Abstract
Framboidal diagenetic pyrite occurs in low-grade turbidites on the northeast margin of the Otago Schist. Laser ablation inductively coupled plasma mass spectrometry (ICP-MS) analyses of these framboids show that the main metals present, in addition to Fe, are As, Pb, Zn, Te, Ag and Mo; Au is present at between 0.5 and 2 ppm. Minor Au and As anomalies also occur sporadically in the turbidite matrix. Porphyroblastic pyrite at the nearby Macraes mine contains micron-scale native gold and solid solution gold up to 30 ppm. These pyrite porphyroblasts are also enriched in As, W, Ni, Co and Bi. Lead, Zn, Te and Mo contents are distinctly lower than in the low-grade framboids. These results show that low-grade turbidites were a fertile source for Au and As for orogenic gold deposits such as Macraes. The pyrite to pyrrhotite transition, which occurred mainly under greenschist facies conditions, was probably the most effective As and Au mobilisation reaction during Mesozoic metamorphism.
Introduction
Progressive metamorphism of sedimentary rocks has been shown to mobilise elements that, when concentrated, make up orogenic gold deposits (Pitcairn et al. Citation2006, Citation2010; Large et al. Citation2007, Citation2009, Citation2011). These elements, including gold, were present in trace concentrations in the original sedimentary rocks, and were released from those rocks in metamorphic fluids that are generated by metamorphic dehydration reactions (Pitcairn et al. Citation2006, Citation2010; Large et al. Citation2011). At least some of these trace metals can be concentrated in the sediments by diagenetic processes on the sea floor, particularly into diagenetic pyrite (Large et al. Citation2007, Citation2009, Citation2011). Hence, recrystallisation and decomposition of this diagenetic pyrite during prograde metamorphism are potentially fertile sources for gold and other trace elements during orogenesis (Pitcairn et al. Citation2006; Scott et al. Citation2009; Large et al. Citation2011).
In the Otago Schist of southern New Zealand, trace metals were released from the metasedimentary pile over a wide range of metamorphic conditions, from subgreenschist facies to amphibolite facies, but primarily within the greenschist facies (Pitcairn et al. Citation2010). The Macraes mine, a world-class orogenic gold deposit, formed under greenschist facies conditions in the same metamorphic pile, with mineralisation driven by metamorphic processes (Craw et al. Citation1999; Craw Citation2002). Pyrite is an important constituent of the mineralised rocks at the Macraes mine and hosts a significant proportion of the gold (Craw et al. Citation1999). Hence, pyrite is a key mineral throughout the metamorphic–hydrothermal mineralisation process in the Otago Schist, at the source of metals in low-grade rocks, during metamorphic mobilisation of metals from those rocks, and at the site of deposition under greenschist facies conditions.
In this study, we focus on the trace element compositions of diagenetic pyrite in low-grade rocks, and on ore pyrite in the Macraes mine, in order to compare and contrast the trace element contents of pyrite in the potential source rocks and those in the end results of the mineralisation process. From these comparisons, we show that diagenetic pyrite and its low-grade host rocks were fertile sources for Au and As concentrations in orogenic gold deposits, but other metals were largely dispersed through the metamorphic pile.
General setting
Otago Schist geology
The Otago Schist consists of Mesozoic metaturbidites, with minor metabasites, that were deformed, metamorphosed and uplifted in the Jurassic and early to middle Cretaceous (Mortimer Citation1993; Deckert et al. Citation2002). Rocks in the schist belt range from weakly cleaved or foliated pumpellyite–actinolite facies metasediments on the margins to pervasively foliated greenschist facies schists in the core (). The schist belt is flanked on both sides by prehnite–pumpellyite facies turbidites () that have no metamorphic fabric. The higher grade rocks of the schist belt were at least partially exhumed by Cretaceous normal faults that resulted in the juxtaposition of rocks of different metamorphic grades in a tectonically thinned metamorphic transition ( and A, 2B; Deckert et al. Citation2002; Gray & Foster Citation2004; Henne et al. Citation2011).
Figure 1 Location map of the Otago Schist and adjacent low-grade rocks, showing the localities described in the text (section lines in are indicated with grey boxes) in relation to the metamorphic transition on the northeast side of the schist belt. Cretaceous normal faults responsible for condensing the metamorphic section are indicated: WFZ, Waihemo Fault Zone; BLFZ, Blue Lake Fault Zone (as in A, B).
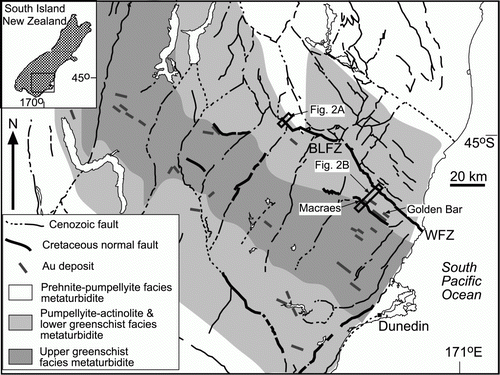
Low-grade turbidites on the flanks of the schist belt (Mackinnon Citation1983; Mackinnon & Howell Citation1984) have bedding as their only structural fabric (A, B). Primary features are progressively obscured at higher metamorphic grades, and greenschist facies rocks are almost completely recrystallised to metamorphic quartz, albite, muscovite and chlorite. Recrystallisation was accompanied by at least two stages of isoclinal folding and transposition of layering (Mortimer Citation1993). These higher grade schists are also extensively segregated, with centimetre-scale foliation–parallel metamorphic quartz–albite veins separated by micaceous laminae on the 1–10 cm scale. Distinction between sandstone, siltstone and mudstone turbidite protoliths is not possible at greenschist facies, although quartzofeldspathic and micaceous schist variants occur interlayered on the 0.1–10 m scale.
After exhumation in the Cretaceous, the schist belt and adjacent low-grade rocks have been deeply eroded to low relief topography and covered with a veneer of Cenozoic sediments. The basement rocks were variably oxidised and kaolinitised to as deep as 20 m below the sedimentary unconformity (Craw Citation1994). Renewed regional deformation and uplift in the late Cenozoic reactivated many Cretaceous faults as reverse structures and developed new Cenozoic faults (). The enhanced relief has facilitated localised erosion through the oxidised basement to expose unoxidised rock in some river gorges and on fault scarps.
