Abstract
Latest Eocene–earliest Miocene strata in the subsurface of the Taranaki Basin provide important new regional paleogeographic and tectonic constraints not available from outcrop. Six paleogeographic maps of the Taranaki Basin region have been produced utilising extensive well, seismic reflection and outcrop data. These record three broad periods of sedimentation characterised by (1) variable transgression and initial deformation (c. 40–30 Ma); (2) maximum transgression with moderate deformation (c. 30–21 Ma); and (3) regression with accelerated deformation (< 21 Ma). Local sedimentation patterns were influenced by reverse faulting, producing depocentres and topographic highs adjacent to the Taranaki Fault System. Reverse faulting commenced as early as c. 40 Ma and may signify the onset of subduction beneath the North Island. In common with other parts of New Zealand, the region reached maximum marine inundation in the Waitakian (c. 23 Ma). However, the deposition of thick clastic sediments in eastern parts of Taranaki Basin, coupled with ongoing tectonism, suggests the presence of land throughout the Oligocene and Early Miocene, and is inconsistent with total Oligocene drowning of Zealandia.
Introduction
For many years, paleogeographic models of New Zealand have included significant persistent land areas throughout the Oligocene (e.g. Fleming Citation1962; Kamp Citation1986a; King et al. Citation2011). Recently, Landis et al. (Citation2008) proposed an alternative model in which the New Zealand landmass may have become entirely submerged in the latest Oligocene to earliest Miocene (New Zealand Waitakian Stage, c. 23 Ma). Evidence supporting the ‘total drowning’ hypothesis is mainly derived from onshore outcrops of Oligocene strata which are dominated by limestone successions and devoid of non-marine deposits or strata that indicate the presence of nearby persistent landmasses. In addition to onshore outcrop, subsurface data from both onshore and predominantly offshore areas, in the form of wells and seismic reflection lines, reveal thick Cenozoic successions that provide important geological constraints to test the ‘total drowning’ hypothesis.
The New Zealand mini-continent (referred to here as Zealandia) is currently c. 90% submerged (). Offshore seismic reflection lines and wells show a thick (up to 10 km in some basins) Cretaceous–Cenozoic sequence that comprises strata far more continuous than those encountered onshore (e.g. Nathan et al. Citation1986; Herzer Citation1995; King & Thrasher Citation1996; Field et al. Citation1997; Cook et al. Citation1999). The offshore geology is particularly well resolved in the Taranaki Basin where, due to over 100 years of petroleum exploration and production, there is an extensive dataset of subsurface wells and seismic reflection lines that constrain the region's tectonostratigraphic evolution since c. 100 Ma (e.g. King & Thrasher Citation1992, Citation1996; Giba et al. Citation2010; King et al. Citation2010; Baur Citation2012; Hemmings-Sykes Citation2012). We use these subsurface data in conjunction with outcrop in western North Island and northern South Island (e.g. Nathan et al. Citation1986; Nelson et al. Citation1994; Begg & Johnston Citation2000; Kamp et al. Citation2004; Edbrooke Citation2005; Townsend et al. Citation2008) to construct a series of new paleogeographic maps for the Late Eocene to Early Miocene () covering an area of c. 140,000 km2 (). These maps cover the proposed time of maximum submergence of Zealandia (e.g. Landis et al. Citation2008) and provide important constraints for assessing key geological questions. These include: (1) how did the paleogeography of the Taranaki Basin region evolve through the middle Cenozoic (Late Eocene to Early Miocene) and (2) what does this indicate about the inception and evolution of the plate boundary through New Zealand? (3) What does this evolution reveal about sedimentary and erosional processes east and south of the Taranaki Basin region and (4) do the paleogeographic maps (and their underpinning data) provide additional information that can be used to test the ‘total drowning’ hypothesis? In this paper we present data and interpretation that address these questions.
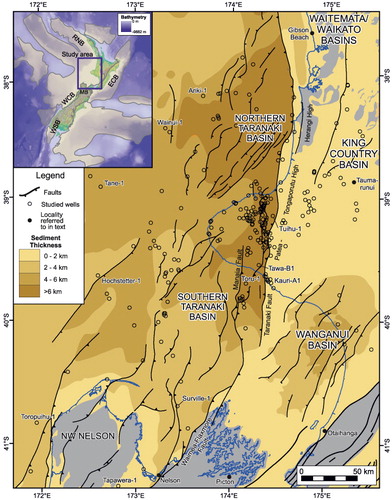
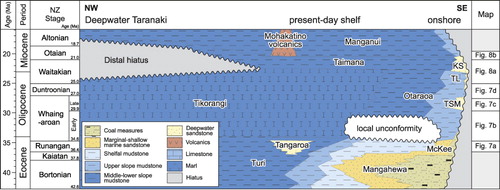
Geological setting
The Taranaki Basin currently lies mostly offshore of western North Island in a predominantly retro-arc foreland basin setting, and is bounded along its eastern margin by the buried crustal-scale Taranaki Fault System which is at least 450 km long (King & Thrasher Citation1996; Stagpoole & Nicol Citation2008). The basin underlies the present-day shelf, but also extends into the deeper waters of the New Caledonia Basin. The basin contains a sedimentary succession of mid-Cretaceous (c. 100 Ma) to Recent age up to 9 km thick (), which records the sedimentation, structure and geological evolution of the region (King & Thrasher Citation1992, Citation1996; Kamp et al. Citation2004; Stagpoole & Nicol Citation2008; King et al. Citation2010; Strogen Citation2011). The Taranaki Basin is one of a series of connected Cretaceous–Cenozoic basins, including the Reinga–Northland (e.g. Isaac et al. Citation1994; Bache et al. Citation2012) and West Coast (e.g. Nathan et al. Citation1986; Kamp et al. Citation1996; Rattenbury et al. Citation1998; Suggate & Waight Citation1999) basins that extend along the western margin of New Zealand (King et al. Citation2011; ). Immediately east of the Taranaki Fault () lie the Waikato–King Country–Wanganui basins (e.g. Anderton Citation1981; Edbrooke et al. Citation1994; Kamp et al. Citation2004; Townsend et al. Citation2008; Tripathi & Kamp Citation2008), which typically contain thinner and younger infill than the Taranaki Basin. In this study, we utilise data from all of these basins.
The Taranaki Basin and wider Zealandia region have undergone a complex tectonic history, from long-lived Paleozoic and Mesozoic subduction, terrane accretion and magmatism, to separation from eastern Gondwana during Cretaceous rifting, and finally to the tectonics associated with convergence across the Australian–Pacific plate boundary that now bisects New Zealand (e.g. Walcott Citation1978; Kamp Citation1986b; Bradshaw Citation1989; King & Thrasher Citation1996; King Citation2000; Wood & Stagpoole Citation2007). Infill of the Taranaki and adjacent basins primarily records continental rifting and passive subsidence related to Gondwana break-up (c. 100–55 Ma) followed by uplift, subsidence and rifting (c. 40–0 Ma) associated with formation of the modern plate boundary through Zealandia.
Across the region of study, the tectonic expression of Cretaceous rifting has been variably overprinted by Australian–Pacific convergent plate boundary tectonics (Nathan et al. Citation1986; King & Thrasher Citation1992, Citation1996; King Citation2000; Ghisetti & Sibson Citation2006; Stagpoole & Nicol Citation2008; Giba et al. Citation2012). Eastern and southern parts of the Taranaki Basin, for example, are within the active plate boundary deformation zone, whereas western parts have remained relatively undeformed since the latest Paleocene (c. 55 Ma) when Tasman Sea opening ceased and passive margin subsidence commenced (Gaina et al. Citation1998).
Contraction in the Taranaki Basin associated with convergence of the Australian and Pacific plates commenced during the Late Eocene to Early Miocene (King & Thrasher Citation1992, Citation1996; Stern et al. Citation1992; Voggenreiter Citation1993; Holt & Stern Citation1994; Stagpoole & Nicol Citation2008). Although the precise timing of the onset of contraction remains a point of debate (see Holt & Stern Citation1994; Stagpoole & Nicol Citation2008; Furlong & Kamp Citation2013), there is wide agreement that the rates of reverse faulting accelerated during the Early Miocene with the highest rates of deformation within the west-verging fold–thrust belt (which include the Taranaki, Tarata Thrust, Manaia and Waimea–Flaxmore faults and are collectively referred to as the Taranaki Fault System), along the eastern margin of the basin (–). In the northern basin, contraction was followed by mainly Middle Miocene and younger normal faulting and volcanism that migrated southwards with time, gradually driving the locus of contraction towards the South Island (King & Thrasher Citation1996; Stagpoole & Nicol Citation2008; Giba et al. Citation2010, Citation2013). The amount of Cenozoic deformation in the Taranaki Basin region is sufficiently low so as to neither mask the earlier patterns of sedimentation nor to significantly change the relative locations of the well and seismic reflection data used to construct the paleogeographic maps. Therefore, the maps are shown relative to the current coastline with all data plotted in their current geographical location.
