogue dataset. The relative abundances of the three most common taxa in samples that they dominate in core SR1 () are indicated with arrows.
![Figure 3 Relative abundance (and 95% confidence limits) of main saltmarsh foraminiferal taxa plotted against standardised proportional tidal elevation (LAT to HAT=1) for samples from the Shag River estuary transect [square symbol] and other samples [diamond symbol] in our modern analogue dataset. The relative abundances of the three most common taxa in samples that they dominate in core SR1 (Fig. 4) are indicated with arrows.](/cms/asset/fce436bb-90c5-4dd8-a206-60659ece0d59/tnzg_a_671181_o_f0003g.jpg)
Core SR1 stratigraphy and foraminiferal faunas
Core SR1 was located in Sarcocornia saltmarsh within 1 m of the edge of the eroding southern bank of the estuary (). The lower 200 cm of core (250–50 cm) comprised medium sand with sparse organic matter. At c. 50 cm, 2–3 cm of muddy organic sand contained charcoal and is correlated with the Archaic moa-hunter occupation debris recorded by archaeologists (e.g. Anderson et al. Citation1996). This has been dated at AD 1340–1410 using 32 calibrated radiocarbon dates from 25 cultural horizons from throughout the site (). The nearest acceptable date to this core site, from an excavation in the nearby saltmarsh (SM/C:Swamp, layer 2), has a charcoal date of AD 1305–1405 (Anderson et al. Citation1996). The top 50 cm of the core consisted of 30 cm of muddy, carbonaceous sand or sandy peat (50–20 cm) overlain by 20 cm of loose well-sorted fine–medium sand ().
Figure 4 Relative abundance (and 95% confidence limits) of common foraminiferal species, absolute abundance of foraminifera and mud% in Shag River estuary core, SR1. Also shown is a photo of the upper 55 cm of the core (left) and MAT estimates of the tidal elevation at which the foraminiferal faunas accumulated (right). Note the change of scale at 50 cm downcore.
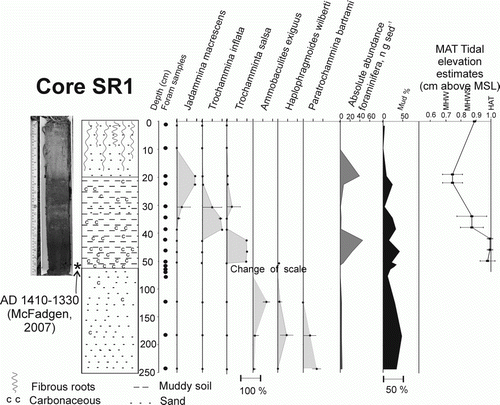
The sand between 75 and 51 cm downcore was non-fossiliferous, as was the upper loose sand layer (). The three samples from the deeper sand layer (240, 160, 120 cm) have sparse faunas comprised entirely of agglutinated species (). In the muddy organic sand between 50 and 20 cm downcore, the agglutinated faunas exhibit an upwards change from Trochamminita salsa-dominated (50–40 cm) through Trochammina inflata-dominated (40–30 cm) to Jadammina macrescens-dominated (30–20 cm).
None of the foraminiferal faunas above the cultural layer contain any specimens of taxa that live outside saltmarshes. There is therefore no evidence of any tsunami or large storm deposits in this sequence, as sediment redeposited by these events invariably contain tests of foraminiferal species that lived off the open exposed coast (e.g. Cochran et al. Citation2005).
