Abstract
Granite Harbour Intrusive Complex xenoliths in McMurdo Volcanic Group rocks and in situ outcrops have been studied from Mount Morning, western McMurdo Sound, Antarctica. Calc-alkalic samples have whole rock signatures and normative compositions similar to the Dry Valleys 1b suite, and zircon grains in one specimen yield a 545.2 ± 4.4 Ma crystallisation age. This supports subduction-related magmatism initiating in Southern Victoria Land by 545 Ma. A second group of xenoliths is alkalic, with titanite grains in one xenolith from this group dated at 538 ± 8 Ma. Whole rock chemistry, normative compositions and geochronology of the alkalic group are comparable to the Koettlitz Glacier Alkaline Suite (KGAS). The position of a proposed lower crustal discontinuity that may form a significant basement suture in the McMurdo Sound region is newly constrained to the east of Mount Morning, perhaps along the trace of the Discovery Glacier. The boundary between East and West Antarctica may also pass along the trace of the Discovery Glacier if, as previously hypothesised, its location is controlled by the basement suture. A significant basement suture may also have provided the necessary egress for the (regionally) early and sustained magmatic activity observed at Mount Morning over the last 24 million years.
Introduction
Antarctica's Ross Orogen formed during Neoproterozoic and early Paleozoic Gondwanaland amalgamation, creating part of one of the world's longest orogenic belts, the Terra Australis orogen (Cawood Citation2005). In the McMurdo Sound region of Antarctica, the stretched and thinned lithosphere underlying the Ross Sea, associated with the West Antarctic Rift System, sits adjacent to the up-thrown Ross Orogen Transantarctic Mountains, to provide dramatic topographic relief of up to 5000 m between East and West Antarctica (–). The boundary, or front (Transantarctic Mountains Front or TMF), between East and West Antarctica is at the eastern edge of the Transantarctic Mountains. In the McMurdo Sound region the position of the TMF has been seismically mapped north of a prominent, approximately east-west trending lineament (the Blue Lineament; Wilson Citation1999) at approximately 78ºS (McGuiness et al. Citation1985; Barrett et al. Citation1995), but south of this the location of the TMF is debated (). In the McMurdo Sound region a major and significant discontinuity in the lower crust has been proposed based upon geophysics (Smithson Citation1972) and isotopic and geochemical studies of lower crustal xenoliths entrained in Cenozoic magmas (Kalamarides et al. Citation1987; Berg et al. Citation1989; Berg Citation1991; Kalamarides & Berg Citation1991). It has also been suggested that this discontinuity may mark a difference in age, although no supporting geochronological evidence has been presented (Kalamarides et al. Citation1987). The discontinuity is thought to occur at the TMF between East and West Antarctica, and Kalamarides et al. (Citation1987) proposed that ‘the isotopic differences in the lower crust across the boundary suggest that the current faulting and rifting may coincide with an older crustal suture.’ They drew the TMF position to coincide with the seismically mapped boundary north of the Blue Lineament and south of the Blue Lineament they defined an approximate position for the TMF using information obtained from lower crustal xenolith information (). One limitation of their study was the availability of lower crustal xenolith localities close to the approximate TMF position south of Brown Peninsula (). A slightly different position for the TMF is suggested by Wilson et al. (Citation2007) in their figure 1 ( of this study), where they infer the TMF to continue south of the Blue Lineament to intersect a second, approximately east-west, prominent lineament (the Radian Lineament; Wilson Citation1999). Wilson et al. (Citation2007) infer a c. 10 km dextral offset of the TMF at the Radian Lineament. To the south of the Radian Lineament, the TMF is inferred to follow a third, approximately north-south trending, prominent structural feature: the Pyramid Lineament (Wilson Citation1999).
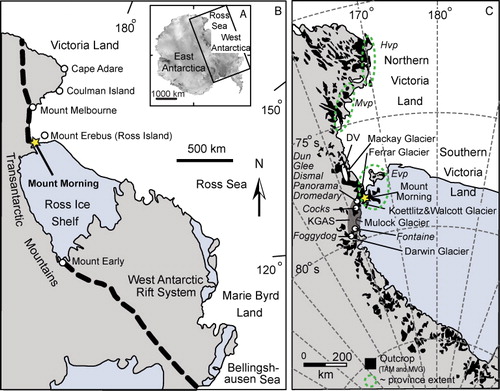
In this study a suite of 46 crustal xenoliths and two in situ outcrops of basement from Mount Morning, a Cenozoic eruptive centre in McMurdo Sound, have been examined. Mount Morning is located immediately south of the Radian Lineament and east of the Pyramid Lineament in the area of the inferred position of the TMF (–). The crustal sample suite has been examined using petrography and whole rock geochemistry and a sub-set of samples has been analysed for mineral geochemistry, thermometry, and geochronology to distinguish between an East Antarctica (Transantarctic Mountain-like) or West Antarctica (Ross Sea-like) affinity for the crust beneath Mount Morning. The results are significant because (i) they refine the position of the lower crustal discontinuity first proposed by Kalamarides et al. (Citation1987); (ii) they can be used to assess the effect of refining the position of the lower crustal discontinuity on the inferred position of the TMF; (iii) they can advance understanding of the petrogenesis of the crust beneath Mount Morning as it relates to either the Transantarctic Mountains or McMurdo Sound; and iv) they can be used to discuss the control, if any, of the pre-existing basement boundary suture (Kalamarides et al. Citation1987) on the distribution of Cenozoic volcanism.
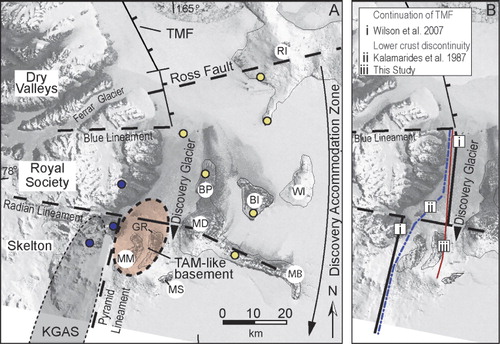
Geological setting
Mount Morning is a stratovolcano cropping out in the Ross Sea c. 100km south-west of the active Mount Erebus volcano, within the West Antarctic Rift System (). Mount Morning has been active for at least 18.7 Ma and probably as far back as at least 24.1 Ma (Martin et al. Citation2010). This is longer lived than any other Cenozoic eruptive centre or province in Antarctica (LeMasurier & Thompson Citation1990; Martin et al. Citation2010). The volcano consists of the strongly alkalic Mason Spur Lineage and the mildly alkalic Riviera Ridge Lineage (Martin et al. Citation2013). The crustal xenoliths were collected exclusively in Riviera Ridge Lineage rocks that erupted between at least 6.13 and 0.02 Ma (Martin et al. Citation2010). Faults, interpreted as the onshore continuation of part of the TMF fault array, have been mapped on the north-east flank of Mount Morning (Kyle & Muncy Citation1989; Martin & Cooper Citation2010). Based upon thermobarometry of plagioclase-bearing mantle xenoliths, the crust beneath Mount Morning is thought to be < 20 km thick (Martin et al. Citation2014). To the east of Mount Morning the Ross Sea crust is estimated to be 18–20 km thick and it has been suggested that to the west of Mount Morning, beneath the Transantarctic Mountains, the crustal thickness increases to around 40 km (Berg et al. Citation1989; Bannister et al. Citation2003).
