Abstract
Oligocene and Miocene strata of the Nile Group are dominated by neritic cool-water carbonates formed within the Paparoa Trough along the western flank of the Challenger Rift System. In outcrop west of the Paparoa Range, the Nile Group contains three formations, the Waitakere Limestone, dominated by photozoan floatstone and grainstone facies, the Tiropahi Limestone, containing both impure heterozoan packstones and wackestones, and the Potikohua Limestone, consisting entirely of skeletal heterozoan grainstones. These correlative formations represent lateral depositional facies interpreted to record a submarine depression bounded by a northern irregular palaeohigh and a southern open marine plateau. It is proposed that these variations are the product of reactivation of structures associated with the underlying Paparoa Core Complex superimposed on the NE–SW rift axis emphasized within current basin models. This interpretation is consistent with exposures elsewhere in the West Coast region and highlights the need for palaeogeographic models of South Island to take older, rift-related basement structures into account.
Introduction
The West Coast region of New Zealand has experienced multiple generations of rifting prior to and since the inception of Zealandia as a distinct landmass in the Late Cretaceous (Nathan et al. Citation1986). These rift events locally overprinted one another, forming faults at different orientations, each stage of rifting increasing the complexity of the basin structure and in doing so obscuring the relationship between syn-sedimentary faulting, basin subsidence and geometry, and the overlying sedimentary record. Despite the West Coast's complex history, cross-sections and palaeogeographic models of the region focus on a NNE–SSW-trending extensional fault set initially developed as normal faults in the Late Cretaceous and Paleogene (Nathan Citation1975, Citation1978a,Citationb; Laird Citation1988; Rattenbury et al. Citation1998; Nathan et al. Citation2002) and episodically active until the latest Oligocene when the structures reactivated as reverse and transpressional faults (Furlong & Kamp Citation2013). While the younger Neogene fault set was the dominant control on Cenozoic basin geometry, detailed analysis suggests significant local reactivation of older structures has contributed to sedimentation patterns and basin geometry.
This study examines the Paleogene strata of the West Coast region in order to evaluate the influence of mid-Cretaceous core complex-related faults on local and regional Cenozoic sedimentation and palaeogeographic development. Oligocene sedimentology and stratigraphy receive especially close attention, as their predominantly carbonate lithologies record the culmination of rift-related early Cenozoic sedimentation and have the greatest potential for demonstrating the influence of basement topography and reactivation of pre-existing faults. Reassessment of the palaeogeography within this more complex framework may also shed light on the existence and proximity of land area during the Late Oligocene, a subject of recent debate with both geological and biological implications (Cooper & Cooper Citation1995; King Citation2000).
Regional setting
The New Zealand continent originated as part of the east coast of Gondwana, rifting away throughout the Cretaceous (c. 125–100–83 Ma; Laird & Bradshaw Citation2004). The initial stage of rifting is recorded by a number of igneous intrusions and localized volcanism (Muir et al. Citation1997) within an accretionary wedge of Ordovician metasediments (Greenland Group; Tulloch & Kimbrough Citation1989) and Devonian granitoids which comprise the Buller Terrane (Mortimer Citation2004). This extension and subsequent thinning of continental crust was accommodated by several metamorphic core complexes (e.g. Sisters Shear Zone in Stewart Island, Kula et al. Citation2009; western Fiordland orthogneiss and Tuhua Sequence, Gibson et al. Citation1988; Otago examples, Forster & Lister Citation2003; Paparoa Core Complex, Tulloch & Kimbrough Citation1989). Ongoing extension was accommodated along a spreading centre associated with the opening and growth of the Tasman Sea (Bishop Citation1992). In the West Coast region, the resulting assemblage of Paleozoic and Mesozoic basement rocks consists of an amalgamation of Ordovician metasediments (Greenland Group; Laird Citation1971), Devonian and Cretaceous intrusive plutons (Karamea Batholith, Nathan et al. Citation2002), and a suite of foliated granites, gneisses and mylonites (Kimbrough & Tulloch Citation1989; Nathan et al. Citation2002; ) forming the metamorphic core of the Paparoa Core Complex (Tulloch & Kimbrough Citation1989).
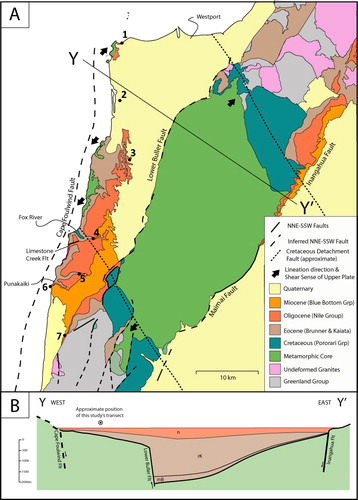
The basement rocks of the West Coast between Westport and Punakaiki are composed of the southern half of the Paparoa Metamorphic Core Complex (). The basement rocks of the northern half of this stretch of coastline (Westport to Fox River) are composed of the metamorphic core of the lower plate, and the basement rocks of the southern half (Fox River to Punakaiki) are composed of undeformed granite and metasediments of the upper plate. These lithologically distinct blocks are divided by a low-angle (10–20°), south-dipping detachment fault. The boundary between the lower and upper plate is also marked by sedimentary breccia of the Pororari Group (Laird Citation1995; Tulloch & Kimbrough Citation1989) which were deposited within fault-parallel half-graben basins proximal to the detachment fault and on top of the upper plate.
A second rift phase began in the Early Eocene, forming an orthogonal fault set trending NNE–SSW. Laird (Citation1994) proposed that the orthogonal relationship between these two fault sets reflects the younger phase of rifting utilizing transform faults associated with the previous rift event. This period of extension formed the Challenger Rift System, an extensional lineament running the entire length of the New Zealand continent (Kamp Citation1986; Furlong & Kamp Citation2013). In the West Coast region, this second, predominantly Paleogene stage of rifting is recorded by the formation and sedimentary infill of the Paparoa Trough (Nathan et al. Citation1986), a NNE–SSW elongate and fault-controlled basin on the western shoulder of the Challenger Rift System (). This trough-like feature extended from Greymouth to Westport, with the trough axis and greatest palaeodepth (upper-bathyal, 200–600 m) corresponding with the NNE–SSW-trending Paparoa Range crest that exists today and cross-cutting the Paparoa Core Complex and associated WNW–ESE-trending faults (Nathan et al. Citation2002).
In the West Coast region, rifting persisted into the Late Oligocene, shifting to convergent tectonics in the latest Oligocene (Furlong & Kamp Citation2013). This switch to a compressional regime prompted basin inversion throughout the Paparoa Trough, resulting in the uplift and erosion of the majority of the sedimentary rocks from the deepest part of the trough. The preserved Oligocene strata are relatively thin packages of non-marine, shelf and platform facies that would have formed along the flanks of the axis of the Paparoa Trough.
Methods
Six stratigraphic columns were compiled from measurements and observations of Oligocene strata from Westport in the north to near Punakaiki in the south and combined with drill hole data at Okari Lagoon (Okari-1, Okari-2; Nathan Citation1986). Columns were used to construct a NNE–SSW cross-section running parallel to and c. 10 km west of the axis of the Paparoa Trough (). Stratigraphic columns all lie between the same prominent NNE–SSW, basin-bounding faults (Cape Foulwind and Lower Buller faults) and emphasize palaeobathymetric variations parallel to the trough axis as opposed to variation across the axis of the trough.
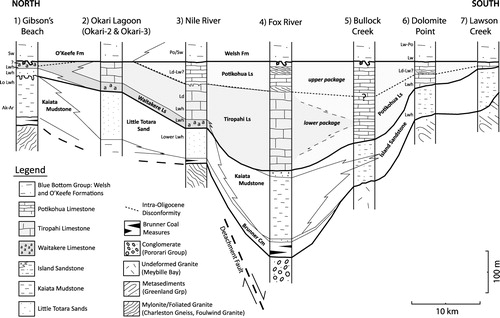
Cenozoic sedimentology and stratigraphy
Paleogene clastic and carbonate lithologies crop out along the western flank of the Paparoa Range, in river-cut cliffs and coastal headlands. Exposures consist of 75–450 m of unconformity-bound Eocene and Oligocene successions (i.e. Brunner Coal Measures, Rapahoe Group, Nile Group, Nathan Citation1974; Nathan et al. Citation2002), overlying a variety of basement lithologies (igneous, metamorphic, metasedimentary, sedimentary) and capped by Miocene siltstones and sandstones of the Blue Bottom Group.