Macraes mine geology
The Macraes orogenic gold mine is a world-class deposit (> 7 Moz contained Au) that is being developed in a regional scale mineralised shear zone, the Hyde-Macraes Shear Zone (B). The shear zone is a shallow-dipping (15–20 NE) foliation–parallel thrust system that formed in lower greenschist facies schists and was mineralised under lower greenschist facies conditions, as the schist was being uplifted through the brittle–ductile transition ( and B; Teagle et al. Citation1990; Craw et al. Citation1999). Mineralisation occurred in the early Cretaceous (Mortensen et al. Citation2010), and the mineralised rocks were juxtaposed against upper greenschist facies schists along a middle Cretaceous normal fault, the Footwall Fault (B).
Figure 2 Sketch cross-sections through the metamorphic transition on the northeast side of the Otago Schist belt, to show relationships between the localities described in the text. A, Section through the Blue Lake Fault Zone exposed in the gorge of the Manuherikia River, showing the low-grade turbidites (right; A) in relation to the lower greenschist facies schists (left). Samples for this study were collected near the northeastern edge of this section. B, Section across the Hyde-Macraes Shear Zone in lower greenschist facies rocks, in relation to higher and lower grade rocks.
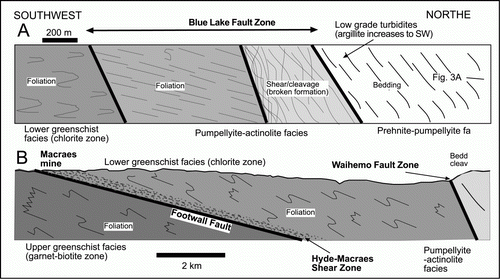
The Hyde-Macraes Shear Zone is more than 250 m thick and has at least 30 km traceable strike length (Teagle et al. Citation1990; Jones et al. Citation2007). Mineralised rocks are typically highly micaceous schists, although some mineralisation of more feldspathic schists has occurred also (Petrie et al. Citation2005). Mineralisation was mainly focused into discrete shears up to 5 m thick that have been enriched in hydrothermal graphite (C; Craw et al. Citation1999; Pitcairn et al. Citation2005; Henne & Craw Citation2012). Gold is disseminated through these sheared schists, commonly with porphyroblastic textures, although brittle overprints have brecciated and remineralised some such rocks. Mineralised quartz veins are discontinuous on the 0.5–2 m scale, and many of these have been deformed within the shear zone. Gold is almost invariably closely associated with, and typically enclosed within, pyrite and arsenopyrite in both schist and quartz veins. Scheelite occurs in quartz veins in the mineralised zone; this mineral was mined historically for its W content, but is not currently saved in the modern mining process.
The Macraes mine has been active since 1990, primarily as a series of open pits developed along the strike of the Hyde-Macraes Shear Zone (Mitchell et al. Citation2006). Most such pits have been developed within 100 m structurally above the Footwall Fault (B). Underground mining has followed the shear zone more than 500 m down dip thus far, from one of the open pits. A satellite open pit, Golden Bar pit ( and D), was developed in a sheared and veined mineralised zone more than 250 m structurally above the Footwall Fault.
Figure 3 Structural and lithological setting of sample collection sites for this study. A, Cross-section through the low-grade turbidites sampled for framboidal pyrite. B, Outcrop photograph of thin-bedded turbidites (grid reference NZTM 1354334 5024550) that contain framboidal pyrite. Bedding is near-vertical; Mdst, mudstone; Sst, sandstone; Sltst, siltstone. C, Photograph of sawn face of a hand specimen from the graphitic micaceous shear (GMS) in the Golden Bar pit, Macraes mine (grid reference NZTM 1407243 4968355). Black shear seams anastomose through the rock and disrupt micaceous laminae (dark) and metamorphic quartz–albite segregations (MQ). Circled white dots are prominent pyrite porphyroblasts (Py). D, Sketch cross-section through the base of the Golden Bar pit (partly after Jones et al. 2007), showing the sampled graphitic micaceous shear in relation to other mineralized features.
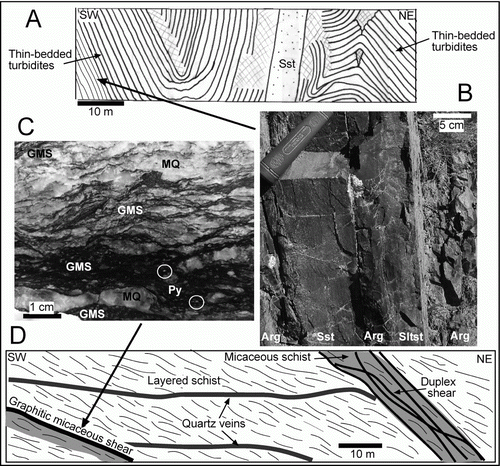
Methods
The first part of this study examines diagenetic pyrite preserved in low-grade (prehnite–pumpellyite facies) turbidites that are exposed in a deep gorge that has been eroded on the flanks of an active tectonic zone (A). The samples were taken from the wall of the gorge, within reach of high flood waters. This setting ensures that the rocks are fresh and unoxidised, and all sulfides are preserved. No limonite staining is evident in these samples. The second part of the study examines mineralised pyrite in sheared micaceous schist from the Macraes mine ( and B). This material was collected from near the floor of a c. 50 m deep open pit at Golden Bar, which is structurally high in the Hyde-Macraes Shear Zone (). The pit was recently inactive at the time of sample collection. The sulfide minerals (pyrite and arsenopyrite) are completely unoxidised, and no limonite is present in the rocks.
Polished mounts were etched with concentrated nitric acid to reveal internal zonation within pyrite grains. Etch times varied from 20 to 60 s, depending on the As content and degree of crystallisation of the pyrite.