West of the West Antarctic Rift System are the Transantarctic Mountains (–), a product of rift flank uplift in Southern Victoria Land since the Palaeogene (Fitzgerald Citation1992). The Transantarctic Mountains comprise late Precambrian to Cambrian Ross Orogen lithologies of the Skelton Group (Cooper et al. Citation2011) that include various greywackes, schists, metaconglomerates, argillites, quartzites, marbles, and metavolcanic rocks (Borg & DePaolo Citation1991; Stump Citation1992, Citation1995; Cook & Craw Citation2001; Cox et al. Citation2012). These are unconformably overlain by Devonian to Triassic aged sediments of the Beacon Supergroup (Barrett Citation1981, Citation1991; Collinson Citation1991; Woolfe & Barrett Citation1995), into which the Ferrar Dolerite was injected during the Jurassic (Elliot Citation1992; Heimann et al. Citation1994). The development of the Ross Orogen was punctuated by voluminous magmatism, with the emplacement of plutons that are collectively referred to as the Granite Harbour Intrusive Complex (GHIC; Gunn & Warren Citation1962; Findlay Citation1985; Stump Citation1995; Cox et al. Citation2012). The GHIC rocks were emplaced into the Skelton Group between the late Precambrian and early Paleozoic (e.g. Encarnacion & Grunow Citation1996; Cox et al. Citation2000; Allibone & Wysoczanski Citation2002; Tulloch & Ramezani Citation2012) and the history is summarised in Cox et al. (Citation2012) and Goodge et al. (Citation2012). Despite the size of the Ross Orogen, its study is challenged by remoteness, inclement weather, long periods of darkness, extreme relief, and obscuring ice cover. In the Transantarctic Mountains, the GHIC comprises four distinct suites of plutonic rocks. Three of these (Dry Valleys 1a–DV1a; Dry Valleys 1b–DV1b and Dry Valleys 2–DV2) are calc-alkalic or alkalic-calcic and consist mainly of granitoids (Smillie Citation1992; Allibone et al. Citation1993a,Citationb; Cox et al. Citation2000; Allibone & Wysoczanski Citation2002). Both the DV1a and DV1b suites have chemistry consistent with their generation and emplacement in a convergent margin setting. The DV1a Suite plutons have calc-alkalic compositions with relatively low Sr/Y ratios (LoSY; Tulloch & Kimbrough Citation2003), whereas DV1b Suite plutons have alkalic-calcic chemistry and high Sr/Y ratios (HiSY), which has also been referred to as ‘adakite-like’. The DV2 suite is alkalic-calcic and associated with high-K and shoshonitic dyke swarms (e.g. Keiller Citation1991). The fourth plutonic suite is the alkalic, Precambrian to Cambrian Koettlitz Glacier Alkaline Suite (KGAS) that crops out between the Walcott and Darwin Glaciers (Cooper et al. Citation1997; Read et al. Citation2002; Cottle & Cooper Citation2006a; ). Rock types include syenites, carbonatites, and A-type granite intrusives (Rowell et al. Citation1993; Hall et al. Citation1995; Read et al. Citation2002; Read Citation2010). Despite the overall compressional regime in this section of Antarctica during the Precambrian to early Palaeozoic (Gunn & Warren Citation1962; Borg & DePaolo Citation1991; Stump Citation1992, Citation1995), the KGAS alkalic chemical associations are considered to have been emplaced in an extensional tectonic setting (Read et al. Citation2002) that may have been part of an extensional jog associated with oblique compression during the Ross Orogeny (Encarnacion & Grunow Citation1996; Read et al. Citation2002) or represent a period of more widespread extension prior to the onset of convergence (Allibone Citation1988).
The lower crustal discontinuity in the McMurdo Sound region is based upon the study of mainly granulites (Kalamarides et al. Citation1987; Berg et al. Citation1989; Berg Citation1991; Kalamarides & Berg Citation1991) the location of which is shown in . The granulite xenoliths from the Transantarctic Mountains are, relative to granulite from the Ross Sea area, characterised by higher δ18O, 87Sr/86Sr and SiO2 content, and by retention of original calc-alkalic major and trace element signatures despite granulite facies metamorphism (Kalamarides et al. Citation1987; Berg et al. Citation1989; Berg Citation1991; Kalamarides & Berg Citation1991). The granulite xenoliths from the Ross Sea area are reported to contain lower silica and are more basic relative to granulite from the Transantarctic Mountains, and they may have originated from alkalic or tholeiitic parents. It has also been speculated that an age difference may exist between Transantarctic Mountain-like lower crust and lower crust beneath the Ross Sea (Kalamarides et al. Citation1987; Kalamarides & Berg Citation1991), although there is little geochronological evidence published to support this hypothesis.
Methods
Whole rock chemistry
Representative bulk rock samples from Mount Morning were analysed by X-ray fluorescence (XRF) at the University of Otago, New Zealand, on a Philips PW2400 XRF spectrometer, following analytical procedures described in Martin et al. (Citation2013) and Norrish & Chappell (Citation1977) and using the SuperQ qualitative software control program (3rd ed.). Samples were crushed using a TEMA swing mill fitted with a WC head. Martin et al. (Citation2013) have demonstrated that for samples prepared in WC carbide, significant contamination is limited to W and Co. Fused discs were produced for major element analyses (and subsequently used for some trace element analyses) and pressed powder pellets for trace elements. The lower limit of detection is better than 0.004 wt% (weight percent) oxide (for Al2O3). Loss on ignition is at 1100 °C. In representative, selected samples, whole rock trace elements were analysed by laser-ablation inductively coupled mass spectrometry (LA-ICPMS) in the Otago Community Trust Centre for Trace Element Analysis at the University of Otago. After major element analysis, the XRF discs were cut, mounted in epoxy resin and polished. Laser ablation was conducted with a pulsed NewWave 213 nm laser operated at 50%, 10 Hz, a 100 µm spot size and a tracking rate of 10 µm/s. Ablated material was carried by He gas from the sample cell, mixed with Ar and inlet into an Agilent 7500 ICP-MS. Background (laser off) data were acquired for 20 s followed by 40 s of analyses with the laser on giving about 100 mass scans from a 400 µm track approximately 5 µm deep. A purge time of at least 120 s was allowed between each spot analysis. Each set of tracks on ten unknowns was bracketed by analyses of standard glass NIST 610 (Pearce et al. Citation1997). Trace-element concentrations were obtained by normalising count rates for each element to those for Si in the sample and standard using known SiO2 and trace element concentrations in NIST 610 and the SiO2 in the fused discs obtained previously by XRF analysis. The J-series rock standards, by comparison with results reported in Orihashi et al. (Citation2003), show precision (1σ relative) of ≤ 5% for ≥ 10 ppm, ≤ 20% for 1–10 ppm and ≤ 50% for 0.1–1 ppm. In terms of accuracy, all results are within 25% (and most within 10%) of values reported by Orihashi et al. (Citation2003).
Mineral chemistry
The JEOL JXA-8600 electron microprobe at the University of Otago was used to determine mineral major element chemistry in wavelength dispersive mode, operated with accelerating voltage 15 kV, current 1 μA and beam diameter 20 μm. A standard ZAF correction was applied to all data. Natural silicates and oxides were used as standards. A typical lower limit of detection is shown in the online supplementary material. Zircon mineral rare earth element (REE) and Ti chemistry were collected from specimen OU78656 at the same time as U-Pb data was collected for geochronology (see below for instrument configuration) at the Australian National University. Count rates for each element were normalised to Si, assuming SiO2 to be stoichiometric in zircon with a concentration of 32.8 wt%, and multiplying by a correction factor based on measurement of standard glass NIST 610 with concentration values recommended by Pearce et al. (Citation1997). The low abundance 49Ti was chosen to avoid interference with 96Zr on the major 48Ti peak.
U-Pb chronology
Zircon grains from sample OU78656 were analysed by LA-ICPMS at the Australian National University. Grains were separated by conventional techniques, handpicked and mounted in polished epoxy mounts. Cathodoluminescence images were used to target specific grains and zones within grains for analysis. Instrument settings were set at a 35 µm spot diameter at 5 Hz with 70% power, with fluence values c. 2.47 J/cm2 typical, and further details are given in Ballard et al. (Citation2001). Data reduction and uncertainty propagation methods are described in Scott & Palin (Citation2008). Standard data from NIST-610 were collected and zircon reference material TEMORA-1 (Black et al. Citation2003) was used as the primary standard: the reproducibility of the reference material was < 5% (2σ) for both 207Pb/206Pb and 206Pb/238U.
Uranium-Pb geochronology of titanite was determined at the NERC Isotope Geosciences Laboratory (NIGL), Keyworth, Nottingham, using a polished thin section of sample OU78658. Analyses were made in situ with a Nu Instruments AttoM single collector ICPMS and a New Wave Research 193FX laser ablation system. The full method and instrument parameters employed are described in Thomas et al. (Citation2013). Laser ablation was conducted with a 35μm static spot at a fluence of c. 2.2 J/cm2 for 30 seconds. Sample data were normalised to Ontario-2 titanite reference material (Spencer et al. Citation2013), and Khan and Fish Canyon Tuff titanite reference materials were also analysed during the session to check on precision and accuracy. Analyses of Khan titanite gave an age of 516 ± 7 Ma 2σ (ID-TIMS age: 522 Ma; unpublished NIGL data) and analyses of Fish Canyon Tuff titanite gave an age of 30.1 ± 1.7 Ma 2σ (ID-TIMS age: 28.5 Ma; Schmitz & Bowring Citation2001).
