Abstract
New radiocarbon ages for wood samples retrieved from the base of the Acheron rock avalanche near Porters Pass, Canterbury, show a clustering of ages between 1370 and 1101 yr BP. This is significantly dissimilar to the established radiocarbon age of 500±69 yr BP (NZ547), from weathering-rind thickness measurements and from lichen studies. This contradiction impacts on current calibrations of lichenometric and weathering-rind dating methods, which has serious implications for landslide and earthquake dates based on them. A 500–600 yr BP earthquake event along the Porters Pass–Amberley Fault Zone has been dated in an adjacent trench and is consistent with previous dates but does not correspond to the Acheron rock avalanche emplacement as previously proposed. The landslide may have been caused by either a Porters Pass Fault event (1100–800 yr BP) or by the better-constrained Round Top event (1010±50 yr BP) on the Alpine Fault.
Introduction
This study reports new radiocarbon dates for the Acheron rock avalanche in Canterbury. This was previously dated by a single radiocarbon sample, whose date (500±69 yr BP; Burrows Citation1975) was subsequently used to calibrate weathering-rind and lichenometric dating methods. The substantially older (1100–1370 yr BP) new dates have significant implications for rock avalanches and palaeoearthquakes that have previously been dated by weathering-rinds and lichenometry.
The Acheron rock avalanche is representative of those that occur within tectonically active mountain zones throughout the world (Korup et al. Citationin press). In New Zealand, rock avalanches occur in elevated granite, gneissic greywacke, Torlesse greywacke and schist bedrock and have been occurring during the development of the Southern Alps over the last 5 Ma; some recent examples are reported by McSaveney (Citation2002). Whitehouse (Citation1981, Citation1983) and Whitehouse & Griffiths (Citation1983) identified 42 greywacke-derived rock avalanches in the central Southern Alps including the Acheron deposit. Since erosional censoring can rapidly degrade deposits, the actual number that have occurred will be much greater (McSaveney pers. comm. 2003).
In New Zealand, some historic rock avalanches have been triggered by earthquakes (e.g. Falling Mountain in 1929; Davies & McSaveney Citation2002). By contrast, others had no evident trigger (Mt Cook in 1991 and Mt Fletcher I, II, McSaveney Citation2002; Mt Adams in 1999, Hancox et al. Citation2005; Young River in 2007). It is notable that none of these aseismic events were deep-seated. Deep-seated failures like that of the Acheron landslide are thought most likely to be seismically triggered (Bazgard et al. Citation2009).
The Acheron rock avalanche deposit in Canterbury (Figs ) was first identified and dated by Burrows (Citation1975). Its source (1520 m) is on the northern side of the eastern summit of Red Hill (1641 m) in the southern part of the Torlesse Range west of Lake Lyndon on SH 73. From the source the deposit extends c. 3.5 km to the southeast, descending to an elevation of c. 800 m. It forms a notable depositional feature in the upper catchment of Acheron River, a tributary of the Rakaia River (Smith et al. Citation2006). The Holocene-active Porters Pass Fault (a component of the Porters Pass–Amberley Fault Zone) is overlain by the rock avalanche deposit at the junction of two cross valleys (Figs ), and there are indications that the deposit has been affected by the fault.
This paper first outlines the local geology and geomorphology, and describes the rock avalanche and earlier investigations of its age. We then describe the acquisition and nature of the samples and their dates. This leads to a discussion of the age discrepancy found by the present study and its implications for the landslide trigger and for dating of faults and landslides in the Southern Alps.
Setting
Geology and geomorphology
The source rock of the avalanche is Mesozoic Torlesse Supergroup greywacke consisting of interbedded layers of massive greywacke sandstone and thinly bedded argillaceous mudstones and siltstones. The basement rock is ubiquitously covered by a veneer of colluvium and solifluction scree materials of varying thickness, and outcrop is confined to elevated ridgelines and steep waterways. The largest section of intact outcrop is found in the source scar () with bedding dipping steeply northwards into the centre of the present source basin. The most elevated unit is the massive sandstone (>5 m thickness) that displays close to moderate defect spacing and overlies thinly bedded argillite and sandstone sequences 200–600 mm thick. It is likely that failure occurred along the weaker argillite and sandstone bedding contacts in combination with persistent steep south-dipping and shallow north-dipping defects. The major avalanche features (source scar, remnant debris, levees, rims, depressions) are shown in and .
In the lower section of the Red Hill Stream valley, incision of Quaternary deposits by tributaries of the Acheron River during the Holocene has formed the distinctive terrace units seen in . These surfaces are thought to be derived from Holocene aggradation and Pleistocene glacio-fluvial sediments related to the early Rakaia Bayfield I–II advances > 19,000 yr BP (Soons & Burrows Citation1978). Glacial moraines and outwash surfaces are prominent in the immediate Lake Coleridge and Acheron River area, although it is unlikely that glacier ice was present within the Red Hill Stream valley at that time (Soons & Burrows Citation1978). The local stratigraphy of the Red Hill valley is summarised in .
Porters Pass–Amberley Fault Zone (PPAFZ)
The dominant structural feature of the immediate area is a dextral strike-slip fault called the Porters Pass Fault (buried by the Acheron rock avalanche as seen in Figs ). This fault is a prominent segment of the Porters Pass–Amberley Fault Zone (or PPAFZ, after Cowan et al. Citation1996) and is the only known Holocene-active fault crossing the Red Hill Stream valley. The PPAFZ is a hybrid zone of strike-slip and reverse-thrust faulting extending over c. 100 km from the Rakaia River immediately southwest of Lake Coleridge to the north of Christchurch as shown in (Cowan et al. Citation1996; Howard et al. Citation2005). The zone is described as a juvenile southwards-migrating extension of the Marlborough Fault Zone, a fault system transferring strain from the convergent margin of the colliding Pacific–Australian plates in the east to the Alpine Fault in the west (Cowan Citation1992; Cowan et al. Citation1996; Pettinga et al. Citation2001).
Figure 1 Map of the central Southern Alps showing the location of the Acheron rock avalanche deposit.