Palaeo-elevation estimates
Core SR1 is located at a tidal elevation of 0.89 m above MSL (0.04 m above MHWS). The sparse agglutinated faunas in the three lowest samples (240–120 cm) are dominated by Ammobaculites exiguus and Paratrochammina bartrami (). These species are minor components of dominantly calcareous faunas in modern low tidal to subtidal estuaries today (Hayward et al. Citation1999b). Also present are smaller numbers of species characteristic of high-tidal saltmarsh (H. wilberti, T. inflata). The most logical inference is that these faunas are the undissolvable remnants of low-tidal to shallow-subtidal outer estuarine faunas (with some transported-in higher-tidal specimens). Such faunas would usually be dominated by calcareous Ammonia and Elphidium species (e.g. Hayward et al. Citation1999b) but these specimens have most likely been leached away by more acidic water passing through the sand (as has been noted in some brackish settings around New Zealand, especially after increased freshwater runoff following forest clearance e.g. Hayward et al. Citation2010a,Citationb). No MAT estimates of tidal elevation were attempted for these faunas because of the high level of inaccuracy of the relative abundances (low specimen numbers) and the lack of closely similar modern analogues (due to inferred taphonomic mixing and dissolution that has occurred).
The three moderately rich faunas from between 51 and 42 cm in SR1 had 96–100% T. salsa (). Faunas of this composition characterise the highest saltmarsh foraminiferal zone in New Zealand and occur above MHWS (). MAT estimated the elevation of accumulation for these three faunas just below HAT (0.98–0.99±0.02–0.05 m above MSL; ).
The two faunas from 39–34 cm had 68–92% T. inflata (). Such faunas characteristically occur around MHWS (). MAT estimates provide support for palaeo-elevations close to MHWS (0.87±0.1 m above MSL; ).
The two faunas from 23–19 cm both had 91% J. macrescens (). Faunas dominated by this species only occur in saltmarshes but have a wide tidal range between MHWN and MHWS (). The presence of small numbers of T. salsa in both samples suggests a palaeo-elevation above MHW (). MAT estimates support the above inference with palaeo-elevations between MHW and MHWS (0.74±0.07 m above MSL; ).
Sea-level record
The broad low-tidal shallow-subtidal palaeo-elevation assessment for the two lowest faunas (240 cm, 180 cm) in SR1 indicate that sea level at the time could have been similar to today (±0.3 m), or up to 1 m or so higher. The sparse fauna from 120 cm (0.3 m below MSL) with an inferred low-tidal shallow-subtidal palaeo-elevation (>0.6 m below MSL today) was deposited when sea level was probably >0.3 m above present.
There is no palaeo-elevation estimate for the non-fossiliferous sand between 75 and 52 cm downcore. It was either deposited sub-aerially or all the estuarine microfauna has been subsequently destroyed. The relatively flat-lying nature of the cultural layer at the top of this sand (c. 50 cm) inboard of the older line of sand dunes in the vicinity of the study site suggests that the sand was probably deposited on intertidal flats. The lack of preserved rootlets or any soil/peat development directly below the cultural layer implies that this part of the site was not vegetated when occupied by the moa hunters. The most plausible interpretation is that it was a high-tidal sand flat on the margin of the estuary, probably around HAT level as the fresh charcoal and other debris is likely to have been washed away by frequent tidal inundation. In other studies (e.g. Hayward et al. Citation2010c) we have noted that all foraminiferal tests are often destroyed by oxidative weathering when they occur in sand that lies above the permanent water table, as would happen here if the site was situated at or above MHWS. This could explain the lack of foraminifera in the sand below the cultural layer, even though it may have been deposited intertidally in the estuary.
Foraminifera within the charcoal-bearing cultural layer are probably the infauna that colonised it an indeterminate time after this part of the site had been abandoned by the moa-hunter and rising sea level had started advancing over it. The T. salsa fauna would have been the first to establish in the highest saltmarsh zone above MHWS. The horizon is now c. 0.59±0.05 m lower than the MAT-estimated palaeo-elevation, indicating that there has been this much sea-level rise since the time of moa-hunter occupation (AD 1340–1410; ). Because there are no dates available for any of the sequence above this level we cannot directly determine at this site when the rise occurred (the clean fine sand in the upper 20 cm of the core is unsuitable for lead and caesium isotope measurements). There is no obvious irregular erosion surface or sharp lithologic boundaries within the sequence (except below the cultural layer) that might record an erosional event, but undoubtedly there are hiatuses or intervals with extremely low sedimentation rates.
