Abstract
The first comprehensive geological map, a summary of lithologies and new radiogenic isotope data (U–Pb, Rb–Sr) are presented for crystalline rocks of the Sub-Antarctic Snares Islands/Tini Heke, 150 km south of Stewart Island. The main lithology is Snares Granite (c. 109 Ma from U–Pb dating of zircon), which intrudes Broughton Granodiorite (c. 114 Ma from U–Pb zircon) on Broughton Island. Rafts of schist within Snares Granite are common on the outlying Western Chain islets, and rare on North East and Broughton islands. Zircon grains extracted from one schistose raft on Broughton Island are prismatic and yield an essentially unimodal age population of c. 116 Ma that is within error of the granodiorite. These properties suggest that the dated raft represents a meta-igneous rock despite its mica-rich nature. Some schistose rocks on the Western Chain contain coarse relict plagioclase phenocrysts and appear to have an igneous protolith. No conclusive metasedimentary rocks have been identified, although sillimanite-bearing mica-rich schist occurs on Rua. Deformation of the crystalline rocks occurred after Snares Granite intrusion and before cooling below muscovite K–Ar closure at 400 ± 50 °C at 95 Ma. This period overlaps the age of extensional ductile shear zones on Stewart Island. The discovery of several basaltic dykes, which cut across fabrics and are unmetamorphosed, indicates that volcanic rocks are associated with all Sub-Antarctic island groups. The larger of the islands are overlain by peat, which on North East Island also contains gravel deposits.
Introduction
Despite the submerged Campbell Plateau representing c. 30% of Zealandia, very little is known about its composition. Material representing crystalline ‘basement’ rocks is restricted to samples from scattered exploration wells (Beggs et al. Citation1990), dredge hauls (Cullen Citation1975; Adams & Cullen Citation1978; Challis et al. Citation1982; Herzer et al. Citation1989; Timm et al. Citation2010) and exposures on the uninhabited and rarely visited Sub-Antarctic Antipodes, Auckland, Bounty, Campbell and Snares Islands/Tini Heke island groups (). (The name of the latter group was changed to Snares Islands/Tini Heke following the 1998 Ngāi Tahu Claims Settlement Act.) It is the island groups that provide the best insights into Campbell Plateau basement composition because they commonly expose a range of lithologies for which field relationships can be established. Although the broad composition of each island group has long been known (Marshall & Browne Citation1909; Speight & Finlayson Citation1909), detailed geological mapping has only been conducted on Campbell Island (Oliver et al. Citation1950; Beggs Citation1978; Morris Citation1984), small areas of the Auckland Islands (Wright Citation1966, Citation1967; Gamble & Adams Citation1985; Ritchie et al. Citation1985) and the Antipodes Islands (Scott et al. Citation2013).
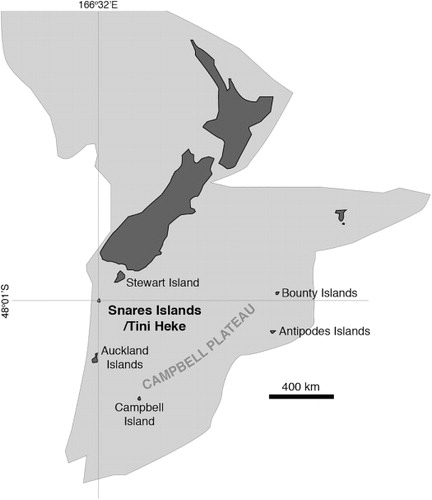
In this paper we present a geological map, a summary of lithologies, new Rb–Sr data and the first U–Pb zircon dates of rocks from the Sub-Antarctic Snares Islands/Tini Heke (). This island group, 150 km south of Stewart Island, is a World Heritage Site because of its spectacular and unmodified flora and fauna. Access to the island group can only be granted by the Department of Conservation. On two visits to the Snares in 2014, JMS and IMT mapped the main (North East) island, and made a geological examination of the second-largest island (Broughton) and several of the islets forming the Western Chain. Several new lithologies are reported, including hornblende granodiorite and basaltic dykes. Prior to the discovery of basaltic dykes, it was thought that the closest unmetamorphosed mafic volcanic-hyperabyssal rocks were those of the Auckland Islands, approximately 270 km to the south (Fleming Citation1951).