The analytical instrumentation used in this study includes a New Wave 213 nm solid-state laser microprobe coupled to an Agilent 4500 quadrupole inductively coupled plasma mass spectrometer (ICP-MS) housed at the CODES LA-ICP-MS analytical facility, University of Tasmania (Large et al. Citation2009). Quantitative LA-ICP-MS analyses were performed by ablating spots ranging in size from 25 to 40 µm. Laser repetition rate was typically 5 Hz and laser beam energy at the sample was maintained between 4 and 5 J cm−2. The analysis time for each sample was 100 s, comprising a 30 s measurement of background (laser off) and a 70 s analysis with the laser on. Data reduction was undertaken using Fe as the internal standard. An in-house Li–borate fused glass of a pyrite/sphalerite mixture was used as the primary calibration standard. The standard was analysed twice every one and a half hours to account for the instrument drift, with a 100-µm beam and at 10 Hz. Imaging of pyrite was performed by ablating sets of parallel lines in a grid across the samples. Depending on the sample size, lines were ablated with a beam size of 15 or 25 µm. The spacing between the lines was kept constant at the same size as the laser beam. Lines were ablated at a repetition rate of 10 Hz, rastering at 15 or 25 µm s−1, depending on the spot size. Thus, every position on the sample was ablated 10 times and its composition contributed to 10 consecutive pixels on the image, resulting in an unprocessed effective resolution matching the beam size. A set of 20 elements was analysed with acquisition time for most masses set to 0.002 s, however, to improve their detection limits, Se was measured for 0.004 s and Ag, Te and Au were measured for 0.04 s. The total sweep time was c. 0.2 s. Further details of the analytical methods are provided in Large et al. (Citation2009).
Pyrite in low-grade turbidites
Host rocks
The low-grade rocks for this study were taken from a sequence of prehnite–pumpellyite facies turbidites which have no metamorphic fabric. The low-grade rocks are in the hanging wall of a major Cretaceous fault zone, the Blue Lake Fault Zone (A; Forsyth Citation2001; Henne et al. Citation2011). This fault zone has caused extreme structural thinning of the Otago Schist margin, and has juxtaposed blocks of differing metamorphic grade up to lower greenschist facies (A). Fault zone deformation was focused into well-defined cataclastic gouge zones at and near the margins of these blocks, and there has been little deformation internally within the blocks. Samples for this study were taken from steeply dipping thin-bedded turbidites c. 500 m upstream of the fault strand that defines the boundary of the prehnite–pumpellyite facies rocks (A, A). This sequence is on the limb of a tight fold of bedding, with a sheared hinge zone (A).
The thin-bedded turbidites have sandstones, siltstones and mudstones interlayered on the 10 cm scale (B) with variably incomplete Bouma sequences. Primary features such as clastic textures, graded bedding and sedimentary structures are well preserved. The rocks have abundant angular to subrounded feldspar, quartz and quartzofeldspathic lithic clasts, with a matrix of fine-grained muscovite and chlorite that has been weakly altered by diagenesis or low-grade metamorphism. Detrital organic fragments (10–100 µm) are scattered through the sediments, with some fragments displaying relict cell structures. Total organic carbon contents of all rock types are low (< 0.1 wt%).
Diagenetic pyrite
Scattered grains of diagenetic pyrite have grown in the matrix of most sandstones, siltstones and mudstones, although total pyrite content estimated from S analyses is < 0.3 wt%. The pyrite has framboidal textures, with framboids ranging from 30 to 300 µm across (A–D). Individual pyrite microcrysts within the framboids are typically c. 1–5 µm across, although many of these have merged to make irregular pyrite masses within framboids (A–D). Most framboids have grown within silicate substrate, but some framboids have nucleated in relict cells of organic detritus.
Figure 4 Scanning electron images of pyrite framboids (white) in low-grade turbidite samples, with LA-ICP-MS spot analyses indicated (all in ppm). A, B, Mudstones. C, Siltstone. D, Sandstone.
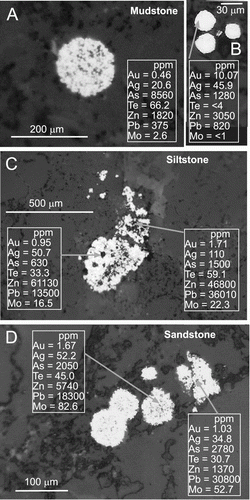
Analysis of framboidal pyrite by LA-ICP-MS yields bulk results integrated over many separate microcrysts in close proximity. In this study, the laser beam was focused on sites where the microcrysts have merged, to minimise interference from the intervening matrix, in order to determine the trace metal contents (A–4D). These results show that As, Pb and Zn are the dominant trace metals in the diagenetic pyrite, with As up to 0.9 wt%, Pb up to 3.6 wt% and Zn up to 10 wt% (A–D, A). Occurrences of dispersed fine-grained (submicron) sphalerite and galena in the pyrite framboids (as described by Pitcairn et al. Citation2006, Citation2010) cannot be discounted as sources of the locally high Pb and Zn contents.
The gold content of the framboids is typically between 0.5 and 2 ppm (A–D, A), although one spot, on a small mass of merged microcrysts in a mudstone, yielded 10 ppm Au (B). Silver contents are high (c. 100 ppm), and W contents are low, near 1 ppm (B, C). Tellurium contents typically range from < 10 to c. 100 ppm, and spots with higher Te generally have higher Au contents as well (A–D, D). Molybdenum contents range from < 1 to 100 ppm (A–D, E). The typical trace metal contents of framboidal pyrite appear to be similar in sandstone, siltstone and mudstone (A–D). However, some framboids in sandstones and siltstones, texturally indistinguishable from those described above, have no detectable Au and distinctly lower levels of other trace metals, with As, Pb and Zn all < 200 ppm.
Figure 5 Geochemical plots of LA-ICP-MS spot analyses of framboidal pyrite in turbidites (□) and porphyroblastic pyrite from Macraes mine (•). All scales are logarithmic. A, As vs Au. B, Ag vs Au. C, W vs Au. D, Te vs Au. E, Ag vs Mo. F, Zn vs Pb.