This study presents greater detail for the Oligocene strata of the Nile Group (Nathan Citation1974). The study also focuses on the final stage of Cenozoic rifting and the record of the subsequent maximum transgression and culmination of the Paleogene megasequence, which are the subject of many questions regarding the palaeogeography of New Zealand. The dominance of carbonate deposition and absence of significant terrigenous supply that characterizes the Oligocene record allows basin depth to be determined using the distribution of photozoan and heterozoan biotas which are sensitive to subtle changes in basin topography and water depth.
Along the western Paparoa Range, the Nile Group consists of two packages of limestone divided by a disconformity. The lower package, containing the lower Waitakere Limestone and Tiropahi Limestone, is finer grained, locally carbonate-poor (45–90%), bioturbated packstone, wackestone, floatstone and grainstone with poorly preserved or no sedimentary structures. The upper package contains the Potikohua Limestone, a flaggy-bedded, carbonate-rich (85–97% CaCO3), and locally cross-bedded bryozoan and echinoid-rich skeletal grainstone, as well as the very uppermost Waitakere Limestone. The following sections present descriptions and interpretations of seven stratigraphic columns along a transect through the field area () and compiled into a north to south cross section along the western edge of the Paparoa Trough ().
Gibson Beach
Description
The Gibson Beach section contains around 85–95 m of Eocene strata, beginning with a thin 5–15 m layer of poorly exposed, poorly sorted, coarse quartz sandstone of the Brunner Coal Measures overlying intensely weathered, foliated biotite granite of the Karamea Suite (Foulwind Granite). These sandstones are themselves overlain by 80–100 m of brown, increasingly calcareous mudstone and algal limestone of the Kaiata Formation (Rapahoe Group; Nathan Citation1974, Citation1975). The top of the Kaiata Formation is marked by a planar disconformity surface followed by c. 15 m of trough cross-bedded, medium-grained, micaceous, feldspathic quartz sandstone of the Little Totara Sand (Lever Citation2001, Citation2007). The Little Totara Sand at Gibson Beach is Oligocene, not Eocene in age, and the upper contact between Little Totara Sand and Nile Group is concealed by slumped unconsolidated Quaternary sediments.
The Nile Group at Gibson Beach consists of c. 10 m of the Waitakere Limestone. Metre-scale (60–90 cm), moderately to well-cemented, and laterally continuous beds of bioturbated algal floatstone form the base of the section. Algal grains are moderately rounded, poorly sorted, fine to very coarse sand. Extensive, multigenerational burrow systems (Thalassinoides, Planolites) are ubiquitous, resulting in common to complete bioturbation with increasing intensity in the upper half of individual beds and a nodular appearance in outcrop. Sedimentary structures are conspicuously absent. Indurated floatstones are composed of non-geniculate, branching coralline algae (35%) and small (5–15%) and large benthic foraminifera (Amphistegina sp.) (5–10%). Bivalves (Ostrea sp., Lentipecten sp.), gastropods, regular and irregular echinoids, and bryozoans (robust branching) are present as macrofossils, but are rare in the sand-size fraction. The regular echinoid and bryozoan macrofossils are notably intact and well preserved. Allogenic minerals include abundant fine to very coarse quartz sand (25%), and rare biotite and muscovite (< 10%). Allochems are supported in a bioclastic, quartz-rich (c. 10%) packstone to grainstone, and locally, algal floatstone that grades into especially quartz-rich (c. 40%), less-indurated lithologies.
There is a sharp change to cross-bedded, algal-rich quartz sandstones and algal grainstones across a gently undulating unconformity. These carbonate-rich clastic lithologies comprise the upper 3 m of the Waitakere Limestone. Cross-bedded algal grainstones are compositionally similar to the lower algal facies, but texturally distinct. The facies is sub-planar and trough cross-bedded (length = 1–3 m, height = 15–40 cm, palaeoflow of c. 350°) and forms laterally discontinuous N–S-trending lenses. Grains are medium to coarse, well-sorted sand, with intense abrasion and fragmentation. Small vertical burrows cross-cut master bedding planes. Beds are moderately cemented. The grainstone contains coralline algae (20–70%), quartz (25–38%) and echinoid fragments (< 10%), and less common contributions (< 5%) from bryozoans, gastropods, large benthic foraminifera (Amphistegina sp., Asterigerina sp.) and small benthic foraminifera. Muscovite and biotite are rare, forming no more than 5% of the sediment, and authigenic mineralization is negligible. The top of the Nile Group is unconformably overlain by non-calcareous, muddy, fine-very fine sandstone of the O'Keefe Formation (Middle Miocene, Blue Bottom Group) along a burrowed firmground surface. The scant amount of the upper Waitakere Limestone may be the result of Early Miocene erosion, however, no carbonate lithoclasts or planation was observed at this locality.
Interpretation
The coralline algae in these facies are indicative of an illuminated seafloor and the algae are most commonly found in wave-protected, shallow settings along temperate coasts with moderate trophic resources (Scoffin Citation1988; Freiwald Citation1993; Henrich et al. Citation1995; Betzler et al. Citation1997) well within the euphotic zone. A closer look at the branching morphology of the majority of algal components suggests that production persisted at greater depths, higher sedimentation rates, and quieter conditions than ideal for rhodolith growth (Nebelsick et al. Citation2001; Rasser & Nebelsick Citation2003). The absence of binding and encrusting algae indicate that organisms are derived from adjacent algal banks more suitable for carbonate production. Symbiont-bearing, large benthic foraminifera support this interpretation of an illuminated seafloor (< 30 m), and suggest that minimum temperatures were above 15 °C (Betzler et al. Citation1997). The presence of shallowly burrowing echinoids and ubiquitous deposit feeders in the lower half of the Waitakere Limestone is indicative of primarily unconsolidated sediment rarely reworked by waves and currents (Kanazawa Citation1992). The abundance of well-preserved delicate fossils, absence of preserved physical structures and appreciable mud content are also consistent with a protected environment and weak hydrodynamics.
A significant change in oceanography is indicated by the shift from biological to physical sedimentary structures into the upper Waitakere Limestone. Thin planar cross-bedding, increased sorting and fragmentation within the grainstone and sandstone lithologies suggest that the upper facies was regularly reworked by moderate wave-generated and/or tidal currents in a shallow marine setting. The presence, albeit rare, of exclusively robust bivalves and abundance of detrital quartz along with abundant coralline algae is consistent with an inner shelf depositional environment.
Okari Lagoon
Description
Data for this stratigraphic column are taken from the Okari-2 and Okari-3 drill hole summary of Nathan et al (Citation1986, stratigraphic column K29/c3) and have not been directly examined by the authors. No basement rock was reported from either drill hole. The section begins in the Rapahoe Group with a 150 m thick succession of micaceous quartzofeldspathic Little Totara Sand conformably overlying an unknown thickness of sandy calcareous mudstone of the uppermost Kaiata Formation (Point Elizabeth Member; Nathan et al. Citation1986).
Only summary details on the Nile Group are provided in the core description (Nathan et al. Citation1986). The basal 20 m consists of hard white algal grainstone, floatstone or rudstone interbedded with soft quartz sand (Waitakere Limestone). The upper 50 m consist of Tiropahi Limestone, however, the contact between the two units was not described. The base of the Tiropahi Limestone is a dark brown, calcareous sandstone which grades upwards into hard, brown to grey packstone or wackestone, ending in a burrowed firmground surface unconformably overlain by non-calcareous, muddy, very fine sandstone of the O'Keefe Formation (Middle Miocene, Blue Bottom Group; Nathan et al. Citation1986).