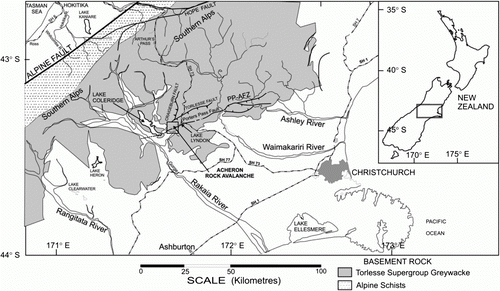
Studies of the PPAFZ by Cowan (Citation1992) and Cowan et al. (Citation1996) used palaeo-landslides for additional information to identify earthquake events and magnitudes along the fault zone. Applying ages from ten dated large landslides, Cowan et al. (Citation1996) were able to identify a cluster of six landslides including the Acheron deposit (based on the NZ547 age) around 700–500 yr BP spread along the length of the PPAFZ (see for weathering-rind ages of three of the six landslides). This concentration of landslide ages located in close proximity to the PPAFZ implied that one or more rupture events occurred during 700–500 yr BP, triggering slope instability along the fault zone. Cowan et al. (Citation1996) expressed some uncertainty with this conclusion due to the landslide history including geomorphic modification and unknown trigger mechanisms; any coseismic interpretation is tentative and dependent on the frequency of seismic events found in the geological record (Cowan et al. Citation1996).
Howard et al. (Citation2005) showed the Porters Pass Fault to be Holocene-active using trench excavations along the Porters Pass Fault (which crosses the Acheron rock avalanche deposit) at sites more than 5 km from the rock avalanche deposit, and measured displaced geomorphic markers along the discontinuous trace. These investigations revealed six earthquakes with an average total horizontal displacement of 33 m or 5–7 m/event and a total vertical displacement of 1.7 m. The ages for the rupture events suggest an average earthquake return interval of c. 1500 years with magnitudes within the range M W 7.1–7.7 depending on rupture lengths. Howard et al. (Citation2005) found evidence for a rupture c. 600–500 yr BP and for another at 1100–800 yr BP.
Looking beyond the PPAFZ, Cowan (Citation1992) recalibrated the weathering-rind ages of rock avalanches in the central Southern Alps to the calibration curve of McSaveney (Citation1992) using Whitehouse and Griffiths’ (Citation1983) rock avalanche age data. This identified regional patterns of large-scale instability in the 700–500 yr BP period. Two earlier age modes of instability were identified: c. 1050–1400 yr BP (340 yr range) and c. 1700–2100 yr BP (400 yr range; Cowan et al. Citation1996). The lack of regional overlap with landslides identified along the PPAFZ during 700–500 yr BP was interpreted to indicate that the latter reflected a localised earthquake event, most probably along the PPAFZ (Cowan et al. Citation1996).
Acheron rock avalanche deposit
Morphology
In interpreting and co-relating dates derived from different parts of the avalanche deposit, an overall picture of its emplacement process and morphology is helpful. The rock avalanche (Smith et al. Citation2006) resulted from a deep-seated failure of the source area: about 107 m3 of greywacke rock moved first eastwards, then turned 80° to the south-southeast to follow the straight valley of Red Hill Stream, which is deeply terraced in its lower part (see ). The intensely fragmented deposit forms distinct morphology including levees, lateral rims, hummocky terrain and a central depression. It lies directly across an adjoining cross-valley (collinear with the Porters Pass Fault) without spreading into it. The deposit is thin (<10 m) in places in its distal part, where the base is visible in the sides of incised stream sections.
The source area is an arcuate basin mantled with debris from the event. Subsequent erosion has formed extensive scree deposits within the basin. Immediately below the basin is a smooth undulating surface descending at right angles to the main valley axis. The debris spreads at the junction with the main valley axis, resulting in a partial deflective rise and runup of debris on the true left valley side (referred to here as runup one) and a (double) trim line on the true right side (). Down-valley of runup one, the upper runout section is partly buried by an extensive talus fan from a wedge failure on the true left of the valley. Opposite this fan, three mounds of debris are located against a hill slope. They indicate where debris impacted (referred to here as runup two), causing some material to stop while the remainder was deflected down-valley (Figs ).
Only sporadic exposures of debris were observed above the runup two zone (), much of the deposit being overlain by more recent alluvium with no exposure of the base of the deposit. Consequently, it is difficult to infer a thickness in this area. Below runup two a straight trim line or levee on the true left extends 900 m from the talus fan down-valley reaching a height of 35 m above the deposit. This is likely to have resulted from topographic interference associated with runup two forcing the debris to become super-elevated on the true left side in a glancing motion down the valley wall to the junction with the adjoining valleys (). This suggests that the debris mass was moving with significant velocity in multiple pulses.
Figure 3 Geomorphic map of the Acheron rock avalanche deposit surface showing the lobate surface morphology and the site of the lichen and weathering-rind (W.R) measurements relative to the radiocarbon sample locations (this study). The location of the trench investigation is identified by the blue rectangle on the east side of the rock avalanche. The Porters Pass Fault trace is shown in red.
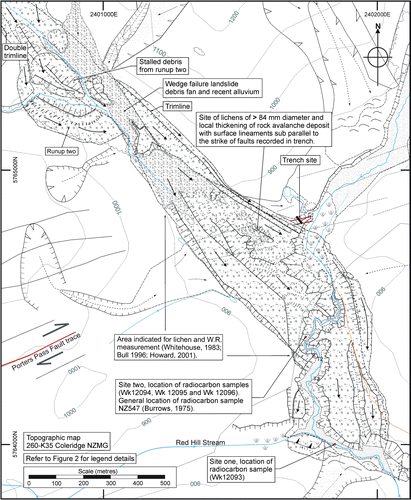
The lower half of the deposit displays steep lateral edges or ridges defining the debris margins and partially overlies a set of terraces providing good control on deposit thickness and width. It also shows an unusual and distinct central depression, almost certainly related to underlying terrace topography. The most distal debris consists of a thin layer of material plastered against a terrace surface ( and ).
Dimensions
The rock avalanche travelled almost 3.5 km down 700 m (vertical) at velocities of 140–160 km hr−1. The Farböschung (the ratio of total vertical fall height to total run-out length) was 0.19, indicating high mobility. The deposit area was estimated using a GPS outline of the deposit (horizontal accuracy of ±5 m or better), which gave a reliable planimetric area of 720,000 m2 (Smith et al. Citation2006). From this, a minimum deposit volume of 8.9×106 m3 was calculated using an estimated mean depth derived from observed and estimated thicknesses for different morphological zones within the deposit. By assuming a rock mass dilation of 20% (McSaveney Citation1978; Evans et al. Citation2001) the source rock mass volume was estimated to be 7.4×106 m3.