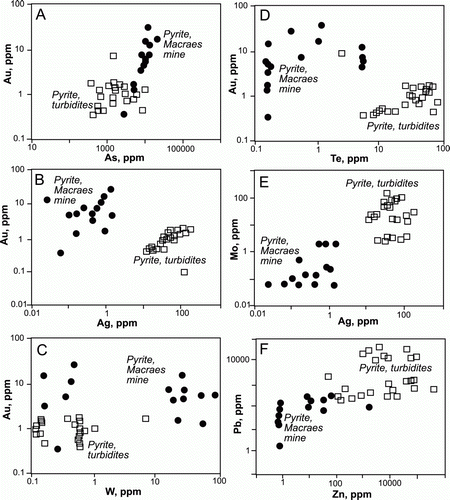
Laser scanning across parts of a siltstone without visible pyrite shows that there are irregular distributions of As and Au at detectable and locally relatively high levels, at the <100 µm scale (). The anomalous Au is more than 10 times higher than background levels of c. 6 ppb (). As and Au anomalies generally do not coincide in the traverse. Likewise, most of these locally anomalous Au and As spots do not coincide with pyrite, as indicated by the presence of high Fe and S (). The laser beam did pass a visible pyrite framboid in the traverse in , and this resulted in high Fe and S coinciding with high As and high Au. A traverse across a bedding boundary from sandstone into mudstone, with no visible pyrite, has highly variable Au and As background in the sandstone because of the presence of large detrital silicate clasts, and greater homogeneity of background in the mudstone (). This traverse also showed similar anomalous Au and As spots () to those in . There was no coincidence of Au and As, and there are no Fe anomalies indicative of pyrite coincident with high Au in this traverse either ().
Pyrite in a Macraes mine shear
Host rocks
Rock for this study was collected from the Golden Bar pit ( and D) because of the relative ease of access and because the sheared micaceous mineralised rocks have relatively less brittle overprinting deformation than those that are structurally lower in the Hyde-Macraes Shear Zone. The Golden Bar pit has two main foliation–parallel shears (D). The structurally higher shear is a complex duplex structure that is focused in micaceous schists, but includes lenses of more feldspathic schist and silicified schist, with some mineralised quartz veining, that has been resheared after initial mineralisation (Jones et al. Citation2007). The lower, graphitic micaceous shear (D), is also focused in micaceous schist, but had less silicification associated with mineralisation, and negligible mineralised quartz vein development. Some undeformed mineralised quartz veins cut the unmineralised schists between these two shears (D).
Figure 7 Scanning electron image (upper) of boundary between a sandstone (left) and mudstone (right) across which a LA-ICP-MS scan obtained elemental variations (lower).
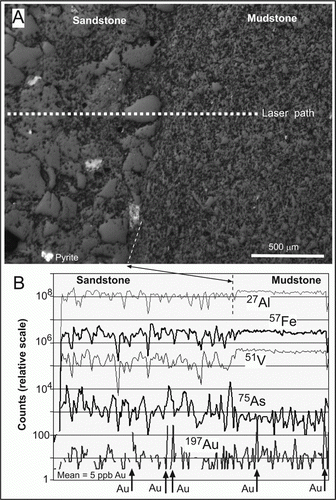
The sampled rocks are fissile graphitic micaceous schists from the black shear, with abundant hydrothermal graphite (c. 1–2 wt%) and disseminated pyrite and arsenopyrite (total S = 0.5–2 wt%). Graphite, sulfides and recrystallised muscovite and rutile define a shear fabric that developed parallel to, and locally enhanced, the metamorphic foliation (C). Anastomosing shears pervaded the rock with c. 1 cm spacing, forming dark laminae rich in graphite, micas and sulfide minerals (C). Graphitic micaceous shear seams have also cut through and locally disrupted unmineralised metamorphic quartz–albite segregations and veins (C). The graphite and sulfides have been cataclastically deformed during brittle reactivation to form a soft black shiny coating < 1 mm thick on some sheared foliation surfaces, with a spacing of 2–10 cm. Between these cataclastic seams, pyrite and arsenopyrite commonly show porphyroblastic textures where they have grown across the metamorphic foliation but within the later subparallel recrystallised shear fabric. Many of the porphyroblasts have quartz pressure shadows around their margins, cutting across both foliation and shear fabric. Porphyroblastic pyrite grains without an overprint of brittle fractures are the focus of the present study.
Pyrite internal textures and compositions
Pyrite porphyroblasts in the graphitic micaceous shear are typically 0.5–2 mm across and have subhedral to euhedral crystal shapes (A, B, D). Most large porphyroblasts have an internal structure with complex zonation showing several different generations of pyrite preserved with growth zonation in some generations (A, B, D). Evidence for at least three pyrite generations is commonly present. The central pyrite generations in A and B are clear and inclusion-free, as is part of the the outer pyrite in A. Intervening pyrite growth has numerous micron-scale inclusions, some of which are ordered into growth zones (A, B). By contrast, the pyrite porphyroblasts in D and have an inclusion-rich core, clear pyrite in an inner zone and inclusion-rich growth zones in the outer generation. Some porphyroblasts are composite, with several intergrown smaller grains making a more irregularly shaped whole.
Figure 8 Textures and compositions of some pyrite porphyroblasts from a graphitic micaceous shear at Golden Bar pit, Macraes mine. LA-ICP-MS spot analyses for Au, As and W are in ppm; P1, P2, P3 refer to specific growth generations mentioned in the text. A, B, Scanning electron images of etched porphyroblasts, showing several growth zones. C, LA-ICP-MS traverse across part of the porphyroblast in B (thick white line). D, Scanning electron image of a pyrite porphyroblast. E, LA-ICP-MS Au map at the same scale as image in D. Colour scale is relative and logarithmic, with lowest concentrations in shades of black and blue, and highest concentrations in shades of red and orange. Red zone (lower left) is a native gold grain.
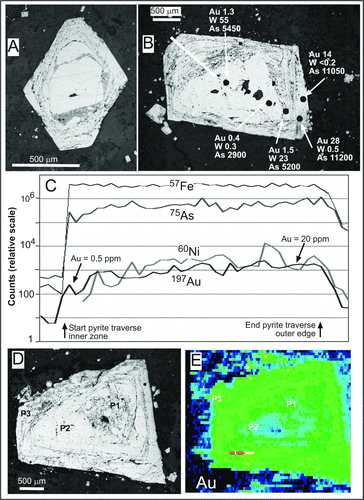
Arsenic is the principal trace element in the Macraes porphyroblasts, with As contents typically c. 1 wt% (A, B). Lead and zinc both have low concentrations, generally near 100 ppm (F). Likewise, Te and Mo contents are low (< 10 ppm; D, E). Some pyrite grains have elevated Ni, up to c. 20 ppm. Tungsten is distinctly elevated, commonly > 20 ppm, in most porphyroblasts (B, ). A spot analysis with 55 ppm W was obtained on clear inclusion-free pyrite (B), so inclusions of scheelite can be discounted as a source for that elevated W. However, elevated W at and beyond the edge of the porphyroblast in may reflect dispersed scheelite in the rock.