Figure 5 Curve showing relative sea-level rise as the upper 50 cm of core SR1 accumulated, based on MAT estimates (mean of 5 nearest analogues) of tidal elevation of fossil foraminiferal faunas in the core (). Error bars show standard deviation of five nearest modern analogues.
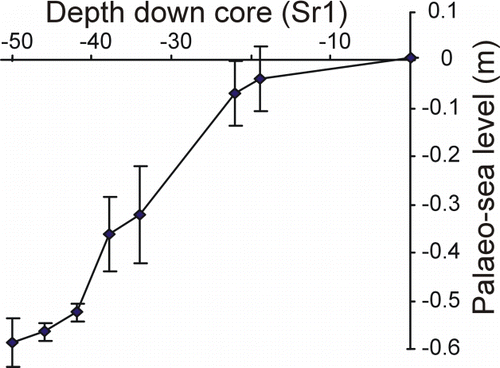
The 30 cm of sandy peat (50–20 cm in SR1) would not have been deposited rapidly and, by comparison with similar saltmarsh peats that we have been able to date from around New Zealand (e.g. Gehrels et al. Citation2008; Grenfell et al. Citation2012), it probably took c. 100 years or more to accumulate. The progressive change of foraminiferal composition up through the sandy peat () also supports the inferred progressive, rather than instantaneous, deposition. The palaeo-elevation estimates for the foraminiferal faunas between 50 and 20 cm downcore in SR1 indicate that sea level rose progressively at a rate slightly faster than that at which the peat was deposited ().
If the MAT estimate for the palaeo-elevation of fauna at 20 cm is accepted, then it indicates that the top 20 cm of root-bearing sand accumulated faster than sea-level rise which appears to have been c. 0.1 m over this period (). The small mud component in this sand implies that the site was still intertidal, albeit c. MHWS throughout the period of sand accumulation, with the mud brought in by the estuary and the sand blown in from active dunes (devegetated or new frontal belt). Today this site is covered in Sarcornia which is inferred to have been growing here throughout the sand accumulation. The absence of foraminifera in the upper 20 cm of SR1 is probably due to the high sand content and high tidal elevation (above MHWS) resulting in a relatively dry habitat subject to oxidative weathering. Note that the five transect samples containing foraminiferal faunas at Shag River were all at lower elevations ().
The 0.55 m of relative sea-level rise indicated here in SR1 since the moa-hunter period is similar to that recorded by saltmarsh foraminifera 150–200 km further south, where 0.4–0.5 m of rise has been recorded over a similar time period at Pounawea and Waikawa Harbour (Gehrels et al. Citation2008; Figueira et al. Citation2009). At both these latter locations the cored saltmarsh sediment sits directly on well-packed sand and greywacke basement, excluding the possibility of a sediment-compaction-driven explanation for any of the recorded sea-level rise. By comparison with these two sites, there is no need to invoke compaction of finer-grained sediment at depth beneath the moa-hunter layer in SR1 to account for the estimated eustatic sea-level rise, although a small component (perhaps c. 0.1 m) of compaction cannot be ruled out as possible.
The internally well-dated record from Pounawea indicates that there was 0.28±0.05 m of sea-level rise in that saltmarsh during the 20th century, although the tide gauge record from the nearest reliable port (Lyttelton, 250 km north of Shag R.) indicates a lesser amount over approximately the same time interval (0.21±0.01 m, Hannah Citation2004). We therefore infer that c. 0.25±0.05 m of the estimated sea-level rise in SR1 also occurred since AD 1900. MAT estimates indicate c. 35 cm of sediment accumulated () during this time, including the upper 20 cm of mostly wind-blown fine sand.
If we accept that the upper c. 35 cm in SR1 was deposited since AD 1900, then the sediment that accumulated between 50 and 35 cm contains a record of 0.3±0.05 m of relative sea-level rise over the preceding 500 years (back to c. AD 1400). A sea level of c. 0.55 m below present at c. AD 1400 is compatible with the record we have at Waikawa Harbour (Figueira et al. Citation2009). It is also consistent with the 0.7–0.8 m drop in sea level reported to have occurred throughout most of the Pacific around the start of the Little Ice Age (c. AD 1300), which resulted in the lowest sea level of the last millenium (summarised in Nunn Citation2007). This time (late 14th century) was only a few decades after the most prolonged (50 years) cold interval in the last 900 years in Antarctica (Bertler et al. Citation2011), at the start of Antarctica's Little Ice Age (AD 1280–1800). This may have resulted (through decreased ocean temperatures and increased ice formation) in the low sea levels recorded soon afterwards.