Internal composition
The rock avalanche deposit structure displays three distinct lithological divisions (). The upper section is defined by a surface veneer of angular blocky rock fragments typically 400 mm in length, with accumulations of larger blocks exceeding 3 m in longitudinal dimension. Below the blocky layer, a highly pulverised bluish-grey unit of angular rock fragments <200 mm in diameter occurs in a coarse sand matrix visible both in the incised channel and the deposit distal section. Elongated fragments of highly shattered argillite and sandstone boulders, which have the texture of fault gouge when wet, can be discerned. The lower basal layer comprises pale-grey silty sand material containing subrounded clasts (probably entrained from the pre-existing surface) and some wood fragments and buried trees with random orientations. The contact between the basal layer and the fragmented material appears irregular and stepped.
Figure 5 The profile of the Acheron rock avalanche deposit and Late Quaternary/Late Triassic stratigraphy of the Red Hill valley.
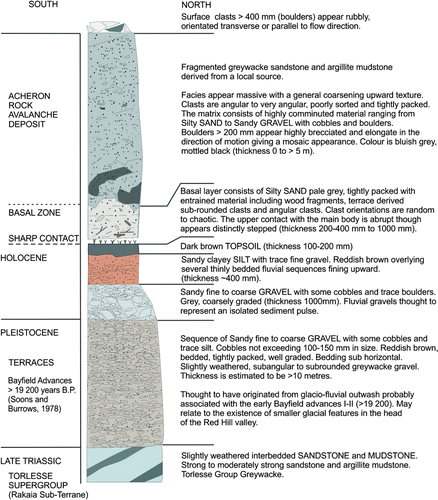
Figure 6 Timing of earthquakes on the Alpine Fault and Porters Pass Fault relative to geomorphic dating evidence of the Acheron rock avalanche and regional instability. The new radiocarbon ages for Acheron rock avalanche and the Porters Pass Fault trench investigation (this study) are presented as two-sigma calibrated calendar ages.
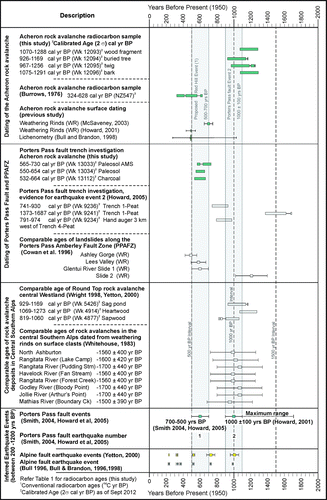
Previous dates
The first date for the Acheron rock avalanche deposit was derived from a river-cut exposure in a tributary of the Red Hill Stream (see ; in Burrows Citation1975). Burrows (Citation1975) described a buried forest horizon overlying yellow-brown soils located on reddish horizontally bedded gravels. Above this layer, Burrows (Citation1975) noted the unweathered poorly sorted angular pebble and cobble gravels which were interpreted to represent the rock avalanche deposit (). Burrows (Citation1975) extracted wood samples from along the exposure which were radiocarbon dated at 500±69 yr BP (324–628 cal yr BP; NZ547) and concluded that this date represented the emplacement of the Acheron rock avalanche triggered by an earthquake on the Porters Pass Fault.
Figure 8 View of the exposed contact between the Acheron rock avalanche deposit and the underlying terrace surface (person arrowed for scale), described in Burrows (Citation1975). The location of the buried tree radiocarbon sample (Wk 12094; also referred to as site two), is along the continuation of the contact (see dashed line) below the bottom right of the photograph (second arrow indicates location).
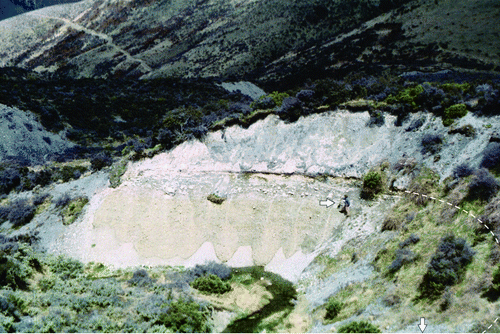
Later age studies of the Acheron rock avalanche deposit were aimed at assessing the potential of the deposit as a palaeoseismic indicator for Holocene rupture along either the Alpine Fault or the PPAFZ. These included a lichenometry analysis (Bull & Brandon Citation1998) and three separate rock weathering-rind thickness studies (Whitehouse & Griffith Citation1983; Howard Citation2001; McSaveney pers. comm. 2003). The source of the rock weathering-rind and lichen sample populations is shown in , generally confined to the mid-section of the deposit below runup two and up-valley of the NZ547 sample site.
Bull (Citation1996) and Bull & Brandon (Citation1998) examined regional rockfall events associated with ruptures on the Alpine Fault, and used a polymodal distribution of the measured maximum diameters of lichens to determine emplacement ages and thus episodic rupture events. Plentiful lichen diameters of 84 mm or less were measured at the Acheron deposit, representing an age of 460±10 yr BP (using the normal Gaussian distribution) supporting the NZ547 age of 500±69 yr BP (Bull pers. comm. 2003). A second much smaller lichen population with diameters up to 172 mm was measured on the forested true left side of the deposit indicated in . These lichen diameters represented an approximate age of 1000 yr BP. Bull (pers. comm. 2003) considered this to be the result of the occurrence of an older rock avalanche and suggested that this reflects ongoing instability in the source scar, considering regional instability around 1000 yr BP and the broad appearance of separate lobe morphology between the two lichen population sites (see ). Interestingly, the site Bull described for the larger lichen is covered with beech trees and large greywacke boulders; by contrast, the maximum lichen diameters of 84 mm or less dominated on a smoother surface with few large boulders and no beech trees.
Two weathering-rind studies (thought to have been undertaken in the same debris zone as the lichen study) identified slightly differing modal peak thicknesses. Whitehouse & Griffiths (Citation1983) calculated 0.66 mm while Howard (Citation2001) measured a modal peak of 0.83 mm. The 0.83 mm thickness from Howard's study represents an age of 490±55 yr BP supporting the NZ547 age (Howard Citation2001). A third (unpublished) weathering-rind study was conducted on rock avalanche debris just up-valley of the runup two and tributary on the true right side of the run-out path (see and ). This returned a rind thickness of 1.07 mm, equivalent to an age of 700±140 yr (McSaveney pers. comm. 2003). McSaveney (pers. comm. 2003) commented that it seems unlikely that a younger rock avalanche could have bypassed this sampling site.