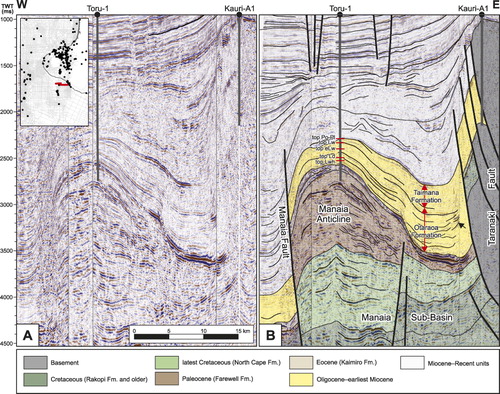
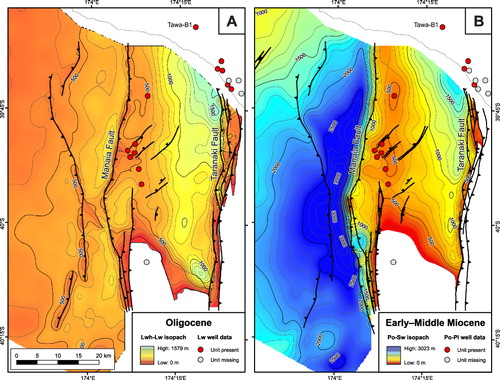
Paleogeographic map production
The paleogeographic maps in and were compiled by interrogating all of the available open-file datasets for the Taranaki Basin and surrounding areas. The wealth of open-file subsurface data available for the Taranaki region, as a result of decades of petroleum exploration and production, is unique in New Zealand and significant globally. These data provide critical observations and constraints for determining the paleogeographic evolution of the region and for testing the ‘total drowning’ hypothesis. These datasets and analyses include: (1) interpretation of 2D and 3D seismic reflection lines (e.g. & ) which were used to generate isopach and structure contour maps (e.g. ); (2) seismic facies analysis of 2D and 3D seismic reflection lines (e.g. Baur Citation2012; Baur et al. Citation2011, Citation2013); (3) reassessment of lithostratigraphic units, lithology, thicknesses, biostratigraphic age and paleobathymetry data in 262 wells (e.g. ) in Taranaki Basin (e.g. Hayward Citation1986; Roncaglia et al. Citation2008, Citation2010; Fohrmann et al. Citation2012); (4) interrogation of existing paleogeographic maps, such as those of Thrasher et al. (Citation1995), King & Thrasher (Citation1996), Higgs et al. (Citation2001) and Morgans et al. (Citation2007); and (5) compilation of outcrop data from the 1 : 250,000 QMAP sheets covering the Auckland (Edbrooke Citation2001), Waikato (Edbrooke Citation2005), Taranaki (Townsend et al. Citation2008), Wellington (Begg & Johnston Citation2000), Wairarapa (Lee & Begg Citation2002), Nelson (Rattenbury et al. Citation1998) and Kaikōura (Rattenbury et al. Citation2006) regions. For more detailed descriptions of the raw data that underpin the paleogeographic maps, including well locations and a full listing of the relevant data sources, see Strogen (Citation2011).
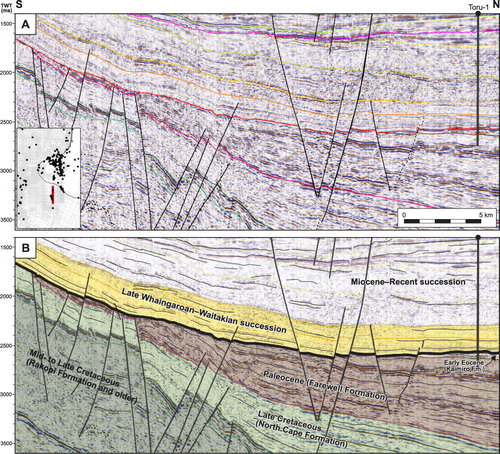
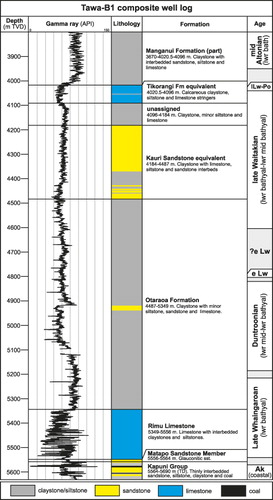
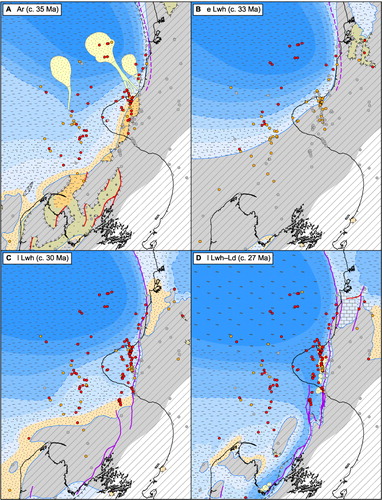
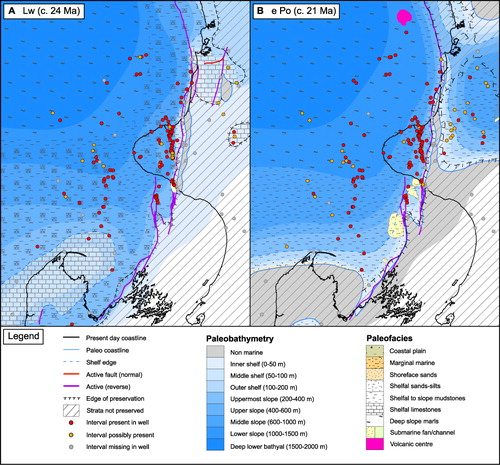
To augment our maps in the regions surrounding the Taranaki Basin, available pre-existing paleogeographic maps have been compiled in a georeferenced ArcGIS database and include maps from Deepwater Taranaki (Uruski et al. Citation2008), the West Coast region (Nathan et al. Citation1986), the Waikato and King Country basins (Edbrooke et al. Citation1994; Cartwright Citation2003; Tripathi & Kamp Citation2008) and from Reinga–Northland Basin (Isaac et al. Citation1994; Stagpoole et al. Citation2009; Bache et al. Citation2012). Six maps illustrating the latest Eocene–earliest Miocene history of the basin are presented in and (see the online supplementary material for larger and more detailed versions; Figs S1–S6), a subset of a more comprehensive suite of 35 maps covering the mid-Cretaceous–Recent geological history of the Taranaki Basin (see Strogen Citation2011). The maps have been assigned approximate absolute ages (in Ma), utilising the updated New Zealand geological timescale of Raine et al. (Citation2012; ). These ages represent roughly the middle of the age range that each map covers, and not a precise age for each map. We have attempted to depict paleobathymetry on the maps even where strata are not preserved. Lithofacies, however, are only shown where strata occur, and are bounded either by a line marking the edge of sediment preservation, or by the paleo-coastline. Non-marine strata are only shown where they are known to exist, or there is a compelling reason to think they are present. Wells and localities named in the text are indicated on .
The paleogeographic maps, together with their underpinning data, provide a basis for considering evolution of the Taranaki Basin. For the Late Eocene to Early Miocene time interval there were three key episodes of basin evolution: (1) transgression and the onset of minor deformation (c. 40–30 Ma, –); (2) maximum transgression with moderate deformation (c. 30–21 Ma, –); and (3) regression with rapid deformation (< 21 Ma, ). These three episodes of basin evolution can be recognised throughout the study area and are particularly important in eastern and southern regions where paleowater depths and total subsidence ranged up to c. 1.5 km and c. 6 km, respectively (). The evidence for, and implications of, these episodes are discussed in the following three sections.
Transgression and onset of deformation
Following the cessation of Gondwana breakup (post c. 55 Ma) the Taranaki Basin experienced 10–15 Myr of tectonic quiescence, with only gradual increases in paleowater depths, associated with thermal subsidence (see Tane-1 subsidence curve, ; Hayward & Wood Citation1989; Strogen Citation2011; Baur et al. Citation2013). Throughout much of the Paleocene and Eocene a coastal plain–shoreface–shallow marine shelf system was established across the central to southwestern Taranaki Basin, resulting in deposition of the proximal Farewell, Kaimiro and Mangahewa formations and the shelfal Turi Formation (King & Thrasher Citation1996; Strogen Citation2011). Gradual transgression across the Taranaki Basin into areas of northwest Nelson, West Coast and the Challenger Plateau, began in the Bortonian (42.6–37.8 Ma) as indicated by the spread of initial coastal plain, and subsequent shallow marine facies into these areas (Strogen Citation2011).
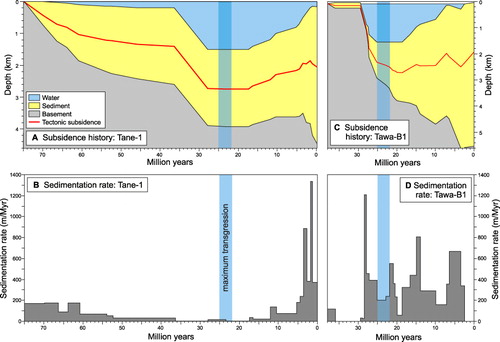
During the Runangan (36.4–34.6 Ma), transgression led to deposition of shelfal Turi Formation mudstones across much of the Taranaki Basin, with limited non- to marginal–marine facies present in southern areas. In northern Taranaki Basin, deeper more basinal Turi Formation facies were present during the Runangan, along with submarine fan sandstones of the Tangaroa Formation (). These represent the oldest fan deposits drilled in the basin, and are up to 150 m thick. Their shape is well constrained by 2D seismic lines, although it is unclear whether they were sourced from the east (Gresko et al. Citation1992) or from the south–southeast (King & Thrasher Citation1996). What triggered the deposition of these fans, which are restricted to the Runangan (Strong Citation2006), during a time of general transgression is unknown, but it is possible that it is linked to the onset of convergent tectonics and a rejuvenation of sediment sources (Baur et al. Citation2013). To the east, in the Waikato–King Country region, coal measures of Late Eocene–Early Oligocene age (Kear & Schofield Citation1959; Nelson Citation1978; Edbrooke et al. Citation1994; Tripathi & Kamp Citation2008) were deposited unconformably on basement. In the Waikato region, the unconformity surface has a valley and ridge morphology that is interpreted to have formed due to stream incision, erosion and uplift (Hall et al. Citation2006) and the age of coal measures resting on basement youngs from Kaiatan in the north to mid-Whaingaroan in the south (Edbrooke et al. Citation1994; Tripathi & Kamp Citation2008). Erosion in the Waikato–King Country region and formation of Runangan fans may have been induced by reverse displacement on the Taranaki Fault. Movement on the fault between c. 40 and 35 Ma is also consistent with paleobathymetry in the northern part of the basin, which steepens and deepens (to > 1000 m) westwards across the Taranaki Fault, with paleobathymetric contours swinging to an orientation parallel to the fault from the Bortonian–Kaiatan onwards (Morgans et al. Citation2007).
