Abstract
A synthesis of published information on mountain uplift and river capture in Otago suggests that the Clutha River catchment has evolved westwards and expanded since the Pliocene. River capture events that facilitated catchment expansion are indicated by sediment provenance, drainage geometry and freshwater fish genetics. The catchment has been partly confined by NW-trending ranges and the Southern Alps to the west, and drainage geometry was disrupted by subsequent growth of NE-trending ranges. Examination of crustal-scale deformation via an established numerical model, which portrays the Otago Schist basement as rheologically weak compared to adjacent greywacke-dominated structural blocks, suggests that uplift geometry was controlled by the inherited Cretaceous boundary between these crustal blocks. Pre-existing faults had relatively minor effects on uplift geometry. A low-relief corridor between Canterbury and Southland permitted genetic connections of freshwater fish populations through to <1 Ma, to the west of the developing Clutha catchment.
Introduction
The Clutha River (A) is one of the largest rivers in New Zealand with a length of c. 340 km, catchment area of nearly 22000 km2 and mean discharge of > 500 m3 s−1 (Murray Citation1975). The river catchment is almost entirely on Otago Schist basement, a lithologically distinctive crustal block in the southern South Island (A, A). The river drains high mountains in the west and passes through a complex topographic system of basins and ranges farther east before emptying into the South Pacific Ocean (A, A). Almost all the topographic features of the Clutha River catchment directly reflect the late Cenozoic and active tectonic processes that are occurring in southern South Island as a result of deformation along the nearby plate boundary, which is defined by the Alpine Fault (A; Jackson et al. Citation1996). Development of these tectonic features has therefore controlled the evolution and geometry of the Clutha River catchment in the late Cenozoic and, more particularly, in the Quaternary.
Figure 1 A, DEM image(used with permission of www.geographx.co.nz) of the southern South Island showing the underlying crustal blocks and their relative crustal strengths. B, Geological terrane map of southern South Island (partly after Mortimer Citation1993; Turnbull Citation2000; Forsyth Citation2001) showing sources of principal rock types relevant to sediment provenance during evolution of the Clutha catchment. Dotted lines show margins of schist belt. Heavy dashed line shows the southern portion of the Miocene lamprophyre dyke swarm (after Cooper et al. Citation1987). The present Clutha River main stem is shown in blue.
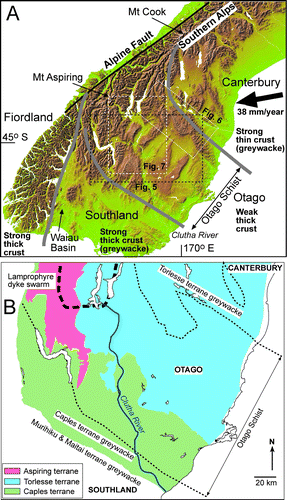
The Clutha catchment hosted most of the alluvial gold that prompted the 19th century Otago gold rush that ultimately produced 8 million ounces (c. 240 tonnes) of gold (Williams Citation1974). The tectonic evolution of the catchment and the mountain ranges within it has controlled the distribution and evolution of the gold placers, with numerous stages of recycling of gold and host sediments (Youngson & Craw Citation1993, Citation1995, Citation1996; Youngson et al. Citation1998; McDonnell & Craw Citation2003; Craw Citation2010). The geology of placer gold in Otago is therefore intimately tied to the growth and diversions of the Clutha catchment as a whole.
Despite the importance of the Clutha River catchment as an economic and geomorphological feature in New Zealand, the overall evolutionary history of that catchment has never been documented from a geological perspective. Numerous studies have addressed the geomorphological development of small parts of the catchment (), but these studies have never been integrated into a catchment-wide evolutionary history. This study addresses this lack of a holistic view of catchment geomorphological development by providing a synthesis of previously published information. Our study integrates published data and interpretations from a wide range of geological settings and scales, from the structure and rheology of the underlying crust, through the structure and evolution of individual mountain ranges, to the effects of interactions of Quaternary glaciation and tectonic features on river drainage geometry. We examine the observed changes in fluvial stratigraphy and sediment provenance variations of the Otago region in the context of a previously published numerical model that evaluates the effects of underlying crustal structure on geometry and relative timing of mountain uplift. In addition, because the Clutha River catchment extends across the South Island from high mountains to the sea, we suggest that the topographic evolution of the catchment has exerted controls on the evolution of biota within that catchment in relation to biota in adjacent catchments. We use biological data (freshwater fish genetics) to elucidate the nature and timing of some stages of the catchment evolution and geological data (sediment provenance and geomorphology) to elucidate the nature and timing of some biological population separation events during catchment evolution.
Table 1 Summary of principal river capture and drainage reorientation events during Quaternary evolution of the Clutha River catchment in Otago, New Zealand. Locations of numbered capture points and abandoned gaps are shown in , .
General setting
The Otago Schist belt, which underlies the Clutha River catchment (A), is a thick pile of Mesozoic metasedimentary rocks that have a gently dipping micaceous metamorphic foliation over most of the exposed area (Mortimer Citation1993; Mortimer et al. Citation2002). The Otago Schist crustal block is lithologically different from the adjacent crustal block of Canterbury (A, B), which is dominated by Mesozoic greywacke (Mackinnon Citation1983). The crust of Southland, on the other side of Otago (A, B), is also dominated by Palaeozoic–Mesozoic greywacke (Mortimer et al. Citation2002). The Otago crustal block has therefore been inferred to have a rheologically weak mid-lower crust that is readily deformed tectonically (Upton et al. Citation2009).
The Otago Schist belt is generally lithologically uniform, consisting of quartzofeldspathic rocks that range in metamorphic grade from pumpellyite-actinolite facies semischists to upper greenschist facies schists. Intercalated metabasites are rare in most of the schist belt but are abundant in a lithologically distinct zone (the Aspiring terrane) in the Southern Alps, where it is centred on Mount Aspiring (A, B; Norris & Craw Citation1987). The Aspiring terrane also contains abundant metacherts, including distinctive piemontite metacherts, and pelitic schists (Norris & Craw Citation1987; Turnbull Citation2000).
The metamorphic grade decreases on the flanks of the schist belt to NE and SW, across a series of Cretaceous normal fault blocks (A; Mortimer Citation1993; Turnbull Citation2000; Forsyth Citation2001; Henne et al. Citation2011). These normal faults are most prominent on the north-eastern flanks (A), where they have been responsible for the sharp decrease in crustal thickness towards Canterbury (Deckert et al. Citation2002; Upton et al. Citation2009; Henne et al. Citation2011). This faulted zone is at least 50 km wide from the Waihemo Fault System to the Waitaki Fault System, with their inland extensions (A). Most of these faults have been reactivated by subsequent events and some are still active, although movement senses and amounts of offset are variable (Forsyth Citation2001; Cox & Barrell Citation2007; Henne et al. Citation2011). Some of the faults have been instrumental in the rise of late Cenozoic mountain ranges with 1–2 km of offset, whereas others have less than 10 m of reactivation motion (Youngson et al. Citation1998; Forsyth Citation2001; Cox & Barrell Citation2007; Henne et al. Citation2011).