We note here that lichenometry is subject to a number of uncertainties (e.g. Orwin et al. Citation2008); some of these uncertainties are related to post-emplacement disturbance that can also reset weathering rinds. We outline some of these later (see Discussion, p. 13).
Field data
Trench evidence in Red Hill valley
In order to obtain more information about the history of the Porters Pass Fault in the immediate vicinity of the Acheron rock avalanche, we excavated a trench across the fault within the Red Hill valley where it forms a northwest-facing scarp in the Bluff river valley (immediately adjacent to the eastern side of the rock avalanche deposit; and ). The trench yielded evidence that this segment of the fault ruptured after 700 yr BP (Smith Citation2004). Two soil horizons were identified in the north end of the trench within a total of 1.1 m of clayey silt overlying coarse material (), representing three episodes of sedimentation. The clayey silt layer contained very little to no sand and fluvial structure, and therefore most likely represents overbank deposits resulting from flood events or blockage of the adjacent Bluff River. This is supported by the lack of evidence for similar clayey silt stratigraphy below Unit 2 within the trench (). The probable cause of the initial inundation is emplacement of the Acheron rock avalanche deposit which filled the Red Hill valley, completely blocking the river several hundred metres south of the trench site. Radiocarbon ages for the lower buried soil horizon in this trench − 661±34 yr BP (550–654 cal yr BP; Wk 13034 palaeosol) and 653±54 yr BP (532–664 cal yr BP; Wk 13112 charcoal) – support Cowan et al.'s (1996) 700–500 BP event. See for conventional radiocarbon dates and two-sigma calibrated ages.
Figure 9 Section of trench through Porters Pass Fault immediately southeast of the rock avalanche deposit.
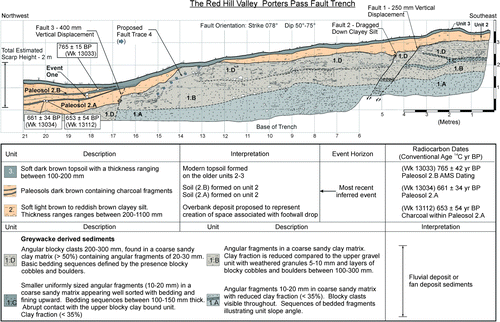
Table 1 Radiocarbon age results from the Acheron rock avalanche deposit and the Porters Pass Fault; includes radiocarbon results from this study and selected radiocarbon results from previous studies. Calibrated ages are reported to two-sigma.
An assessment of aerial photographs of the deposit where it overlies the approximate position of the Porters Pass Fault show subtle surface expressions or minor lineaments trending obliquely across the rock avalanche deposit surface and terminating against the eastern limb of Hill 1249 (). This is highlighted by a thickening and localised increase in elevation of the deposit bounded on either side by two post-deposition stands of beech trees extending westwards away from the main tree stands (which trend south in the direction of rock avalanche deposition; ). These lineaments suggest that the rock avalanche pre-dates the 600–500 yr BP rupture event (Smith Citation2004). It should be noted that while there are morphological features present, there is no clear fault evidence across the rock avalanche; however, Howard (Citation2001) suggests this could indicate the presence of steps or a bend in the fault trace as it crosses the Red Hill valley.
Figure 10 Aerial photograph showing the mid zone of the Acheron rock avalanche crossed by the Porters Pass Fault. A zone of subdued surface expressions obliquely crossing the rock avalanche deposit is marked by the black arrows. Ridge rents and other fault-related lineaments are shown by red arrows. The white arrows show the trend of geomorphic features such as redundant water channels that correspond to the black arrows. The triangles indicate areas of coarse boulders, debris mass thickening and high elevation on the deposit that may reflect the presence of a buried fault scarp.
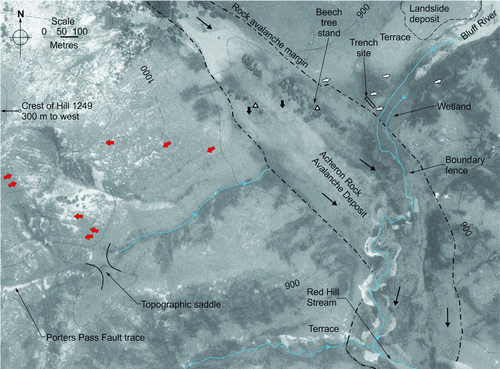
Dating the rock avalanche
To establish the age of the rock avalanche and find evidence of a relationship to Holocene palaeoseismic activity along the Porters Pass Fault, the northeast margin of the site identified in Burrows (Citation1975) was resampled and a new site several hundred metres downstream was sampled for material suitable for radiocarbon dating (). All grid references for sample locations are Sheet K35 Ed 1. 1989 Ltd Rev 199, presented as northing and easting NZMG. Samples collected for radiocarbon dating are presented in .
Site one: 2401840 E, 5764010 N NZMG
In the most distal section of the rock avalanche (Grid ref. K35/018 640), the river has eroded and removed most of the rock avalanche deposit. However, remnants of the basal zone consisting of pale grey silty sand with angular to subrounded clasts in random orientations and wood fragments are exposed in a small riverbank as shown in . This site is downstream of the confluence of tributaries of Red Hill Stream, away from that identified by Burrows (Citation1975), and is within a basal mixing zone interpreted to be immediately above the contact with the pre-rock-avalanche surface. A wood sample identified as Mountain Beech (Nothofagus solandri var. cliffortioides) was retrieved from this site (A). The extremities of the wood were rounded, suggesting entry into the fluvial system or damage during burial by the Acheron rock avalanche deposit.
Figure 11 Photographs of the four samples sent to Waikato University Radiocarbon Laboratory for dating (scale bar is 3 cm). A, Wk12093 wood fragment from site one, 1319±51 yr BP. B, Wk12094 cut cross-section from buried tree at site two, 1152±51 yr BP. C, Wk12095 buried twig from site two 1232±52 yr BP. D, Wk12096 buried bark fragments retrieved from site two, 1327±52 yr BP.