The timing of Taranaki Fault movement inferred from the paleobathymetry, erosion and fans is consistent with that of Stagpoole & Nicol (Citation2008), who suggest that faulting was induced by convergence across the Australian–Pacific plate boundary (currently east of the basin) and may mark the onset of subduction. The fault displacement rate during the Kaiatan to Early Whaingaroan (c. 38–30 Ma) was probably significantly lower than during the succeeding Late Whaingaroan–Waitakian (c. 30–21 Ma), as indicated by the more limited influence of the earlier phase on regional paleobathymetric patterns, with no distinct foredeep developed, and also by a lack of evidence for active tectonism onshore in the King Country (Tripathi & Kamp Citation2008). This Late Oligocene–Early Miocene increase in fault displacement rates likely reflects an increase in plate convergence rates (Seebeck Citation2013).
In the northwestern Taranaki Basin, deposition of shelfal to lower-slope mudstones and calcareous mudstones (Otaraoa and Tikorangi formations) in the Early Whaingaroan () represents a continuation of similar, if more calcareous, facies to those of the latest Eocene Turi Formation (King & Thrasher Citation1996; Strogen Citation2011). By contrast, in southern parts of the basin a phase of uplift and erosion also occurred during the Early Oligocene. Rocks of Early Whaingaroan (34.6–29.9 Ma) age are absent in most wells in southern and eastern parts of the basin (grey well symbols, ). Seismic reflection lines throughout the southern basin, as in the vicinity of the Kupe gas/condensate field (), show an angular unconformity (≤5°) overlain by strata of Late Whaingaroan (29.9–27 Ma) age (Bierbrauer Citation2010; Fohrmann et al. Citation2012). Tilted strata beneath the unconformity become progressively older to the south, from Middle Eocene in the north to Late Cretaceous in the south. These angular relationships suggest that higher uplift in the south (compared with the north) resulted in differential erosion. Similar, probable Early Whaingaroan (c. 33–30 Ma) angular unconformities are observed in seismic reflection lines as far west as the Hochstetter-1 well (Baur Citation2012), while poorly dated shallow-marine Whaingaroan strata cropping out at Ōtaihanga close to Wellington (Grant-Taylor & Williams Citation1977) and near Picton in Marlborough (Nicol & Campbell Citation1990) rest with angular uniformity on basement. Therefore, outcrop, well and seismic reflection data record uplift and erosion encompassing a large region of at least c. 100,000 km2. This uplift and erosion event had a limited southward extent beyond the Taranaki Basin, with marine sedimentation continuing throughout the Whaingaroan in the Murchison Basin to the south (Nathan et al. Citation1986). Unconformity formation ceased by c. 30 Ma, with renewed flooding and the deposition of the transgressive Matapo Sandstone Member across much of the southern Taranaki Basin.
On the northern margin of the Challenger Plateau, in the far west of the study area, a prograding (possibly deltaic) unit of latest Eocene or earliest Oligocene age has been identified in seismic data (Uruski et al. Citation2002; Baur Citation2012; Baur et al. Citation2013) and it is possible these prograding sediments are genetically related to Early Oligocene uplift and erosion in the Taranaki Basin region. The magnitude and location of the proposed regional uplift is also consistent with predicted far-field effects of early subduction development (e.g. Baur Citation2012; Baur et al. Citation2013), although the precise location of the subduction system (relative to the Taranaki Basin) and the magnitude of the rate of convergence remain points of debate (see Stagpoole & Nicol Citation2008; Furlong & Kamp Citation2009, Citation2013; Sutherland et al. Citation2010; Baur et al. Citation2013; Seebeck Citation2013).
Maximum transgression during moderate deformation
The Taranaki Basin entered a renewed phase of regional basin subsidence and transgression from the Late Whaingaroan (c. 30 Ma) (, ), which is manifest by a south and eastward advance of marine inundation and overall paleobathymetric deepening of the Taranaki and King Country basins. Transgression culminated in maximum marine submergence during the Waitakian (c. 25–21 Ma).
Well data indicate that, over million-year timescales, sedimentation was continuous throughout the Late Whaingaroan to Waitakian in most areas, although in some distal western parts of the basin the entire Oligocene sequence is highly condensed (≤ 20 m) and may contain significant hiatuses. In northern parts of the basin deposition of distal deep-water clastic and calcareous mudstones of the Otaraoa and Tikorangi formations continued through into the Waitakian. These deep-water deposits give way to shallow marine strata to the east of the Taranaki Fault. Marine limestones on the eastern limb of the Herangi High (), were deposited in the immediate hanging wall of the fault and decrease in dip up-section (Nelson et al. Citation1994). This syn-depositional fanning of strata suggest that the fault accrued displacement during the Late Whaingaroan to Waitakian (Nelson et al. Citation1994; Tripathi & Kamp Citation2008). In the Waikato–King Country region, east of the fault, southward transgression occurred during Late Whaingaroan and Duntroonian time, producing a gradual onlapping and overtopping of coal measures and basement by shelf carbonate and siltstone lithofacies of the Te Kuiti Group (Kear & Schofield Citation1959; Stainton Citation1966; Nelson Citation1978; Edbrooke et al. Citation1994; Cartwright Citation2003). Transgression produced a marine embayment that reached south to the King Country Basin, with shallow- to marginal-marine lithofacies throughout (; Stainton Citation1966; Cartwright Citation2003; Tripathi & Kamp Citation2008). The presence of thin, shallow-marine facies of the Pungapunga Formation (Cartwright Citation2003) cropping out east of Taumarunui suggests a coastline may have lain in this area during the Oligocene. The absence of Waitakian strata across most of this region (Cartwright Citation2003) may indicate that much of it remained emergent across the time of peak marine transgression.
This southward transgression is also recorded offshore, where deep bathyal depths (> 1000 m) became established (; Hayward Citation1985; Hayward & Wood Citation1989; Baur et al. Citation2013) in areas where shelfal water depths had persisted through gradual Paleocene–Eocene deepening (Strogen Citation2011). In distal wells, such as Tane-1 (and also Ariki-1 and Wainui-1; Baur et al. Citation2013), the dating of this rapid deepening is poorly bracketed as Runangan to Whaingaroan (c. 37–28 Ma) due to a lack of biostratigraphic and paleobathymetric resolution ().
In the southern basin, transgression was also occurring by the Late Whaingaroan (30 Ma; ). The initial transgression was marked by the shelfal deposition of the thin (< 50 m) glauconitic Matapo Sandstone Member (of the Otaraoa Formation). Although some workers (Cartwright Citation2003; Tripathi & Kamp Citation2008; Hopcroft Citation2009) have postulated that this member spans the entire Whaingaroan Stage, analysis of available biostratigraphic data from Taranaki wells shows it is of Late Whaingaroan age (Roncaglia et al. Citation2008), consistent with the absence of Early Whaingaroan strata in many parts of the southern basin. The glauconitic Matapo Sandstone Member indicates some stratigraphic condensation and restricted sediment supply into the system, suggesting that any emergent areas in the immediate hinterland were probably only of low relief at this time and not acting as a source of large quantities of sediment. As deposition of the overlying deeper-water mudstones of the Otaraoa Formation began within the Late Whaingaroan (; Higgs et al. Citation2001) sediment starvation and deposition of the Matapo Sandstone Member lasted no more than c. 3 Myr.
In the southern basin the faults of the Taranaki Fault System were strongly influencing the patterns of uplift/subsidence and sediment dispersal by the Late Whaingaroan (c. 30–27 Ma). From this time, rapid subsidence was occurring immediately west of the Taranaki Fault System, with a resultant rapid deepening from shallow-marine conditions, as indicated by the transition from Matapo Sandstone Member to slope mudstones of the Otaraoa Formation (). This subsidence reflects the development of a foredeep, the formation of which has been attributed to the onset of increased rates of reverse displacement on the fault system (King & Thrasher Citation1992; Stern et al. Citation1992; Holt & Stern Citation1994; Stagpoole & Nicol Citation2008). The geometry of the foredeep is clearly illustrated by seismic mapping in southeastern Taranaki Basin, with the Otaraoa Formation thickening into the footwalls of both the Taranaki and Manaia faults from c. 200 m up to 1200 m (–; King & Thrasher Citation1996; Fohrmann et al. Citation2012). Foraminiferal datasets, such as those from the Tawa-B1 well (; Swift Energy Citation2005), show that deep mid-lower bathyal water depths were maintained throughout deposition of the Otaraoa Formation (c. 28–23 Ma) in the footwalls of the Taranaki and Manaia faults. The stability of these water depths indicates that the rates of sedimentation (including sediment compaction) and subsidence were approximately matched, and that the accommodation space locally generated by faulting was not entirely filled by sediments eroded from nearby highs (see also ). The area immediately east of the Taranaki Fault System was one such topographic high, which shed coarse terrigenous sediment across the fault to form the submarine fans of the Late Whaingaroan Tariki Sandstone Member (Higgs et al. Citation2001). Deposition of these thick, coarse-grained fans suggests the presence of a nearby, probably emergent, sediment source in the Late Whaingaroan (). Although deposition of the Tariki Sandstone Member did not continue into Duntroonian, the presence of Duntroonian to Waitakian turbiditic sandstones (the ‘Kauri sandstone’) in the Rimu Field (K. Higgs, pers. comm. 2009) and in the Tuihu-1 and Tawa-B1 wells (Higgs & Morgans Citation2001; Swift Energy Citation2005), all of which are in the immediate footwall of the fault, provide evidence for topographic highs further east, although the precise location of the coastline at this time remains uncertain.