Interpretation
The lack of available detailed data for this section limits facies interpretations. The presence of algal- and quartz-rich Waitakere Limestone is consistent with that of neighbouring sections at Gibson Beach described above. Algal-rich facies record shallow-water deposition on or near an illuminated seafloor, and the quartz sand, depending on grain size, could support a shallow marine interpretation.
Conversely, the muddy texture of the Tiropahi Limestone indicates deeper and/or quieter water, mid-shelf environments. The calcareous sandstone at the base of the Tiropahi Limestone indicates a temporary source of clastic sediments, perhaps related to a fall in sea level and exposure of a nearby palaeohigh. However, these shallower conditions were short lived, as the absence of physical structures reported in the Tiropahi suggests deposition was consistently below fair weather wave base. Also, the absence of algal material in the Tiropahi suggests a seafloor below the photic zone and may reflect a relative rise in sea level. Taken together, these two limestones are interpreted as a record of an overall deepening upwards trend from a shallow illuminated algal carbonate factory and proximal clastic source to a protected embayment at mid-shelf depths.
Nile River
Description
The section begins with 70 m of Eocene strata lying unconformably upon garnet biotite gneiss of the Charleston Metamorphic Group. The base contains 10 m of interbedded white quartz sandstone and coal seams of the Brunner Coal Measures overlain by 60 m of orange, cross-bedded quartzofeldspathic Little Totara Sand (Rapahoe Group; Nathan et al. Citation1986, stratigraphic column K30/c1). The contact with the overlying Nile Group was not observed.
The Nile River is the type section for the Nile Group and contains exposures of all three formations present along the western Paparoa Range, the Waitakere, Tiropahi and Potikohua limestones. The Waitakere Limestone exposed along the lower Nile River consists of 20 m of thin, massive beds of algal grainstone and floatstone. Large and small benthic foraminifera make up over 10% of the rock by volume. However, quartz and micas are rare (< 5%). The upper 5 m fine upwards from grainstone into impure coralline algal packstone with a notable increase in angular to sub-rounded, very fine sand-sized quartz (20%), mica (5%), glauconite (3%) and phosphate (3%), along with carbonate mud (c. 10%) within the dominantly algal limestone (50%).
Five to ten metres of a soft and silty quartz-rich skeletal packstone informally known as the Madman's siltstone sits atop the Waitakere Limestone and marks the base of the Tiropahi Limestone (Nathan Citation1975). The member is only present where the Tiropahi Limestone overlies the Waitakere Limestone. The Tiropahi Limestone forms prominent 30–50 m cliffs bounding the river. The formation, totalling 80 m, is composed of massive, micaceous skeletal packstone. Beds are 60–200 cm thick, moderately cemented, and laterally continuous at outcrop with gradational bioturbated bed boundaries. Sub-horizontal Planolites burrows are abundant and Scolicia burrows are present but rare. Grains are sub-angular, moderately sorted, clay to fine sand (> 30% fines), reflecting mild abrasion and fragmentation during transport and deposition.
The packstone is composed of echinoid fragments (20%), quartz grains (10–20%) and benthic foraminifera (5–15%). Locally, molluscs (calcitic), bryozoans and coralline algae form 5–10% of skeletal particles. Interstitial material is composed of bioclastic micrite, which makes up 25–45% of the rock volume. In addition, muscovite, biotite and feldspars are minor but consistent components (c. 5%). Leaf fossils and rare carbonaceous material were observed in float but not seen in situ.
Overlying the Tiropahi Limestone is c. 45 m of well-cemented, flaggy Potikohua Limestone. The two formations are divided by a planar disconformity marked by a horizon of Thalassinoides burrows filled with glauconite-rich skeletal grainstone. Potikohua Limestone flags are defined by well-developed dissolution seams and are 10–25 cm thick, laterally discontinuous, pinching out over metres to tens of metres. Cross-stratification was not observed in this locality. Texturally, clastic constituents are moderately sorted, rounded to sub-rounded, fine to very coarse (medium to coarse on average) sand. Bryozoans dominate skeletal components (c. 40%), with lesser contributions from irregular echinoids (10–20%). Bivalves, barnacles, coralline algae, and small and large benthic foraminifera form small but consistent contributions (< 10%). Quartz, plagioclase, and oxides are notably rare in this facies, comprising < 10%. These rocks are characteristically well-indurated, with sparry, blocky and epitaxial cements filling inter- and intraparticle pore spaces and making up 20–35% of the rock by volume. The Nile Group is capped by a burrowed firmground overlain by calcareous, muddy fine sandstone of the Welsh Formation (Early Miocene, Blue Bottom Group).
Interpretation
The abundance of light-dependent coralline algae and large benthic foraminifers and the absence of carbonate mud in the Waitakere Limestone at Nile River are interpreted to reflect deposition on or near to an illuminated seafloor winnowed by currents. The overlying muddy, finer-grained and impure algal packstone and Madman's siltstone are interpreted as a deepening upwards trend with diminishing wave and current influence. This is supported by the decrease and loss of algal components. Furthermore, the relative abundance of fine and very-fine sand-sized quartz and micas, which comprise the bulk of the Madman's siltstone, also support a shut off of algal carbonate factories and accumulation of siliciclastics. Taken together, these basal lithologies record uninterrupted sedimentation during a transgression culminating in the transition between the Waitakere Limestone and Tiropahi Limestone. Gradational transitions such as these are typical of deeper water environments and/or those with greater and more persistent accommodation space.
The absence of physical structures and the abundance of biological structures in the Tiropahi Limestone are indicative of low-energy conditions and oxygenated seafloor reminiscent of mid- to outer shelf environments. The minor contribution and small grain size of clastic material (i.e. micas and quartz silt), suggest that deposition was below the fair weather wave base. This interpretation of mid-outer shelf depths is also supported by the absence of algal material and minor contribution from other light-dependent biota (e.g. large benthic foraminifers). These characteristics, together with the impoverished assemblage of infaunal heterozoan elements and minor plant debris, are indicative of accumulation at moderate depths in low–moderate energy, mesotrophic conditions with limited terrestrial sediment sources. The disconformity at the top of the Tiropahi resembles a marine firmground where a hiatus in sedimentation prompts minor seafloor cementation and open burrow systems that are subsequently filled by mobile sediments.
The Potikohua Limestone lacks appreciable detrital components and any evidence of bioturbation, and is instead composed of relatively well-sorted coarse-grained grainstones. The biota of the Potikohua Limestone are reminiscent of mid-shelf environments, but from those within more open marine, energetic conditions. Bryozoan-dominated, locally cross-bedded lithologies are likely deposited at 40–60 m water depth, below the photic zone, but still regularly reworked by wave and tidal currents. Small (1–2%) contributions of large benthic foraminifers are interpreted as allochthonous, derived from a nearby inner shelf environments. Alternatively, large benthic foraminifera could have formed in situ during periodic seafloor illumination.
Fox River
Description
The Fox River area contains the thickest Eocene–Oligocene record in the study area, exceeding 400 m. The mouth of the Fox River, the base of the section, is marked by a small headland of Cretaceous non-marine breccias of the Pororari Group. Twenty-five metres of interbedded quartz sandstone and coal seams of the Brunner Coal Measures sit atop these breccia along an angular unconformity followed by 10 m of cross-bedded Little Totara Sand and 160 m of silty calcareous Kaiata Formation (Lever Citation2001; Wellman et al. Citation1981, stratigraphic column S37/c2). The top of this section is best exposed at Seal Island and consists of 10 m of Island Sandstone with a metre of burrowed algal floatstone, the top of which forms a planar, burrowed disconformity with the overlying Nile Group (Lever Citation2007).