Figure 2 A, Shaded topographic image of southern South Island with principal rivers (blue) showing the Clutha River catchment boundary (dashed black line); the main stem of the Clutha River is enhanced in blue. Some of the principal faults that have affected topographic development are indicated with red lines (from Turnbull Citation2000; Forsyth Citation2001; Cox & Barrell Citation2007). Von-Ōreti River capture area (13A,C; ) is indicated. B, Inferred middle Quaternary palaeodrainage geometry for the same region as depicted in A, derived from observations and references in . Rivers are colour-coded for different catchments. The present Clutha River main stem is shown in pale blue for comparison.
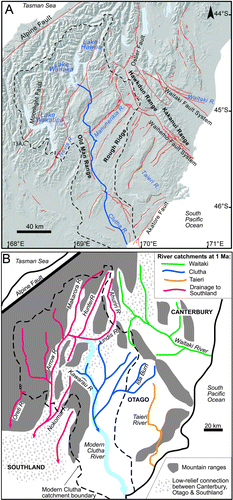
The Otago crustal block is currently undergoing compressional deformation associated with oblique convergence at c. 38 mm yr−1 along the Pacific–Australian plate boundary (Alpine Fault, A; DeMets et al. Citation1994). This plate boundary was initiated in the Miocene, when the Southern Alps on the western edge of the schist block near Mount Aspiring began to rise (A). These mountains are referred to here as the ‘Otago mountains’ to distinguish them from the main Southern Alps chain that developed further NE from Pliocene to Recent (Craw Citation1995). Miocene uplift of the Otago mountains was accompanied by intrusion of structurally-controlled lamprophyre dykes in the mountains (B; Cooper et al. Citation1987; Craw Citation1995). The compressional deformation has subsequently caused folding of the schist to form broad (10–20 km scale) antiforms and synforms of the foliation in the Otago crustal block (NE-trending ranges) and development of faulted ridges in the adjacent greywacke to the NE and SW (NW-trending ranges; A; Jackson et al. Citation1996; Bennett et al. Citation2005, Citation2006).
Early Miocene quartzose fluvial sediments of the Dunstan Formation were deposited on the Otago crustal block, associated with the early Miocene uplift further west (; Douglas Citation1986; Youngson et al. Citation1998), and these sediments were also transported to eastern Southland (; Stein et al. Citation2011). A broad shallow lake, Lake Manuherikia, developed in a broad depression in the basement (Douglas Citation1986) and lacustrine sediments from the lake (Bannockburn Formation) overlie the Dunstan Formation (). The lake was progressively filled with fluvial sediments (Maniototo Conglomerate) in the late Miocene and Pliocene, derived from the NW-trending ranges, especially to the north and east (; Douglas Citation1986; Youngson et al. Citation1998). The Miocene and Pliocene sediments have been folded and faulted by on-going deformation, with erosion of sediments from rising NE-trending basement ridges into adjacent valleys to form a veneer of low-relief Quaternary fluvial gravels (Youngson et al. Citation1998; Turnbull Citation2000). Moraines and glaciofluvial gravels derived from the mountains to the west have contributed to the Quaternary sedimentary veneer (Turnbull Citation2000) that fills valley floors in the central and western parts of Otago (A, ).
Figure 3 Tectonic stratigraphic columns for Miocene–Recent sedimentary sequences in central Otago and Southland, with their provenances indicated. The columns are generalised for these two areas, and do not represent specific localities. The evolution of the Clutha River catchment in this context is indicated in a box. Time axis is not to scale.
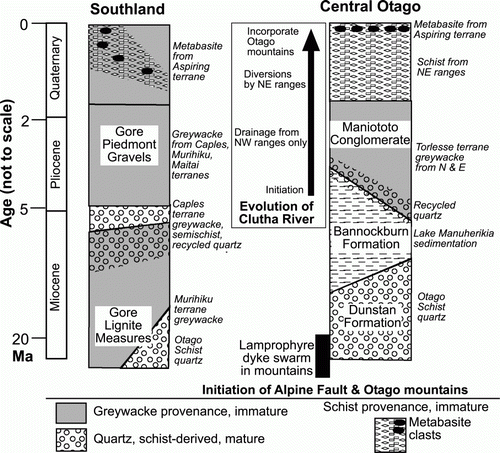
Methods
Since this paper is a synthesis of previous work on a wide range of topics and scales, we rely heavily on published methods and results in the numerous references included here. We discuss the model of crustal structure and its implications at the beginning of our account, because that can be readily integrated with the established tectonic stratigraphy and structure presented in the previous section. We then present an integrated account of river drainage reorientations in the context of both the model and the regional structure and stratigraphy, combined with individual case studies outlined and referenced in .
The effects of large-scale crustal structure in a transpressional regime on surface topography have been evaluated by numerical modelling using FLAC3D version 3.1 (Itasca Citation2006; Upton et al. Citation2009). Boundary conditions and key parameter values for this modelling have been previously documented in detail (Upton et al. Citation2009; Koons et al. Citation2012) for the Canterbury–Otago crustal block couple. The model () examines the effects on topography of a pre-existing crustal discontinuity at a high angle to the transpressional boundary, for a boundary between thin strong mid-lower crust and thick weak mid-lower crust.
Figure 4 Map view of results of a numerical model of topographic development in heterogeneous crust with an inherited crustal boundary at a high angle to a transpressional plate boundary (plate vector indicated). Model topographic results adapted from models with boundary conditions defined by Upton et al. (Citation2009). Resultant uplift zones relevant to Otago are 1: mountains parallel to the plate boundary; 2: range perpendicular to the plate boundary at the crustal discontinuity (horizontal dotted arrow); and 3: ranges emanating perpendicular to 2 (parallel to vertical dotted arrow). Model space is equivalent to Canterbury and Otago crustal blocks (A), with the right margin equivalent to Otago east coast.