Results
Petrography and sample description
Forty-eight samples were selected from a range of localities across Mount Morning. The most prolific crustal xenolith site occurs in an isolated cinder cone on the upper flanks of Mount Morning (78.26.044 south; 164.26.479 east; altitude 1118 m). All sample locations are given in the online supplement Table S1. Xenolith diameters range between < 1 cm and 50 cm (–). In rare cases crustal xenoliths comprise 20% of the outcrop of the host basanite (Paulsen Citation2008 and see ) but abundances are commonly much lower (). In addition, two in situ outcrops of rocks belonging to the GHIC (Kyle & Muncy Citation1989; Martin & Cooper Citation2010) were also sampled; the petrographic characteristics of these samples have been described previously (Martin & Cooper Citation2010). Based upon macroscopic texture the 48 samples have been divided into metamorphic (granulite) and igneous (plutonic) sub-types. The petrographic details are summarised in . Of the thirteen granulites collected, only 1 is mafic, with the remaining igneous sub-types representing a full range of felsic to intermediate lithologies.
Table 1 Summary of sample petrography.
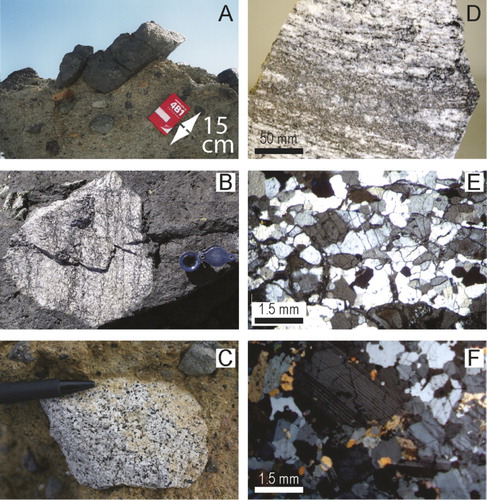
The granulite xenoliths have a foliation defined by the common orientation of the long axis of mineral grains and alternation of leucocratic and melanocratic bands (2 to 20 mm-thick; ), with the banding defined by the abundance of pyroxene. No garnet was identified in any granulite specimen analysed as part of this study or observed in the field. One felsic granulite specimen (OU78656) has been isotopically dated and is described here. The sample is leucocratic and equigranular (0.75 mm) with a foliation defined (–) by the common orientation of the long-axes of quartz, alkali feldspar, plagioclase, orthopyroxene, and deformed FeTi-oxide grains and the alternation of mm-scale light and dark bands (pyroxene-poor and -rich respectively). Accessory minerals are zircon (Fig. S1), apatite, and titanite. One igneous-textured specimen (OU78658) has also been dated. This sample has an equigranular (1 mm), coarse-grained texture () and is composed of labradorite, microcline, clinopyroxene, ferropargasitic amphibole, titanite, and quartz, plus trace amounts of biotite, apatite, and magnetite.
Whole rock chemistry and normative QAP compositions
The whole rock data are summarised in with full details provided in the online supplementary Table S1. Whole rock compositions are plotted onto the plutonic TAS (total alkalis versus silica) diagram of Middlemost (Citation1994) in . The samples that plot above the alkalic–sub-alkalic division line of either Macdonald & Katsura (Citation1964) or Kuno (Citation1966) are alkalic (black stars on ). The remaining samples are sub-alkalic and plot in the calc-alkalic field of Irvine & Baragar (Citation1971; online supplementary figure S2). All the metamorphic textured granulites are calc-alkalic with the igneous textured xenoliths being both calc-alkalic (the majority) and alkalic. None of the samples has a tholeiitic composition.
Table 2 Whole rock geochemistry.
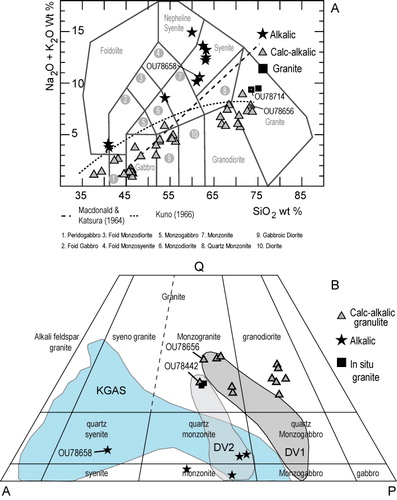
The normative compositions of the granulite xenoliths have been calculated and plotted onto the quartz-alkali feldspar-plagioclase feldspar (QAP; Streckeisen Citation1976) diagram where they plot in the granodiorite and monzogranite fields (). The majority of monzogranite data, including dated specimen OU78656, overlap with the field defined for DV1 suite rocks defined by Smillie (Citation1992). One monzogranite specimen (OU78442) and the two samples from in situ outcrop overlap with the field defined for DV2 rocks, though they also plot very close to the DV1 field. The granulite specimens with granodiorite protolith compositions do not overlap with either of the DV1 or DV2 fields defined by Smillie (Citation1992). The normative compositions of the crustal xenoliths with alkalic whole rock compositions are also plotted on . Four specimens, including dated specimen OU78658, lie in the field defined for KGAS by Read (Citation2010). One specimen plots with a monzonite composition slightly outside of the KGAS field and five samples (not shown) have nepheline normative compositions. The Mount Morning granulite xenoliths have high SiO2 contents between c. 65 and 75 wt%, and Fe2O3 less than about 5 wt% (). The whole rock compositions have around 15 wt% Al2O3, variable P2O5 between 0.05 and 0.15 wt% () and up to 5000 ppm Ba ().
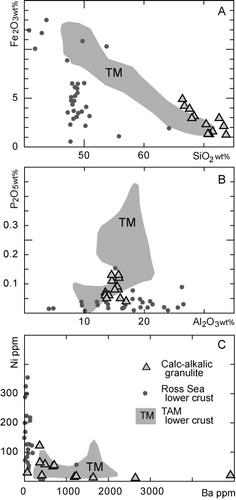
One alkalic igneous (OU78658) specimen, one calc-alkalic metamorphic (OU78656) specimen, and one calc-alkalic igneous (OU78714) specimen are plotted on a normal mid ocean ridge basalt (N-MORB) normalised extended element plot (), with other whole rock crustal xenolith patterns shown in grey lines for comparison. All patterns have decreasing enrichment levels with increasing element compatibility and marked negative anomalies (relative to the element immediately before and after) of Nb, P, and Ti and enrichments in K, Pb, and Nd. For comparison, a representative alkalic Riviera Ridge Lineage volcanic specimen (OU78540) from Mount Morning is plotted in . This sample lacks a negative Nb or Ti anomaly and does not have a significant K, Pb, or Nd anomaly. Whole rock analyses from representative GHIC samples in the Transantarctic Mountains are shown in for further comparison and are discussed below.
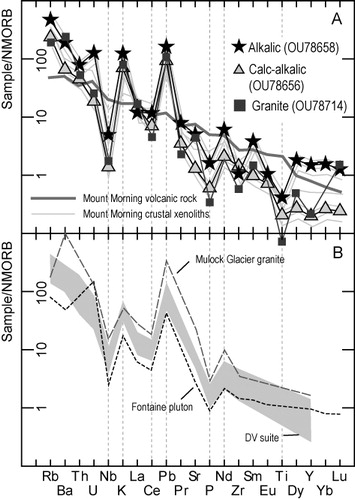
The felsic granulite xenoliths and the two in situ Gandalf Ridge granites have been plotted onto granite discrimination diagrams in – and lie within the volcanic arc granite and the I- and S-type granite fields. On a Y versus Sr/Y plot () the granites and felsic granulites have low Y and high Sr and almost all plot within the adakite field defined by Defant et al. (Citation2002) and may be described as HiSY.
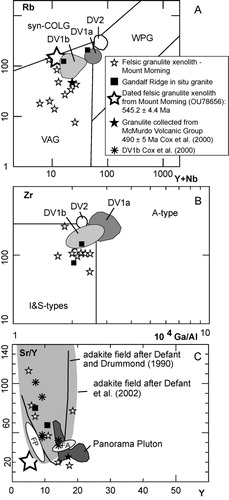
Mineral chemistry and thermometry
Feldspar and zircon mineral chemistry was collected from dated specimen OU78656 with representative compositions shown in and the complete analyses given in the online supplement Table S2. Two feldspar compositions are present: plagioclase with a typical andesine composition of An32Ab59Or9; and orthoclase with a typical composition of An2Ab27Or71 (). In zircon, REEs and Ti have been measured. The mantle normalised zircon REE patterns have a steeply positive trend and Nd and Eu depletions relative to the elements with similar compatibility (). The measured Ti abundance varies between 9 and 43 ppm, with the median value being 16 ppm (n = 8).
Table 3 Mineral chemistry from granulite specimen OU78656.