Geological background
Early geological observations found North East Island to consist of granite overlain by a thick peat blanket (Marshall & Browne Citation1909). Fleming (Citation1953), Reed (Citation1953), Watters & Fleming (Citation1975) and Denison & Coombs (Citation1977) presented field and/or petrographic descriptions of the granite, with each of these authors noting its muscovite-rich nature and variable degrees of recrystallisation. Very small mica-schist fragments collected from the top of Rua in the Western Chain were interpreted to be roof pendants of metasedimentary rock (Fleming Citation1953; Reed Citation1953; Watters & Fleming Citation1975). The granite and schist have been correlated on a petrographic basis with rocks on Stewart Island (Watters & Fleming Citation1975). Isotopic data are restricted to several whole-rock samples and muscovite separates from the main granite that yielded variable two-point muscovite-whole-rock or whole-rock/feldspar model Rb–Sr ages of 148–96 Ma and muscovite K–Ar ages of 95–92 Ma (Denison & Coombs Citation1977). These data were interpreted to show a maximum emplacement age of 170 Ma with cooling below the muscovite Ar closure temperature between c. 103 and 93 Ma (Denison & Coombs Citation1977). The pollen assemblage of the overlying peat is described in Harris (Citation1953) and McGlone (Citation2002).
Lithologies
The revised geology of Snares Islands/Tini Heke is summarised in . North East Island, Broughton Island, and the islets of Rima, Toru and Rua in the Western Chain were mapped and sampled in the course of our study. Wha and Tahi were not landed upon, but the geology of both was viewed from very close from an inflatable boat. Other outlying islets (Daption Rocks, Alert and Emerald stacks, Vancouver Rock, and several un-named stacks off Broughton Island) have not yet been visited, but observations from the sea suggest they are all granitic. Specimens collected prefixed with ‘OU’ are catalogued in the University of Otago rock collection, whereas samples with a ‘P’ prefix are housed in the GNS Science collection. Samples are lodged in the online PETLAB database (www.pet.gns.cri.nz). Lithologies are reported below in order of inferred age.
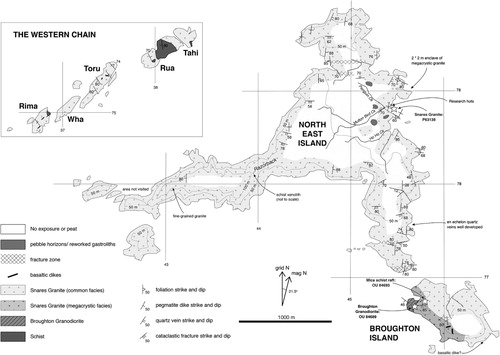
Mica schist
Numerous rafts and enclaves of muscovite- and biotite-bearing schist, ranging from banded to thinly laminated and sometimes mesoscopically folded schist to massive fine- to medium-grained mica-quartz-plagioclase rocks, occur within Snares Granite on islets of the Western Chain. Similar enclaves occur on Broughton Island within a megacrystic facies of Snares Granite. A single tiny (10 × 3 cm wide) schist enclave was found in granite on The Razorback on North East Island.
Two fragments of mica schist ‘lying on the surface near nests of Cape pigeons’ (Reed Citation1953, p. 29) on Rua were described by Reed (Citation1953) and Watters & Fleming (Citation1975). Just under half of Rua is found to be composed of mica schist (). It comprises mica-rich and mica-poor horizons that are foliated, folded, and cut by numerous Snares Granite and pegmatite dykes (). Thin-sections show the schist to be rich in biotite, muscovite and quartz. Traces of fibrolitic sillimanite occur within muscovite and quartz grains. There is very little plagioclase, and the protolith was evidently rich in K and Al but poor in Ca and Na. These rafts are tentatively inferred to be of sedimentary origin.