The Nile Group in the Fox River section is comprised of a 190 m package of skeletal packstone and grainstone (Tiropahi Limestone) overlain by a 45 m package of Potikohua Limestone. Sheer cliffs make logging of continuous section difficult, although broad changes are observable. The basal 20 m of the Tiropahi Limestone is composed of quartz-rich echinoid grainstone and packstone with < 10% carbonate mud and a unique mix of both cm-scale bedding and distinct sub-vertical Ophiomorpha burrows, horizons of rhodoliths (10–20%) and glauconite (10–15%). The middle reaches of the Tiropahi Limestone are a mix of packstones and wackestones and contain additional planktic foraminifera (5–10%) and carbonate mud (10–20%). The upper 50 m of Tiropahi Limestone consists of bioturbated, micaceous and glauconitic fine sand-sized skeletal packstone and grainstone. The uppermost bounding surface was only observed from a distance.
The overlying Potikohua Limestone, cropping out at the junction between Dilemma Creek and the Fox River, is composed of cross- and flaggy-bedded skeletal grainstone. The base of the section is composed of 10 m of trough cross-bedded (50 cm high by 1–4 m long) quartzose echinoid grainstone. The middle 15 m section exposed along the Fox River contains thin flaggy beds with a higher percentage of carbonate (> 90%), and a more diverse faunal assemblage including abundant branching bryozoans, irregular echinoids, small benthic foraminifers and calcitic molluscs. Large benthic foraminifers and coralline algae, however, remain conspicuously absent. The upper Potikohua Limestone contains prominent medium- and large-scale tabular cross-beds (length = 5–20 m, height = 2–4 m) and a similar composition to the flaggy mid-Potikohua Limestone directly below. Palaeoflow directions measured on small (< 1 m) cross-beds range from 020° to 070°. Larger structures (e.g. bedforms > 1 m in height) were only observed in cliff faces, not directly measured with a compass, and thus provide only a general palaeoflow direction (e.g. southwest). The uppermost Nile Group consists of a few final metres of 20–30 cm thick flaggy bedded quartzose echinoid grainstone capped by a burrowed firmground surface. The surface is unconformably overlain by gritty and glauconitic quartzose sandstones of the Early Miocene Welsh Formation (Blue Bottom Group).
Interpretation
Ubiquitous bioturbation, abundant heterozoans, most notably irregular echinoids, and the presence, albeit small, of carbonate mud all point towards mid-shelf depths. The appreciable amount of glauconite in the basal Tiropahi Limestone indicates slow rates of sedimentation consistent with a mid-shelf depositional environment. Algal components in the basal Tiropahi Limestone hint at a shallower seafloor within the photic zone; however, their small contributions and round rhodolith morphology necessitate formation under persistent wave action and are inconsistent with the majority of other lithologic characteristics. Instead, these light-dependent biota are likely allochthonous, having been swept from inner-shelf carbonate factories. The increase in carbonate mud and the appearance of planktic foraminifera in the middle Tiropahi indicate deeper, more open marine conditions. Conversely, the coarser grain size and minimal carbonate mud in the upper Tiropahi suggest a return to shallower conditions with regular winnowing. Nevertheless, the persistent dominance of biological sedimentary structures precludes shallowing above fair weather wave base.
The absence of carbonate mud and abundance of medium- and large-scale trough and tabular cross-beds in the Potikohua Limestone are interpreted as a switch to current-controlled sedimentation. The abundance and size of the cross-bedding in the Potikohua Limestone reflect exposure to intense wave and current action at the early and later stages of deposition. The absence of light-dependent organisms suggests that the more intense water movement is a function of increased current strength rather than shallower depths. The switch from mobile irregular echinoids in cross-bedded lithologies to rooted and branching bryozoans more characteristic of the flaggy bedded lithologies of the middle Potikohua Limestone suggests a change from persistent and prolonged reworking of the seafloor by currents to episodic reworking of the seafloor (e.g. storm events). Likewise, the return to cross-bedded grainstones in the upper Potikohua suggests a return to prolonged reworking by unidirectional currents (Anastas et al. Citation2006).
Bullock Creek
Description
The Bullock Creek section begins with a 150 m thick package of Island Sandstone sitting unconformably on undeformed muscovite biotite granite (Meybille Bay Granite). Cross-bedded, gritty sandstone forms the basal few metres of the section and grades upwards into the remainder of the Rapahoe Group, which is composed of the Island Sandstone, here a brown, thickly bedded, intensely bioturbated, and muddy fine-grained quartz sandstone divided in two by a 40 m section of Kaiata Formation (Wellman et al. Citation1981, stratigraphic column S37/c1; Nathan et al. Citation1986, K30/c3; Lever Citation2001). The Nile Group overlies the Island Sandstone along an angular unconformity marked by a coarse condensed lag of phosphatized carbonate lithoclasts, macrofossils and rounded quartz pebbles.
The Potikohua Limestone is the sole Nile Group formation in the Bullock Creek section. The sheer cliffs make continuous logging of this section difficult and so descriptions are limited. The basal 35 m of the limestone is a massive quartz-rich echinoid grainstone with additional, albeit minor, contributions from bryozoans, small benthic foraminifera and coralline algae (< 5%). These impure grainstones rapidly grade into c. 90 m of thin, flaggy-bedded, moderately sorted, bryozoan grainstone that persists throughout the middle of the section. Echinoids (10–20%), small benthic foraminifera (c. 5%) and quartz (c. 5%) also contribute to these grainstones, however, bryozoans consistently comprise the bulk of components (> 40%). Rare centimetre-scale cross-beds are present within flags, however, large-scale cross-bedding was not observed. The top 45 m of the Potikohua Limestone at Bullock Creek is composed of fine-grained micaceous packstone and grainstone compositionally similar to the middle section but containing additional carbonate mud and muscovite. The top of the Nile Group is marked by a burrowed firmground surface unconformably overlain by muddy, fine-grained sandstone of the Early Miocene Welsh Formation (Blue Bottom Group).
Interpretation
The presence of a lag at the base of the Nile Group is interpreted as a prolonged hiatus in sedimentation. The carbonate composition and pebble-size of litho- and bioclasts suggest this hiatus was a function of marine erosion and winnowing. The overlying quartz- and echinoid-rich lithologies grading upwards into increasing carbonate-rich grainstones are interpreted as the gradual increase in carbonate sedimentation rates and replacement of terrestrial clastic sources by proximal and in situ carbonate production (i.e. echinoids, bryozoans).
The dominance of bryozoans and other heterozoan biota within the bulk of the Potikohua Limestone is interpreted as a record of deposition at open marine, mid-shelf depths distal to any landmass or other clastic source. The presence of small cross-beds, the lack of macrofossils and moderately sorted sand-sized texture are consistent with this interpretation. These features also suggest that despite the seafloor being below the photic zone and fair weather wave-base, clasts were periodically reworked by currents and storms. The presence of carbonate mud and micas within the uppermost Potikohua Limestone at Bullock Creek is interpreted as a decrease in energy either from a relative rise in sea level or diminished current and wave activity unrelated to a change in sea level.
Dolomite Point
Description
The Dolomite Point section begins with a meagre and discontinuous few metres of quartz sandstone and coal seam of the Brunner Coal Measures and Little Totara Sand (Rapahoe Group) overlying greywacke basement of the Greenland Group (Wellman et al. Citation1981, stratigraphic column S37/c3). The remainder of the Rapahoe Group consists of 70 m of massive, calcareous, and argillaceous fine-grained quartzose sandstones of the Island Sandstone. The uppermost Island Sandstone and contact with the Nile Group were not observed in this locality.
The Nile Group at Dolomite Point is composed of 55 m of Potikohua Limestone containing a lower and upper member divided by an intraformational disconformity. The lower member is composed of 25 m of thick- to thin-bedded, massive to laminated, quartz-rich echinoid grainstone. These grainstones are dominated by fragments of irregular echinoids, as well as a significant clastic component, with 10–20% quartz and 5% micas and plagioclase. Glauconite is also present, but does not exceed a few per cent. Additional carbonate components include bryozoans, benthic foraminifera and scattered coralline algae. A prominent and planar hardground surface covered by a thin lag of pebble-size phosphatized carbonate lithoclasts marks the top of the lower limestone member. Lithoclasts within the lag have a similar composition to underlying lithologies, albeit with the addition of authigenic minerals.