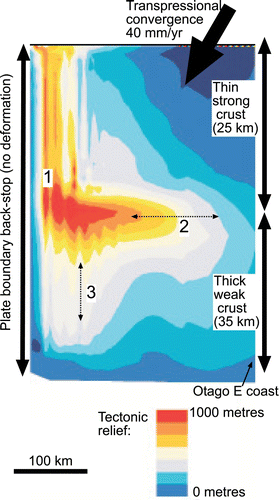
The geological history of the Otago area can be deduced from tectonic stratigraphy of the late Cenozoic sedimentary sequence (Youngson et al. Citation1998). This history has been elucidated by observations on provenance of distinctive sedimentary clasts through the sequence (Youngson et al. Citation1998; McDonnell & Craw Citation2003; Stein et al. Citation2011). Results of past studies, combined with observations made as part of this study, have been incorporated into the deductions throughout this paper. The variations in sediment provenance are summarised in and , with principal geological features that contribute distinctive clasts summarised in B.
River capture events that are an important part of the Clutha River evolutionary history have been documented by a wide variety of geological methods, as summarised in . Details of the methods, and data to support the various capture events, are contained in the references cited in . The locations of key geographic points in these river capture events are indicated on A, , and . For each capture event, there is a ‘capture point’ (denoted C in and the various figures) at which two previously separate rivers have joined. For each capture event, there is also an ‘abandoned gap’ (denoted A in and the various figures) through which a river previously passed but is now a dry topographic divide between stream catchments. Middle Quaternary palaeodrainage geometry of Otago, inferred from the observations and references in , is presented in B.
Endemic freshwater-limited Galaxias fish species are widespread in New Zealand and, unlike their close relatives, they do not spend part of their life cycle in the sea (McDowall Citation2000; Burridge et al. Citation2011). At least nine species of freshwater-limited Galaxias have been identified on the basis of morphology and genetics, although not all of these species have been formally described and several have informal names (McDowall Citation2000; Waters & Wallis Citation2001; Burridge et al. Citation2011). Because the fish are freshwater-limited, the distribution of species between different catchments provides useful biogeographical information on historic connections between those catchments (Waters et al. Citation2001; Burridge et al. Citation2006, Citation2007, Citation2008; Craw et al. Citation2007a, Citationb, Citation2008a, Citationb). Fish distributions within catchments have been extensively determined but generally have little biogeographical significance because of localised predation by introduced trout, habitat preferences and anthropogenic habitat modification (McDowall Citation2000; Waters et al. Citation2001; Burridge et al. Citation2006, Citation2007, Citation2008). However, some genetically distinct populations of one species, Galaxias ‘species D’, occur in different parts of the Clutha catchment (; Waters et al. Citation2001).
Distributions of these freshwater-limited fish have been used extensively for studies of freshwater biogeography and drainage evolution, including the Canterbury, Otago and Southland regions (Waters et al. Citation2001, Citation2010; Burridge et al. Citation2006, Citation2007, Citation2008, Citation2011). Occurrences of distinctive species in different catchments imply freshwater connections between those catchments at some time (Waters et al. Citation2001; Burridge et al. Citation2006, Citation2007, Citation2008). Conversely, different but related fish species in adjacent catchments imply reproductive isolation for sufficient time for the separate species to evolve (Waters et al. Citation2010; Burridge et al. Citation2011). Similarly, genetic differences between populations of the same species in different catchments also imply some degree of reproductive isolation (Waters et al. Citation2001, Citation2010; Burridge et al. Citation2006, Citation2007, Citation2008). Freshwater Galaxiis species distribution is therefore a useful tool for semi-quantitative documentation of river capture, as outlined here for the Clutha catchment.
In addition to providing evidence of past river capture events, freshwater Galaxias species can be used to provide an estimate of the time since the capture event (Waters et al. Citation2001; Craw et al. Citation2008a). Fish populations separated by capture events immediately begin to diverge genetically because of spontaneous mutations of DNA, and the amount of this genetic divergence increases with time (Waters et al. Citation2001; Craw et al. Citation2008a). This time-dependence of genetic divergence has led to the concept of the ‘molecular clock’ (Waters et al. Citation2001; Bromham & Penny Citation2003; Burridge et al. Citation2006, Citation2007, Citation2008). A molecular clock has been calibrated for mitochondrial DNA in New Zealand freshwater Galaxias species for the Quaternary (Waters et al. Citation2001; Burridge et al. Citation2006, Citation2007, Citation2008; Craw et al. Citation2008a) and projected back to the Miocene for both freshwater and sea-going species (Burridge et al. Citation2011). Relative uncertainties in age estimates in the middle to late Quaternary are c. 33% (Craw et al. Citation2008a). Some of the river capture events relevant to Quaternary Clutha River catchment evolution in this synthesis have been documented and dated with Galaxias genetics (; ).
Effects of crustal structure on Otago geomorphology
The Otago crustal block has numerous faults inherited from its Mesozoic geological history, and many of these have been reactivated in the Cenozoic–Recent deformation regime (A). These faults provide zones of rock weakness that can influence topographic development associated with the current deformation. However, the underlying crustal structure and the major regional differences in crustal strength (A) are also likely to have some influence on topographic development. We address this large-scale structural and topographic evolution with the numerical model from which the numerous pre-existing faults have been excluded, and only the major Canterbury–Otago crustal boundary is involved (Upton et al. Citation2009).
Upton et al. (Citation2009) present the case for rheological differences in the ductile mid-lower crust between Canterbury and Otago. They use several lines of evidence, including (a) the presence of garnet-grade schists exposed in Otago compared to low-grade greywacke in Canterbury, signifying considerably more pre-Cenozoic exhumation in Otago compared to Canterbury; (b) estimates of pre-collisional crustal thickness based on present-day crustal thickness at the edge of the continent being greater in Otago than Canterbury (Eberhart-Phillips & Bannister Citation2002; Scherwath et al. Citation2003; Van Avendonk et al. Citation2004); and (c) consideration of thermal re-equilibration following Cretaceous thickening of the crustal pile in Otago and thinning of the Canterbury crust. They interpret strong thin (~20 km) crust in Canterbury compared to weak thick (30–35 km) crust in Otago and their regional-scale model forms the basis of the simulation presented here.
The numerical model was run with rock strength parameters (Upton et al. Citation2009) that reflect impingement of a block of thin strong crust (representative of Canterbury) on a block of thick weak crust (representative of Otago) during dextral transpression at a plate boundary against a non-deforming backstop (Australian Plate). Note that the strength difference between these two blocks is in the ductile mid-lower crust, not the brittle upper crust (Upton et al. Citation2009). The boundary between the thin strong crust and thick weak crust is perpendicular to the plate boundary in the model. The numerical model results () show that three principal geomorphic features arise as a result of the transpressional deformation with inhomogeneous crust: mountains parallel to the plate boundary; high ranges perpendicular to the plate boundary at the crustal discontinuity; and ridges extending perpendicular to these high ranges along the crustal discontinuity. The latter ridges develop after the other mountains formed in the model.