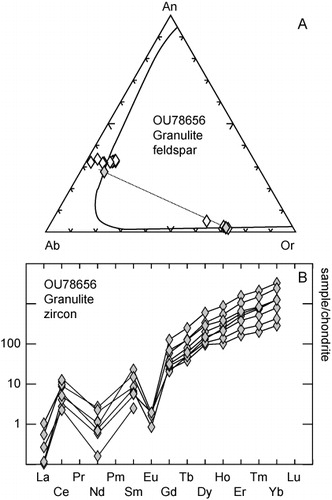
Two-feldspar geothermometry is based upon the exchange between An-Ab-Or components of co-existing orthoclase and plagioclase feldspars such as seen in OU78656. Problems exist with the application of two-feldspar geothermometry to metamorphic rocks, as noted by Elkins & Grove (Citation1990) and Fuhrman & Lindsley (Citation1988) and reviewed by Kroll et al. (Citation1993). The major problem is that often the two feldspar pairs do not plot on a single isotherm, which invariably leads to error in the temperature calculation (Kroll et al. Citation1993). The two-feldspar analyses in OU78656 do, however, plot on a single isotherm (), thus in this instance the two-feldspar geothermometry method is applicable. A maximum pressure of 15 kb is thought reasonable for the geothermometry calculations based upon the thickness of crust beneath the adjacent Transantarctic Mountains (Berg et al. Citation1989; Bannister et al. Citation2003). Application of two different geothermometers returns very similar results of 871 °C (Fuhrman & Lindsley Citation1988) and 887 °C (Elkins & Grove Citation1990). Natural and experimental results have defined a log-linear relationship between temperature and Ti content in zircon (Watson & Harrison Citation2005; Watson et al. Citation2006; Ferry & Watson Citation2007) that has been extensively tested using natural suites of samples, for example see the reviews of Fu et al. (Citation2008), Ewing et al. (Citation2013) and references there-in. Using the Ti values from zircons in sample OU78656, a range of temperatures is calculated between 732 and 887 °C with a median temperature of 779 °C (n = 8).
Geochronology
The petrography of the dated samples is described above and the geochronology results for these are presented in . Zircon grains separated from felsic granulite specimen OU78656 are elongate, optically clear grains ≤ 200 µm in maximum dimension. Oscillatory concentric zoning, typical of magmatic zircon, is discernible on cathodoluminescence images (supplementary Fig. S3). Eighteen zircons were analysed by LA-ICPMS, yielding concordant (± 4%; ) 206Pb/238U results between c. 565 and 531 Ma and a weighted average 206Pb/238U age at 545.2 ± 4.4 Ma (MSWD = 1.2, probability of fit = 0.23; ). For alkalic specimen OU78658, 24 spots were measured across three titanite crystals. These yield a regression towards common lead giving a 206Pb/238U age of 538 ± 8 Ma (2σ; MSWD = 0.94; ).
Table 4 U-Th-Pb isotope data for grains analysed by LA-ICPMS from sample OU78656 and OU78658.
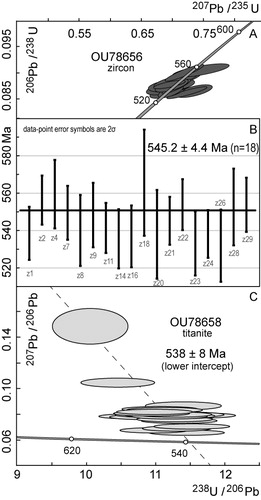
Discussion
Association of Mount Morning lower crust granulite samples
A calc-alkalic composition is evident in Mount Morning lower crustal xenoliths despite granulite facies metamorphism (Fig. S2). This is one of the characteristics Kalamarides et al. (Citation1987) used to define lower crust associated with the Transantarctic Mountains. The Mount Morning granulite data overlap with the Transantarctic Mountain-like field and plot discretely from the Ross Sea-like field – with these discriminatory fields defined by Kalamarides & Berg (Citation1991) – on whole rock major element variation diagrams (). On a QAP diagram, granulite xenoliths representing monzogranite protoliths overlap with the fields for the DV 1 and 2 suites of rocks (). The granulite and in situ granite whole rock multi-element diagrams have negative Nb, P, Ti, and Ce? anomalies and positive K, Pb, and Nd anomalies that are very similar to the whole rock multi-element patterns of the Fontaine Pluton and DV intrusives () and the Panorama Pluton (not shown). The calc-alkalic felsic granulite data and in situ granite data plot in the volcanic arc granite field of the Y+Nb versus Rb diagram and overlap with the field for DV1b suite rocks (). The bulk rock chemistry of several granulite xenoliths, and the in situ granite samples, features relatively low Y values and high Sr/Y ratios () that have been described as either adakite-like or HiSY. The age of the dated granulite specimen, OU78656, overlaps with that of the Fontaine Pluton (and is discussed in detail below). We interpret the petrology, geochemistry and geochronology to indicate that the lower crustal granulite xenoliths from Mount Morning have the defining characteristics provided by Kalamarides et al. (Citation1987) for Transantarctic Mountain-like lower crust and that at least some samples can be specifically related to the DV1b and DV2 suites of rocks (and the Fontaine Pluton).
A possible adakite-like signature in the granulite samples
The bulk rock chemistry of several granulite xenoliths, and the in situ granite samples, features relatively low Y values and high Sr/Y ratios (). These characteristics, along with SiO2 ≥ 56 wt%, Al2O3 ≥ 15 wt%, MgO < 3 wt% are typical of adakite-like rocks (Castillo Citation2006). Defant & Drummond (Citation1990) identified rocks with low Y and Yb values and high Sr/Y and La/Yb ratios in a Cenozoic arc and described them as adakites, and rocks elsewhere in the world with comparable geochemistry are commonly referred to as ‘adakite-like’ (Castillo Citation2006; Wang et al. Citation2007). Much controversy exists over the origin(s) of rocks with an adakitic geochemical signature (see Castillo (Citation2006) for a comprehensive review). The geochemistry is generally interpreted to reflect the involvement of garnet in melting or fractionation processes; Y and HREE such as Yb are strongly partitioned into garnet relative to melt. It has been variously argued that adakite-like rocks are: (a) possible examples of partial melts of subducted oceanic crust (Defant & Drummond Citation1990; Kay et al. Citation1993; Stern & Kilian Citation1996; Defant et al. Citation2002; Martin et al. Citation2005); (b) formed via fractional crystallisation and assimilation processes acting on parental basaltic magmas in an arc setting (Castillo et al. Citation1999); or (c) derived by partial melting of thickened lower crust or a basaltic underplate (Atherton & Petford Citation1993; Wang et al. Citation2007). Mount Morning is underlain by a basement whose age and chemistry are comparable to those of rocks formed during the Ross Orogeny. Ross Orogen plutons have long been convincingly argued to be the product of subduction processes (Borg et al. Citation1990; Encarnacion & Grunow Citation1996; Cox et al. Citation2000; Cottle & Cooper Citation2006b). The close geochemical affinity between Mount Morning basement xenoliths and Transantarctic Mountain plutons (–) can be interpreted to suggest that at least part of the Mount Morning basement originated through magmatic processes taking place in a Cambrian to Precambrian arc. While this does not rule out the possibility that the adakite-like basement rocks at Mount Morning are the product of partial melting of a thickened lower crust, it is considered more likely that they formed from the partial melting of subducted oceanic crust. Martin et al. (Citation2013, Citation2014) have also argued that the metasomatic fluids responsible for mantle refertilisation in the lithosphere beneath Mount Morning were derived from melts formed during early Palaeozoic subduction.
Age of the granulite samples and their significance to Ross Orogen magmatism
Ross Orogen magmatism is thought to have been initiated around 590 Ma as defined by zircon ages in glacial granitoid clasts from the central Transantarctic Mountains (Goodge et al. Citation2012). This age estimate is in agreement with the detrital zircon record preserved in syn- to post-orogenic molasse sediments; these have an age estimated at c. 580 Ma (Goodge et al. Citation2002). Magmatism is thought to have continued to around 480 Ma (Goodge et al. Citation2012). In the central Transantarctic Mountains the magmatism has been divided by Goodge et al. (Citation2012) into three phases. The first phase (I) represents early igneous activity within a developing arc between 590 and 555 Ma. This was followed by a phase of syn-tectonic magma generation (phase II) represented by plutons that were emplaced within deep parts of the Ross Orogen between 555 and 515 Ma, and the third phase (III) of mostly post-tectonic granitoid bodies was emplaced within a rapidly growing arc between 515 and 480 Ma. In Southern Victoria Land Ross Orogen magmatism has been divided into four suites (Cox et al. Citation2012 and see Introduction). The age ranges of various plutons within each suite are summarised on . The early KGAS has distinctive alkaline and A-type affinities with a range of ages reported between 579 to 495 Ma, whereas the DV1a suite is interpreted to have formed between 521 Ma and 485 Ma. The DV1b suite has distinctive adakite-like or HiSY chemistry and has ages reported to be between 536 and 486 Ma. The DV2 suite is interpreted to have been emplaced post-subduction and has ages reported to be between 505 and 492 Ma ().