The upper member consists of 30 m of thin, flaggy-bedded bryozoan grainstone with additional echinoids fragments (10–20%), small benthic foraminifera (5–10%), and quartz, bivalves and large benthic foraminifera (< 5%). The base of the upper grainstone facies contains 30–50 cm thick cross-beds trending north and south, however, as some aspects of exposure within Dolomite Point are not easily accessible on foot, precise palaeoflow measurements were not obtained. The basal few metres of the upper section also contains c. 10% large benthic foraminifera. Smaller 10–20 cm high cross-beds occur locally higher in the section. The uppermost Nile Group is capped by a burrowed firmground surface unconformably overlain by muddy fine-grained sandstone of the Early Miocene Welsh Formation (Blue Bottom Group).
Interpretation
The abundance of bioturbation and scarcity of physical sedimentary structures within the majority of the lower member of the Nile Group are interpreted to reflect mid-shelf deposition below both fair weather wave base and the photic zone. The abundance of quartz and other clastic minerals in these grainstones indicates a proximal source for clastic material. The dominance of infaunal life modes (e.g. irregular echinoids) also supports a good supply of nutrients into this quiet water system.
The transition between the lower quartz-rich echinoid and upper bryozoan grainstone, defined by the planar hardground and phosphatized lithoclasts, is interpreted as the product of wave planation and nearby marine erosion forming an iron-stained hardground surface. This intraformational disconformity is interpreted as a sequence boundary formed through a relative fall in sea level to relatively shallow, inner shelf conditions.
The bryozoan grainstone itself records a transition to increasingly open marine conditions and intensified current-controlled conditions. The cross-bedded base of the upper section is interpreted as transgressive deposits influenced by wave or tidal currents, which is consistent with the large benthic foraminifera within it reflecting shallow water. Whereas the overlying thin flaggy bedded grainstones suggest less-persistent reworking by currents below or near fair weather wave base. The lack of detrital clastic material and dominance of epifaunal life modes resembles a heterozoan, specifically bryozoan carbonate factory on a marine plateau.
The thin, coarse-grained and locally cross-bedded character of this section records two periods of sedimentation in an increasingly wave- and/or current-dominated environment. The absence of light-dependent organisms and diminishing clastic contributions, however, suggest the seafloor remained below the photic zone and unperturbed by terrestrially derived sediments. To reconcile these differences, we propose a marine plateau around mid-shelf depths allowing for a seafloor at or near fair weather wave base, but not atop an illuminated palaeohigh or close to a palaeoshoreline due to the absence of algal and clastic material.
Lawson Creek
Description
The thinnest of all the sections, Lawson Creek, contains 40 m of Island Sandstone (Rapahoe Group) sitting unconformably on Ordovician metasediments of the Greenland Group. Outcrop of the Nile Group is exposed solely in creek beds and is a composite from both Lawson and Hibernia Creeks. The Nile Group here is composed of c. 20 m of Potikohua Limestone containing a lower and upper member divided by an intraformational disconformity. The lower member is an estimated 10 m of bioturbated fine to medium sand-sized skeletal packstone to grainstone composed of echinoids (25–35%), bryozoans (15–20%), small and large benthic foraminifera (5–10%), carbonate mud (5–10%), moderately rounded, fine sand size quartz (c. 5%), and minor mica and glauconite (< 5%). The remaining fraction of grains is undifferentiated skeletal particles. The lower package is capped by a burrowed firmground surface. The 10 m of Potikohua Limestone overlying this intraformational disconformity is composed of medium to coarse sand-sized flaggy-bedded skeletal grainstone. The basal 20 cm contain c. 15% glauconite and grade rapidly into glauconite poor (1–2%) skeletal grainstones composed of bryozoans (35–60%), echinoids (25–40%), small and large benthic foraminifera (5–10%), bivalves (5%), and minor quartz and muscovite (5%). The Nile Group is overlain by the Welsh Formation (Blue Bottom Group), however, this contact was not observed in the creek bed.
Interpretation
The incomplete exposure within these creeks limits the reliability of interpretation. Nevertheless, the dominantly heterozoan composition and bioturbated texture of the packstone and grainstone indicate that the environment was below the photic zone and protected from regular reworking by waves. Conversely, the limited amount of carbonate mud suggests some winnowing was taking place. The additional clastic material in the packstone reflects a minor clastic source. The fine grain size of the quartz and mica is reminiscent of mid-shelf deposits. To reconcile these characteristics, the environment was likely near fair weather wave base, but distal from any significant source of clastic material.
The burrowed firmground that caps the lower limestone is interpreted as a hiatus in sedimentation. This interpretation is supported by the abundance of glauconite filling and overlying the surface. The coarser grain size and higher carbonate content in the upper limestone records more energetic hydrodynamic conditions, regular and prolonged reworking of the seafloor, and a productive heterozoan carbonate factory, similar to that of an open marine plateau with limited clastic supply.
Oligocene palaeoenvironments and palaeogeography
From the descriptions above, we can see that the Waitakere, Tiropahi and Potikohua limestones represent facies variation within the Nile Group. This variation is recorded entirely on the flank of the Paparoa Trough along a transect running parallel to and west of the axis of the basin, suggesting the trough margin is more complex than a single NNE-trending fault. This margin was likely quite complex, but of particular interest and utility is the recognition of a submarine depression centred around the Fox River and bounded by two relative palaeohighs, one centred in the north around Westport and the other in the south around Punakaiki.
These three regions roughly correspond with the three formations and broad facies in the region. The algal-rich Waitakere Limestone is the product of light-dependent carbonate factories and is a record of the aprons of carbonate and clastic sediments shed off these palaeohighs. The muddy, micaceous and bioturbated Tiropahi Limestone records deposition within variably protected submarine lows, from those proximal to the aforementioned palaeohighs to more distal, regularly winnowed and reworked seafloor beyond the protection of these highs. Lastly, the clastic-poor and relatively well-sorted and coarse-grained Potikohua Limestone records production and accumulation of carbonate sediments along an open marine shelf and plateau.
Because of the clear relationship between each formation and a single broad environment, the distribution of each formation can be used to reconstruct the palaeogeography of the region. Transformation of the region took place gradually, although the record contained within the Nile Group, divided broadly into an upper and lower package, allows the creation of two palaeogeographic maps roughly corresponding to the Early and Late Oligocene (). These maps illustrate trends contained within the cross-section presented in , pertaining to the lateral distribution of individual formations and thickness variation within the entire Nile Group. The following sections present these data and interpretations of the geometry and evolution of Oligocene palaeogeography in the West Coast region. Early Oligocene (Whaingaroan to Duntroonian) palaeogeography is based on the lower package of the Nile Group and Late Oligocene (Duntroonian to Waitakian) palaeogeography is based on the upper package of the Nile Group.
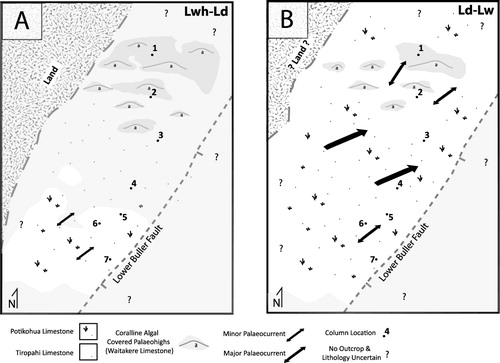
Early Oligocene (Whaingaroan to Duntroonian) palaeogeography
The algal-rich Waitakere Limestone forms the basal formation of the Nile Group from Gibson Beach to the Nile River but is not present at or farther south of the Fox River. Algal components are present in the basal 10 m of the Tiropahi Limestone at the Fox River, but these are only a minor component forming a single thin, discontinuous bed and rarely more than 5% of the rock by volume. From this, we conclude that Oligocene algal facies are essentially confined to the base of the Nile Group north of the Fox River. The limited distribution of these light-dependent facies suggests that the area north of the Fox River formed a regional palaeohigh ().