The model results () are representative of topographic development of the Southern Alps and the north-eastern boundary of the Otago crustal block (A) during the Pliocene and Quaternary, with the real situation rotated c. 50° clockwise compared to the model. The model mountains parallel to the plate boundary are equivalent to the Otago mountains, the uplift perpendicular to the plate boundary is equivalent to the NW ranges between Canterbury and Otago (A, ) and the ridges perpendicular to these NW ranges are equivalent to the NE ranges on the Otago crustal block (A). An important conclusion from this model is that large-scale differences in crustal thickness and strength may be all that is needed to facilitate the complex topography of Otago and that pre-existing faults (A) may only be of secondary significance.
Rise of NW ranges
The numerical model () predicts that a NW-trending range should have developed first, near the crustal discontinuity between Otago and Canterbury. A broadly similar crustal discontinuity occurs between Otago and the rheologically strong crustal blocks of Southland and Fiordland (B). The stratigraphic evidence supports inferences of the model, in that the first immature sediments associated with central Otago uplift were shed from NW-trending ranges. The Old Man Range and associated ranges on the SW side of the Otago block rose in the middle to late Miocene, and almost completely separated Otago drainages from eastern Southland (Stein et al. Citation2011). Sediment transported directly from Otago ceased to appear in eastern Southland at that time, and was replaced by detritus from the rising intervening ranges including the Old Man Range. This detritus included greywacke and semischist from the south-western margin of the schist belt and quartzose material derived from older sedimentary deposits resting on the rising ranges (; Isaac & Lindqvist Citation1990; Stein et al. Citation2011).
The stratigraphic record suggests that the NW-trending ranges between Otago and Canterbury (A) rose in the late Miocene or early Pliocene, after initiation of the Old Man Range uplift (; Douglas Citation1986). The Kakanui, Hawkdun and St Bathans ranges (, ) rose as separate ridges with intervening topographic lows (Craw et al. Citation2007a). These ranges consist of greywacke and semischist and have formed along, and were locally controlled by, the normal faults that marked the crustal boundary between Otago and Canterbury crustal blocks (Upton et al. Citation2009; Henne et al. Citation2011). The ranges were initiated and developed separately because of this structural control by reverse reactivation of these pre-existing normal faults ().
Figure 6 DEM of the NW-trending Hawkdun, St Bathans and Kakanui ranges showing points relevant to river capture events (; red numbers). Black lines are Cretaceous normal faults that have been locally reactivated as reverse faults. DP: Dansey Pass.
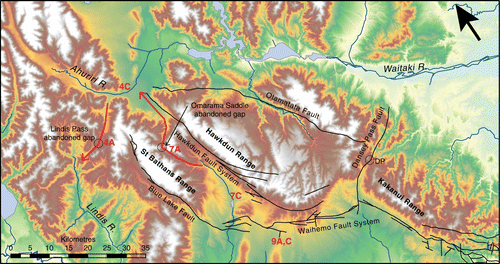
The rising ranges along the Otago–Canterbury crustal boundary shed large volumes of immature debris towards the south, to form a semi-continuous braid plain over much of inland Otago. This sediment, the Maniototo Conglomerate, filled the remnants of Lake Manuherikia and buried the Miocene sediments (). The initial stages of this erosion and sedimentation were marked by pulses of mature quartzose debris recycled from Miocene sediments uplifted on the ranges (; Youngson et al. Citation1998). This quartzose debris is mixed with immature greywacke and semischist clasts, which rapidly dominate up-section and form most of the Maniototo Conglomerate unit ().
Figure 7 DEM showing south-western drainage pattern in the Pliocene and early Quaternary. Major drainages flow to the south. Red numbers show points relevant to river capture events (). Dashed line shows the approximate geometry of glacial moraine (after Turnbull Citation2000) that was instrumental in the redirection of the Clutha River from a route through Thomson Saddle (6A) to its present-day route through the Cromwell Gorge (6C).
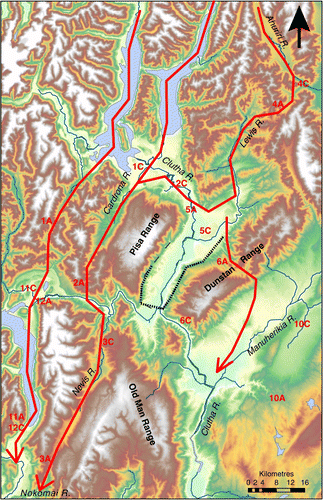
The NW-trending ranges along the Otago–Canterbury boundary have continued to rise and expand laterally, and they are still tectonically active features. The ongoing lateral expansion has caused the ranges to converge to form a continuous topographic barrier (A, ). This barrier continues to the west of the St Bathans Range, where that range has become continuous with the more easterly ranges of the Southern Alps (A, A, ). The last gaps in this barrier occurred at what is now Lindis Pass (4A; ; ) and Ōmarama Saddle (7A; ; ). These last gaps in the barrier were the final stream linkages between the Waitaki River catchment and what is now the Clutha river catchment (, ). The Lindis Pass gap (4A; ) represents a former channel of the Ahuriri River (4C, ) that flowed to the south, whereas the Ōmarama Saddle gap (7A, ) represents a former channel of what is now the upper Manuherikia River (7C, ) that flowed north (Craw et al. Citation2007a). Closure by river capture of both of these former connections between Otago and Canterbury resulted in isolation of populations of Galaxias paucispondylus, and this occurred at about 800 ka (; Craw et al. Citation2007a). A topographic low at Dansey Pass (DP; ) represents the site of impingement of the Kakanui and Hawkdun Ranges, but no information is available on the relative timing of closure of this gap which is no longer in the Clutha catchment.
Figure 8 Summary evolutionary trees (after Burridge et al. Citation2006, Citation2007, Citation2008; Craw et al. Citation2007a, Citationb; Waters et al. Citation2001, Citation2010) for mtDNA of some non-migratory galaxiid fish affected by the tectonic evolution discussed in this study. The scale bars shows the magnitude of relative genetic differences between the galaxiids in different river catchments and the approximate timing of separation of populations (after Craw et al. Citation2007a, Citation2008a). Numbers on trees refer to river capture events in .