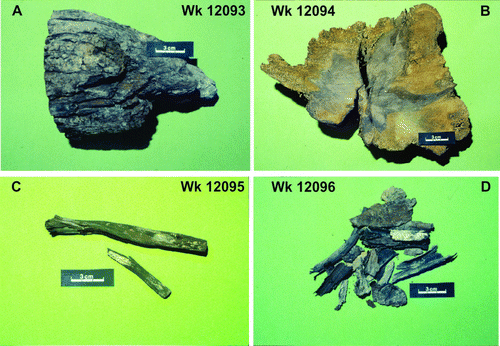
Site two: 2401730 E, 5764420 N NZMG
At the southeast edge of the river cut exposure shown in (Grid ref K35/017644), a buried tree, significant amounts of leaf litter, small twigs and bark fragments were all exposed within the contact between the rock avalanche and the terrace (). This site faces southeast (137°) away from the sun, reducing diurnal wetting and drying of the samples and offering a good opportunity for dating the contact. Certainty can be given to the burial of the samples by the Acheron rock avalanche, particularly by their orientation which appears flattened in a south-easterly (down-valley) direction. The stratigraphy displays reddish-brown sandy gravels overlain by yellowish-brown silty clay interpreted to represent the terrace surface. Above this extends 3–4 m of bluish-grey angular coarse rock avalanche debris.
Figure 12 Photograph of sample site two containing the buried tree (Wk 12094), twigs (Wk 12095) and bark fragments (Wk 12096) within the contact between the rock avalanche and the terrace material (Grid ref K35/017 644). The buried tree is visible between the white arrow and 15 cm ruler.
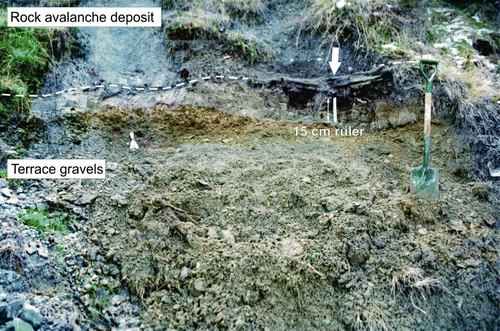
The wood material was found directly in the contact and the top 50 mm of the terrace silty clay layer. The material within the silty clay layer is thought to be the result of the tree sample being pushed into the top of the terrace by the weight of the overlying rock avalanche debris. Three wood and bark samples were retrieved from this site for radiocarbon dating.
Brief sample description
Sample Wk 12094 (B) consisted of a cross-section cut from the trunk of a buried tree identified as Nothofagus solandri var. cliffortioides excavated from the top of the terrace surface (see ). A growth age of 70–80 years was estimated based on the 76±5 counted growth rings. The tree was lying in an easterly or down-valley direction; this is strong evidence for its being flattened during the rock avalanche event. Around the buried tree in the upper silty clay layer of the terrace were abundant stick fragments with bark and bark fragments. Twig sample (Wk 12095) was collected displaying growth rings of 10±5 years as seen in C. Bark fragments (Wk 12096) interpreted to be from the buried tree and twigs located around the buried tree (Wk 12094) within the silty clay of the upper terrace layer were also collected (D).
Interpretation of results
Radiocarbon ages () are presented as conventional ages (as given in the age reports by Waikato University Radiocarbon Dating Laboratory). Conventional ages can be calibrated against the southern hemisphere calibration curve SHCal04 (McCormac et al. Citation2004) for terrestrial samples using the OxCal 4.1 program (Bronk Ramsey Citation2001). Dates presented as calibrated include the prefix cal and are reported as two-sigma ages (see ).
The location of wood fragment (Wk12093) within the pale-grey silty sand basal material implies that it was incorporated into the rock avalanche base from an unknown position further up-valley. The age of 1319±51 yr BP (1070–1288 cal yr BP) is the second oldest date, suggesting an unknown inbuilt age. Sample Wk 12094 (the buried tree) was cut from the base of the trunk of the buried tree. The outer ten rings were dated to best characterise the time when the tree was killed. This sample is therefore the most contemporaneous with the event of all the samples dated suggesting that the inbuilt age was solely the growth age. The resulting age 1152±51 yr BP (926–1169 cal yr BP) would therefore reflect an age contemporaneous with the landslide event. This is the preferred representative age of the Acheron rock avalanche. The twig sample (Wk 12095) has the second youngest date 1232±52 yr BP (967–1256 cal yr BP), which reflects the average age of the entire specimen. The inbuilt age is expected to be negligible and was retrieved contemporaneously, giving a close age for the emplacement. The conventional radiocarbon age of the twig is however older than the buried tree sample (Wk 12094), which implies an inbuilt age. This may represent storage of the specimen on the forest floor before the actual event, resulting in an age older than the rock avalanche. The age of 1327±52 yr BP (1075–1291 cal yr BP) for the bark fragments (Wk 12096) is the oldest of the four samples and is considered to reflect the growth age of a tree. Because bark accumulates over the life of the tree, dated bark material may represent an age older than that of the younger wood immediately beneath, which may be why it has the oldest date. Equally, it may be derived from another buried tree present within the exposure. The combined four dates show a consistent overlapping range of ages as seen in and presented in . This demonstrates a probable timing for the emplacement of the Acheron rock avalanche as post-dating the age range.
To test if all four sample ages are consistent or if the age ‘determinations are estimating the same date’ (Ward and Wilson Citation1978, p. 21) a simple statistical analysis based on the chi-square distribution was carried out. Calibrated ages for the four samples were combined using the OxCal freeware program which applies the standard method described in Ward and Wilson (Citation1978). The results (, Run no. 1) show X2-Test: d.f.=3; T = 7.7 (5% 7.8) where the T value is less than 7.8; the ages are therefore shown to ‘not be significantly different’ (Ward and Wilson Citation1978, p. 21) and could be combined forming a ‘pooled age’ as discussed in Ward and Wilson (Citation1978). A number of the chi-square tests were run with different sample combinations; results are listed in . Two test failures occurred () when the buried tree sample (Wk 12094) was combined with only one of either the distal wood fragment (Wk 12093) or the bark sample (Wk 12096), both of which had the oldest age determinations and have the potential for unknown inbuilt ages. Regardless of the statistical similarities of the combined four ages or the differences of the two oldest ages, the close contemporaneous position of the buried tree (Wk 12094) with the youngest age of 1152±51 yr BP, flattened in a down-valley direction, is considered to best represent the approximate age at which the rock avalanche occurred.
Table 2 Chi-square test results from combinations of radiocarbon age determinations for the Acheron rock avalanche deposit.