The Taranaki Fault System also influenced sedimentation in the northern South Island during the Late Whaingaroan and Duntroonian stages, and was probably active along a strike length of at least c. 450 km at this time. In the Nelson city area (), rocks of the Magazine Point Formation are interpreted to have been deposited into a narrow foredeep at middle to upper slope depths as part of a submarine fan system (Higgs et al. Citation2004). This foredeep was both narrower and shallower (e.g. Waitakian upper slope facies in well Tapawera-1; Rainey & Waghorn Citation1989) than that next to the Taranaki Fault further north and has been interpreted to have developed during the Duntroonian in response to local subsidence induced by reverse displacement on the Waimea–Flaxmore Fault System.
Outcrops from northern South Island and southern North Island support the view that marine conditions became widespread and extended well beyond the Taranaki Basin in Late Whaingaroan and Duntroonian time. Small outliers of Whaingaroan marginal- to shallow-marine strata crop out at Picton in Marlborough (Nicol & Campbell Citation1990) and at Ōtaihanga close to Wellington (Grant-Taylor & Williams Citation1977). Although the relationship of these small Oligocene outliers to the rest of the Taranaki Basin is unclear due to the large amount of post-Oligocene tectonic deformation within this part of Zealandia (e.g. King Citation2000; Wood & Stagpoole Citation2007), they support the hypothesis of widespread marine incursion in the Whaingaroan. Elsewhere in the northwest Nelson and northern West Coast regions, remnant topographic highs were gradually onlapped during the Late Whaingaroan to Duntroonian and completely submerged by the Waitakian, forming the shelfal Abel Head, Takaka [Limestone] and Matiri formations (Nathan et al. Citation1986). A similar progressive drowning of low-lying basement areas has been mapped offshore of northwest Nelson (King et al. Citation1999). For example, non-marine strata of Whaingaroan–Duntroonian age have been recorded in the Surville-1 well (J.I. Raine, pers. comm. 2011), which are overtopped by shelfal marine strata of Waitakian age. Further south in the Murchison Basin and most of the West Coast, marine conditions prevailed throughout the Duntroonian (Nathan et al. Citation1986) indicating that marine inundation of these areas occurred earlier than further north in the Taranaki Basin.
Maximum transgression was reached during the Waitakian Stage (25–21 Ma) in the Taranaki Basin () and over most of the Zealandia subcontinent (e.g. Landis et al. Citation2008). At this time, sedimentation in the Taranaki Basin was dominated by marine mudstones, limestones and calcareous mudstones of the Otaraoa, Tikorangi and Taimana formations, respectively. In distal parts of the western Taranaki Basin, fine-grained carbonates were deposited in bathyal environments as relatively thin, condensed sequences. The depositional hiatus in the western basin during the Waitakian reflects a low supply of clastic sediment to distal areas, which was probably due to the submergence of much of Zealandia (and the reduction of potential sediment source areas), but also to the preferential trapping of sediments in foredeeps developed along the Taranaki Fault System to the east. Deposition of Waitakian sediments in the accommodation space generated by fault movement is supported by seismic reflection lines (e.g. ). Analysis of the Tikorangi Formation, an oil-bearing reservoir in the TAWN fields of onshore Taranaki Peninsula, suggests that, at least locally, these thickened Waitakian strata comprise shelf-derived skeletal carbonate material shed off a high east of the Taranaki Fault and transported into the foredeep west of the fault (Hood et al. Citation2003). The presence of a carbonate shelf immediately to the east of the fault on the Patea–Tongaporutu High indicates that, at least locally, it was probably submerged at shallow shelfal depths during this time.
While the patterns of sedimentation in the Taranaki Basin during the Late Whaingaroan and Duntroonian were strongly influenced by movement on the Taranaki Fault System, the region of subsidence and transgression extended far beyond the fault to regions including the Challenger Plateau, as shown by the rapid bathymetric deepening of Oligocene strata in distal wells such as Tane-1 () and elsewhere in the distal parts of Taranaki Basin (Hayward & Wood Citation1989; Baur et al. Citation2013). Both movement on the Taranaki Fault and the regional pattern of subsidence are inferred to be related to subduction of the Pacific Plate beneath northern New Zealand, including the Taranaki Basin region (Holt & Stern Citation1994; Stagpoole & Nicol Citation2008; Sutherland et al. Citation2010; Baur Citation2012; Baur et al. Citation2013). Rapid deepening of the basin and surrounding region during the Whaingaroan to Waitakian may reflect a regional lithospheric response to renewed subduction, the ‘pre-arc’ basin of Baur et al. (Citation2013). Potential mechanisms that may explain regional subsidence include: pull-down of the overriding plate due to the onset of frictional resistance to slip on the subduction thrust (e.g. Stern et al. Citation1992); the onset of slab pull and downward convection of the mantle (Hall et al. Citation2003); lithosphere delamination with foundering of lower crust and mantle (Sutherland et al. Citation2010; Baur Citation2012; Baur et al. Citation2013); incipient slab steepening (Seebeck et al. Citation2013); and/or crustal pull-down associated with the formation of a mantle dip due to lithospheric density inversion (Stern et al. Citation2013). Common to many of these models is the suggestion that lithospheric processes resulted in regional subsidence, although the precise nature of these processes remains unclear.
Onset of regression and rapid deformation
An increase in the rates of shortening and uplift commenced throughout much of North Island and northern South Island during the Otaian Stage (21–18.7 Ma) (Rait et al. Citation1991; Lihou Citation1993; King & Thrasher Citation1992, Citation1996; Kamp et al. Citation1996; Field et al. Citation1997; Nicol et al. Citation2007). In Taranaki Basin, accelerated tectonic activity during the Otaian is marked by an increase in displacement rates on the Taranaki Fault System (King & Thrasher Citation1996), particularly on the Manaia Fault (; Hemmings-Sykes Citation2012). This increase in the rates of tectonism resulted in the onset of regional uplift and regression at c. 21 Ma.
Regression in the Taranaki Basin from the Otaian is expressed by the deposition of marls and less calcareous mudstones of the Taimana and Manganui formations. Although predominantly fine-grained, these units can be observed on regional seismic lines (Baur Citation2012) to represent the initial progradation of the slope to shelfal system out into the basin, marking the onset of the overall regressional motif that characterises the Taranaki Basin throughout the Miocene (King & Thrasher Citation1996). In the east of the basin, proximal to the Taranaki Fault System, these formations are thick (c. 3000 m of Otaian to Waiauan strata are seen in the immediate footwall of the Manaia Fault; Fohrmann et al. Citation2012; Hemmings-Sykes Citation2012; ), and record an increase in the rates of sedimentation in the Early–Middle Miocene compared to the Oligocene (compare and ). In the western parts of the basin, distal to uplifted areas and to the Taranaki Fault System, the Taimana Formation is much thinner (generally 10–100 m) and is commonly highly condensed. In the southeast of the basin, adjacent to the Taranaki Fault, thick ‘Kauri sandstone’ fans were deposited (). Seismic facies mapping offshore, adjacent to the southern Manaia Fault, indicates that small fans of Early Miocene age were also present in this area (Baur et al. Citation2011; Baur Citation2012). Otaian–Altonian fans appear to be thicker and more widely distributed than those in the Duntroonian–Waitakian (K. Higgs, pers. comm. 2009), consistent with the view that uplift and erosion increased during the Otaian.
The patterns of Otaian sedimentation in the Taranaki Basin can be mainly attributed to the local growth of reverse faults and folds (Lihou Citation1993; King & Thrasher Citation1996; Stagpoole & Nicol Citation2008; Hemmings-Sykes Citation2012). To the northeast of the Taranaki Basin, uplift and erosion of the Otorohanga Limestone at Gibson Beach close to the Waitakian–Otaian boundary is inferred to have resulted from movement on the nearby Taranaki Fault (Hood & Nelson Citation2012). Following the cessation of movement of the northern end of the Taranaki Fault during the Otaian (Stagpoole & Nicol Citation2008), the Waitemata Basin formed, with initial shallow marine lithofacies deposited across much of the basin (Hayward & Brook Citation1994), succeeded by rapid deepening to bathyal conditions during the early Otaian (Isaac et al. Citation1994). At this time, a depocentre also formed in the King Country Basin to the east of the Taranaki Fault and Patea–Tongaporutu High. Up to 1 km of Mahoenui Group strata accumulated almost entirely within the Otaian Stage (Topping Citation1978). This includes a significant thickness of Taumarunui Formation turbidite sands (Glennie Citation1959; Nelson & Hume Citation1977), which given the maximum 20% sand content of the Mahoenui Group (McQuillan Citation1977), may equate to a sand thickness of up to 200 m. The location of the southern termination of this depocentre remains unclear, due to late Neogene uplift and erosion and the presence of extensive late Quaternary volcaniclastic cover. A topographic high was probably present to the south of the King Country Basin during the Otaian, providing a sediment source for the thick and extensive basin-floor fans of the Taumarunui Formation and the Taumatamaire Mudstone (Cartwright Citation2003; Kamp et al. Citation2004). The present-day distribution of shelfal rhodolith-bearing intra-Mahoenui Group limestones around the Herangi Range, such as the Black Creek and Awakino limestones (Cochrane Citation1988), favours a model in which the Patea–Tongaporutu–Herangi High separating the Taranaki and King Country basins formed an archipelago rather than a single emergent high during the Otaian. Our depiction of the northern termination of the high follows that of Tripathi & Kamp (Citation2008; their map C), rather than that of Cartwright (Citation2003), as the former better fits with paleogeographic data from the Waitemata Basin.
In northern South Island, both basin formation and erosion occurred during the Otaian. depicts uplift and erosion in the northwest Nelson area, following Nathan et al. (Citation1986), although we have expanded the area of erosion to accommodate an unconformity at this stratigraphic level in Toropuihu-1 (Amoco NZ Citation1987). By contrast, marine deposition continued throughout the Otaian in the Murchison Basin to the south (Nathan et al. Citation1986), where local subsidence occurred in the downthrown footwalls of reverse faults. Packages of earliest Miocene rocks thicken towards the footwalls of the faults, indicating active reverse movement, as seen in Taranaki Basin (–).