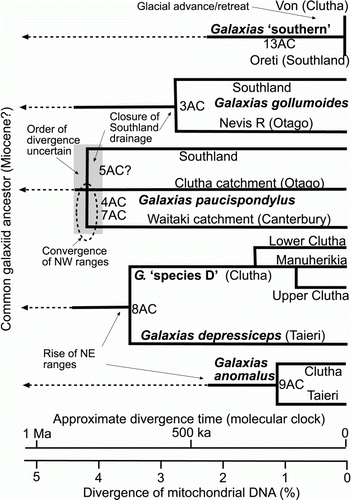
Rise of NE ranges
Antiformal ranges with a NE trend dominate the present topography of the Otago block (A, A, ), and these ranges began to rise in the Quaternary (Jackson et al. Citation1996; Bennett et al. Citation2005, Citation2006). Sedimentary and structural observations, supported by our modelling (), show that the NE ranges evolved after the NW ranges as minor ridges extending from the dominant NW ranges (Koons et al. Citation2012). Intervening NE-trending basins are synformal, and the folded topography has formed above NW-dipping reverse faults which have only minor surface expression, typically on valley floors on the SE sides of ranges (Jackson et al. Citation1996; Bennett et al. Citation2005, Citation2006). The antiformal ranges were initiated as domal structures that progressively expanded vertically and laterally, and this expansion is on-going (Jackson et al. Citation1996; Bennett et al. Citation2005, Citation2006).
Figure 9 Generalised catchment maps of Otago and adjacent regions showing evolution of the extent of the Clutha catchment through the Quaternary. Ages are approximate. A, Early Quaternary (c. 1.5 Ma). B, Late Quaternary (c. 500 ka). C, Present day. The present main stem of the Clutha River and principal modern lakes are shown on all three maps for reference. A middle Quaternary catchment map, for the period between A and B, is shown with specific river courses in B.
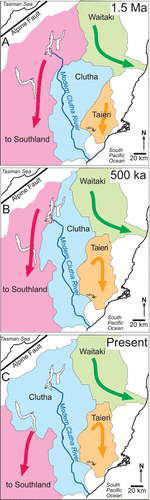
The rise of the antiformal ranges disrupted the Maniototo Conglomerate braid plain, and streams emanating from the NW-trending ranges were channelled into the intervening synformal basins (Jackson et al. Citation1996; Youngson & Craw Citation1995; Youngson et al. Citation1998). The basins are still structural depressions and not erosional features, and only minor erosion has occurred locally in the basins. Instead, the basins have become repositories for sediments derived from both the NW and NE ranges, and these Quaternary sediments rest unconformably on folded Miocene and Pliocene deposits (). The Quaternary sediments are dominated by immature schist debris from adjacent NE ranges, although greywacke clasts derived from NW ranges can dominate in axial streams because these clasts are more resistant to comminution (McDonnell & Craw Citation2003).
The development of NE-trending Rough Ridge () was one of the most significant features of this stage of Clutha catchment evolution, as this range came to form the eastern boundary of the catchment (A). Rough Ridge is a complex series of NE-trending antiforms that have merged as they grew over the past million years (Jackson et al. Citation1996; Bennett et al. Citation2005, Citation2006). Before the rise of these antiforms, southward drainage from the Kakanui Range (, ) was part of the Maniototo Conglomerate braid plain that fed the Clutha River (Youngson et al. Citation1998). Rough Ridge disrupted this braid plain and diverted part of it into what is now the Taieri River catchment (). Closure of stream connections across what is now Rough Ridge (8A; , ) is thought to have caused isolation of populations of Galaxias fish () which are now considered to be distinct species: Galaxias depressiceps and Galaxias ‘species D’ (the latter not yet formally described; Waters et al. Citation2010). This species isolation occurred c. 500 ka (; Craw et al. Citation2007a). Continued northwards propagation of one of the Rough Ridge antiforms caused recapture of the headwaters of the Ida Burn catchment (9A, C; ; , ) by the Clutha catchment, accompanied by redistribution of Galaxias anomalus at c. 60 ka (; Craw et al. Citation2007a).
Similar complex range development to Rough Ridge has affected the Pool Burn catchment (10A, C; ; , ), which originally flowed southwards as part of the Maniototo Conglomerate braid plain. Range uplift has diverted the Pool Burn northwards, then west to the Manuherikia River (; Jackson et al. Citation1996). The westwards reach of the Pool Burn catchment has been forced to cut a deep basement gorge as the composite antiforms of the Raggedy Range have grown and converged (Jackson et al. Citation1996). These processes have merely reorganised the drainage geometry within this part of the Clutha catchment. Rise and expansion of the uplands at the head of the Taieri River and Pool Burn (10A; , ), coupled with the continued rise of the Old Man Range, has caused the Clutha River to cut gorges through basement rocks in its lower reaches ().
The Dunstan Range () initially formed as two NE-trending ridges, separated at what is now Thomsons Saddle (6A; , ). This topographic low has been partly controlled by erosion along NW-striking basement structural features that have relatively soft fault rocks (Cox et al. Citation2006). The main Clutha River flowed through this gap, joining the Manuherikia River more than 10 km upstream of their present confluence (). Distinctive basanite cobbles and titaniferous magnetite and ilmenite sand occur in this palaeo-confluence area, and these were derived from the lamprophyre dyke swarm near Lake Hāwea (; Youngson et al. Citation1998). Middle Quaternary glacial advances extended from the Southern Alps down the west side of the Dunstan Range and these advances, combined with on-going growth and impingement of the two parts of the Dunstan Range, may have diverted the Clutha River to its present course (6C; , ).
Drainage to the SW
The Clutha River catchment that evolved during the rise of the NW ranges and initial rise of the NE ranges had its headwaters near the present Lindis River, not the Southern Alps in the west as at present (A, B, B, ). Drainage from the Southern Alps was towards the SW in a set of subparallel rivers that extended from the mountains of Canterbury to Southland (; Waters et al. Citation2001; McDonnell & Craw Citation2003). The south-western drainage was facilitated by the uplift of the Southern Alps and erosion along mountain-parallel faults in the eastern mountains (Koons Citation1995). This mountain-parallel topography immediately east of the plate boundary is also apparent in the numerical model results (; Upton et al. Citation2009).
Remnants of sediments deposited by one of these rivers are preserved in the Cardrona valley (). These extensive deposits of Pliocene gravel with greywacke clasts were transported at least 70 km from the mountains to the north, possibly from as far away as the Mt Cook area (. 1A; McDonnell & Craw Citation2003). A low-relief zone, dominated by fluvial plains, apparently formed a corridor immediately east of the Southern Alps all the way from Canterbury to Southland (B). The rise of the NE-trending Pisa Range in the Quaternary cut off southwards drainage in the Cardrona valley (2A; , , ) and forced the upper reaches of this river system to flow north (B) and ultimately join the Clutha catchment (2C; , ). The lower reaches of this river system originally passed through what are now the Nevis and Nokomai valleys to Southland (, ). Growth and impingement of NE ranges flanking these valleys also cut this part of the river system, forcing what is now the Nevis River to flow NE (3A,C; ; , ; Waters et al. Citation2001). This latter river capture event trapped a population of Galaxias gollumoides, a widespread Southland fish species, in the Nevis River for the past 300–500 ka (Waters et al. Citation2001; Craw et al. Citation2008a).