These trace elements are not uniformly distributed through the porphyroblasts, and distinct zonation of trace element contents occur (). Most of this trace element zonation can be broadly linked to the textural zonation defining different growth generations of the pyrite grains. Nickel, Co, Se, W, Bi and Pb are relatively enriched in the outermost pyrite generation (P3; ) and these elements are also locally enriched in the core pyrite generation (P1; ). This element suite is relatively depleted in the inner zone (P2; ). A similar, but less clear-cut zonation occurs with Bi distribution (). Arsenic has limited systematic zonation (), but does show considerable compositional variation within porphyroblasts, with As contents of 0.5 and 2 wt% in close proximity, and greatest As contents near the outer rim (B, C).
Gold contents of pyrite porphyroblasts are also highly variable. Some gold occurs as separate grains (1–10 µm) enclosed in the pyrite (E, B). However, there is also significant gold (up to 30 ppm) distributed through the pyrite grains in solid solution (B, C). These elevated gold concentrations generally occur in the outermost pyrite generation (B, C), and distinct zonation in gold contents occur (P3; D, E). The core pyrite generation (P1) in is sporadically enriched in gold, whereas the outer generation (P3) is only sporadically enriched.
Figure 9 Texture and composition of a pyrite porphyroblast with growth zones (P1, P2, P3), with LA-ICP-MS spot analyses (Au, W = ppm; As = wt%). A, Scanning electron image B, Gold map at the same scale as in A, overlain with interpreted growth generation boundaries (dotted black lines, P1; solid black lines, P2). A suite of smaller scale LA-ICP-MS maps of the same porphyroblast (elements indicated) is depicted on the right. Colour scales for all LA-ICP-MS maps are relative and logarithmic, with lowest concentrations in shades of black and blue, and highest concentrations in shades of red and orange.
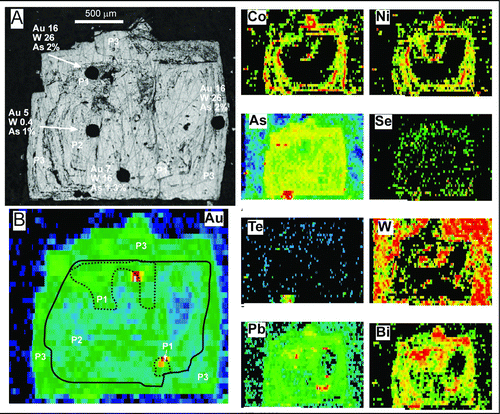
Discussion
Low-grade rocks as sources for Au and As
Orogenic gold deposits in Otago Schist are characterised by elevated Au and As, and these elements are generally thought to have been derived from their host rocks during metamorphism (Henley et al. Citation1976; Paterson Citation1986; Pitcairn et al. Citation2006, Citation2010; Bierlein & Craw Citation2009; Large et al. Citation2011). Some involvement of magmatic processes has also been suggested (de Ronde et al. Citation2000), although no specific coeval igneous bodies have yet been identified near the deposits. Pitcairn et al. (Citation2006, Citation2010) showed that there was general depletion of Au and As with increasing metamorphic grade, that oxide and sulfide minerals were important repositories for metals at low grade, and that recrystallisation of these oxide and sulfide minerals during prograde metamorphism was important for mobilisation of the metals. The results of the present study support this contention and add some more specific information on potential metal sources.
The data provided above show that some diagenetic pyrite in low-grade turbidites has low but significant Au contents (c. 1 ppm), up to 1000 times typical rock background levels (A, ). Likewise, the diagenetic pyrite is commonly substantially enriched in As (1000–10,000 ppm; A), also up to 1000 times typical rock background levels. Hence, this diagenetic pyrite is a potent source for Au and As in the metamorphic pile, similar to the turbidite hosts in the Victorian goldfield of Australia (Thomas et al. Citation2011). This Au and As was concentrated into the pyrite during growth of the diagenetic framboids after sedimentation, probably near to the sediment/water interface (Large et al. Citation2011). The diagenetic Au and As concentration process, and framboidal pyrite deposition in general, apparently affected all rocks (sandstone, siltstones and mudstones) in the thin-bedded turbidite sequence examined in this study, whereas the process is commonly confined to organic shales in other settings (Large et al. Citation2011). Conversely, the degree of framboidal pyrite enrichment in these turbidites is low compared to organic shales that can have up to 5% total S as pyrite and 10% organic carbon (Large et al. Citation2011).
In addition to the framboidal pyrite, there is also other material enriched in gold and arsenic in the low-grade turbidites. This material, which is too fine grained to be identified in this study, shows up as isolated Au and As peaks in the laser beam traverses across pyrite-free sediments, and occurs in the diagenetically affected matrix of the sediments ( and ). The Au and As peaks do not coincide, so these elements are clearly decoupled in this material, but are also both separate from pyrite hosts. Similar material, presumed to be diagenetic nanoparticulate precipitates, some attached to organic matter, has been described in organic shales elsewhere by Large et al. (Citation2011). Like the framboidal pyrite, the more dispersed but locally concentrated Au and As are potential sources for mobilisable Au and As in these low-grade turbidites.
Comparison of diagenetic pyrite and mineralisation pyrite
The host schists at the Macraes mine are greenschist facies equivalents of the low-grade turbidites on the flanks of the schist belt ( and A, B). Hence, there is a possibility that pyrite in the turbidites may have persisted in some form to higher metamorphic grades, as has been observed in other orogenic gold-bearing metasedimentary belts (Large et al. Citation2007, Citation2011). However, the extensive silicate recrystallisation that accompanied development of the greenschist facies foliations, followed by further recrystallisation during development of the Hyde-Macraes Shear Zone, has ensured that any relict pyrite from a low-grade protolith is likely to be substantially modified texturally. There is no textural evidence from the observations in this study for the persistence of diagenetic framboids in greenschist facies mineralised rocks (A, B, D, , A). Even the earliest generations of pyrite in the Macraes mineralised rocks appear to be either of metamorphic or of late metamorphic mineralisation origin, as they occur within the metamorphic foliation and/or mineralisation-related graphitic micaceous shears (C).