Discussion
Late Eocene to Early Miocene rocks in the Taranaki Basin record three broad periods of sedimentation: (1) variable transgression along with initial mild deformation (c. 40–30 Ma); (2) subsidence and transgression with moderate fault-related deformation (c. 30–21 Ma); and (3) regression with severe fault-related deformation (from c. 21 Ma). The vertical movements of the continental crust responsible for these broad changes in sedimentation patterns, together with their regional extent, are consistent with the view that they primarily reflect changes in plate boundary tectonic processes. Initial deformation in the Late Eocene may have been triggered by the onset of subduction beneath North Island, with subsequent subsidence and transgression reflecting pull-down of the crust driven by lithospheric movements. Later increases in contraction of the upper plate were partly induced by increases in plate convergence rates. Whatever the precise mechanisms of deformation it is clear that the Taranaki Basin region was tectonically active throughout much of the Late Eocene to Early Miocene, with tectonic quiescence during the Cenozoic being limited mainly to the Early and Middle Eocene (c. 55–40 Ma) in proximal areas (but spanning a much longer time period in distal western areas). Further analysis of the sedimentary record may provide key information for constraining the deformation history; this is particularly true in offshore regions, such as Taranaki Basin, where the stratigraphic record is often more complete than in onshore areas which have experienced Neogene uplift and erosion.
Seismic reflection and well datasets may also provide constraints for the total drowning hypothesis of Landis et al. (Citation2008). In areas of northern onshore Taranaki, such as the TAWN fields, Waitakian strata are dominated by the resedimented shelfal limestones of the Tikorangi Formation, which suggest that any high related to the Taranaki Fault System in this area formed a shallow carbonate-dominated shelf, and therefore was mostly submerged to shallow shelfal water depths at this time. However, further to the south, terrigenous sediment was deposited throughout the Oligocene, including during the Waitakian when peak drowning was postulated to have occurred. Much of this terrigenous sedimentation was deposited in the immediate footwall of the Taranaki Fault System where subsidence rates were locally accelerated by reverse fault movements. The presence of coarse clastics such as the Tariki and ‘Kauri’ sandstones in the footwall of the Taranaki Fault System throughout the Oligocene–earliest Miocene, together with the sheer thickness of terrigenous material in the Kupe () to southern onshore Taranaki region (e.g. Tawa-B1 well; ), suggests the presence of an actively eroding, most likely subaerial, sediment source at this time. A terrestrial source is also supported by the volume and rates of deposition of sediments. For example, in the Tawa-B1 well, Waitakian strata are c. 750 m thick () and were deposited at rates of 200–600 m/Myr (). These rates are comparable with values calculated for the Early–Middle Miocene, when subaerial exposure and associated erosion is widely accepted. We therefore propose that continental crust remained above sea-level east of the Taranaki Basin, although neither the precise location of the shoreline at this time nor the areal extent of land can be estimated with confidence. Collection and analysis of additional offshore well and seismic data may provide additional clues to the extent of land in the Waitakian, although New Zealand-wide Oligocene paleogeographic maps will need to be restored to a state prior to extensive Miocene and younger plate boundary deformation to maximise their utility.
The Taranaki Basin is not the only location across Zealandia where thick Waitakian-aged clastic successions are encountered. The Murchison Basin also records a thick (> 1 km) sequence of Waitakian-aged clastics, which includes a significant component of sandy flysch facies (Crooks & Carter Citation1976; Nathan et al. Citation1986). Thick, Duntroonian–Waitakian clastic units also occur within the East Coast Basin, and include Waitakian sandy flysch facies observed within the Weber Formation in the East Coast Basin (Field et al. Citation1997). Thick (c. 700 m of Waitakian) clastic facies comprising a mix of mudstone, siltstone and sandstone are also recorded in the Waitakian Wheao Formation (both early and late Waitakian) in the Rere-1 well close to Gisborne (de Bock et al. Citation1986), and in a number of wells in the Wairoa area (e.g. Makareao-1; Ian R Brown Associates Citation1998). Flysch facies are also reported from the early Waitakian Puhokio Formation of Makara in coastal central Hawke's Bay (Field et al. Citation1997). Thick clastic flysch facies also occur in Western Southland (Turnbull et al. Citation1993), and whilst platformal Waitakian facies in this region are mainly carbonate dominated, localised deposition of thick clastic basinal facies occurred through the Waitakian in the Waiau, Burwood and Te Anau sub-basins (Norris & Turnbull Citation1993; Turnbull et al. Citation1993). Over 450 m of coarse clastic Waitakian strata were intercepted in the offshore Solander-1 well (BP Oil Exploration Citation1986). These occurrences, along with those in Taranaki Basin, suggest a regionally extensive source of terrigenous sediment within the greater central Zealandia region. Such a major sediment source was probably located east and south of Taranaki Basin in an area that has suffered major Neogene shortening and where no pre-Late Miocene strata are preserved (). It is clear, however, that the Late Oligocene was a period of significant tectonic activity in central Zealandia, and not a period of passive drowning, at least in those areas affected by ongoing plate boundary processes. It would seem very unlikely that such a region of active tectonism would be without areas undergoing uplift and subaerial erosion.
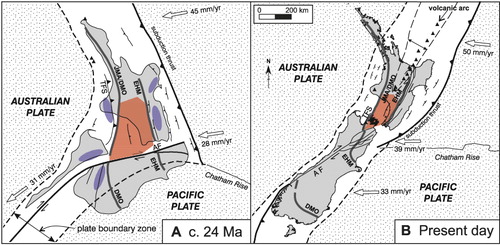
By the earliest Miocene, uplift and emergence of southern areas to the east of the Taranaki Fault System can be demonstrated by the deposition of thick flysch facies of the Mahoenui Group, which clearly required a significant sediment source area. The exact location of the shoreline and the extent of any highs acting as sediment sources are poorly constrained. A similar intensification in tectonics and coarse-grained sedimentation is recorded in the East Coast Basin, with the thick flysch facies of the Whakataki Formation, which are as old as late Waitakian (i.e. earliest Miocene) and extend into the Otaian and Altonian (Field et al. Citation1997).
As yet, no terrestrial facies of Duntroonian or Waitakian age have been identified in the region of study (). The youngest (pre-Middle Miocene) non-marine facies positively identified in the greater Taranaki Basin region are of mid-Whaingaroan (King Country, Benneydale Coal Measures; Nelson Citation1978; Edbrooke et al. Citation1994) or Late Whaingaroan–Duntroonian age (coastal plain facies in Surville-1 in southern Taranaki Basin). However, Duntroonian–Waitakian paleogeography is very poorly constrained throughout central Zealandia due to severe post-Oligocene deformation, the scattered spatial distribution of Oligocene rocks and poor age control. This may be exacerbated by apparent uncertainties in the correlation of non-marine and marine biozones throughout the Oligocene (D. Mildenhall, pers. comm. 2013). Therefore, further work is required to better define the position of latest Oligocene–earliest Miocene shorelines and to determine the sources of clastic sediment during maximum flooding.
Acceleration in the regional rates of uplift, erosion and tectonic shortening during the Otaian is often attributed to the formation of a through-going plate boundary, connecting an active subduction zone in the northeast to the proto-Alpine Fault zone (e.g. Walcott Citation1978; Kamp Citation1986b; Rait et al. Citation1991; King Citation2000). This hypothesis is consistent with a Waitakian–Otaian (c. 25–20 Ma) inferred age of inception of the Alpine Fault (Kamp Citation1986b; Cooper et al. Citation1987) and possibly also with the commencement of subduction beneath North Island at c. 30–24 Ma (Ballance Citation1976; Rait et al. Citation1991; Field et al. Citation1997; Kamp Citation1999; Stern et al. Citation2006). However, we believe that mild contractional deformation in the Taranaki Basin region between c. 40 and 30 Ma may signal the onset of subduction beneath North Island. This is supported by the folding of Late Eocene age seen in the Reinga–Northland Basin (Bache et al. Citation2012) as well as the anomalous subsidence in the distal Taranaki and New Caledonia basins (Baur et al. Citation2013), both of which have been linked to the onset of subduction during the Late Eocene to Early Oligocene.
A pre-Otaian age for the inception of subduction is supported by the onset of arc volcanism in Northland and the northern Taranaki Basin at c. 24–20 Ma (Herzer Citation1995; Hayward & Smale Citation1992; Giba et al. Citation2013; ). Given the rates of Australian–Pacific plate convergence estimated from seafloor spreading data (Cande & Stock Citation2004), subduction of the Pacific Plate may have commenced ≥ 38 Ma in order to reach the 100 km depths necessary to produce arc volcanism by this time (Seebeck Citation2013). If subduction beneath North Island initiated during the Late Eocene–Early Oligocene, then an alternative explanation is required for the onset of rapid deformation across North Island and the Taranaki Basin region in the Otaian. We propose that this acceleration of deformation could result in part from an increase in the rate of plate convergence during the Otaian associated with southward migration of the Euler pole that describes the motion of the Pacific Plate relative to the Australian Plate in the New Zealand region (Seebeck Citation2013).
Conclusions
An abundance of subsurface petroleum exploration data in Taranaki Basin allow the latest Eocene–earliest Miocene geological evolution to be studied in detail. This is critical to understanding the ‘total drowning’ hypothesis and the regional tectonic evolution, due to poor outcrop control through this time period in central parts of Zealandia. Three main phases of basin evolution have been distinguished:
1. Variable transgression and initial deformation (Runangan to Early Whaingaroan)
Subsidence largely continued the Late Eocene trend of gradual deepening, but was punctuated by rapid Early Oligocene deepening, observed in northwestern wells. Coastal plain and shallow marine facies spread southward through the Waikato region. A short-lived uplift event in the southern part of the basin, suggested by localised unconformities and an absence of Early Whaingaroan strata, was followed by southward-directed transgression. Displacement on the Taranaki Fault System affected paleobathymetry in northern parts of the basin from the Late Eocene, although deformation was more subtle than that seen from the Late Whaingaroan onwards.