Late Holocene history
The Shag River estuary was formed in the early Holocene by post-glacial sea-level rise flooding this part of the valley. Longshore drift from the south built a sand spit across the mouth of the estuary with river discharge likely maintaining a permanently open entrance. Fine–medium sand (SR1, 250–50 cm) accumulated subtidally and intertidally in the outer part of the estuary adjacent to the sand spit, possibly in part during higher sea levels than today (pre-AD 1340).
This and many other coastal Otago and Southland sites were occupied (perhaps seasonally) by early human colonisers (moa-hunters) about the time of onset (14th century) of the Little Ice Age. At Shag River estuary the ‘camp site’ was located on and adjacent to the leeward side of the sand spit dunes (Anderson et al. Citation1996). Sea level by this time had dropped to c. 0.5 m lower than present and the lower parts of the Shag River occupation site appear to have encroached onto an unvegetated extreme high-tidal sand flat (SR1) on the edge of the estuary, with an inferred elevation at that time of c. EHWS–HAT.
McFadgen (Citation2007) has inferred that an increase in the proportion of estuarine bird and shellfish remains in middens partway through the short occupation record (Higham Citation1996; McGovern-Wilson et al. Citation1996) can be attributed to a coseismic compaction-induced subsidence event causing the estuary to enlarge and part of the cultural site to be submerged. This study found no lithologic or fossil evidence that supports a sudden subsidence occurring at the top of the now subtidal part of the cultural layer in SR1; one could have occurred, but left no evidence if the unvegetated sand flat being occupied was at a greater elevation than the high-tidal level inferred in this study. The change in midden contents over such a short time span is more plausibly explained by a shift in diet following the local depletion of the preferred larger food items (fur seal, moa, penguin), as suggested by Anderson et al. (Citation1996, p. 285).
We also suggest that a 14th century date for the last major movement on the Akatore (and Settlement?) Fault is debatable (e.g. Litchfield & Norris Citation2000; Hayward et al. Citation2007; McFadgen Citation2007) and that coseismic compaction of estuarine sediments along a 300 km length of coastline from Waitaki to Bluff (as suggested by McFadgen Citation2007) associated with one major upper-plate fault-related earthquake (Murihiku Event) would be highly unusual.
Radiocarbon dating suggests that occupation at Shag River mouth lasted less than a century before it was abandoned (Anderson et al. Citation1996). The foraminiferal and sedimentalogical record in SR1 indicates the abandonment of the study site by moa-hunters was followed (at some undetermined time) by a gradual sea-level rise, establishment of saltmarsh vegetation and accumulation of organic-rich sandy peat (SR1, 50–20 cm).
Site SR1 has remained in saltmarsh cover throughout the accumulation of the upper c. 50 cm of peat and sand with progressive, presumably episodic, sea-level rise, accelerating in the 20th century. Rapid accumulation of wind-blown fine sand in the saltmarsh is inferred to have occurred in the latter part of the 20th century (SR1, 20–0 cm).
We therefore infer that the lower elevations of the Shag River moa-hunter site, and probably also those at Pounawea and elsewhere along the Otago–Southland coast, were gradually submerged by rising sea level after the peak low episode in the early part of the Little Ice Age.