Textural evidence for the lack of relict diagenetic pyrite in the Macraes rocks is supported by the analytical data which show clear chemical distinctions between diagenetic pyrite and mineralisation pyrite (A–F). Diagenetic pyrite is enriched in Pb, Zn, Te, Mo and Ag compared with mineralisation pyrite, whereas mineralisation pyrite is locally enriched in Ni and W compared with diagenetic pyrite. Both types of pyrite are enriched in Au and As, although the most prominent Au and As enrichment in the mineralised rocks occurs on the outer edges of grains (B, D, ), and is of undoubted hydrothermal origin. Hydrothermal pyrite has Au/Ag > 1, compared with diagenetic pyrite where Au/Ag << 1 (B). Some turbidite pyrite, with euhedral rather than framboidal texture, in the Otago Schist belt is locally enriched in Co via inclusions of cobaltite (CoAsS; Pitcairn et al. Citation2010), and there has been some Co enrichment of Macraes pyrite during porphyroblast bgrowth (). Hence, the two types of pyrite have Au, As and Co enrichment in common, but numerous other metal contents are distinctly different and these differences combined with the associated textural differences show that the mineralisation pyrite at Macraes has been deposited entirely separately from any diagenetic precursor pyrite that may have been in the host rock protoliths.
Metamorphic mobilisation of metals from pyrite and rock
Diagenetic pyrite recrystallised first to metamorphic pyrite and then to pyrrhotite with prograde metamorphism in the Otago Schist (Jamieson & Craw Citation1986; Pitcairn et al. Citation2006, Citation2010). These iron sulfide transformations were controlled by host rock compositions, particularly redox conditions, at a range of metamorphic grades (Jamieson & Craw Citation1986; Pitcairn et al. Citation2010). The transformation of pyrite to pyrrhotite occurred mainly under greenschist facies conditions, and pyrite is rare as a metamorphic mineral in the amphibolite facies.
Metamorphic pyrrhotite does not contain abundant trace metals like the diagenetic pyrite does, so the pyrite to pyrrhotite transition was an important reaction for mobilising trace metals during metamorphism (Pitcairn et al. Citation2006, Citation2010; Large et al. Citation2009, Citation2011). Hence, mobilisation of Au and As is likely to have occurred in this transition, contributing these elements to a metamorphic fluid that was being generated by mineral dehydration reactions at the same time (Pitcairn et al. Citation2006; Upton & Craw Citation2009). Metamorphic dissolution of dispersed gold and arsenic in the turbidites, of probable diagenetic origin ( and ), was probably an additional source of these elements for the mineralising fluids. The Au and As were then available for structurally controlled depositional concentration, such as in the Macraes deposit (). Assuming the source rock contained 0.1 wt% pyrite, and that pyrite contained 1 ppm Au, 1 km3 of this rock could release 2600 kg (84000 ounces) of gold. The known gold resource in the Hyde-Macraes Shear Zone could have been derived from < 100 km3 of rock, equivalent to a zone c. 1 km wide and c. 3 km deep beneath the 30 km strike length of the shear zone. Gold extraction during metamorphism was not 100% efficient (Pitcairn et al. Citation2006), but tectonic processes constantly added rock to the metamorphic pile, and a dynamic collisional system could have produced enough gold for the Macraes deposit in less than 3 myr (Craw Citation2011).
Lesser proportions of Ag, Te and possibly Co from the diagenetic pyrite were also mobilised into the Macraes deposit during mineralisation, but some of these metals were dispersed into the metamorphic pile (). Molybdenum is only weakly enriched in the Macraes deposit (Craw Citation2002), and less enriched than in the diagenetic pyrite (E), so some synmetamorphic dispersal of Mo apparently occurred as well. Hydrothermal molybdenite was deposited during greenschist facies metasomatic reactions on the margins of metabasites in the schist belt (; Cooper & Reay Citation1983; AF Cooper, University of Otago, pers. comm., 2011), and this may reflect elevated Mo in some greenschist facies metamorphic fluids combined with particular fluid–rock interaction reactions. Abundant Pb and Zn in the diagenetic pyrite has not been mobilised during metamorphism and has stayed in the rock, possibly in sphalerite, galena and/or silicate minerals (Pitcairn et al. Citation2006, Citation2010).
Figure 10 Summary conceptual diagram, depicting prograde metamorphic mobilisation of metals from diagenetic pyrite as it was transformed to pyrrhotite. The Au and As are concentrated in a Macraes-like structure, and other elements are partially dispersed. Tungsten, Cr, and Ni have different pathways from the host rocks (see text).
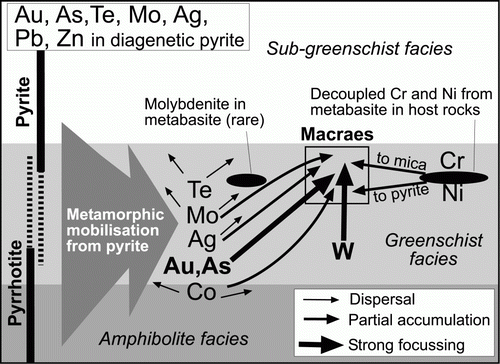
Some Macraes mine host rocks contain elevated Cr and Ni because of anomalous mafic and ultramafic detrital components in the metasediments (Craw & Angus Citation1993). Hydrothermal processes have decoupled Cr from Ni during mineralisation, so that some mineralised rocks are distinctly Cr-enriched (Craw Citation2002), with Cr presumably situated in micas (cf Craw & Angus Citation1993). Conversely, mineralisation-related pyrite is locally enriched in Ni (C, ; Petrie et al. Citation2005), and this was probably the sink for Ni mobilised from immediate host rocks at the same time as was Cr. Hence, whereas Au, As, Ag, and to a lesser extent Mo were mobilised into the Macraes deposit from diagenetic pyrite over an unknown distance (probably kilometres; Pitcairn et al. Citation2006) during metamorphism, the elevated Ni in mineralisation pyrite was probably derived from the immediate host rocks (metres to tens of metres).