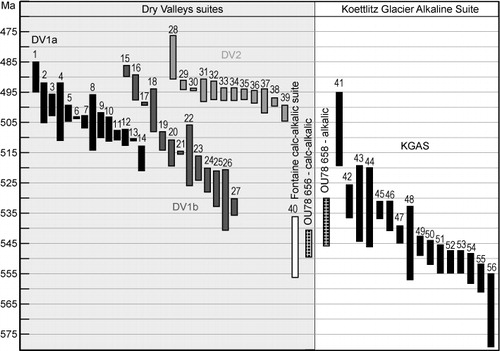
Calc-alkalic granulite specimen OU78656 (this study) is chemically similar to DV1b suite rocks. Both two-feldspar thermometry and Ti in zircon thermometry provide a maximum estimated temperature of 887 °C for this sample (), which is below the zircon closure temperature at about 1000 °C (e.g. Flowers et al. Citation2005). There is some debate as to whether Ti in zircon thermometry represents a crystallisation temperature or cooling temperature (e.g. Ewing et al. Citation2013) but the concentric zoning (Fig. S3) and the REE pattern () in the zircon are typical of igneous zircon (Belousova et al. Citation2002; Fu et al. Citation2009). The agreement between different thermometers is also evidence of rock equilibration and consequently the radiometric data are interpreted to indicate a magmatic crystallisation age. In the context of the regional geology this also makes sense, as the age of sample OU78656 at 545.2 ± 4.4 Ma is at least 5 Ma older than the oldest age reported from the DV1b suite (datum 27 on ), which agrees more with a crystallisation rather than a cooling age. This age is comparable to the Fontaine Pluton some 150 km south of Mount Morning (Cottle & Cooper Citation2006b; ) and further supports the hypothesis that Ross Orogen magmatism was initiated in Southern Victoria Land at around 545 Ma. This age falls within the range of phase II (555 to 515 Ma) Ross Orogen magmatism in the central Transantarctic Mountains and is consistent with development of an early magmatic arc during this period.
Association of Mount Morning alkalic crustal xenoliths
The alkalic xenolith whole rock trace element patterns in have Nb, K, Pb, P, and Ti anomalies similar to those observed in samples of Transantarctic Mountain plutons (including KGAS rocks; ); characteristics not shared with typical Cenozoic alkalic whole rock trace element patterns obtained for Mount Morning volcanic rocks. This is an indication that the alkalic xenoliths are not the plutonic equivalent of Cenozoic volcanic rocks seen at Mount Morning but are in fact chemically similar to GHIC alkalic rocks in the Transantarctic Mountains. In particular the alkalic whole rock chemistry is comparable to the KGAS ( & ). As part of this study, titanite from an alkalic xenolith (OU78658) has been dated at 538 ± 8 Ma, which indicates that this sample is also temporally associated with the GHIC (). Ages derived from titanite grains in KGAS rocks are usually interpreted to reflect cooling ages (e.g. Read Citation2010). Some workers have proposed that U content in titanite is a discriminator between metamorphic and igneous titanite. For example Aleinikoff et al. (Citation2002) suggest metamorphic titanite contains < 100 ppm U; the titanite grains in sample OU78658 contain hundreds of ppm U () as expected in igneous titanite. The euhedral and unzoned morphology of the titanite grains is also consistent with an igneous origin. Although we cannot rule out that the 538 ± 8 Ma date is a cooling age rather than a crystallisation age, the overall conclusion that sample OU78658 is associated with the KGAS () remains valid. By inference the chemistry and chronology are consistent with the suggestion that all of the alkalic xenoliths considered in this study are most appropriately assigned to the KGAS. This interpretation suggests the boundary of the KGAS extends eastwards to include the basement area beneath Mount Morning and this extension is drawn (with approximate borders) in . Even though the broad regional tectonic regime in the TAM at the time of emplacement of the KGAS is compressional, the KGAS chemistry is thought to reflect a localised extensional jog that has been comprehensively discussed in a number of studies (Encarnacion & Grunow Citation1996; Read et al. Citation2002; Read et al. Citation2003; Read Citation2010).
Location of the lower crustal discontinuity
The Mount Morning lower crustal granulites described in this study are geochemically and temporally associated with the DV1b and DV2 rocks and the alkalic xenoliths with the KGAS, and both may be associated with the Transantarctic Mountain-like lower crust described by Kalamarides et al. (Citation1987). Following Kalamarides et al. (Citation1987) this allows the geochemical discontinuity between the Transantarctic Mountain basement and the Ross Sea basement to be drawn east of Mount Morning, reducing the possible uncertainty in the basement discontinuity location to < 25 km. Based upon this interpretation the lower crustal discontinuity probably passes east of Mount Morning and west of Mount Discovery and Minna Bluff, perhaps along the line of the Discovery Glacier (). Constrained by the southern-most extent of the seismically mapped section of the TMF and the occurrences of granulite xenoliths at Mount Morning, the lower crustal discontinuity is a straight line closely aligned with the position of the TMF inferred by Wilson et al. (Citation2007) between the Blue and Radian Lineaments (). However, the new position of the TMF inferred from this study, east of Mount Morning, indicates that little offset of this boundary occurs along the Radian Lineament. The location of the lower crustal boundary to the south of Mount Morning is unknown.
The possible trace of the Transantarctic Mountain front fault and melt migration
Kalamarides et al. (Citation1987) have speculated that the position of the TMF in the McMurdo Sound region is controlled by an ancient lower crustal suture, which they have identified from markedly different isotopic ratios, geochemistry, and possibly chronology in lower crustal granulites collected either side of the TMF. If this line of argument is applied to the observations and conclusions derived from the present study, with the lower crustal geochemical discontinuity lying to the east of Mount Morning, the TMF must also lie to the east of Mount Morning, possibly along the trace of the Discovery Glacier (). The presence of post 3.9 Ma onshore faulting on the north-eastern flank of Mount Morning (Martin & Cooper Citation2010), inferred to form part of the TMF fault array, may support this interpretation. Mantle xenolith geothermobarometry implies that the lithosphere beneath Mount Morning is < 20 km thick (Martin et al. Citation2014), indicating that the crust has been thinned relative to the approximately 40 km-thick crust associated with the adjacent Transantarctic Mountains. This abrupt gradient in crustal thickness may indicate that significant faults associated with the TMF occur to the west of Mount Morning. The final word on the location of the TMF south of the Blue Lineament awaits further geophysical work. However, if Kalamarides et al. (Citation1987) are correct and a lower crustal discontinuity can be mapped in granulite xenoliths in the McMurdo Sound region, then it seems plausible that Pliocene, or earlier, onshore faulting at Mount Morning is closely following the trend of a pre-existing lower crustal suture. In this scenario it is unsurprising that magmatic activity is known earliest at Mount Morning (since 24.1 Ma) out of all the Southern Victoria Land volcanic centres and that magmatism is known to have occurred to at least 0.02 Ma (Martin et al. Citation2010).
Conclusions
A suite of crustal xenoliths, including lower crustal granulites, and two in situ outcrops have been analysed from Mount Morning, Southern Victoria Land, Antarctica. At least some show affinities with Granite Harbour Intrusive Complex rocks from the adjacent Transantarctic Mountains. In particular, the lower crustal granulite xenolith petrography, whole rock chemistry, normative mineralogy, and geochronology are comparable to DV1b suite rocks in the adjacent Transantarctic Mountains. Furthermore, a sub-set of these rocks has adakite-like (or HiSY) compositions that can be explained by partial melting of, or modification by fluids derived from, subducted oceanic crust. Zircon grains from one granulite sample yield a 545.2 ± 4.4 Ma age that is interpreted as a crystallisation age, comparable to the emplacement age of the Fontaine Pluton, and this supports the proposal for the existence of a magmatic arc during this period. The Mount Morning alkalic xenoliths are geochemically comparable to the Koettlitz Glacier Alkaline Suite (KGAS). Titanite grains in one alkalic xenolith yield a 538 ± 8 Ma lower intercept age that may reflect cooling but is within the range of crystallisation and cooling ages reported for KGAS rocks. Based on the study of granulite xenoliths, Kalamarides et al. (Citation1987) have proposed a lower crustal discontinuity in the McMurdo Sound region. The Transantarctic Mountain-like compositions in the Mount Morning granulite suite are consistent with the proposal that this lower crustal discontinuity occurs east of Mount Morning, perhaps along the trace of the Discovery Glacier. The discontinuity could be a significant suture in the lower crust. The Transantarctic Mountain range bounding fault may also pass along the trace of the Discovery Glacier if its location is controlled by the lower crustal suture, as hypothesised by Kalamarides et al. (Citation1987). The extended Cenozoic magmatic history at Mount Morning (more than 24 Myr duration) could be the result of magma exploiting this lower crustal suture.