To the west of the Hunter–Cardrona–Nevis–Nokomai river system, another parallel river also drained SW down what is now Lakes Wānaka and Wakatipu (; B, ). This river system has also been segmented by Quaternary events (1A,C; 11A,C; 12A,C; ; ). The low divide between Lake Wānaka and the Clutha catchment (1C; ) was breached during glaciation in the late Quaternary. The timing of this breach is indicated by the first appearance of abundant Aspiring terrane metabasites and piemontite metacherts in c. 60 ka glaciofluvial sediments in the downstream Clutha catchment (; Turnbull Citation2000; McDonnell & Craw Citation2003).
Drainage from the Southern Alps towards the SW down Lake Wakatipu to Southland (B, ) has occurred extensively in the Quaternary, and persisted to the end of the last glaciation (c. 12–15 ka; Turnbull Citation2000). A low moraine ridge (<100 m high) at the south end of Lake Wakatipu is all that currently prevents this drainage continuing. Large volumes of sediment from the Southern Alps, including Aspiring terrane rocks, have been discharged to Southland via this pathway throughout the Quaternary (). The same Quaternary glacial activity and postglacial river drainage facilitated development and deep erosion of the Kawarau River that flows east across the old SW-draining river courses to join the Clutha River on the west side of the Dunstan Range (, ). The Lake Wakatipu and Kawarau River portion of the Clutha catchment is still a tenuous component that could be removed by minor erosion at the south end of Lake Wakatipu.
Quaternary glacial advances in the Lake Wakatipu basin repeatedly crossed a low topographic divide between the Von River (Clutha catchment) and Southland (13A,C; , A; Turnbull Citation2000). The last breach of this divide was c. 12 ka, after which populations of Southland Galaxias ‘southern’ and G. gollumoides became isolated in the Von River (; Burridge et al. Citation2007; Craw et al. Citation2007b).
While the genetic connection between the Clutha and Southland populations of G. paucispondylus was severed approximately 800 ka, some subsequent freshwater connections between these drainages were employed by several galaxiid species (). Catchment-wide migration of these galaxiids has apparently been prevented by habitat and substrate variations, lakes, glaciers and/or glacier-fed waters with high suspended sediment contents.
Discussion and conclusions
The Clutha River catchment has developed on the Otago Schist crustal block as a direct result of the underlying crustal structure: a block of relatively thick and weak schist that is being deformed between blocks of comparatively thin and strong crust during dextral transpression. The boundaries between these crustal blocks were inherited from the Mesozoic tectonic development of southern South Island, and are defined by zones of NW-striking normal faults (Deckert et al. Citation2002; Upton et al. Citation2009; Henne et al. Citation2011). These pre-existing faults affected the development of NW-trending ranges during the Miocene and Pliocene, although the model () suggests that the underlying crustal structural differences provided the first-order control on range development.
The development of NW-trending ranges on both sides of the Otago crustal block resulted in focusing of stream drainage into the Otago block as the ranges grew. The ranges formed topographic barriers between central Otago and the crustal blocks of Canterbury and Southland (A, A). The rise of these ranges and the associated filling of Lake Manuherikia marked the initiation of the Clutha River as a significant Otago river draining to the SE. This river catchment developed in size as the ranges grew through the late Miocene and into the Pliocene (). Significant offshore sedimentation in the Bounty Trough 1000 km to the SE of Otago resumed at about 3.5 Ma after a mid–late Miocene hiatus, probably facilitated by Lake Manuherikia (Marsaglia et al. Citation2011).
The NW ranges formed the main boundaries to the Clutha River catchment in the Quaternary, as they still do, and the subsequent rise of the NE ranges was responsible for the internal complexity of the current catchment drainage geometry between the NW range boundaries (A, A). As the NE ranges rose, they forced streams either to migrate laterally or, if lateral migration was obstructed, to cut gorges on the schist basement (Jackson et al. Citation1996; Craw et al. Citation2007a). When ranges or sections of ranges impinged on each other, some streams were dammed and forced to flow in different directions, commonly resulting in river capture (). River capture was locally facilitated by glacial advances from the western mountains ().
The Clutha River catchment that developed between the NW-trending ranges, interrupted by the rising NE ranges, drained only the central and eastern parts of the Otago Schist block. Streams from the high mountains to the west drained mainly to the SW, avoiding the Clutha catchment (A, ). This south-westwards drainage was progressively fragmented by river capture events that allowed the Clutha River catchment to expand westwards (). Progressive growth and westward expansion of the Clutha catchment through the Quaternary is summarised in A–C.
The SW drainage from the Southern Alps, and fragmentation of that drainage that led to river capture and expansion of the Clutha River catchment during the Quaternary (A–C), ensured that there were periodic river connections between Otago and Southland through the Pliocene and into the Quaternary. These river connections facilitated genetic exchange among populations of Galaxias paucispondylus between Otago and Southland via a low-relief corridor of fluvial plains (B), but these genetic connections ceased at c. 800 ka (). One point of this severance occurred at capture of what is now the Lindis River by the Clutha River (5A,C; , ), the details of which are unknown. Subsequent river connections between Otago and Southland were dominated by glacial activity which apparently prevented migration of G. paucispondylus. The timing of severance of G. paucispondylus connections between Otago and Southland is similar to that of severance of similar connections between Otago and Canterbury (Waitaki catchment) by the convergence of the NW-trending ranges (, ). Prior to this time, it is likely that there were sufficient stream connections between the Waitaki catchment through Otago to Southland to maintain genetic connectivity across the whole region (B, ).
The middle Quaternary corridor of river connections and associated fish genetic connections between Canterbury, north-western Otago and Southland existed between the main zones of tectonic uplift: the Southern Alps to the west and central Otago to the east (A, B). Drainages of these different tectonic uplift zones have become connected only in the late Quaternary as the Clutha River catchment expanded westwards (A–C). The Otago-wide extent of the Clutha River catchment, from high mountains to the sea, is therefore a relatively young phenomenon (<100 ka).
The Clutha River hosts three genetically distinct groups of Galaxias ‘species D’: in the upper catchment near Lakes Wānaka and Hāwea; in the middle of the catchment (Manuherikia River); and in the lower catchment at the south end of the Old Man Range (, ; Waters et al. Citation2001). These observations imply that the fish populations have been isolated within the Clutha catchment, presumably by habitat restrictions (Waters et al. Citation2001). We suggest that late Quaternary westward expansion of the Clutha catchment (, B, C) provided new habitat for colonisation of the upper Clutha from the middle reaches c. 100 ka (), and that the colonising populations have remained isolated by the intervening gorge through the rising Dunstan Range (6C; ) since then. The genetically distinct populations in the middle and lower Clutha catchment have apparently been isolated for even longer, possibly >200 ka (). We infer that the on-going rise of the Old Man Range in the late Quaternary has enhanced the gorge between these latter populations () and has limited their genetic contact. In addition to catchment expansion the Clutha River has undergone tectonically driven segmentation into distinct reaches, and this segmentation is on-going.