Conclusions
1. | A core (SR1) in saltmarsh alongside Shag River estuary, South Island, New Zealand, contains an Archaic moa-hunter occupation layer (AD 1340–1410) buried by c. 30 cm of saltmarsh sandy peat overlain by 20 cm of fine sand inferred to have blown into a high-tidal saltmarsh setting. | ||||
2. | The saltmarsh peat contains typical high-tidal saltmarsh assemblages of foraminiferal shells with palaeo-elevation (Modern Analogue Technique) estimates of c. MHWS±0.15 m. | ||||
3. | The nature of the location and sediment sequence suggest that this part of the moa-hunter site was located on an extreme high-tidal sand flat beside the estuary. The foraminiferal assemblages indicate that, at some undetermined time after abandonment, the cultural layer was progressively drowned by slowly rising sea level. | ||||
4. | Palaeo-elevation estimates indicate a rise in relative sea level of c. 0.59±0.05 m since c. AD 1400. Comparisons with similar studies in southern New Zealand saltmarshes and with the Lyttelton tide gauge records suggest that 0.2–0.3 m of this sea-level rise probably occurred during the 20th century. | ||||
5. | Moa-hunter occupation of the Shag River site (AD 1340–1410) was in the early part of the Little Ice Age with sea level apparently at its lowest level (c. 0.5 m lower than present) in the last millennium. | ||||
6. | There is no lithologic or foraminiferal evidence to support a sudden subsidence event that has been hypothesised to have inundated this part of the Shag River moa-hunter site during the 14th century (McFadgen Citation2007). |
tnzg_a_671181_sup_26104368.xls
Download MS Excel (60 KB)Acknowledgements
We thank Graeme Goh for alerting us to the inundated Shag River moa-hunter site and together with Brigida Figueira, Liesbeth van Kerckhoven and Chrissie Fildes for accompanying us in the field. Jon Kay assisted with foraminiferal faunal preparation. The manuscript has benefited from critical reading by Bruce McFadgen, although his interpretation differs from ours. We thank four referees and associate editor Kari Bassett for their suggested improvements to the manuscript.
Notes
Supplementary data available online at www.tandfonline.com/10.1080/00288306.2012.671181
Supplementary File: Foraminiferal census data for the modern analogue transect and core SR1, Shag River estuary, North Otago, New Zealand. Also included are the MAT sea-level estimates for SR1 foraminiferal samples
References
- Anderson AJ , Allingham B , Smith IWG 1996 . Shag River mouth: the archaeology of an early southern Maori village. Research Papers in Archaeology and Natural History 27 , Australian National University , Canberra , 294 p.
- Beanland , S and Berryman , KR . 1989 . Style and episodicity of late Quaternary activity on the Pisa-Grandview Fault Zone, Central Otago, New Zealand . New Zealand Journal of Geology and Geophysics , 32 : 451 – 461 .
- Bertler , NAN , Mayewski , PA and Carter , L . 2011 . Cold conditions in Antarctica during the Little Ice Age — Implications for abrupt climate change mechanisms . Earth and Planetary Science Letters , 308 : 41 – 51 .
- Bishop DG , Turnbull IM 1996 . Geology of the Dunedin area . 1:250,000 Geological Map 21, Institute of Geological and Nuclear Sciences Ltd, Lower Hutt, New Zealand .
- Cochran , U , Berryman , K , Mildenhall , DC , Hayward , BW , Southall , K and Hollis , C . 2005 . Towards a record of Holocene tsunami and storms for northern Hawke's Bay, New Zealand . New Zealand Journal of Geology and Geophysics , 48 : 507 – 515 .
- Curran C , Norris RM , Rieser U 2011 . The Waihemo Fault of North Otago: constraints on Late Quaternary activity . Abstract volume, Geosciences 2011 Conference, Nelson, New Zealand . Geoscience Society of New Zealand Miscellaneous Publication 130A : 29 .
- Figueira B , Hayward BW , Grenfell HR , Gehrels R 2009 . Salt-marsh foraminiferal proxy records of modern sea-level rise-preliminary results from the South Island, New Zealand . IGP 495 Annual Conference and Field Meeting , Program and Abstracts: 38 , Myrtle Beach, South Carolina , , USA : .
- Forsyth PJ 2001 Geology of the Waitaki area . Institute of Geological and Nuclear Sciences 1:250 000 geological map 19. GNS Science, Lower Hutt .