Tungsten is enriched in the Macraes deposit, as both scheelite and in solid solution in some parts of the porphyroblastic pyrite ( and C). Conversely, W is not enriched in diagenetic pyrite in the low-grade turbidite protoliths, so derivation of W for synmineralisation enrichment from the pyrite to pyrrhotite transition is implausible. Instead, the W in the mineralising fluids was probably mobilised during the bulk rock metamorphic transformations under pumpellyite-actinolite and/or greenschist facies conditions (; Craw & Norris Citation1991) from, for example, disseminated galena (Pitcairn et al. Citation2006).
The observations in this study provide additional support for the hypothesis of metamorphic mobilisation of metals proposed by Pitcairn et al. (Citation2006, Citation2010). However, the processes of metal transport and deposition in concentrated amounts are still poorly understood in the context of this metamorphic mobilisation hypothesis. These processes apparently lead to a wide range of metal pathways (), with Au and As being the only elements maintaining a consistent mutual relationship. Geochemical processes that mobilise some metals but retain Pb and Zn in the source rocks are as yet poorly defined, although Johnstone et al. (Citation1990) suggest that chloride-rich fluid can mobilise base metals from these rocks. The strong enrichment of W in the Macraes deposit is not predictable from the observed geochemistry of the low-grade rocks described in this study, and additional processes and/or sources involving W enrichment would be a fruitful topic for future research.
Conclusions
Framboidal diagenetic pyrite is preserved in prehnite–pumpellyite facies turbidites on the northeast margin of the Otago Schist. Framboids occur in sandstones, siltstones and mudstones as 30–300 µm spheres consisting of micron-scale pyrite microcrysts. Laser ablation ICP-MS analyses of these framboids show that the main metals present are As (up to 0.9 wt%), Pb (up to 3.6 wt%) and Zn (up to 10 wt%). Minor Te, Ag and Mo occur, up to c. 100 ppm of each. The Au content of the framboids is typically between 0.5 and 2 ppm. Minor Au and As anomalies also occur sporadically in the matrix of the turbidites, independent of pyrite. Pyrite in graphitic micaceous shears at the nearby Macraes mine, which was mineralised under lower greenschist facies conditions, has several growth generations to form millimetre-scale euhedral porphyroblasts. These porphyroblasts contain micron-scale native gold, and solid solution gold up to 30 ppm that is most strongly enriched in outer growth zones. These pyrite porphyroblasts are also enriched in As (c. 1 wt%), W and Ni are locally enriched to > 20 ppm, and minor enrichment of Co and Bi has occurred in some growth zones. Lead, Zn, Te and Mo contents are distinctly lower than in the low-grade framboids. These results show that low-grade turbidites were potentially a fertile source for Au and As during metamorphism, from both framboidal pyrite and the matrix. The pyrite to pyrrhotite transition, which occurred mainly under greenschist facies conditions, was probably the most effective As and Au mobilisation reaction during Mesozoic metamorphism. The Au and As that is known to have been mobilised during metamorphism (Pitcairn et al. Citation2006, Citation2010) may have been concentrated in late metamorphic structures, such as at Macraes mine. Elevated Pb and Zn in diagenetic framboids were apparently retained in the source rocks. Tungsten, Cr and Ni, that are locally enriched in the Macraes ore, may have been derived from host rocks via separate mechanisms from the Au and As.
Acknowledgements
This research was funded by Glass Earth Gold Ltd, University of Otago, New Zealand Ministry for Science and Innovation, and the Australian Research Council. Ben Baxter of Oceana Gold Ltd facilitated collection of mine samples from Golden Bar pit. Discussions with Anthony Coote, Philip Jones, Doug MacKenzie, Leonid Danyushevsky and John Youngson helped to clarify ideas expressed herein. Able technical assistance was provided by Damian Walls, Brent Pooley, Sarah Gilbert and Shaun Inglis. Helpful comments from Nick Mortimer, Iain Pitcairn, and two anonymous referees helped to improve the presentation of the manuscript.
References
- Bierlein , FP and Craw , D . 2009 . Petrogenetic character and provenance of metabasalts in the Aspiring and Torlesse Terranes, South Island, New Zealand: implications for the gold endowment of the Otago Schist? . Chemical Geology , 260 : 330 – 344 .
- Cooper , AF and Reay , A . 1983 . Lithology, field relationships, and structure of the Pounamu Ultramafics from the Whitcomb and Hokitika Rivers, Westland, New Zealand . New Zealand Journal of Geology and Geophysics , 26 : 359 – 379 .
- Craw , D . 1994 . Contrasting alteration mineralogy at an unconformity beneath auriferous terrestrial sediments, central Otago, New Zealand . Sedimentary Geology , 92 : 17 – 30 .
- Craw , D . 2002 . Geochemistry of late metamorphic hydrothermal alteration and graphitisation of host rock, Macraes gold mine, Otago Schist, New Zealand . Chemical Geology , 191 : 257 – 275 .
- Craw D 2011 . Lithology and metal sources and sinks, Otago Schist . Australasian Institute of Mining and Metallurgy New Zealand Branch Annual Conference Proceedings , Queenstown , New Zealand . 71 – 79 .
- Craw , D and Norris , RJ . 1991 . Metamorphogenic Au–W veins and regional tectonics: mineralisation throughout the uplift history of the Haast Schist, New Zealand . New Zealand Journal of Geology and Geophysics , 34 : 373 – 383 .
- Craw , D and Angus , PV . 1993 . Mafic/ultramafic clasts in deformed biotite zone metaconglomerate, Macraes mine, Haast Schist, New Zealand . New Zealand Journal of Geology and Geophysics , 36 : 395 – 398 .
- Craw , D , Windle , SJ and Angus , PV . 1999 . Gold mineralization without quartz veins in a ductile–brittle shear zone, Macraes Mine, Otago Schist, New Zealand . Mineralium Deposita , 34 : 382 – 394 .
- Deckert , H , Ring , U and Mortimer , N . 2002 . Tectonic significance of Cretaceous bivergent extensional shear zones in the Torlesse accretionary wedge, central Otago Schist, New Zealand . New Zealand Journal of Geology and Geophysics , 45 : 537 – 547 .
- de Ronde , CEJ , Faure , K , Bray , CJ and Whitford , DJ . 2000 . Round Hill Shear Zone hosted gold deposit, Macraes Flat, Otago, New Zealand: evidence of a magmatic ore fluid . Economic Geology , 95 : 1025 – 1048 .