Acknowledgements
This study was supported financially by Marsden Fund, ISAT Linkages Fund administered by the Royal Society of New Zealand, University of Otago and GNS Science. Discussions with Alan Cooper, Doug Coombs, Chuck Landis, Richard Norris, Ian Turnbull and John Youngson were helpful in developing some of our ideas. Thorough reviews by K Marsaglia and D Barrell were helpful in improving the manuscript. Helpful and practical input from Associate Editor Simon Cox substantially enhanced the presentation.
References
- Bennett ER , Youngson JH , Jackson JA , Norris RJ , Raisbeck GM , Yiou F , Fielding E 2005 . Growth of South Rough Ridge, Central Otago, New Zealand: using in situ cosmogenic isotopes and geomorphology to study an active blind reverse fault . Journal of Geophysical Research 110 , B020404 . doi: 10.1029/2004JB003184
- Bennett , ER , Youngson , JH , Jackson , JA , Norris , RJ , Raisbeck , GM and Yiou , F . 2006 . Combining geomorphic observations with in situ cosmogenic isotope measurements to study anticline growth and fault propagation in central Otago, New Zealand . New Zealand Journal of Geology & Geophysics , 49 : 217 – 231 . doi: 10.1080/00288306.2006.9515161
- Bromham , L and Penny , D . 2003 . The modern molecular clock . Nature Reviews Genetics , 4 : 216 – 224 . doi: 10.1038/nrg1020
- Burridge , CP , Craw , D and Waters , JM . 2006 . River capture, range expansion, and cladogenesis: the genetic signature of freshwater vicariance . Evolution , 60 : 1038 – 1049 .
- Burridge , CP , Craw , D and Waters , JM . 2007 . An empirical test of freshwater vicariance via river capture . Molecular Ecology , 16 : 1883 – 1895 . doi: 10.1111/j.1365-294X.2006.03196.x
- Burridge , CP , Craw , D , Fletcher , D and Waters , JM . 2008 . Geological dates and molecular rates: Fish DNA sheds light on time dependency . Molecular Biology and Evolution , 25 : 624 – 633 . doi: 10.1093/molbev/msm271
- Burridge , CP , McDowall , RM , Craw , D , Wilson , MVH and Waters , JM . 2011 . Marine dispersal as a pre-requisite for Gondwanan vicariance among elements of the galaxiid fish fauna . Journal of Biogeography , 39 : 306 – 321 . doi: 10.1111/j.1365-2699.2011.02600.x
- Cooper , AF , Barreiro , BA , Kimbrough , DL and Mattinson , JM . 1987 . Lamprophyre dike intrusion and the age of the Alpine fault, New Zealand . Geology , 15 : 941 – 944 . doi: 10.1130/0091-7613(1987)15%3C941:LDIATA%3E2.0.CO;2
- Cox , L , MacKenzie , DJ , Craw , D , Norris , RJ and Frew , R . 2006 . Structure and geochemistry of the Rise & Shine Shear Zone mesothermal gold system, Otago Schist, New Zealand . New Zealand Journal of Geology and Geophysics , 49 : 429 – 442 . doi: 10.1080/00288306.2006.9515179
- Cox SC , Barrell DJA 2007 . Geology of the Aoraki area . Institute of Geological & Nuclear Sciences 1:250 000 geological map 15. 1 sheet + 71 pp . Lower Hutt , , New Zealand . Institute of Geological & Nuclear Sciences Ltd .
- Craw , D . 1995 . Reinterpretation of the erosion profile across the southern portion of the Southern Alps, Mt Aspiring area, Otago, New Zealand . New Zealand Journal of Geology and Geophysics , 38 : 501 – 507 . doi: 10.1080/00288306.1995.9514676
- Craw , D . 2010 . Delayed accumulation of placers during exhumation of orogenic gold in southern New Zealand . Ore Geology Reviews , 37 : 224 – 235 . doi: 10.1016/j.oregeorev.2010.03.006
- Craw , D , Burridge , C and Waters , J . 2007a . Geological and biological evidence for drainage reorientation during uplift of alluvial basins, central Otago, New Zealand . New Zealand Journal of Geology and Geophysics , 50 : 367 – 376 . doi: 10.1080/00288300709509844
- Craw , D , Burridge , C , Anderson , L and Waters , JM . 2007b . Late Quaternary river drainage and fish evolution, Southland, New Zealand . Geomorphology , 84 : 98 – 110 . doi: 10.1016/j.geomorph.2006.07.008
- Craw , D , Burridge , C , Norris , RJ and Waters , JM . 2008a . Genetic ages for Quaternary topographic evolution: a new dating tool . Geology , 36 : 19 – 22 . doi: 10.1130/G24126A.1
- Craw , D , Burridge , CP , Upton , P , Rowe , DL and Waters , JM . 2008b . Evolution of biological dispersal corridors through a tectonically active mountain range in New Zealand . Journal of Biogeography , 35 : 1790 – 1802 . doi: 10.1111/j.1365-2699.2008.01936.x
- Deckert , H , Ring , U and Mortimer , N . 2002 . Tectonic significance of Cretaceous bivergent extensional shear zones in the Torlesse accretionary wedge, central Otago Schist, New Zealand . New Zealand Journal of Geology and Geophysics , 45 : 537 – 547 . doi: 10.1080/00288306.2002.9514990
- DeMets , C , Gordon , RG , Argus , D and Stein , S . 1994 . Effect of recent revisions to the geomagnetic reversal time scale on estimates of current plate motion . Geophyiscal Research Letters , 21 : 2191 – 2194 . doi: 10.1029/94GL02118
- Douglas BJ 1986 . Lignite resources of Central Otago . N.Z. Energy Research and Development Committee, University of Auckland , Auckland , , New Zealand , Publication P104 . 368 p.
- Eberhart-Phillips D , Bannister S 2002 . Three-dimensional crustal structure in the Southern Alps region of New Zealand from inversion of local earthquake and active source data . Journal of Geophysical Research 107 ( B10 ): 2262 . doi: 10.1029/2001JB000567
- Forsyth PJ 2001 . Geology of the Waitaki area . Institute of Geological & Nuclear Sciences 1:250 000 geological map 19. 1 sheet + 64 pp . Lower Hutt , , New Zealand . Institute of Geological & Nuclear Sciences Ltd .