- Gehrels WR , Hayward BW , Newnham RM , Southall KE 2008 . A 20th century acceleration in sea-level rise in New Zealand. Geophysical Research Letters 35 , L02717 . doi: 10.1029/2007GL032632
- Grenfell HR , Hayward BW , Nomura R , Sabaa AT 2012 . Proxy record of 20th century sea-level rise in the Manukau Harbour, New Zealand . Marine and Freshwater Research . 63 : 370 – 384 .
- Haast , J . 1875 . Notes on the moa-hunter encampment at Shag Point, Otago . Transactions and Proceedings of the New Zealand Institute , 7 : 91 – 98 .
- Hamel J 2001 . The Archaeology of Otago . Department of Conservation Science Publication , Wellington , 221 pp.
- Hannah J 2004 . An updated analysis of the long term sea level change in New Zealand . Geophysical Research Letters 31, L03307 . doi: 10.1029/2003GL019166
- Hayward , BW , Grenfell , HR and Scott , DB . 1999a . Tidal range of marsh foraminifera for determining former sea-level heights in New Zealand . New Zealand Journal of Geology and Geophysics , 42 : 395 – 413 .
- Hayward BW , Grenfell HR , Reid CM , Hayward KA 1999b . Recent New Zealand shallow-water benthic foraminifera: Taxonomy, ecologic distribution, biogeography and use in paleoenvironmental assessment . Institute of Geological and Nuclear Sciences Monograph 21 , 264 p.
- Hayward , BW , Scott , GH , Grenfell , HR , Carter , R and Lipps , JH . 2004 . Estimation of tidal elevation and salinity histories of sheltered harbours and estuaries using benthic foraminifera . The Holocene , 14 : 218 – 231 .
- Hayward , BW , Grenfell , HR , Sabaa , AT , Southall , KE and Gehrels , WR . 2007 . Foraminiferal evidence of Holocene fault displacements in coastal South Otago, New Zealand . Journal of Foraminiferal Research , 37 : 344 – 359 .
- Hayward , BW , Grenfell , HR , Sabaa , AT , Kay , J , Daymond-King , R and Cochran , U . 2010a . Holocene subsidence at the transition between strike-slip and subduction on the Pacific-Australian plate boundary, Marlborough Sounds, New Zealand . Quaternary Science Reviews , 29 : 648 – 661 .
- Hayward , BW , Grenfell , HR , Sabaa , AT and Kay , J . 2010b . Using foraminiferal faunas as proxies for low tide level in the estimation of Holocene tectonic subsidence close to the Pacific-Australian plate boundary, New Zealand . Marine Micropaleontology , 76 : 23 – 36 .
- Hayward , BW , Wilson , K , Morley , MS , Cochran , U , Grenfell , HR , Sabaa , AT and Daymond-King , R . 2010c . Microfossil record of the Holocene evolution of coastal wetlands in a tectonically-active region of New Zealand . The Holocene , 20 : 405 – 421 .
- Higham TFG 1996 . Shellfish and seasonality . In : Anderson AJ , Allingham , B , Smith , IWG . Shag River mouth: the archaeology of an early southern Maori village . Research Papers in Archaeology and Natural History 27, Australian National University , Canberra , 245 – 254 .
- Hutton , FW . 1876 . Notes on the Maori cooking places at the mouth of the Shag River . Transactions and Proceedings of the New Zealand Institute , 8 : 103 – 108 .
- Litchfield , NJ and Norris , RJ . 2000 . Holocene motion on the Akatore Fault, south Otago coast, New Zealand . New Zealand Journal of Geology and Geophysics , 43 : 405 – 418 .
- Litchfield , NJ and Lian , OB . 2004 . Luminescence age estimates of Pleistocene marine terrace and alluvial fan sediments associated with tectonic activity along coastal Otago, New Zealand . New Zealand Journal of Geology and Geophysics , 47 : 29 – 37 .
- McFadgen , BG . 1985 . Late Holocene stratigraphy of coastal deposits between Auckland and Dunedin, New Zealand . Journal of the Royal Society of New Zealand , 15 : 27 – 65 .