2. Maximum transgression accompanying moderate deformation (Late Whaingaroan–Waitakian)
Transgression reached its maximum extent in the Waitakian, with inundation and carbonate deposition across distal areas, northwest Nelson and much of the Waikato–King Country region; the typical Waitakian ‘drowned Zealandia’ sedimentary motif. However, intensification of activity on the Taranaki Fault System created rapidly subsiding foredeeps adjacent to faults, where thick bathyal mudstones and sandy turbidite units were deposited. The presence of these sandstone facies, as well as high sedimentation rates through the Waitakian suggest an emergent sediment source in the hinterland. Similar thick clastic units, including significant sandstone facies, elsewhere in central Zealandia, supports the presence of a significant area of erosion supplying sediment to basins.
3. Onset of regression and rapid deformation (early Otaian onwards)
In the early Otaian, a further increase in the rate of tectonic deformation occurred, with renewed uplift in northwest Nelson and to the south of the King Country Basin, where a short-lived basin accumulated c. 1 km of mudstones and flysch deposits. Thin marls were deposited in distal areas of the Taranaki Basin, but strata thicken towards the Taranaki Fault System, where thick mudstones are interbedded with thick deep-water fan sands.
Supplementary files
Figure file 1: Figure 1. Runangan paleogeographic map (c. 35 Ma, Priabonian).
Figure file 2: Figure 2. Early Whaingaroan paleogeographic map (c. 33 Ma, Rupelian).
Figure file 3: Figure 3. Late Whaingaroan paleogeographic map (c. 30 Ma, late Rupelian-early Chattian).
Figure file 4: Figure 4. latest Whaingaroan–Duntroonian paleogeographic map (c. 27 Ma, early Chattian).
Figure file 5: Figure 5. Waitakian paleogeographic map (24 Ma, Chattian-Aquitanian).
Figure file 6: Figure 6. Early Otaian paleogeographic map (21 Ma, late Aquitanian).
Set of six full size latest Eocene–Early Miocene paleogeographic maps.
Download PDF (6.4 MB)Acknowledgements
This study forms part of ongoing research into the evolution of New Zealand's sedimentary basins, within the Petroleum Basin Research (PBR) programme at GNS Science, funded by direct core funding provided to GNS Science by the New Zealand Government, and previously via the Foundation for Research and Technology (contracts C05X0302 and C05X0905). This paper is based upon the work of, and has benefitted from, valuable discussions with many colleagues throughout the Petroleum Geoscience and Paleontology departments at GNS Science. Thanks to Karsten Kroeger, Malcolm Arnot and Steve Edbrooke for internal reviews of earlier versions of this paper and to Cam Nelson and Mike Isaac for their helpful and insightful reviews.
References
- Amoco NZ Exploration Co Ltd 1987. Toropuihi-1 final well report PPL 38058. New Zealand Unpublished Openfile Petroleum Report PR1288. 789 p + 14 enclosures.
- Anderton PW 1981. Structure and evolution of the South Wanganui Basin, New Zealand. New Zealand Journal of Geology and Geophysics 24: 39–63. 10.1080/00288306.1981.10422697
- Bache F, Sutherland R, Stagpoole V, Herzer R, Collot J, Rouillard P 2012. Stratigraphy of the southern Norfolk Ridge and the Reinga Basin: a record of initiation of Tonga-Kermadec-Northland subduction in the southwest Pacific. Earth and Planetary Science Letters 321–322: 41–53.
- Ballance PF 1976. Evolution of the Upper Cenozoic magmatic arc and plate boundary in northern New Zealand. Earth and Planetary Science Letters 28: 356–370. 10.1016/0012-821X(76)90197-7
- Baur JR 2012. Regional seismic attribute analysis and tectono-stratigraphy of offshore south-western Taranaki Basin, New Zealand. Unpublished PhD thesis. Wellington, Victoria University of Wellington. 391 p.
- Baur JR, King PR, Stern T, Leitner B 2011. Development and seismic geomorphology of a Miocene slope channel megasystem, Offshore Taranaki Basin, New Zealand. In: Wood L, Simo T, Rosen N ed. Seismic imaging of depositional and geomorphic systems: SEPM Foundation, Gulf Coast Section, 30th Annual Research Conference Proceedings. Houston, TX, GCSSEPM. Pp. 618–649.
- Baur J, Sutherland R, Stern T 2013. Anomalous passive subsidence of deep-water sedimentary basins: a prearc basin example, southern New Caledonia Trough and Taranaki Basin, New Zealand. Basin Research 25: 242–268.
- Begg JG, Johnston MR 2000. Geology of the Wellington area. Institute of Geological & Nuclear Sciences 1:250,000 geological map 10. Lower Hutt, Institute of Geological & Nuclear Sciences. 64 p + 1 map.
- Bierbrauer K 2010. Eocene deformation and erosion in the southern Taranaki Basin – implications for a tectonic subdivision of the Kapuni Group. New Zealand Petroleum Conference Proceedings 2010. Wellington, Ministry of Economic Development. 25 p.
- BP Oil Exploration Co (NZ) Ltd 1986. Well completion report. Solander-1. PPL 38206. New Zealand Unpublished Openfile Petroleum Report PR1149. 595 p.
- Bradshaw JD 1989. Cretaceous geotectonic patterns in the New Zealand region. Tectonics 8: 803–820. 10.1029/TC008i004p00803
- Cande S, Stock J 2004. Pacific–Antarctic–Australian motion and the formation of the Macquarie Plate. Geophysical Journal International 157: 399–414. 10.1111/j.1365-246X.2004.02224.x
- Cartwright SJ 2003. Cenozoic geological evolution of the central eastern King Country Basin, North Island. Unpublished MSc thesis. Hamilton, The University of Waikato. 200 p.
- Cochrane PR 1988. The stratigraphy and structure of the Early Miocene Mahoenui Group northern Awakino Gorge: controls on cyclic carbonate-terrigenous sedimentation. Unpublished MSc thesis. Hamilton, The University of Waikato. 240 p.
- Cook RA, Sutherland R, Zhu H, Funnell RH, Killops SD 1999. Cretaceous-Cenozoic geology and petroleum systems of the Great South Basin, New Zealand. Institute of Geological & Nuclear Sciences Monograph 20. Lower Hutt, Institute of Geological & Nuclear Sciences. 188 p + 2 enclosures.
- Cooper A, Barreiro B, Kimbrough DL, Mattinson JM 1987. Lamprophyre dike intrusion and the age of the Alpine fault, New Zealand. Geology 15: 941–944. 10.1130/0091-7613(1987)15%3C941:LDIATA%3E2.0.CO;2
- Crooks I, Carter RM 1976. Stratigraphy of Maruia and Matiri Formations in their type section (Trent Stream, Matiri river, Murchison). Journal of the Royal Society of New Zealand 6: 459–487. 10.1080/03036758.1976.10421485
- de Bock JF, Kelly C, Lock R, Bulte G 1986. Well completion report Rere-1 PPL 38082. New Zealand Unpublished Openfile Petroleum Report PR1231. 1202 p + 8 enclosures.
- Edbrooke SW 2001. Geology of the Auckland area. Institute of Geological & Nuclear Sciences 1:250,000 geological map 3. Lower Hutt, Institute of Geological & Nuclear Sciences. 74 p + 1 map.
- Edbrooke SW 2005. Geology of the Waikato area. Institute of Geological & Nuclear Sciences 1:250,000 geological map 4. Lower Hutt, Institute of Geological & Nuclear Sciences. 68 p + 1 map.
- Edbrooke SW, Sykes R, Pocknall DT 1994. Geology of the Waikato Coal Measures, Waikato Coal Region, New Zealand. Institute of Geological & Nuclear Sciences Monograph 6. Lower Hutt, Institute of Geological & Nuclear Sciences. 236 p + 8 maps.
- Field BD, Uruski CI, Beu AG, Browne GH, Crampton JS, Funnell RH et al. 1997. Cretaceous-Cenozoic geology and petroleum systems of the East Coast region, New Zealand. Lower Hutt, Institute of Geological & Nuclear Sciences Monograph 19: 2 v.
- Fleming CA 1962. New Zealand biogeography: a paleontologist's approach. Tuatara 10: 53–108.
- Fohrmann M, Hill M, Reid E, King PR, Zhu H, Bland KJ et al. 2012. Seismic reflection character, mapping and tectono-stratigraphic history of the Kupe area (4D Taranaki Project), south-eastern Taranaki Basin. GNS Science Report, 2012/36. 62 p.
- Furlong KP, Kamp PJJ 2009. The lithospheric geodynamics of plate boundary transpression in New Zealand: initiating and emplacing subduction along the Hikurangi margin, and the tectonic evolution of the Alpine Fault system. Tectonophysics 474: 449–462. 10.1016/j.tecto.2009.04.023
- Furlong KP, Kamp PJJ 2013. Changes in plate boundary kinematics: punctuated or smoothly varying — evidence from the mid-Cenozoic transition from lithospheric extension to shortening in New Zealand. Tectonophysics 608: 1328–1342. 10.1016/j.tecto.2013.06.008
- Gaina C, Müller DR, Royer J-Y, Stock J, Hardebeck J, Symonds P 1998. The tectonic history of the Tasman Sea: a puzzle with 13 pieces. Journal of Geophysical Research 103: 12413–12433. 10.1029/98JB00386
- Ghisetti FC, Sibson RH 2006. Accommodation of compressional inversion in north-western South Island (New Zealand): old faults versus new? Journal of Structural Geology 28: 1994–2010. 10.1016/j.jsg.2006.06.010
- Giba M, Nicol A, Walsh JJ 2010. Evolution of faulting and volcanism in a back-arc basin and its implications for subduction processes. Tectonics 29: TC2040. 18 p.