The Tiropahi Limestone is limited to the northern and central study area, pinching out beyond Okari Lagoon and being replaced by the Potikohua Limestone south of the Fox River. The Tiropahi Limestone records deposition within variably protected submarine lows at predominantly mid-shelf depths (50–100 m), with the deepest water facies and most open marine conditions (e.g. increase in sand-size fraction, planktic foraminifera and diminished clastic content) at the southern-most extent of the formation near the Fox River possibly extending deeper than 100 m. These facies trends are matched by the formation's overall thickness, which increases to the south and pinches out to the north. The northern limit of the Tiropahi Limestone is interpreted as a facies transition into the Waitakere Limestone proximal to the palaeohighs and the southern extent as a facies transition into the Potikohua Limestone and a slightly shallower, more regularly winnowed marine plateau.
The lower Potikohua Limestone, located south of the Fox River, is texturally a grainstone, but is uncharacteristically bioturbated, clastic-rich and forms rather inconsistent flaggy bedding. These Potikohua Limestone facies are the southern correlatives of the Tiropahi Limestone and are interpreted as a shallower, winnowed equivalent. The coarse, fragmented and well-sorted texture, appreciable quartz and mica, and dominantly heterozoan composition record deposition on an isolated marine plateau. This is supported by the absence of algal components and presence of intense bioturbation, and is interpreted as a disappearance of the palaeohighs and embayments that define the region to the north.
In addition to the distribution of these formations and environments, the overall thickness of the lower Nile Group contributes to our understanding of the palaeogeography during the Early Oligocene. The N–S cross-section () reveals gradual and persistent changes in the thickness of Nile Group strata with the maximum thickness occurring at Fox River. At Bullock Creek and Dolomite Point, the absence of the Waitakere Limestone and Tiropahi Limestone is compensated for by the increased thickness of the Potikohua Limestone. These lateral facies changes indicate that two distinct palaeohighs recorded in strata to the north and south are divided by a depression that persisted throughout the Early Oligocene.
Non-marine sediments were not directly observed in these formations, however, the presence of carbonaceous material and leaf fossils strongly suggests that an adjacent terrestrial environment existed in the Early Oligocene. The abundance of quartz and mica further supports the presence of a siliciclastic source, but is not necessarily terrestrially derived or in the immediate region. The presence of algal-rich carbonates indicates that shallow marine environments were isolated from significant clastic input suggesting a complex topography with localized exposure of isolated islands, and/or a distal terrestrial source from a landmass far to the west. This is consistent with the terrestrial, up-thrown side of the Cape Foulwind Fault proposed by Nathan et al (Citation1986). Carbonaceous and clastic material is increasingly rare or absent further south, making evidence for a western landmass increasingly less compelling south of the Fox River.
Late Oligocene (Duntroonian to Waitakian) palaeogeography
The Potikohua Limestone dominates the upper package of the Nile Group, extending across the majority of the region with the exception of the two northernmost sections (i.e. Gibson Beach and Okari Lagoon) (). These younger grainstone facies are characterized by persistent flaggy-bedding as well as common cross-bedding, which is most prominent at Fox River. The Tiropahi Limestone is replaced by the Potikohua Limestone and hints that the protection provided by embayments was diminished. However, the persistence of the Waitakere Limestone both above and below the internal unconformity within the Nile Group at Gibson Beach suggests that at least part of this northern region contained persistent palaeohighs and isolated, light-dependent carbonate factories throughout the Oligocene.
The spread of the Potikohua Limestone in the later Oligocene reflects the extension of the marine plateau across the region with some locations being the locus for especially intense currents (i.e. Fox River). It is important to note that this is not simply a record of shallowing. The Potikohua Limestone records both intense currents and an almost complete loss of photic biota. We propose that the transition was driven largely by the infilling of relief in the northern region by the Waitakere and Tiropahi limestone paving the way for more consistent sedimentation across the region. The burial and drowning of relative highs coinciding with regional subsidence allowed for oceanic currents to extend onto the previously protected shelf unimpeded.
Modern New Zealand heterozoan carbonates include mid-shelf-depth marine plateaus isolated from siliciclastic supply (e.g. Three Kings Plateau, Foveaux Straight; Nelson et al. Citation1982, Citation1988) and similar lithologies have been documented to the north in the Taranaki Basin (Te Kuiti Group; Nelson Citation1978). Interpretations of these Te Kuiti carbonates invoke a seaway model, whereby more intense oceanic currents flow through broad mid- and inner-shelf-depth channels on a marine plateau (Anastas et al. Citation2006). Within the Nile Group, the huge cross-beds in Fox River resemble a seaway system, with intense tidal or wave-generated currents recorded within large current-driven bedforms, whereas the flaggy-bedded bryozoan-rich Potikohua Limestone in the Nile River and Bullock Creek sections to the north and south record sedimentation along the adjacent marine plateaus exposed to less persistent sediment transport and accumulation.
The compositional consistency of the upper package of the Nile Group, composed almost entirely of the Potikohua Limestone, is matched by only minor thickness variation. The Potikohua Limestone is thickest in the centre of the study area near Fox River and Bullock Creek, thinning to both the north and south by over 50 m. These trends are interpreted to reflect two palaeohighs separated by a minor submarine depression.
Influence of Paparoa Core Complex on palaeogeography
Linear trends in the formation and environmental distribution and thickness described above illustrate an Oligocene palaeogeography inconsistent with simple platform or ramp geometries. Instead, Oligocene palaeogeography of this region appears to be shaped by two distinct palaeohighs divided by a relative low coincident with the Fox River. The lithologic and stratigraphic variations described in this study and illustrated in the N–S cross-section of are documented in the maps and associated bulletins for the Greymouth, Punakaiki and Cape Foulwind areas (Nathan Citation1975, Citation1978b; Laird Citation1988; Nathan et al. Citation2002). Previous unpublished studies by Laird (Citation1970, Haku-1 well) and Anderson (Citation1984) acknowledged these variations and invoke a complex shoreline along the palaeohigh west of the Cape Foulwind Fault to explain these stratigraphic complexities. The findings and illustrations of this study support these previous interpretations. We differ by proposing a structural framework to explain the complex Oligocene palaeotopography and bathymetry. We interpret the complexity of these shorelines as being affected by pre-existing structure related to the underlying Paparoa Core Complex ().
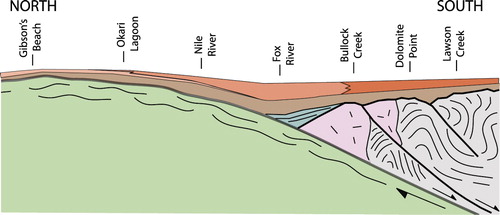
Cretaceous metamorphic core and Oligocene palaeohighs
The postulated palaeohighs and protected embayments inferred from the northern stratigraphic columns sit squarely atop the metamorphic core of the Paparoa Core Complex (). The spatial association between the most shallow-water facies, the most condensed sections, and underlying metamorphic basement suggests that the basement formed higher and more complex topography during the Oligocene relative to southerly unmetamorphosed basement lithologies (i.e. granite and metasediments; ). Specifically, this is indicated by the abundance of photic organisms overlying metamorphic basement and dominance of heterozoans further south.
The detachment fault
The amount of sedimentological and stratigraphic variation within Oligocene strata around the Fox River is striking. The Waitakere Limestone pinches out, the composition, texture, geometry and thickness of the Tiropahi Limestone changes dramatically, most notably by the appearance of planktic foraminifera, and the upper Potikohua Limestone contains unique large cross-bedded grainstones. The river mouth also coincides with where the southern detachment fault of the Paparoa Core Complex intersects the modern coastline. We propose that this geographic association is no accident and that changes in Oligocene facies and thickness here are related to that fault and the southerly transition from metamorphic basement to breccia of the Pororari Group. Essentially, the deepest environments and thickest succession in the Oligocene strata coincide with a mid-Cretaceous basin, which was initially partly filled by breccia of the Pororari Group.