Discussion: Implications of the new ages for the Acheron rock avalanche deposit
Our radiocarbon ages differ substantially from that of the radiocarbon sample NZ547 (500±69 yr BP). Burrows (Citation1975) states that the (NZ547) wood sample was derived from the 40-m-long river cut exposure ( in Burrows Citation1975) referred to as site two in this study (). This is the same site sampled for Wk 12094, Wk 12095 and Wk 12096 which produced ages of more than 1000 yr BP. The sampling conducted at two sites during this study and the overlap of ages between the wood samples strongly suggest that the NZ547 sample dated something other than the rock avalanche emplacement. A part of the NZ547 sample still exists and contains visible charcoal. Basal charcoal has not been detected subsequently at this sample site, and it seems possible that an error may have been made in attributing NZ547 to the stratigraphic position at the contact of the Acheron rock avalanche and the underlying terrace surface.
The new radiocarbon ages also differ from the favoured lichenometry and rock weathering-rind thickness ages for the rock avalanche surface of c. 450 yr BP (Whitehouse Citation1983; Bull & Brandon Citation1998; Howard Citation2001). The lichen modal peak of 84 mm (460±10 yr BP) matched the NZ547 age and has been reported as representing the emplacement of the rock avalanche at c. 500 yr BP (Bull & Brandon Citation1998; Orwin Citation1998; Howard Citation2001). However, the lichenometry also identified a smaller set of lichen populations exceeding 84 mm up to 172 mm (approximate maximum age of 1000 yr BP), which appear to correspond with the new radiocarbon ages.
Because the statistical lichen analysis applies a Gaussian distribution to identify dominant populations within a sample group, it could be possible that the oldest lichen actually represents the remnant evidence of the actual rock avalanche deposition. The 84 mm age (460±10 yr BP) could relate to subsequent changes to the habitat conditions required for lichen longevity by occurrences of surface disturbances rather than a second rock avalanche event. In contrast, WB Bull (pers. comm. 2003) suggests the lichen distribution is representative of several episodes of rock avalanching 500 years apart.
Unlike lichenometry, the weathering-rind studies did not identify the oldest age (c. 1030 yr BP); however, the boulders under tree cover where the oldest lichens were located would not have been sampled as the rocks are shielded from the atmosphere. Interestingly, MJ McSaveney (pers. comm. 2003) found that weathering-rind ages from debris up-valley of a tributary (and runup two) on the true right side of the deposit produced a modal thickness of 1.07 equating to an age of 700 yr±140 yr. This questions further the reliability of the previous weathering-rind dates and adds to the uncertainty of the surface-derived ages.
The variations of dates could indicate problems in the collection and/or measurement process of either dating technique or could be the result of incorrect interpretations of geomorphic surfaces. They raise the possibility of a post-deposition earthquake event or other surface disturbance that did not affect the base of the deposit. If the weathering-rind and lichen dating techniques were shown to be erroneous in their application, this could have implications for previously dated surfaces such as the 29 weathering-rind thickness ages from Whitehouse & Griffiths’ (Citation1983) rock avalanche compilation. However, the consistency of age produced by the different dating techniques suggests that an event such as fire could have resulted in an almost total reset of the lichens and/or that seismic shaking resulted in an almost total reset of the rinds. The latter would have to cause movement or partial disturbance of surface clasts and boulders, causing a fresh rock surface to be exposed to the surface; this would require strong ground motion but, in such close proximity to a known active fault, is certainly possible. Interestingly, the large clasts present on the rock avalanche surface (ranging in length from 0.4 m up to 5 m) occur in a very limited area rather than being distributed over the whole surface as is more normal. This might however be due to the well-known lack of mixing in rock avalanches in combination with the very limited area of massive rock exposed in the headscarp. The larger boulders display a general (along-flow) long-axis orientation which may not be compatible with a ubiquitous and severe seismic disturbance of the surface boulders.
Trenching during this study produced evidence for an earthquake within the Red Hill valley adjacent to the rock avalanche deposit around 700–500 yr BP. This is supported by the observation of similarly oriented lineaments crossing the rock avalanche in the area where both lichen and weathering-rind samples were obtained, suggesting the weathering-rind and lichen ages could record a seismic resetting episode (Smith Citation2004).
Fires appear to have occurred in the Cass–Porters Pass area around 750–400 yr BP (Molloy et al. Citation1963) and could have destroyed pre-500 yr BP lichen evidence on the Acheron deposit. McSaveney (Citation1992) noted charcoal (NZ5407C 660±65) immediately below the base of the Craigieburn rock avalanche several kilometres north of the Red Hill valley, which he interpreted to represent destruction of a beech forest by fire around this time. This corresponds to charcoal (Wk 13112 653±54 yr BP) located in buried soil during our trenching of the fault line in the Red Hill valley. Bull & Brandon (Citation1998) acknowledge the effect of fire on the populations of lichen (referred to as fire kill). We conclude that the occurrence of fire in the Red Hill area may have had an effect on present-day lichen diameters.
We have considered the possibility of more than one deposition event associated with the Acheron deposit as proposed by Bull. That could reasonably explain the differences in age between the base of the deposit, the surface ages and the two sets of lichens populations. We feel that while the presence of multiple events cannot be dismissed, it seems unlikely based on the morphological evidence. The second event would have required a volume enabling it to exceed the run-out of normal rock fall and travel more than 1.8 km along a path underlain by the debris of the first rock avalanche, before appearing to terminate metres from the radiocarbon site two river cut ( and ).
Our view is supported by evidence from the site two exposures ( and ) which show a homogeneous mass with no lithological or structural differences to suggest a later secondary deposition. The exposure is located c. 10 m south of the possible termination of the smooth surfaces lobe indicated by Bull as the principal source of the 84 mm lichens. The distinct depression broadly separating the two age groups is most likely caused by the underlying terrace morphology rather than by two distinct rock avalanche episodes. Further, the double trim line between runup one and two is evidence for pulses of activity and lobate behaviour within a single rock avalanche rather than separate events ().
Figure 13 Double trim line between runups one and two. The distance between the single middle arrow and the stand of beech trees in the lower left of the photo is c. 100 m.