- Forsyth PJ 2001 . Geology of the Waitaki area . Institute of Geological and Nuclear Sciences 1:250 000 Geological Map 19. 1 sheet + 64 pp .
- Gray , DR and Foster , DA . 2004 . 40Ar/39Ar thermochronologic constraints on deformation, metamorphism and cooling/exhumation of a Mesozoic accretionary wedge, Otago Schist, New Zealand . Tectonophysics , 385 : 181 – 210 .
- Henley , RW , Norris , RJ and Paterson , CJ . 1976 . Multistage ore genesis in the New Zealand geosyncline: a history of post-metamorphic lode emplacement . Mineralium Deposita , 11 : 180 – 196 .
- Henne A , Craw D 2012 . Synmetamorphic carbon mobility and graphite enrichment in metaturbidites as a precursor to orogenic gold mineralization, Otago Schist, New Zealand . Mineralium Deposita . doi: 10.1007/s00126-012-0399-2
- Henne , A , Craw , D and MacKenzie , D . 2011 . Structure of the Blue Lake Fault Zone, Otago Schist, New Zealand . New Zealand Journal of Geology and Geophysics , 54 : 311 – 328 .
- Jamieson , RA and Craw , D . 1986 . Sphalerite geobarometry in metamorphic terranes: an appraisal with implications for metamorphic pressure in Otago Schist . Journal of Metamorphic Geology , 4 : 87 – 99 .
- Johnstone , RD , Craw , D and Rattenbury , MS . 1990 . Southern Alps Cu–Au hydrothermal system, Westland, New Zealand . Mineralium Deposita , 25 : 118 – 125 .
- Jones , P , Craw , D and Norris , RJ . 2007 . Golden Bar gold deposit as an extension of the Hyde-Macraes Shear Zone, east Otago, New Zealand . New Zealand Journal of Geology and Geophysics , 50 : 271 – 281 .
- Large , RR , Maslennikov , VV , Robert , F , Danyushevshy , LV and Chang , Z . 2007 . Multistage sedimentary and metamorphic origin of pyrite and gold in the giant Sukhoi Log deposit, Lena Gold Province, Russia . Economic Geology , 105 : 1233 – 1267 .
- Large , RR , Danyushevsky , L , Hollit , C , Maslennikov , V , Meffre , S , Gilbert , S , Bull , S , Scott , R , Emsbo , P , Thomas , H , Singh , R and Foster , J . 2009 . Gold and trace element zonation in pyrite using a laser imaging technique: Implications for the timing of gold in orogenic and Carlin-style sediment-hosted deposits . Economic Geology , 104 : 635 – 668 .
- Large , RR , Bull , SW and Maslennikov , VV . 2011 . A carbonaceous sedimentary source-rock model for Carlin-type and orogenic gold deposits . Economic Geology , 106 : 331 – 358 .
- Mackinnon , TC . 1983 . Origin of Torlesse and related rocks, South Island, New Zealand . Geological Society of America Bulletin , 94 : 967 – 985 .
- Mackinnon , TC and Howell , DG. 1984 . Turbidite facies in an ancient subduction complex: Torlesse Terrane, New Zealand . Geo-Marine Letters , 3 : 211 – 216 .
- Mitchell M , Maw L , Angus PV , Craw D 2006 . The Macraes gold deposit in east Otago . In : Christie AB , Brathwaite RL . Geology and Exploration of New Zealand Mineral Deposits; Australasian Institute of Mining and Metallurgy Monograph 25 : 313 – 318 .
- Mortensen , JK , Craw , D , MacKenzie , DJ , Gabites , JE and Ullrich , T . 2010 . Age and origin of orogenic gold mineralisation in the Otago Schist belt, South Island, New Zealand: constraints from lead isotope and 40Ar/39Ar dating studies . Economic Geology , 105 : 777 – 793 .
- Mortimer , N . 1993 . Jurassic tectonic history of the Otago Schist, New Zealand . Tectonics , 12 : 237 – 244 .
- Paterson , CJ . 1986 . Controls on gold and tungsten mineralization in metamorphic–hydrothermal systems, Otago, New Zealand . Geological Association of Canada Special Paper , 32 : 25 – 39 .
- Petrie , BS , Craw , D and Ryan , CG . 2005 . Geological controls on refractory ore in an orogenic gold deposit, Macraes mine, New Zealand . Mineralium Deposita , 40 : 45 – 58 .
- Pitcairn , IK , Roberts , S , Teagle , DAH and Craw , D . 2005 . Detecting hydrothermal graphite deposition during metamorphism and gold mineralisation. Journal of the Geological Society . London , 162 : 429 – 432 .
- Pitcairn , IK , Teagle , DAH , Craw , D , Olivo , GR , Kerrich , R and Brewer , TS . 2006 . Sources of metals and fluids in orogenic gold deposits: insights from the Otago and Alpine Schists, New Zealand . Economic Geology , 101 : 1525 – 1546 .
- Pitcairn , IK , Olivo , GR , Teagle , DAH and Craw , D . 2010 . Sulfide evolution during prograde metamorphism of the Otago and Alpine Schists, New Zealand . Canadian Mineralogist , 48 : 1267 – 1295 .
- Scott , RJ , Meffre , S , Woodhead , J , Gilbert , SE , Berry , RF and Emsbo , P . 2009 . Development of framboidal pyrite during diagenesis, low-grade regional metamorphism, and hydrothermal alteration . Economic Geology , 104 : 1143 – 1168 .
- Teagle , DAH , Norris , RJ and Craw , D . 1990 . Structural controls on gold-bearing quartz mineralisation in a duplex thrust system, Hyde Macraes shear zone, Otago Schist, New Zealand . Economic Geology , 85 : 1711 – 1719 .
- Thomas , HV , Large , RR , Bull , SW , Maskennekov , VV , Berry , BF , Fraser , R , Froud , S and Moye , R . 2011 . Pyrite and pyrrhotite textures and composition in sedimentary rocks, laminated quartz veins, and gold reefs at the Bendigo mine, Australia: insights for ore genesis . Economic Geology , 106 : 1 – 31 .
- Upton , P and Craw , D . 2009 . Mechanisms of strain rate and reaction dependent permeability in the mid crust of the Southern Alps: insight from 3D mechanical models . Geofluids , 9 : 287 – 302 .