Supplementary data
Supplementary file 1: Figure S1. Characteristics of dated specimen. Calc-alkalic felsic granulite specimen OU78656 in hand specimen with an in situ zircon in crossed polarised light shown in the inset.
Supplementary file 2: Figure S2. Whole rock discrimination diagrams. A, Alkalic–sub-alkalic division line of Irvine & Baragar (Citation1971). B, The samples that plot in the sub-alkalic field of diagram A. All plots in the calc-alkalic field of Irvine & Baragar (Citation1971).
Supplementary file 3: Figure S3. Images of zircon and titanite grains used for dating. A, Cathodoluminescence images of zircon grains from felsic granulite sample OU78656 used in U-Pb geochronology. Red circles mark approximate areas of analysis and zircon number (z3, z11, etc.) correlating with analysis numbers in . B, Plane polarised light photographs of titanite (Ttn) from alkalic sample OU78658.
Supplementary file 4: Tables S1–S3. Table S1. Whole rock chemistry; Table S2. Mineral chemistry; Table S3. Age constraints.
Tables S1–3. Table S1. Whole rock chemistry; Table S2. Mineral chemistry; Table S3. Age constraints.
Download MS Excel (122 KB)Figure S3. Images of zircon and titanite grains used for dating.
Download PDF (614 KB)Figure S2. Whole rock discrimination diagrams.
Download PDF (20.5 KB)Figure S1. Characteristics of dated specimen. Calc-alkalic felsic granulite specimen OU78656 in hand specimen with an in situ zircon in crossed polarised light shown in the inset.
Download PDF (544.8 KB)Acknowledgements
Adam Martin was supported by an Antarctica New Zealand (New Zealand Post) Antarctic scholarship and a University of Otago award from the Department of Geology. Antarctica New Zealand is thanked for logistic support. Brent Pooley and Damion Walls are thanked for help with sample preparation and analysis. JM Palin is thanked for his assistance. Andrew Allibone and an anonymous reviewer are thanked for their helpful reviews and Richard Jongens for editorial handling.
References
- Aleinikoff JN, Wintsch RP, Fanning CM, Dorais MJ 2002. U–Pb geochronology of zircon and polygenetic titanite from the Glastonbury Complex, Connecticut, USA: an integrated SEM, EMPA, TIMS, and SHRIMP study. Chemical Geology 188: 125–147.
- Allibone AH 1988. Koettlitz group meta-sediments and intercalated orthogneisses from the mid Taylor Valley and Ferrar Glacier regions. Unpublished MSc thesis. Dunedin, University of Otago.
- Allibone AH, Cox SC, Graham IJ, Smillie RW, Johnstone RD, Ellery SG et al. 1993a. Granitoids of the Dry Valleys area, southern Victoria Land, Antarctica: plutons, field relationships, and isotopic dating. New Zealand Journal of Geology and Geophysics 36: 281–297.
- Allibone AH, Cox SC, Smillie RW 1993b. Granitoids of the Dry Valleys area, southern Victoria Land: geochemistry and evolution along the early Paleozoic Antarctic Craton margin. New Zealand Journal of Geology and Geophysics 36: 299–316.
- Allibone AH, Wysoczanski R 2002. Initiation of magmatism during the Cambrian-Ordovician Ross orogeny in southern Victoria Land, Antarctica. GSA Bulletin 114: 1007–1018.
- Atherton MP, Petford N 1993. Generation of sodium-rich magmas from newly underplated basaltic crust. Nature 362: 144–146.
- Ballard JR, Palin JM, Williams IS, Campbell IH, Faunes A 2001. Two ages of porphyry intrusion resolved for the super-giant Chuquicamata copper deposit of northern Chile by ELA-ICP-MS and SHRIMP. Geology 29: 383–386.
- Bannister S, Yu J, Leitner B, Kennett BLN 2003. Variations in crustal structure across the transition from West to East Antarctica, Southern Victoria Land. Geophysical Journal International 155: 870–884.
- Barrett PJ 1981. History of the Ross Sea region during the deposition of the Beacon Supergroup 400–180 million years ago. Journal of the Royal Society New Zealand 11: 447–458.
- Barrett PJ 1991. The Devonian to Jurassic Beacon Supergroup of the Transantarctic Mountains. In: Tingey RJ ed. The geology of Antarctica. Oxford, Clarendon. Pp. 120–152.
- Barrett PJ, Henrys SA, Bartek LR, Brancolini G, Busetti M, Davey FJ et al. 1995. Geology of the margin of the Victoria Land basin off Cape Roberts, southwest Ross Sea. In: Cooper AK, Barker PF, Brancolini G eds. Geology and seismic stratigraphy of the Antarctic margin. Washington, DC, American Geophysical Union. Pp. 183–207.
- Belousova E, Griffin W, O'Reilly S, Fisher N 2002. Igneous zircon: trace element composition as an indicator of source rock type. Contributions to Mineralogy and Petrology 143: 602–622.
- Berg JH 1991. Geology, petrology and tectonic implications of crustal xenoliths in Cenozoic volcanic rocks of southern Victoria Land. In: Thomson MRA, Crame JA, Thomson JW eds. Geological evolution of Antarctica – proceedings of the 5th International Symposium on Antarctic Earth Sciences. Cambridge, Cambridge University Press. Pp. 311–315.
- Berg JH, Moscati RJ, Herz DL 1989. A petrologic geotherm from a continental rift in Antarctica. Earth and Planetary Science Letters 93: 98–108.
- Black LP, Kamo SL, Allen CM, Aleinikoff JN, Davis DW, Korsch RJ et al. 2003. TEMORA 1: a new zircon standard for Phanerozoic U–Pb geochronology. Chemical Geology 200: 155–170.
- Borg SG, DePaolo DJ 1991. A Tectonic model of the Antarctic Gondwana margin with implications for southeastern Australia: isotopic and geochemical evidence. Tectonophysics 196: 339–358.
- Borg SG, DePaolo DJ, Smith BM 1990. Isotopic structure and tectonics of the central Transantarctic Mountains. Journal of Geophysical Research 95: 6647–6667.
- Castillo PR 2006. An overview of adakite petrogenesis. Chinese Science Bulletin 51: 257–268.
- Castillo PR, Janney PE, Solidum RU 1999. Petrology and geochemistry of Camiguin Island, southern Philippines: insights to the source of adakites and other lavas in a complex arc setting. Contributions to Mineralogy and Petrology 134: 33–51.
- Cawood PA 2005. Terra Australis Orogen: Rodinia breakup and development of the Pacific and Iapetus margins of Gondwana during the Neoproterozoic and Paleozoic. Earth-Science Reviews 69: 249–279.
- Collinson JW 1991. The paleo-Pacific margin as seen from East Antarctica. In: Thomson MRA, Crame JA, Thomson JW eds. Evolution of Antarctica. New York, Cambridge University Press. Pp. 199–204.
- Cook YA, Craw D 2001. Amalgamation of disparate crustal fragments in the Walcott Bay‐Foster Glacier area, South Victoria Land, Antarctica. New Zealand Journal of Geology and Geophysics 44: 403–416.
- Cooper AF, Maas R, Scott JM, Barber AJW 2011. Dating of volcanism and sedimentation in the Skelton Group, Transantarctic Mountains: implications for the Rodinia-Gondwana transition in southern Victoria Land, Antarctica. Geological Society of America Bulletin 123: 681–702.
- Cooper AF, Worley BA, Armstrong RA, Price RC 1997. Synorogenic alkaline and carbonatitic magmatism in the Transantarctic Mountains of South Victoria Land, Antarctica. In: Ricci CA ed. The Antarctic region: geological evolution and processes. VII International Symposium on Antarctic Earth Sciences. Siena, Terra Antartica Publication. Pp. 245–252.
- Cottle JM 2002. Evolution of a convergent plate margin: a petrological study of Ross Orogeny magmatism in the Carlyon Glacier region, Southern Victoria Land, Antarctica. Unpublished MSc thesis. Dunedin, University of Otago.