- Giba M, Walsh JJ, Nicol A 2012. Segmentation and growth of an obliquely reactivated normal fault. Journal of Structural Geology 39: 253–267. 10.1016/j.jsg.2012.01.004
- Giba M, Walsh JJ, Nicol A, Mouslopoulou V, Seebeck H 2013. Investigation of the spatio-temporal relationship between normal faulting and arc volcanism on million-year timescales. Journal of the Geological Society of London 170: 951–962. 10.1144/jgs2012-121
- Glennie KW 1959. The graded sediments of the Mahoenui Formation (King Country, North Island). New Zealand Journal of Geology and Geophysics 2: 613–621. 10.1080/00288306.1959.10423164
- Grant-Taylor TL, Williams DN 1977. The Otaihanga outlier: a re-interpretation. NZ Geological Survey Staff Conference. 6 p.
- Gresko MJ, Jordan DW, Thompson PR 1992. Sequence stratigraphic analysis of Tangaroa sandstone, northern Taranaki Basin. In: New Zealand Petroleum Conference Proceedings 1991. Wellington, Ministry of Commerce. Pp. 234–244.
- Hall CE, Gurnis M, Sdrolias M, Lavier LL, Müller RD 2003. Catastrophic initiation of subduction following forced convergence across fracture zones. Earth and Planetary Science Letters 212: 15–30. 10.1016/S0012-821X(03)00242-5
- Hall SL, Nicol A, Moore TA, Bassett KN 2006. Timing of normal faulting in the Waikato Coal Measures, New Zealand, and its implications for coal-seam geometry. New Zealand Journal of Geology and Geophysics 49: 101–113. 10.1080/00288306.2006.9515151
- Hayward BW 1985. Foraminiferal biostratigraphy and paleobathymetry of Tane-1 Offshore Well, West Taranaki. PPL 38114. New Zealand Unpublished Openfile Petroleum Report PR1131. 54 p.
- Hayward BW 1986. A guide to paleoenvironmental assessment using New Zealand Cenozoic foraminiferal faunas. New Zealand Geological Survey report PAL 109. Lower Hutt, New Zealand Geological Survey. 73 p.
- Hayward BW, Brook FJ 1994. Foraminiferal paleoecology and initial subsidence of the early Miocene Waitemata Basin, Waiheke Island, Auckland. New Zealand Journal of Geology and Geophysics 37: 11–24. 10.1080/00288306.1994.9514597
- Hayward BW, Smale D 1992. Heavy minerals and the provenance history of Waitemata Basin sediments (early Miocene, Northland, New Zealand). New Zealand Journal of Geology and Geophysics 35: 223–242. 10.1080/00288306.1992.9514516
- Hayward BW, Wood RA 1989. Computer-generated geohistory plots for Taranaki drillhole sequences. New Zealand Geological Survey report PAL 147. Lower Hutt, New Zealand Geological Survey. 73 p.
- Hemmings-Sykes S 2012. The influence of faulting on hydrocarbon migration in the Kupe area, south Taranaki Basin, New Zealand. Unpublished MSc thesis. Wellington, Victoria University of Wellington. 198 p.
- Herzer RH 1995. Seismic stratigraphy of a buried volcanic arc, Northland, New Zealand and implications for Neogene subduction. Marine and Petroleum Geology 12: 511–531. 10.1016/0264-8172(95)91506-K
- Higgs KE, Morgans HEG 2001. Tuihu-1 well study. New Zealand Unpublished Openfile Petroleum Report PR4541. 49 p.
- Higgs KE, Morgans HEG, King PR, Browne GH 2001. A reservoir study of the Tariki sandstone, Onshore Taranaki. New Zealand Unpublished Openfile Petroleum Report PR2616. 350 p + 19 enclosures.
- Higgs KE, King PR, Browne GH, Morgans HEG 2004. Oligocene submarine fan systems in Taranaki: a proven reservoir and an outcrop comparison. New Zealand Petroleum Conference Proceedings 2004. Ministry of Economic Development, Wellington. 10 p.
- Holt WE, Stern TA 1994. Subduction, platform subsidence, and foreland thrust loading: the late Tertiary development of Taranaki Basin, New Zealand. Tectonics 13: 1068–1092. 10.1029/94TC00454
- Hood SD, Nelson CS 2012. Temperate carbonate debrites and short-lived earliest Miocene yo-yo tectonics, eastern Taranaki Basin margin, New Zealand. Sedimentary Geology 247–248: 58–70.
- Hood SD, Nelson CS, Kamp PJJ 2003. Lithostratigraphy and depositional episodes of the Oligocene carbonate-rich Tikorangi Formation, Taranaki Basin, New Zealand. New Zealand Journal of Geology and Geophysics 46: 363–386. 10.1080/00288306.2003.9515015
- Hopcroft BS 2009. Lithology and provenance of Late Eocene-Oligocene sediments in eastern Taranaki Basin margin and implications for paleogeography. Unpublished MSc thesis. Hamilton, The University of Waikato. 176 p.
- Ian R Brown Associates Ltd 1998. Makareao-1 Well Completion Report, PEP 38329. New Zealand Unpublished Openfile Petroleum Report PR2377. 295 p + 4 enclosures.
- Isaac MJ, Herzer RH, Brook FJ, Hayward BW 1994. Cretaceous and Cenozoic sedimentary basins of Northland, New Zealand. Institute of Geological & Nuclear Sciences Monograph 8. Lower Hutt, Institute of Geological & Nuclear Sciences. 230 p.
- Kamp PJJ 1986a. Late Cretaceous-Cenozoic tectonic development of the southwest Pacific region. Tectonophysics 121: 225–251. 10.1016/0040-1951(86)90045-4
- Kamp PJJ 1986b. The mid-Cenozoic Challenger Rift system of western New Zealand and its implications for the age of Alpine Fault inception. Geological Society of America Bulletin 97: 255–281. 10.1130/0016-7606(1986)97%3C255:TMCRSO%3E2.0.CO;2
- Kamp PJJ 1999. Tracking crustal processes by FT thermochronology in a forearc high (Hikurangi margin, New Zealand) involving Cretaceous subduction termination and mid-Cenozoic subduction initiation. Tectonophysics 307: 313–343. 10.1016/S0040-1951(99)00102-X
- Kamp PJJ, Webster KS, Nathan S 1996. Thermal history analysis by integrated modelling of apatite fission track and vitrinite reflectance data: application to an inverted basin (Buller Coalfield, New Zealand). Basin Research 8: 383–402.
- Kamp PJJ, Vonk AJ, Bland KJ, Hansen RJ, Hendy AJW, McIntyre AP et al. 2004. Neogene stratigraphic architecture and tectonic evolution of Wanganui, King Country, and eastern Taranaki basins, New Zealand. New Zealand Journal of Geology and Geophysics 47: 625–644. 10.1080/00288306.2004.9515080
- Kear D, Schofield JC 1959. Te Kuiti Group. New Zealand Journal of Geology and Geophysics 2: 685–717. 10.1080/00288306.1959.10422764
- King PR 2000. Tectonic reconstructions of New Zealand 40 Ma to the present. New Zealand Journal of Geology and Geophysics 43: 611–638. 10.1080/00288306.2000.9514913
- King PR, Thrasher GP 1992. Post-Eocene development of the Taranaki Basin, New Zealand; convergent overprint of a passive margin. In: Watkins JS, Zhiqiang F, McMillen KJ ed. Geology and geophysics of continental margins. AAPG Memoir 53: 93–118.
- King PR, Thrasher GP 1996. Cretaceous-Cenozoic geology and petroleum systems of the Taranaki Basin, New Zealand. Institute of Geological & Nuclear Sciences Monograph 13. Lower Hutt, Institute of Geological & Nuclear Sciences. 243 p + 6 enclosures.
- King PR, Field BD, Sutherland R, Wood RA, Zhu H 1999. Late Cretaceous-Early Cenozoic foundering of the New Zealand sub-continent. In: Wallace C ed. Geological Society of New Zealand Inc 1999 Annual Conference, 29 November–1 December, Massey University, Palmerston North: programme and abstracts. Geological Society of New Zealand miscellaneous publication, 107A. 81 p.
- King PR, Naish TR, Browne GH, Field BD, Edbrooke SW ( compilers) 2011. Cretaceous to Recent sedimentary patterns in New Zealand. Digitally remastered version. Institute of Geological & Nuclear Sciences Folio Series 1a. Lower Hutt, Institute of Geological & Nuclear Sciences. 1 CD.
- King PR, Thrasher GP, Bland KJ, Carthew P, D'Cruz D, Griffin AG et al. 2010. Cretaceous-Cenozoic geology and petroleum systems of the Taranaki Basin, New Zealand. Digitally remastered version. Institute of Geological & Nuclear Sciences Monograph 13. Lower Hutt, Institute of Geological & Nuclear Sciences. 1 CD.
- Landis CA, Campbell HJ, Begg JG, Mildenhall DC, Paterson AM, Trewick SA 2008. The Waipounamu erosion surface: questioning the antiquity of the New Zealand land surface and terrestrial fauna and flora. Geological Magazine 145: 173–197. 10.1017/S0016756807004268
- Lee JM, Begg JG 2002. Geology of the Wairarapa area. Institute of Geological & Nuclear Sciences 1:250,000 geological map 11. Lower Hutt, Institute of Geological & Nuclear Sciences. 66 p + 1 map.
- Lihou JC 1993. The structure and deformation of the Murchison Basin, South Island, New Zealand. New Zealand Journal of Geology and Geophysics 36: 95–105. 10.1080/00288306.1993.9514557
- McQuillan H 1977. Hydrocarbon potential of the North Wanganui Basin, New Zealand. The APEA Journal 17: 94–104.