- Henne , A , Craw , D and MacKenzie , D . 2011 . Structure of the Blue Lake Fault Zone, Otago Schist, New Zealand . New Zealand Journal of Geology and Geophysics , 54 : 311 – 328 . doi: 10.1080/00288306.2011.577080
- Isaac , M and Lindqvist , JK . 1990 . Geology and lignite resources of the Eastern Southland Group, New Zealand . New Zealand Geological Survey Bulletin , 101 : 202 p
- Itasca 2006 . FLAC3D (Fast Lagrangian Analysis of Continua in 3 Dimensions) Version 3.1 User's Guide. Minneapolis, Minnesota, Itasca Consulting Group Inc.
- Jackson , J , Norris , RJ and Youngson , JH . 1996 . The structural evolution of active fault and fold systems in Central Otago, New Zealand: evidence revealed by drainage patterns . Journal of Structural Geology , 18 : 217 – 234 . doi: 10.1016/S0191-8141(96)80046-0
- Koons , PO . 1995 . Modeling the topographic evolution of collisional belts . Annual Review of Earth and Planetary Sciences , 23 : 375 – 408 . doi: 10.1146/annurev.ea.23.050195.002111
- Koons PO , Upton P , Barker AD 2012 . The influence of mechanical properties on the link between tectonic and topographic evolution . Geomorphology 29 : doi: 10.1029/2009TC002463
- Mackinnon , TC . 1983 . Origin of Torlesse and related rocks, South Island, New Zealand . Geological Society of America Bulletin , 94 : 967 – 985 . doi: 10.1130/0016-7606(1983)94%3C967:OOTTTA%3E2.0.CO;2
- Marsaglia , KM , Martin , CE , Kautz , CQ , Shapiro , SA and Carter , L . 2011 . Linking a late Miocene-Pliocene hiatus in the deep-sea Bounty Fan off South Island, New Zealand, to onshore tectonishm and lacustrine sediment storage . Geosphere , 7 : 305 – 312 . doi: 10.1130/GES00621.1
- McDonnell , M and Craw , D . 2003 . Stratigraphy and provenance of Pliocene greywacke-bearing conglomerate, Cardrona valley, Otago, New Zealand . New Zealand Journal of Geology & Geophysics , 46 : 425 – 436 . doi: 10.1080/00288306.2003.9515018
- McDowall RM 2000 . The Reed Field Guide to New Zealand Freshwater Fishes . Reed Auckland .
- Mortimer , N . 1993 . Jurassic tectonic history of the Otago Schist, New Zealand . Tectonics , 12 : 237 – 244 . doi: 10.1029/92TC01563
- Mortimer , N , Davey , F , Melhuish , A , Yu , J and Godfrey , NJ . 2002 . Geological interpretation of a deep seismic reflection profile across the Eastern Province and Median Batholith, New Zealand: crustal architecture of an extended Phanerozoic convergent orogen . New Zealand Journal of Geology and Geophysics , 45 : 349 – 363 . doi: 10.1080/00288306.2002.9514978
- Murray , DL . 1975 . Regional hydrology of the Clutha River . Journal of Hydrology (NZ) , 14 : 83 – 98 .
- Norris , RJ and Craw , D . 1987 . Aspiring terrane: An oceanic assemblage from New Zealand and its implications for terrane accretion in the southwest Pacific . Geodynamics , 19 : 169 – 177 .
- Scherwath M , Stern T , Davey F , Okaya D , Holbrook WS , Davies R , Kleffmann S 2003 . Lithospheric structure across oblique continental collision in New Zealand from wide-angle P wave modeling . Journal of Geophysical Research 108 . doi: 10.1029/2002JB002286
- Stein , J , Craw , D and Pope , J . 2011 . Initial sedimentation and subsequent diagenesis in the Eastern Southland Lignite Basin, southern New Zealand . New Zealand Journal of Geology and Geophysics , 54 : 167 – 180 . doi: 10.1080/00288306.2010.515599
- Turnbull IM 2000 . Geology of the Wakatipu area . Institute of Geological & Nuclear Sciences 1:250 000 geological map 18. 1 sheet + 72p . Lower Hutt , , New Zealand , Institute of Geological & Nuclear Sciences Ltd .
- Upton P , Koons PO , Craw D , Henderson CM , Enlow R 2009 . Along-strike differences in the Southern Alps of New Zealand: Consequences of inherited variation in rheology . Tectonics 28 : TC2007 . doi: 10.1029/2008TC002353
- Van Avendock HJA , Holbrook WS , Okaya D , Austin JK , Davey F , Stern T 2004 . Continental crust under compression: A seismic refraction study of South Island Geophysical Transect I, South Island, New Zealand , Journal of Geophysical Research 109 . doi: 10.1029/2003JB002790
- Waters , JM and Wallis , GP . 2001 . Mitochondrial DNA phylogenetics of the Galaxias vulgaris complex from South Island, New Zealand: rapid radiation of a species flock . Journal of Fish Biology , 58 : 1166 – 1180 . doi: 10.1111/j.1095-8649.2001.tb00563.x
- Waters , JM , Craw , D , Youngson , JH and Wallis , GP . 2001 . Genes meet geology: fish phylogeographic pattern reflects ancient, rather than modern, drainage connections . Evolution , 55 : 1844 – 1851 .
- Waters , JM , Rowe , DL , Burridge , CP and Wallis , GP . 2010 . Gene trees versus species trees: Reassessing life-history evolution in a freshwater fish radiation . Systematic Biology , 59 : 504 – 517 . doi: 10.1093/sysbio/syq031
- Williams , GJ . 1974 . Economic geology of New Zealand . Australasian Institute of Mining and Metallurgy Monograph , 4 : 490 pp
- Youngson , JH and Craw , D . 1993 . Gold nugget growth during tectonically induced sedimentary recycling, Otago, New Zealand . Sedimentary Geology , 84 : 71 – 88 . doi: 10.1016/0037-0738(93)90046-8
- Youngson , JH and Craw , D . 1995 . Evolution of placer gold deposits during regional uplift, central Otago, New Zealand . Economic Geology , 90 : 731 – 745 . doi: 10.2113/gsecongeo.90.4.731
- Youngson , JH and Craw , D . 1996 . Recycling and chemical mobility of alluvial gold in Tertiary and Quaternary sediments, Central and East Otago, New Zealand . New Zealand Journal of Geology and Geophysics , 39 : 493 – 508 . doi: 10.1080/00288306.1996.9514728
- Youngson , JH , Craw , D , Landis , CA and Schmitt , KR . 1998 . Redefinition and interpretation of late Miocene-Pleistocene terrestrial stratigraphy, Central Otago, New Zealand . New Zealand Journal of Geology and Geophysics , 41 : 51 – 68 . doi: 10.1080/00288306.1998.9514790