- Cottle JM, Cooper AF 2006a. Geology, geochemistry, and geochronology of an A-type granite in the Mulock Glacier area, Southern Victoria Land, Antarctica. New Zealand Journal of Geology and Geophysics 49: 191–202.
- Cottle JM, Cooper AF 2006b. The Fontaine Pluton: an early Ross Orogeny calc-alkaline gabbro from southern Victoria Land, Antarctica. New Zealand Journal of Geology and Geophysics 49: 177–189.
- Cox SC, Parkinson DL, Allibone AH, Cooper AF 2000. Isotopic character of Cambro-Ordovician plutonism, Southern Victoria Land, Antarctica. New Zealand Journal of Geology and Geophysics 43: 501–520.
- Cox SC, Turnbull IM, Isaac MJ, Townsend DB, Smith Lyttle B 2012. Geology of southern Victoria Land Antarctica. Institute of Geological and Nuclear Sciences 1:250 000 Geological Map 22. 1 sheet + 135 p. Lower Hutt, New Zealand. GNS Science.
- Defant MJ, Drummond MS 1990. Derivation of some modern arc magmas by melting of young subducted lithosphere. Nature 347: 662–665.
- Defant MJ, Jifeng X, Kepezhinskas P, Wang Q, Zhang Q, Xiao L 2002. Adakites: some variations on a theme. Acta Petrologica Sinica 18: 129–142.
- Elkins LT, Grove TL 1990. Ternary feldspar experiments and thermodynamic models. American Mineralogist 75: 544–559.
- Elliot DH 1992. Jurassic magmatism and tectonism associated with Gondwana breakup: an Antarctic perspective. In: Storey BC, Alabaster T, Pankhurst RJ eds. Magmatism and the causes of continental breakup. London, Geological Society Special Publication. Pp. 165–184.
- Encarnacion J, Grunow A 1996. Changing magmatic and tectonic styles along the paleo-Pacific margin of Gondwana and the onset of early Paleozoic magmatism in Antarctica. Tectonics 15: 1325–1341.
- Ewing T, Hermann J, Rubatto D 2013. The robustness of the Zr-in-rutile and Ti-in-zircon thermometers during high-temperature metamorphism (Ivrea-Verbano Zone, northern Italy). Contributions to Mineralogy and Petrology 165: 757–779.
- Ferry JM, Watson EB 2007. New thermodynamic models and revised calibrations for the Ti-in-zircon and Zr-in-rutile thermometers. Contributions to Mineralogy and Petrology 154: 429–437.
- Findlay RH 1985. The Granite Harbour Intrusive Complex in McMurdo Sound; progress and problems. New Zealand Antarctic Record 6: 10–12.
- Fitzgerald PG 1992. The Transantarctic Mountains of southern Victoria Land: the application of apatite fission track analysis to a rift shoulder uplift. Tectonics 11: 634–662.
- Flowers RM, Bowring SA, Tulloch AJ, Klepeis KA 2005. Tempo of burial and exhumation within the deep roots of a magmatic arc, Fiordland, New Zealand. Geology 33: 17–20.
- Fu B, Mernagh TP, Kita NT, Kemp AIS, Valley JW 2009. Distinguishing magmatic zircon from hydrothermal zircon: a case study from the Gidginbung high-sulphidation Au–Ag–(Cu) deposit, SE Australia. Chemical Geology 259: 131–142.
- Fu B, Page FZ, Cavosie A, Fournelle J, Kita N, Lackey J et al. 2008. Ti-in-zircon thermometry: applications and limitations. Contributions to Mineralogy and Petrology 156: 197–215.
- Fuhrman ML, Lindsley DH 1988. Ternary-feldspar modelling and thermometry. American Mineralogist 73: 201–215.
- Goodge JW, Fanning CM, Norman MD, Bennett VC 2012. Temporal, isotopic and spatial relations of early Paleozoic Gondwana-Margin arc magmatism, central Transantarctic Mountains, Antarctica. Journal of Petrology 53: 2027–2065.
- Goodge JW, Myrow P, Williams IS, Bowring SA 2002. Age and Provenance of the Beardmore Group, Antarctica: constraints on Rodinia supercontinent breakup. The Journal of Geology 110: 393–406.
- Gunn BM, Warren G 1962. Geology of Victoria Land between the Mawson and Mullock Glaciers, Antarctica. Bulletin of the New Zealand Geological Survey 71: 157.
- Hagen-Peter GA, Cottle JM, Tulloch AJ 2011. Exploring the petrochronology of subduction-related magmatism in the Ross Orogen: a case study from Dry Valleys, southern Victoria land, Antarctica. Minneapolis, MI, GSA. 46 p.
- Hall CE, Cooper AF, Parkinson DL 1995. Early Cambrian carbonatite in Antarctica. Journal Geological Society London 152: 721–728.
- Harrington HJ 1958. Nomenclature of rock units in the Ross Sea Region, Antarctica. Nature 182: 290.
- Heimann A, Flemming TH, Elliot DH, Foland KA 1994. A short interval of Jurassic continental flood basalt volcanism in Antarctica as demonstrated by 40Ar/39Ar geochronology. Earth and Planetary Science Letters 121: 19–41.
- Irvine TN, Baragar WRA 1971. A guide to the chemical classification of the common volcanic rocks. Canadian Journal of Earth Sciences 8: 523–548.
- Kalamarides RI, Berg JH 1991. Geochemistry and tectonic implications of lower-crustal granulites included in Cenozoic volcanic rocks of southern Victoria Land. In: Thomson MRA, Crame JA, Thomson JW eds. Geological evolution of Antarctica - proceedings of the 5th International Symposium on Antarctic Earth Sciences. Cambridge, Cambridge University Press. Pp. 305–310.
- Kalamarides RI, Berg JH, Hank RA 1987. Lateral isotopic discontinuity in the lower crust: An example from Antarctica. Science 237: 1192–1195.
- Kay SM, Ramos VA, Marquez M 1993. Evidence in Cerro Pampa volcanic rocks of slab melting prior to ridge trench collision in southern South America. Journal of Geology 101: 703–714.
- Keiller IG 1991. Wright dikes – a geochemical study of dike forming rock types within the Wright Valley, Southern Victoria Land, Antarctica. Unpublished MSc thesis. Dunedin, University of Otago. 291 p.
- Kroll H, Evangelakakis C, Voll G 1993. Two-feldspar geothermometry: a review and revision for slowly cooled rocks. Contributions to Mineralogy and Petrology 114: 510–518.
- Kuno H 1966. Lateral variation of basalt magma type across continental margins and Island Arcs. Bulletin Volcanologique 29: 195–222.
- Kyle PR, Cole JW 1974. Structural control of volcanism in the McMurdo Volcanic Group, Antarctica. Bulletin Volcanologique 38: 16–25.
- Kyle PR, Muncy HL 1989. Geology and geochronology of McMurdo Volcanic Group rocks in the vicinity of Lake Morning, McMurdo Sound, Antarctica. Antarctic Science 1: 345–350.
- LeMasurier WE 2008. Neogene extension and basin deepening in the West Antarctic rift inferred from comparisons with the East African rift and other analogs. Geology 36: 247–250.
- LeMasurier WE, Thompson J 1990. Volcanoes of the Antarctic plate and southern oceans. Antarctic Research Series 48. Washington, DC, American Geophysical Union.
- Macdonald GA, Katsura T 1964. Chemical composition of Hawaiian lavas. Journal of Petrology 5: 82–133.
- Martin AP 2009. Mount Morning, Antarctica: geochemistry, geochronology, petrology, volcanology, and oxygen fugacity of the rifted Antarctic lithosphere. Unpublished PhD thesis. Dunedin, University of Otago. 264 & appendices p.
- Martin AP, Cooper AF 2010. Post 3.9 Ma fault activity within the West Antarctic rift system: onshore evidence from Gandalf Ridge, Mount Morning eruptive centre, southern Victoria Land, Antarctica. Antarctic Science 22: 513–521.
- Martin AP, Cooper AF, Dunlap WJ 2010. Geochronology of Mount Morning, Antarctica: two-phase evolution of a long-lived trachyte-basanite-phonolite eruptive center. Bulletin of Volcanology 72: 357–371. 10.1007/s00445-009-0319-1
- Martin AP, Cooper AF, Price RC 2013. Petrogenesis of Cenozoic, alkalic volcanic lineages at Mount Morning, West Antarctica and their entrained lithospheric mantle xenoliths: lithospheric versus asthenospheric mantle sources. Geochimica et Cosmochimica Acta 122: 127–152.