There is evidence to suggest that the detachment fault, or a similar structure, was active in the Eocene. Lever (Citation2001) noted how the modern Limestone Creek Fault, a few kilometres south of the Fox River, divides a thick package of shoreface sandstone (basal Rapahoe Group) to the south from thin, discontinuous sandstone to the north (Laird Citation1988). The Oligocene section also thickens dramatically to the south of this structure. The location of this structure coincides with the appearance of the Pororari Group and roughly coincides with the southern detachment fault. The Limestone Creek Fault is mapped as trending ENE–WSW which is not consistent with the ESE–WNW orientation of the detachment fault, however, the limited exposure and meagre data indicating fault orientation do not preclude its being an expression and reactivation of an older, slightly more southeasterly trending structure (M.G. Laird, pers. comm. 2013). In fact, the orientation of the Limestone Creek Fault is far more comparable with structures related to the Paparoa Metamorphic Core Complex than those of the Challenger Rift System.
A remaining question is why the locus of Oligocene sedimentation coincides with a mid-Cretaceous depocentre. One explanation is that this area subsided more rapidly than adjacent areas, possibly due to reactivation and movement along high-angle normal faults associated with the detachment zone. This interpretation is consistent with the evidence of Eocene fault movement along E–W-trending structures presented above.
Undeformed basement and a marine plateau
Oligocene strata overlying the hanging wall to the south of the metamorphic core () are characterized by flaggy-bedded, skeletal grainstones, notably lacking in silt and mud of both carbonate and clastic composition. The paucity of detrital quartz and micas and light-dependent organisms suggests the basement formed a submerged moderate palaeohigh. Conversely, the coarser grain size and abundance of cross-bedding indicate that water depths were sufficiently shallow to allow currents to influence the seafloor. An isolated, shallow marine plateau is proposed to encompass these characteristics with quartz-rich lithologies at the base of the Potikohua reflecting exposure and erosion off of low-relief distal topography.
Southward thinning of Oligocene strata along the underlying undeformed basement (Fox River to Lawson Creek) hint at deposition along a gently northerly dipping palaeotopography. This inclined surface coincides with the top of the southern fault block and is consistent with the nature of listric faulting and fault block rotation in the hanging wall expected from extensional movement associated with the core complex. In fact, similar northerly rotation is recorded in the north-dipping bedding of the Pororari Group at Fox River. Relief along this surface may also be related to the variable composition of the hanging wall itself (granites and metasediments).
Eocene basin geometry
The Oligocene record broadly mirrors the facies transitions in the Eocene. Lever (Citation2001) described four sub-basins within the Paparoa Trough based on the N–S distribution of Eocene facies of the Rapahoe Group. The three northern sub-basins correspond to the depocentres defined herein (i.e. regions with metamorphic, sedimentary, and igneous/metasedimentary basement). Lever (Citation2001) interpreted these as shoreface, deep marine and shallow marine environments, respectively, which is consistent with Oligocene facies trends presented in this study. The most northerly sub-basin coincides with the distribution of the Little Totara Sand and isolated algal facies within the Kaiata Formation at Gibson Beach, which are thought to be derived from a palaeohigh at the nearby Cape Foulwind Quarry (Macgregor Citation1983) and roughly match both the distribution and composition of the Waitakere Limestone. The second sub-basin is defined by the Kaiata Formation and coincides with thicker sections of the deeper water Tiropahi Limestone. Finally, the third sub-basin contains Island Sandstone, which matches the distribution of the marine plateau of the Potikohua Limestone in the lower package of the Nile Group.
Miocene basin geometry
The Early Miocene Blue Bottom Group also appears to be affected by the Paparoa Core Complex fault geometry. Along the western flanks of the Paparoa Range, the lower Blue Bottom Group consists of the Welsh and younger O'Keefe formations. The Welsh Formation is absent north of the Fox River (; Nathan Citation1975; Laird Citation1988), thus the longest time gap between Oligocene and Miocene strata lies over the metamorphic core and persistent palaeohigh. South of the Fox River, the Welsh Formation is present between the Nile Group and O'Keefe Formation, and thicknesses of the Welsh Formation are greatest above the Pororari Group basement. Furthermore, to the south, a carbonate horizon (Razorback Member) composed of flaggy grainstone is present in the lower Welsh Formation, corresponding with the dramatic thinning and coarsening of Oligocene lithologies overtop undeformed granites of the southern hanging wall (Laird Citation1988).
Implications for basin geometry and maximum drowning
This study has implications for the question of maximum drowning of New Zealand. The widespread bryozoan grainstones (Potikohua Limestone) that are characteristic of the maximum drowning phase are shown herein to reflect diminished topography; in this case, the filling of a local depocentre, coinciding with a regional relative sea level rise and close to complete shut off of clastic supply to the marine realm. This implies paucity of immediately adjacent land area in the Buller and Grey area during the Late Oligocene (Duntroonian and possibly Waitakian). From the associations between the metamorphic core of the Paparoa Core Complex and greatest relief noted herein, the most likely location of exposure and terrestrial environments is in the northern or southern end of the field area (i.e. distal from the detachment fault) and on the western side of the Cape Foulwind Fault (Laird Citation1970). This is also consistent as a source for the plant debris observed in the Nile River section.
Conclusions
The Nile Group strata along the western Paparoa Range record a complex palaeogeography, including palaeohighs, submarine depressions and marine plateaus separated by an ESE–WNW-trending trough. There is a clear relationship between the structure of the mid-Cretaceous Paparoa Core Complex and overlying Oligocene strata. Despite extensive sedimentation during the Eocene, topographic expression of the Paparoa Core Complex persisted into the Oligocene and possibly into the Miocene as well. With this in mind, palaeogeographic models of the Challenger Rift System should take into account potential influence from these WNW–ESE-trending, core complex- and other older rift-related faults. Compelling evidence for non-marine Oligocene environments points to a western high centred on the western extension of the gneissic and mylonitic basement that formed the centre of the Paparoa Core Complex.
Acknowledgements
This study was made possible by Mason Trust Funds for field work, and a University of Canterbury Doctoral Scholarship to NKR. We thank M. Laird in particular, who shared his knowledge of the West Coast and directed us to many of the important outcrops. This paper was greatly improved by discussions with S. Nathan, C. Nelson, P. Kamp, and F. Ghisetti. Helen Lever and Mark Rattenbury reviewed the manuscript and provided invaluable suggestions.
References
- Anastas AS, Dalrymple RW, James NP, Nelson CS 2006. Lithofacies and dynamics of a cool-water carbonate seaway: mid-Tertiary, Te Kuiti Group, New Zealand. Geological Society, London, Special Publications 255: 245–268.
- Anderson JM 1984. Sedimentology and diagenesis of an Oligocene carbonate sequence (Nile Group), Westport to Punakaiki. Unpublished MSc thesis. Wellington, Victoria University. 390 p.
- Betzler C, Brachert TC, Nebelsick JH 1997. The warm temperate carbonate province: A review of the facies, zonations, and delimitations. Courier Forschungsinstitut Senckenberg 201: 83–99.