- Morgans HEG, Raine JI, Schiøler P, Hollis CJ 2007. Paleoenvironmental and paleogeographic review of nine wells from North Taranaki Bight. New Zealand Unpublished Openfile Petroleum Report PR4585. 67 p.
- Nathan S, Anderson HJ, Cook RA, Herzer RH, Hoskins RH, Raine JI et al. 1986. Cretaceous and Cenozoic sedimentary basins of the West Coast region, South Island, New Zealand. New Zealand Geological Survey Basin Studies, 1. Wellington. 89 p.
- Nelson CS 1978. Stratigraphy and paleontology of Oligocene Te Kuiti Group, Waitomo County, South Auckland, New Zealand. New Zealand Journal of Geology and Geophysics 21: 553–594. 10.1080/00288306.1978.10424086
- Nelson CS, Hume TM 1977. Relative intensity of tectonic events revealed by the tertiary sedimentary record in the North Wanganui Basin and adjacent areas, New Zealand. New Zealand Journal of Geology and Geophysics 20: 369–392. 10.1080/00288306.1977.10420714
- Nelson CS, Kamp PJJ, Young HR 1994. Sedimentology and petrography of mass-emplaced limestone (Orahiri Limestone) on a late Oligocene shelf, western North Island, and tectonic implications for eastern margin development of Taranaki Basin. New Zealand Journal of Geology and Geophysics 37: 269–285. 10.1080/00288306.1994.9514621
- New Zealand Petroleum & Minerals 2013. New Zealand petroleum basins. Wellington, New Zealand, Ministry of Business, Innovation & Employment. 104 p.
- Nicol A, Campbell JK 1990. Late Cenozoic thrust tectonics, Picton, New Zealand. New Zealand Journal of Geology and Geophysics 33: 485–494. 10.1080/00288306.1990.10425703
- Nicol A, Mazengarb C, Chanier F, Rait G, Uruski CI, Wallace LM 2007. Tectonic evolution of the active Hikurangi subduction margin, New Zealand, since the Oligocene. Tectonics 26: TC4002. 10.1029/2006TC002090
- Norris RJ, Turnbull IM 1993. Cenozoic basins adjacent to an evolving transform plate boundary, southwest New Zealand. In: Ballance PF ed. South Pacific sedimentary basins. Sedimentary basins of the world, Vol. 2. Amsterdam, Elsevier Science Publishers. Pp. 251–270.
- Raine JI, Beu AG, Boyes AF, Campbell HJ, Cooper RA, Crundwell MP et al. 2012. New Zealand Geological Timescale v. 2012/1. Lower Hutt, GNS Science. 1 folded chart.
- Rainey S, Waghorn D 1989. Tapawera-1 well completion report, PPL38500. New Zealand Unpublished Openfile Petroleum Report PR1445. 143 p +, 12 enclosures.
- Rait G, Chanier F, Waters DW 1991. Landward- and seaward-directed thrusting accompanying the onset of subduction beneath New Zealand. Geology 19: 230–233. 10.1130/0091-7613(1991)019%3C0230:LASDTA%3E2.3.CO;2
- Rattenbury MS, Cooper RA, Johnston MR 1998. Geology of the Nelson area. Institute of Geological & Nuclear Sciences 1:250,000 geological map 9. Lower Hutt, Institute of Geological & Nuclear Sciences. 67 p + 1 map.
- Rattenbury MS, Townsend DB, Johnston MR 2006. Geology of the Kaikoura area. Institute of Geological & Nuclear Sciences 1:250,000 geological map 13. Lower Hutt, Institute of Geological & Nuclear Sciences. 70 p + 1 map.
- Roncaglia L, Milner M, Baur J, Fohrmann M, Kroeger K, Strogen DP et al. 2010. Procedures and metadata protocols used in modelling Taranaki Basin petroleum systems: guidelines from a pilot case study in the Kupe area. GNS Science Report, 2009/49. Lower Hutt, GNS Science. 94 p.
- Roncaglia L, Morgans HEG, Arnot MJ, Baur J, Zhu H, Bushe H et al. 2008. Stratigraphy, well correlation and seismic-to-well tie in the Upper Cretaceous to Pliocene interval in the Kupe region, Taranaki Basin, New Zealand. GNS Science Report, 2008/07. Lower Hutt, GNS Science 24 p.
- Seebeck HC 2013. Normal faulting, volcanism, and fluid-flow, Hikurangi subduction plate boundary, New Zealand. Unpublished PhD thesis. Christchurch, University of Canterbury. 264 p.
- Seebeck HC, Nicol A, Giba MO, Pettinga JR, Walsh JJ 2013. Geometry of the subducting Pacific plate since 20 Ma, Hikurangi margin, New Zealand. Journal of the Geological Society 171: 131–143.
- Shell BP Todd Oil Services Ltd 1976. Well resume Tane-1 (offshore). New Zealand Unpublished Openfile Petroleum Report PR698. 264 p + 5 enclosures.
- Stagpoole VM, Nicol A 2008. Regional structure and kinematic history of a large subduction back thrust: Taranaki Fault, New Zealand. Journal of Geophysical Research: Solid Earth 113: B01403.
- Stagpoole VM, Browne GH, Ilg B, Herzer RH, Reid E, Bland KJ et al. 2009. Petroleum prospectivity of the Reinga Basin. New Zealand Unpublished Openfile Petroleum Report PR4037. 112 p.
- Stainton PW 1966. Mangaotaki and Te Kuiti formations of the North Wanganui Basin: sedimentological study. New Zealand Unpublished Openfile Petroleum Report PR458. 13 p + 32 enclosures.
- Stern T, Houseman G, Salmon M, Evans L 2013. Instability of a lithospheric step beneath western North Island, New Zealand. Geology 41: 423–426. 10.1130/G34028.1
- Stern TA, Quinlan GM, Holt WE 1992. Basin formation behind an active subduction zone: three dimensional flexural modelling of Wanganui Basin, New Zealand. Basin Research 4: 197–214. 10.1111/j.1365-2117.1992.tb00045.x
- Stern TA, Stratford WR, Salmon ML 2006. Subduction evolution and mantle dynamics at a continental margin: Central North Island, New Zealand. Reviews of Geophysics 44: RG4002. 10.1029/2005RG000171
- Strogen DP ( compiler) 2011. Updated paleogeographic maps for the Taranaki Basin and surrounds. GNS Science Report, 2010/53. Lower Hutt, GNS Science. 83 p.
- Strong CP 2006. Foraminiferal biostratigraphy of the Tangaroa Sandstone, offshore North Taranaki. New Zealand Unpublished Openfile Petroleum Report PR3645. 23 p.
- Suggate RP, Waight TE 1999. Geology of the Kumara-Moana area, Institute of Geological & Nuclear Sciences map 24. Lower Hutt, Institute of Geological & Nuclear Sciences. 124 p + 1 map.
- Sutherland R, Collot J, Lafoy Y, Logan GA, Hackney R, Stagpoole V et al. 2010. Lithosphere delamination with foundering of lower crust and mantle caused permanent subsidence of New Caledonia Trough and transient uplift of Lord Howe Rise during Eocene and Oligocene initiation of Tonga-Kermadec subduction, western Pacific. Tectonics 29: TC2004.
- Swift Energy New Zealand Ltd 2005. Tawa B-1; Tawa B-1 ST1 & Tawa B-1 ST2. New Zealand Unpublished Openfile Petroleum Report PR3207. 1163 p + 6 enclosures.
- Thrasher GP, King PR, Cook RA 1995. Taranaki Basin petroleum atlas. Institute of Geological & Nuclear Sciences. Lower Hutt, Institute of Geological & Nuclear Sciences. 50 maps + booklet.
- Topping RM 1978. Foraminifera from the Mahoenui Group, North Wanganui Basin. Unpublished PhD thesis. Auckland, University of Auckland. 465 p.
- Townsend D, Vonk AJ, Kamp PJJ 2008. Geology of the Taranaki area. Institute of Geological & Nuclear Sciences 1:250,000 geological map 7. Lower Hutt, Institute of Geological and Nuclear Sciences. 77 p + 1 map.
- Tripathi ARP, Kamp PJJ 2008. Timing of initiation of reverse displacement on the Taranaki Fault, northern Taranaki Basin: constraints from the on land record (Oligocene Te Kuiti Group). New Zealand Petroleum Conference Proceedings 2008. Wellington, Ministry of Economic Development. 17 p.
- Turnbull IM, Uruski CI, Anderson HJ, Lindqvist JK, Scott GH, Morgans HEG et al. 1993. Cretaceous and Cenozoic sedimentary basins of western Southland, South Island, New Zealand. Institute of Geological & Nuclear Sciences Monograph 1. Lower Hutt, Institute of Geological & Nuclear Sciences. 86 p.
- Uruski CI, Stagpoole VM, Isaac MJ, King PR, Maslen G 2002. Seismic interpretation report - Astrolabe survey Taranaki Basin, New Zealand. New Zealand Unpublished Openfile Petroleum Report PR3072. 192 p + 29 enclosures.
- Uruski CI, Browne GH, Stagpoole VM, Herzer RH, Bland KJ, Griffin AG et al. 2008. Petroleum prospectivity of the Northland Basin, New Zealand. New Zealand Unpublished Openfile Petroleum Report PR3933. 89 p.
- Voggenreiter WR 1993. Structure and evolution of the Kapuni Anticline, Taranaki Basin, New Zealand: evidence from Kapuni 3D seismic survey. New Zealand Journal of Geology and Geophysics 36: 77–94. 10.1080/00288306.1993.9514556
- Walcott RI 1978. Present tectonics and Late Cenozoic evolution of New Zealand. Geophysical Journal of the Royal Astronomical Society 52: 137–164. 10.1111/j.1365-246X.1978.tb04225.x
- Wood RA, Stagpoole VM 2007. Validation of tectonic reconstructions by crustal volume balance: New Zealand through the Cenozoic. Geological Society of America Bulletin 119: 933–943. 10.1130/B26018.1