- McFadgen BG 1996 . Topography and geomorphology of the Shag River sand spit . In : Anderson AJ , Allingham , B , Smith , IWG . Shag River mouth: the archaeology of an early southern Maori village . Research Papers in Archaeology and Natural History 27, Australian National University , Canberra , 14 – 33 .
- McFadgen BG 2007 . Hostile shores. Catastrophic events in prehistoric New Zealand and their impact on Maori coastal communities . Auckland University Press , Auckland .
- McGovern-Wilson RJ , Kirk F , Smith IWG 1996 Small bird remains . In : Anderson AJ , Allingham , B , Smith , IWG . Shag River mouth: the archaeology of an early southern Maori village . Research Papers in Archaeology and Natural History 27, Australian National University , Canberra , 223 – 236 .
- Norris , RJ , Koons , PO and Cooper , F . 1990 . The obliquely-convergent plate boundary in the South Island of New Zealand: implications for ancient collision zones . Journal of Structural Geology , 12 : 715 – 725 .
- Nunn , PD . 2007 . The A.D. 1300 event in the Pacific Basin . The Geographical Review , 97 : 1 – 23 .
- Paterson , OB . 1941 . The geology of the lower Shag Valley, N.E. Otago . Transactions of the Royal Society of New Zealand , 71 : 32 – 58 .
- Patterson , RT and Fishbein , E . 1989 . Re-examination of the statistical methods used to determine the number of point counts needed for micropaleontological quantitative research . Journal of Paleontology , 63 : 245 – 248 .
- Petchey , F and Higham , TFG . 2000 . Bone diagenesis and radiocarbon dating of fish bones at the Shag River mouth site, New Zealand . Journal of Archaeological Science , 27 : 135 – 150 .
- Smith , IWG . 1999 . Settlement permanence and function at Pleasant River mouth, East Otago, New Zealand . New Zealand Journal of Archaeology , 19 : 27 – 79 .
- Southall , KE , Gehrels , WR and Hayward , BW . 2006 . Foraminifera in a New Zealand salt marsh and their suitability as sea-level indicators . Marine Micropaleontology , 60 : 167 – 179 .
- Teviotdale , D . 1924 . Excavations near the mouth of the Shag River, Otago . Journal of the Polynesian Society , 33 : 4 – 10 .
Appendix
Taxonomic reference list of all foraminiferal species referred to in this paper and its supplementary data. Listed are citations of Figured specimens that reflect the taxonomic concept followed here. The original descriptions of these species can be found in the Ellis and Messina world catalogue of foraminiferal species at http://www.micropress.org
Order Textulariina
Ammobaculites exiguus Cushman and Brönniman 1948. Hayward et al. Citation1999b, plate 1, Figs 19, 20.
Haplophragmoides wilberti Andersen 1953. Hayward et al. Citation1999b, plate 1, Figs 25, 26.
Jadammina macrescens (Brady 1870). Hayward et al. Citation1999b, plate 1, Figs 27–29.
Miliammina fusca (Brady 1870). Hayward et al. Citation1999b, plate 1, Figs 5, 6.
Paratrochammina bartrami (Hedley, Hurdle & Burdett 1967). Hayward et al., Citation1999b, plate 2, Figs 1–3.
Trochammina inflata (Montagu 1808). Hayward et al. Citation1999b, plate 2, Figs 6–8.
Trochamminita salsa (Cushman and Brönnimann 1948). Hayward et al. Citation1999b, plate 1, Figs 30–32.
Order Rotaliina
Ammonia aoteana (Finlay 1940). Hayward and others Citation1999b, plate 16, Figs 7–9.
Elphidium charlottense (Vella 1957). Hayward et al. Citation1999b, plate 17, Figs 6–8.
Elphidium excavatum (Terquem 1875) s.l. Hayward et al. Citation1999b, plate 17, Figs 13, 14.
Notorotalia spp. Hayward et al. Citation1999b, plate 16, Figs 13–33.
Zeaflorilus parri (Cushman 1936). Hayward et al. Citation1999b, plate 15, Figs 18–20.