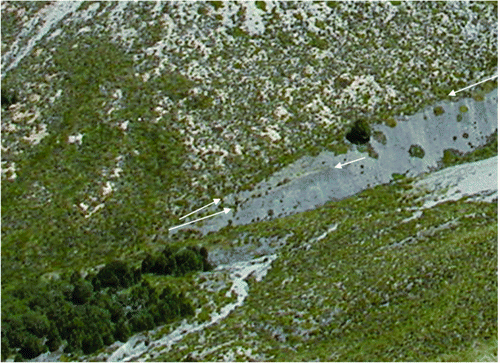
A further possibility is that of a debris flow over the lower true right area of the deposit, where large surface rocks are sparse and the smaller lichens were found. This could have been caused by an event either from the wedge failure gully on the true left of the main valley or from the true right tributary opposite it at any time after the main landslide. A debris flow from either source could have reset the true right part of the deposit. Both these possibilities, however, are almost precluded by their apparent failure to significantly infill the low area bordering the true right edge of the deposit where it crosses the side valley; this appears not to have been infilled at all since the main event, which argues that no significant sediment-carrying events have occurred since then.
While the rock avalanche surface could have been smoothed by a water flow carrying little sediment, the volume of water needed seems unlikely to occur without large volumes of sediment in the highly erodible materials of the Red Hill valley.
Based on these observations it is unlikely that two distinct landslides, or a major event following the first landslide, occurred within the valley; unexplained differences do exist between the inferred ages at the buried contact and the rock avalanche surface, however. This emphasises the need to recheck age-dating evidence in geological studies, especially considering that Bull & Brandon (Citation1998) and McSaveney (Citation1992) referred to the NZ547 date as part of their extensive lichen and weathering-rind calibration data and this date is often referenced in literature relating to weathering-rind dates and rock avalanches in the Southern Alps (Whitehouse Citation1983; Whitehouse & Griffiths Citation1983; McSaveney Citation1992; Cowan et al. Citation1996; Bull & Brandon Citation1998; Orwin Citation1998; Howard Citation2001).
The new radiocarbon ages provide no evidence to support a 600–500 yr BP coseismic relationship between the rock avalanche and the PPAFZ. Although there is no direct evidence, a relationship may however exist with either the 1100–800 yr BP event identified by Howard et al. (Citation2005) in a trench at Porters Pass, or with stratigraphic evidence from the Round Top event (1010±50 yr BP) on the Alpine Fault (Wright Citation1998; Yetton Citation2000).
The timing of earthquake events on the Porters Pass Fault relative to the geomorphic age dating of the Acheron rock avalanche for the last 2000 yr BP is summarised and presented in . In addition, these ages are compared to the regional-instability-specific occurrences of rock avalanches in the central Southern Alps and Alpine Fault earthquake events for the same 2000 year period.
Conclusions
New radiocarbon ages relating to the deposit of the Acheron rock avalanche have been presented. These are interpreted to pre-date or co-date the emplacement of the rock avalanche; the probability of the event killing the tree from which sample Wk 12094 (1152±51 yr BP) was taken however suggests that they closely represent the deposit age. This differs considerably from the (500±69 yr BP NZ547) age previously interpreted to date the Acheron deposit; consequently, we suggest that it may date something other than the rock avalanche emplacement.
Potential discrepancies appear to exist between the geomorphic application of surface dating techniques of lichenometry and weathering-rind thickness, and the NZ547 radiocarbon age. The new radiocarbon age evidence emphasises the intrinsic unreliability of single ages of any sort and the vulnerability of surface-dating techniques to post-emplacement disturbances. While it is possible that two rock avalanches occurred, or that a debris flow occurred long after the rock avalanche, this seems unlikely. The age differences can be explained more realistically by the intricate rock avalanche morphology, complexities affecting the longevity of lichens and disturbances of the surface of the deposit by fire and/or by local seismicity.
Our trench radiocarbon dates suggests that an earthquake did occur around 700–500 yr BP within the Red Hill valley segment of the Porters Pass Fault, offering a source of surface disturbance. This could directly explain the recent weathering-rind ages and the dominant modal peak of the lichens, which are also consistent with the new rock avalanche radiocarbon ages.
The proposed coseismic relationship between the Acheron rock avalanche and the Porters Pass Fault between 600–500 yr BP is disproved by the new age data. Regional instabilities from a rupture on the Alpine Fault (Round Top event) or a Porters Pass event at around 1100–800 yr BP are preferable triggers for the avalanche.
Acknowledgements
We acknowledge the assistance of the Mason Trust, University of Canterbury, in funding this project. We are grateful to the Department of Conservation and the Pastoral Lease holder, Brooksdale Station Ltd, for allowing access and excavation of the trench. Thanks to field assistants Matt Smith and Eric Bird during the trench excavation and logging. Thank you to Mauri McSaveney for contributions of unpublished information about the Acheron rock avalanche and to David Nobes, Jocelyn Campbell and Jarg Pettinga for input and advice. Thanks to Bill Bull for providing his field notes on lichen measurements and Fiona Petchley from the Waikato Radiocarbon Laboratory for input and advice on radiocarbon testing. We thank journal referees John Orwin, Andy Nicol and an anonymous reviewer for their suggestions and comments which have significantly improved this manuscript.
References
- Bazgard A , Buech F , Davies TRH 2009 . Edifice resonance and coseismic landslide volume . Abstract NH41C 1256 , AGU Fall Meeting , San Francisco .
- Bronk Ramsey C 2001 . Development of the radiocarbon calibration program . Radiocarbon 43 : 355 – 363 . http://c14.arch.ox.ac.uk/oxcal.html (accessed 1 September 2012) .
- Bull , WB . 1996 . Prehistoric earthquakes on the Alpine Fault, New Zealand . Journal of Geophysical Research B3 , 101 : 6037 – 6050 . doi: 10.1029/95JB03062
- Bull , WB and Brandon , MT . 1998 . Lichen dating of earthquake generated regional rock-fall events, Southern Alps, New Zealand . GSA Bulletin , 110 ( 1 ) : 60 – 84 . doi: 10.1130/0016-7606(1998)110%3C0060:LDOEGR%3E2.3.CO;2
- Burrows , CJ . 1975 . A 500-year-old landslide in the Acheron River Valley, Canterbury . New Zealand Journal of Geology and Geophysics , 18 ( 2 ) : 357 – 360 . doi: 10.1080/00288306.1975.10418206
- Cowan HA 1992 . Structure, seismicity and tectonics of the Porters Pass–Amberley Fault Zone, North Canterbury . Unpublished PhD thesis. Christchurch, University of Canterbury.