- Bishop DJ 1992. Extensional tectonism and magmatism during the middle Cretaceous to Paleocene, North Westland, New Zealand. New Zealand Journal of Geology and Geophysics 35: 81–91. 10.1080/00288306.1992.9514502
- Cooper A, Cooper RA 1995. The Oligocene bottleneck and New Zealand biota: genetic record of a past environmental crisis. Proceedings of the Royal Society of London 261: 293–302. 10.1098/rspb.1995.0150
- Forster MA, Lister GS 2003. Cretaceous metamorphic core complexes in the Otago Schist, New Zealand. Australian Journal of Earth Sciences 50: 181–198. 10.1046/j.1440-0952.2003.00986.x
- Freiwald A 1993. Coralline algal maerl frameworks - Islands within the phaeophytic kelp belt. Facies 29: 133–148. 10.1007/BF02536925
- Furlong KP, Kamp PJJ 2013. Changes in plate boundary kinematics: punctuated or smoothly varying - Evidence from the mid-Cenozoic transition from lithospheric extension to shortening in New Zealand. Tectonophysics 608: 1328–1342. 10.1016/j.tecto.2013.06.008
- Gibson GM, McDougall I, Ireland TR 1988. Age constraints on metamorphism and the development of a metamorphic core complex in Fiordland, Southern New Zealand. Geology 16: 405–408. 10.1130/0091-7613(1988)016%3C0405:ACOMAT%3E2.3.CO;2
- Henrich R, Freiwald A, Betzler C, Bader B, Schäfer P, Samtleben C et al. 1995. Controls on modern carbonate sedimentation on warm temperate to arctic coasts, shelves and seamounts in the Northern Hemisphere: implications for fossil counterparts. Facies 32: 71–108. 10.1007/BF02536865
- Kamp PJJ 1986. Relationship of the West Coast, North Island, igneous bodies to the mid-Cenozoic Challenger Rift System and subduction of the Pacific plate. New Zealand Journal of Geology and Geophysics 29: 51–60. 10.1080/00288306.1986.10427522
- Kanazawa K 1992. Adaptation of test shape for burrowing and locomotion in spatangoid echinoids. Palaeontology 35: 733–750.
- Kimbrough DL, Tulloch AJ 1989. Early Cretaceous age of orthogneiss from the Charleston Metamorphic Group, New Zealand. Earth and Planetary Science Letters 95: 130–140. 10.1016/0012-821X(89)90172-6
- King P 2000. Tectonic reconstructions of New Zealand: 40 Ma to the present. New Zealand Journal of Geology and Geophysics 43: 611–638. 10.1080/00288306.2000.9514913
- Kula J, Tulloch AJ, Spell TL, Wells ML, Zanetti KA 2009. Thermal evolution of the Sisters Shear Zone, southern New Zealand; Formation of the Great South Basin and onset of Pacific-Antarctic spreading. Tectonics 28: 1–22. 10.1029/2008TC002368
- Laird MG 1970. Notes on the paleogeography of the Haku 1 site and adjacent areas of the West Coast. Unpublished Geological Survey Petroleum Report Haku 1. PR 553. Held at the Geological Survey Library, Lower Hutt. 11 p.
- Laird MG 1971. Sedimentology of the Greenland Group in the Paparoa Range, West Coast, South Island. New Zealand Journal of Geology & Geophysics 15: 372–393. 10.1080/00288306.1972.10422338
- Laird MG 1988. Sheet S37 - Punakaiki. Geological map of New Zealand 1:63,360. New Zealand Geological Survey. Wellington, Department of Scientific and Industrial Research.
- Laird MG 1994. Geological aspects of the opening of the Tasman Sea Basin. In: Van Lingen GJ, Swanson K, Muir RJ ed. The evolution of the Tasman Sea Basin. Rotterdam, Balkema. Pp. 1–17.
- Laird MG 1995. Coarse-grained lacustrine fan-delta deposits (Pororari Group) of the northwestern South Island, New Zealand: evidence for mid-Cretaceous rifting. In: Plint AG ed. Sedimentary Facies Analysis (International Association of Sedimentologists). Oxford, Blackwell Science Ltd. Pp. 197–217.
- Laird MG, Bradshaw JD 2004. The break-up of a long-term relationship: the Cretaceous separation of New Zealand from Gondwana. Gondwana Research 7: 273–286. 10.1016/S1342-937X(05)70325-7
- Lever H 2001. An Eocene to early Oligocene unconformity-bounded sequence in the Punakaiki-Westport area, West Coast, South Island, New Zealand. New Zealand Journal of Geology and Geophysics 44: 355–363. 10.1080/00288306.2001.9514944
- Lever H 2007. Review of unconformities in the late Eocene to early Miocene successions of the South Island, New Zealand: ages, correlations, and causes. New Zealand Journal of Geology and Geophysics 50: 245–261. 10.1080/00288300709509835
- Macgregor AR 1983. The Waitakere Limestone, a temperate algal carbonate in the lower Tertiary of New Zealand. Journal of the Geological Society of London 140: 387–399. 10.1144/gsjgs.140.3.0387
- Mortimer N 2004. New Zealand's Geological Foundations. Gondwana Research 7: 261–272. 10.1016/S1342-937X(05)70324-5
- Muir R, Ireland T, Weaver SD, Bradshaw JD 1997. SHRIMP U-Pb geochronology of Cretaceous magmatism in northwest Nelson-Westland, South Island, New Zealand. New Zealand Journal of Geology & Geophysics 43: 37–41.
- Nathan S 1974. Stratigraphic nomenclature for the Cretaceous-Lower Quaternary rocks of Buller and North Westland, West Coast, South Island, New Zealand. New Zealand Journal of Geology & Geophysics 17: 423–45. 10.1080/00288306.1974.10430401
- Nathan S 1975. Sheets S23 & S30 - Foulwind and Charleston. Geological Map of New Zealand, 1:63,360. New Zealand Geological Survey. Wellington, Department of Scientific and Industrial Research.
- Nathan S 1978a. Sheet S31 and part S32 - Buller - Lyell. Geological map of New Zealand 1:63,360. New Zealand Geological Survey. Wellington, Department of Scientific and Industrial Research.
- Nathan S 1978b. Sheet S44 - Greymouth. Geological map of New Zealand 1:63,360. New Zealand Geological Survey. Wellington, Department of Scientific and Industrial Research.
- Nathan S, Anderson HJ, Cook RA, Herzer RH, Hoskins RH, Raine JI et al. 1986. Cretaceous and Cenozoic sedimentary basins of the West Coast region, South Island, New Zealand. New Zealand Geological Survey basin studies 1. Wellington, SIPC and DSIR. 89 p.
- Nathan S, Rattenbury MS, Suggate RP 2002. Geology of the Greymouth Area, 1 map 1:250,000 scale. Lower Hutt, GNS Science. 58 p.
- Nebelsick J, Stingl V, Rasser M 2001. Autochthonous facies and allochthonous debris flows compared: early Oligocene carbonate facies patterns of the Lower Inn Valley (Tyrol, Austria). Facies 44: 31–46. 10.1007/BF02668165
- Nelson CS 1978. Temperate shelf carbonate sediments in the Cenozoic of New Zealand. Sedimentology 25: 737–771. 10.1111/j.1365-3091.1978.tb00328.x
- Nelson CS, Hancock GE, Kamp PJJ 1982. Shelf to basin, temperate skeletal carbonate sediments, Three Kings Plateau, New Zealand. SEPM Journal of Sedimentary Research 52: 717–732.
- Nelson CS, Keane S, Head P 1988. Non-tropical carbonate deposits on the modern New Zealand shelf. Sedimentary Geology 60: 71–94. 10.1016/0037-0738(88)90111-X
- Rasser MW, Nebelsick JH 2003. Provenance analysis of Oligocene autochthonous and allochthonous coralline algae: a quantitative approach towards reconstructing transported assemblages. Palaeogeography, Palaeoclimatology, Palaeoecology 201: 89–111. 10.1016/S0031-0182(03)00512-1
- Rattenbury MS, Cooper RA, Johnston MR. 1998. Geology of the Nelson Area, 1:250 000 geological map 9. Lower Hutt, Institute of Geological & Nuclear Sciences.
- Scoffin T 1988. The environments of production and deposition of calcareous sediments on the shelf west of Scotland. Sedimentary Geology 60: 107–124. 10.1016/0037-0738(88)90113-3
- Tulloch AJ, Kimbrough DLD 1989. The Paparoa Metamorphic Core Complex, New Zealand: Cretaceous extension associated with fragmentation of the Pacific margin of Gondwana. Tectonics 8: 1217–1234. 10.1029/TC008i006p01217
- Wellman HW, Beck AC, Kear D, Suggate RP, Grindley GW 1981. Stratigraphic columns for the Cretaceous-lower Quaternary sediments of north-west Nelson and the West Coast, South Island. New Zealand Geologic Survey Report 63. Lower Hutt, New Zealand Geological Survey. 102 p.