- Martin AP, Cooper AF, Price RC 2014. Increased mantle heat flow with on-going rifting of the West Antarctic rift system inferred from characterisation of plagioclase peridotite in the shallow Antarctic mantle. Lithos 190–191: 173–190.
- Martin H, Smithies RH, Rapp R, Moyen JF, Champion D 2005. An overview of adakite, tonalite-trondhjemite-granodiorite (TTG), and sanukitoid: relationships and some implications for crustal evolution. Lithos 79: 1–24.
- McDonough WF, Sun S-S 1995. The composition of the Earth. Chemical Geology 120: 223–253.
- McGuiness LD, Bowen RH, Erickson JM, Alfred BJ, Kreamer JL 1985. East-West Antarctic boundary in McMurdo Sound. Tectonophysics 114: 341–356.
- Mellish SD, Cooper AF, Walker NW 2002. The Panorama Pluton: a composite gabbro-monzodiorite, early Ross Orogeny intrusion in southern Victoria Land, Antarctica. In: Gamble JA, Skinner DNB, Henrys SA eds. Antarctica at the close of a millennium. Wellington, The Royal Society of New Zealand Bulletin. Pp. 129–141.
- Middlemost EAK 1994. Naming materials in the magma/igneous rock system. Earth-Science Reviews 37: 215–224.
- Norrish K, Chappell BW 1977. X-ray fluorescence spectrometry. 2nd edition. In: Zussman J ed. Physical methods in determinative mineralogy. London, Academic Press. Pp. 207–272.
- Orihashi Y, Hirata T, Tani K, Yoshida H 2003. Rapid and simultaneous determination of multi-element abundances and U–Pb age for zircon grain using UV laser ablation ICP-MS technique: critical evaluation of the technique with 91500 zircon standard. Journal of Mineralogical and Petrological Sciences 98: 109–117.
- Paulsen H-K 2008. A lithological cross section through Mount Morning, Antarctica: a story told from xenolithic assemblages in a pyroclastic deposit. Unpublished MSc thesis (Geology). Dunedin, University of Otago. 179 p.
- Pearce JA, Harris NBW, Tindle AG 1984. Trace element discrimination diagrams for the tectonic interpretation of granitic rocks. Journal of Petrology 25: 956–983.
- Pearce NJG, Perkins WT, Westgate JA, Gorton MP, Jackson SE, Neal CR et al. 1997. A compilation of new and published major and trace element data for NIST SRM 610 and NIST SRM 612 glass reference materials. Geostandards Newsletter 21: 115–144.
- Read SE 2010. Koettlitz Glacier Alkaline province: late Neoproterozoic extensional magmatsim in southern Victoria Land, Antarctica. Unpublished thesis. Dunedin, University of Otago.
- Read SE, Cooper AF, Cottle JM, Wynyard MJ 2003. Early extension with a convergent margin, Skelton Glacier, Antarctica. Annual Antarctic Conference: programme and abstracts. Hamilton, New Zealand.
- Read SE, Cooper AF, Walker NW 2002. Geochemistry and U-Pb geochronology of the Neoproterozoic-Cambrian Koettlitz Glacier Alkaline Province, Royal Society Range, Transantarctic Mountains, Antarctica. In: Gamble JA, Skinner DNB, Henrys S eds. Antarctica at the close of a millennium. Wellington, The Royal Society of New Zealand Bulletin. Pp. 143–151.
- Rocchi S, Di Vincenzo G, Ghezzo C, Nardini I 2009. Granite-lamprophyre connection in the latest stages of the early Paleozoic Ross Orogeny (Victoria Land, Antarctica). Geological Society of America Bulletin 121: 801–819.
- Rowell AJ, Rees MN, Duebendorfer EM, Wallin ET, Van Schmus WR, Smith EI 1993. An active Neoproterozoic margin: evidence from the Skelton Glacier area, Transantarctic Mountains. Journal of the Geological Society 150: 677–682.
- Schmitz MD, Bowring SA 2001. U-Pb zircon and titanite systematics of the Fish Canyon Tuff: an assessment of high-precision U-Pb geochronology and its application to young volcanic rocks. Geochimica et Cosmochimica Acta 65: 2571–2587.
- Scott JM, Palin JM 2008. LA‐ICP‐MS U‐Pb zircon ages from Mesozoic plutonic rocks in eastern Fiordland, New Zealand. New Zealand Journal of Geology and Geophysics 51: 105–113.
- Smillie RW 1992. Suite subdivision and petrological evolution of granitoids from the Taylor Valley and Ferrar Glacier region, south Victoria Land. Antarctic Science 4: 71–87.
- Smithson SB 1972. Gravity Interpretation in the Trans-Antarctic Mountains near McMurdo Sound, Antarctica. Geological Society of America Bulletin 83: 3437–3442.
- Spencer KJ, Hacker BR, Kylander-Clark ARC, Andersen TB, Cottle JM, Stearns MA et al. 2013. Campaign-style titanite U–Pb dating by laser-ablation ICP: implications for crustal flow, phase transformations and titanite closure. Chemical Geology 341: 84–101.
- Stern CR, Kilian R 1996. Role of the subducted slab, mantle wedge and continental crust in the generation of adakites from the Austral Volcanic Zone. Contributions to Mineralogy and Petrology 123: 263–281.
- Streckeisen A 1976. To each plutonic rock its proper name. Earth-Science Reviews 12: 1–33.
- Stump E 1992. The Ross Orogen of the Transantarctic Mountains in light of the Laurentia-Gondwana split. GSA Today 2: 25–28.
- Stump E 1995. The Ross Orogen of the Transantarctic Mountains. New York, Cambridge University Press.
- Sun S-S, McDonough WF 1989. Chemical and isotopic systematics of oceanic basalts: implications for mantle composition and processes. Geological Society, London, Special Publications 42: 313–345.
- Thomas RJ, Roberts NMW, Jacobs J, Bushi AM, Horstwood MSA, Mruma A 2013. Structural and geochronological constraints on the evolution of the eastern margin of the Tanzania Craton in the Mpwapwa area, central Tanzania. Precambrian Research 224: 671–689.
- Tulloch AJ, Kimbrough DL 2003. Paired plutonic belts in convergent margins and the development of high Sr/Y magmatism: peninsular ranges batholith of Baja California and Median Batholith of New Zealand. Geological Society of America Special Papers 374: 275–295.
- Tulloch AJ, Ramezani J 2012. Immediate report: high precision U-Pb zircon ages of 5 plutons from 3 GHI suites. Techfile report ANT/594, held in the Dunedin Office. Wellington, GNS Science. 2 p.
- Turnbull IM, Allibone AH, Heron DW, Forsyth PJ 1994. Geology of the St. Johns Range, southern Victoria Land. Geologic map 14, scale 1: 50 000. Lower Hutt, Institute of Geological and Nuclear Sciences Ltd. 1 sheet + 52 p.
- Veevers JJ, Belousova EA, Saeed A, Sircombe K, Cooper AF, Read SE 2006. Pan-Gondwanaland detrital zircons from Australia analysed for Hf-isotopes and trace elements reflect an ice-covered Antarctic provenance of 700–500 Ma age, TDM of 2.0–1.0 Ga, and alkaline affinity. Earth-Science Reviews 76: 135–174.
- Wang Q, Wyman DA, Xu J, Jian P, Zhao Z, Li C et al. 2007. Early Cretaceous adakitic granites in the Northern Dabie Complex, central China: Implications for partial melting and delamination of thickened lower crust. Geochimica et Cosmochimica Acta 71: 2609–2636.
- Watson EB, Harrison TM 2005. Zircon thermometer reveals minimum melting conditions on earliest earth. Science 308: 841–844.
- Watson EB, Wark DA, Thomas JB 2006. Crystallization thermometers for zircon and rutile. Contributions to Mineralogy and Petrology 151: 413–433.
- Whalen J, Currie K, Chappell B 1987. A-type granites: geochemical characteristics, discrimination and petrogenesis. Contributions to Mineralogy and Petrology 95: 407–419.
- Whitney DL, Evans BW 2010. Abbreviations for names of rock-forming minerals. American Mineralogist 95: 185–187.
- Wilson G, Damaske D, Möller H-D, Tinto K, Jordan T 2007. The geological evolution of southern McMurdo Sound - new evidence from a high-resolution aeromagnetic survey. Geophysical Journal International 170: 93–100.
- Wilson TJ 1999. Cenozoic structural segmentation of the Transantarctic Mountains rift flank in southern Victoria Land. Global and Planetary Change 23: 105–127.
- Woolfe KJ, Barrett PJ 1995. Constraining the Devonian to Triassic tectonic evolution of the Ross Sea sector. Terra Antartica 2: 7–21.