- Cowan , H , Nicol , A and Tonkin , P . 1996 . A comparison of historical and paleoseismicity in a newly formed fault zone and a mature fault zone, North Canterbury, New Zealand . Journal of Geophysical Research , 101 ( B3 ) : 6021 – 6036 . doi: 10.1029/95JB01588
- Davies , TR and McSaveney , MJ . 2002 . Dynamic simulation of the motion of fragmenting rock avalanches . Canadian Geotechnical Journal , 39 : 789 – 798 . doi: 10.1139/t02-035
- Evans , S , Hungr , O and Clague , JJ . 2001 . Dynamics of the 1984 rock avalanche and associated distal debris flow on Mount Cayley, British Columbia, Canada; implications for landslide hazard assessment on dissected volcanoes . Engineering Geology , 61 : 29 – 51 . doi: 10.1016/S0013-7952(00)00118-6
- Hancox , GT , McSaveney , MJ , Manville , VR and Davies , TR . 2005 . The October 1999 Mt Adams rock avalanche and subsequent landslide dam-break flood and effects in Poerua River, Westland, New Zealand . New Zealand Journal of Geology and Geophysics , 48 : 683 – 705 . doi: 10.1080/00288306.2005.9515141
- Howard ME 2001 . Holocene surface-rupturing earthquakes on the Porters Pass Fault . Unpublished MSc thesis , Christchurch , University of Canterbury .
- Howard , ME , Nicol , A , Campbell , J and Pettinga , JR . 2005 . Holocene paleoearthquakes on the strike–slip Porters Pass Fault, Canterbury, New Zealand . New Zealand Journal of Geology and Geophysics , 48 : 59 – 74 . doi: 10.1080/00288306.2005.9515098
- Korup O , Schneider D , Huggel C , Dufresne A in press . Longrunout landslides. In: Marston R, Stoffel M, Shroder J eds . Treatise on geomorphology. Hazards, applied, anthropogenic and cultural geomorphology: mountain and hillslope geomorphology . San Diego , Academic Press (Elsevier Science) .
- McCormac , FG , Hogg , AG , Blackwell , PG , Buck , CE , Higham , TFG and Reimer , PJ . 2004 . SHCa104 southern hemisphere calibration 0–11.0 cal kyr BP . Radiocarbon , 46 : 1087 – 1092 .
- McSaveney MJ 1978 . Sherman Glacier rock avalanche, Alaska, U.S.A. In: B. Voight ed. Rockslides and Avalanches 1, Natural phenomena. Developments in Geotechnical Engineering 14A(6). Amsterdam, Elsevier . Pp. 197 – 258 .
- McSaveney MJ 1992 . A manual for weathering-rind dating of grey sandstones of the Torlesse Supergroup, New Zealand . Institute of Geological and Nuclear Sciences science report 92/04. Lower Hutt, Institute of Geological and Nuclear Sciences .
- McSaveney MJ 2002 . Recent rockfalls and rock avalanches in Mount Cook National Park, New Zealand . In : Evans SG , De Graff JV Catastrophic landslides: effects, occurrence, and mechanisms. Geological Society of America Reviews in Engineering Geology 15 : 35 – 70 .
- Molloy , BPJ , Burrows , CJ , Cox , JE , Johnston , JA and Wardle , P . 1963 . Distribution of subfossil forest remains, eastern South Island, New Zealand . New Zealand Journal of Botany , 1 : 68 – 77 . doi: 10.1080/0028825X.1963.10429322
- Orwin , JF . 1998 . The application and implications of rock weathering-rind dating to a large rock avalanche, Craigeburn Range, Canterbury, New Zealand . New Zealand Journal of Geology and Geophysics , 41 : 219 – 223 . doi: 10.1080/00288306.1998.9514806
- Orwin , JF , McKinzey , KM , Stephens , MA and Dugmore , AJ . 2008 . Identifying moraine surfaces with similar histories using lichen size distributions and the U2 statistic, southeast Iceland . Geografiska Annaler , 90A ( 2 ) : 151 – 164 . doi: 10.1111/j.1468-0459.2008.00168.x
- Pettinga , JR , Yetton , MD , Van Dissen , RJ and Downes , G . 2001 . Earthquake source identification and characterisation for the Canterbury region, South Island, New Zealand . Bulletin of the New Zealand Society for Earthquake Engineering , 34 ( 4 ) : 282 – 317 .
- Smith GM 2004 . The coseismicity and morphology of the Acheron rock avalanche deposit in the Red Hill valley New Zealand . Unpublished MSc thesis. Christchurch, University of Canterbury.
- Smith , GM , Davies , TRH , McSaveney , MJ and Bell , DH . 2006 . The Acheron rock avalanche, Canterbury, New Zealand–morphology and dynamics . Landslides , 3 : 62 – 72 . doi: 10.1007/s10346-005-0012-1
- Soons , JM and Burrows , CJ . 1978 . Dates for Otiran deposits, including plant microfossils and macrofossils, from the Rakaia valley . New Zealand Journal of Geology and Geophysics , 21 : 607 – 615 . doi: 10.1080/00288306.1978.10424088
- Ward , GK and Wilson , SR . 1978 . Procedures for comparing and combining radiocarbon age determinations . Archaeometry , 20 : 19 – 31 . doi: 10.1111/j.1475-4754.1978.tb00208.x
- Whitehouse , IE . 1981 . A large rock avalanche in the Craigeburn Range, Canterbury . New Zealand Journal of Geology and Geophysics , 24 : 415 – 421 .
- Whitehouse , IE . 1983 . Distribution of large rock avalanche deposits in the central Southern Alps, New Zealand . New Zealand Journal of Geology and Geophysics , 26 : 271 – 279 . doi: 10.1080/00288306.1983.10422240
- Whitehouse , IE and Griffiths , GA . 1983 . Frequency and hazard of large rock avalanches in the central Southern Alps, New Zealand . Geology , 11 : 331 – 334 . doi: 10.1130/0091-7613(1983)11%3C331:FAHOLR%3E2.0.CO;2
- Wright , CA . 1998 . The A.D. 930 long run out Round Top debris avalanche, Westland, New Zealand . New Zealand Journal of Geology and Geophysics , 41 : 493 – 497 . doi: 10.1080/00288306.1998.9514826
- Yetton MD 2000 . The probability and consequences of the next Alpine Fault earthquake . Unpublished PhD thesis. Christchurch, University of Canterbury.