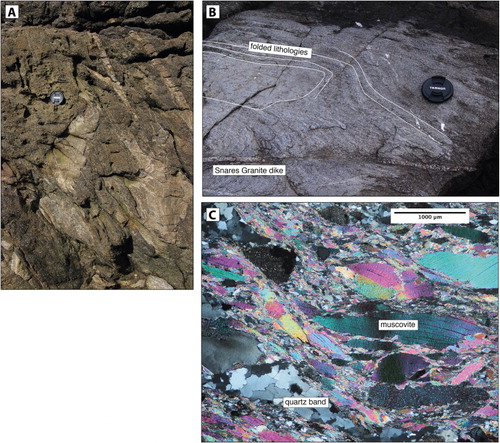
Schist inclusions also occur on Toru and Rima and can be seen in the southern cliffs of Tahi. In these cases they form rafts of c. 1–30 m length and are commonly very narrow. These schists are dark coloured, poorly laminated to massive and contain biotite, muscovite, plagioclase and quartz. Pale green amphibole occurs in one >50 m long raft on the eastern end of Rima. Some plagioclases form coarse grains that are much larger than the matrix. The coarse plagioclase grains are zoned, have dusty cores and appear to be relict igneous minerals now encapsulated in a metamorphic matrix. At first view, these elongate rafts may be metamorphosed dykes; however, they are cross-cut by Snares Granite.
On Broughton Island, the main minerals within a narrow elongate (<1 m wide by <10 m long) schist raft in megacrystic Snares Granite are muscovite, quartz and plagioclase (). Minerals present in accessory proportions are zircon and unusually coarse apatite. This sample looks similar to the mica-rich schist found on Rua, except that many grains have ‘mica fish’ habits and tiny subgrains are present on muscovite margins. Plagioclase forms porphyroclasts, whereas quartz forms polycrystalline aggregates. Quartz veins in the rock have also been recrystallised. This sample appeared to be a metamorphosed aluminous sedimentary rock, but zircon data (discussed in ‘Isotopic dating’) suggest that it may have an igneous origin.
Broughton Granodiorite
The northwest corner of Broughton Island is composed of a medium-grained brown-grey granodiorite (–). The name Broughton Granodiorite is proposed for this new lithology. Broughton Granodiorite is composed of biotite, plagioclase, quartz, K-feldspar and accessory epidote (some with allanite cores), subhedral but commonly discoidal titanite, small grains of hornblende, an opaque oxide and zircon. Biotite is the dominant mafic phase and has dark brown to pale brown pleochroism. The brown pleochroic nature of the biotite, coupled with the presence of allanite/epidote, hornblende and titanate suggests that Broughton Granite is I-type, according to the nomenclature of Chappell & White (Citation2001). Plagioclase grains are zoned and commonly have dusty cores. K-feldspar has myrmekitic margins. Quartz has been recrystallised to fine-grained polycrystalline aggregates with sawtooth margins. The degree of recrystallisation varies between samples; some have igneous textures whereas others are proto-mylonitic.
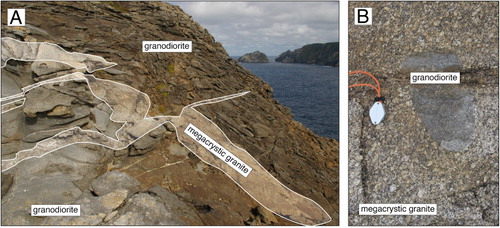
Snares Granite
The dominant lithology found in the island group is pale-coloured muscovite granite (). It occurs over the entirety of North East Island, on the east of Broughton Island and makes up much of the Western Chain. This lithology is formalised as Snares Granite. As noted by previous workers (Marshall & Browne Citation1909; Fleming Citation1953; Reed Citation1953; Watters & Fleming Citation1975), Snares Granite is extensively altered and fresh material is difficult to find. The freshest samples contain muscovite, plagioclase, K-feldspar and quartz with accessory apatite, garnet and zircon (). Snares Granite on the Western Chain also contains biotite (Watters & Fleming Citation1975), sometimes as small schlieren several centimetres in length, as well as topaz and tourmaline. The granite shows recrystallisation textures throughout the islands and is gneissic in some places, especially on Toru. The fabric is, however, subtle and commonly difficult to find in outcrop or hand specimen; consequently, few field measurements were made. On North East Island, foliation generally strikes east with variable but steep dips. Very rarely, the foliation itself has been refolded about subvertical shear planes.
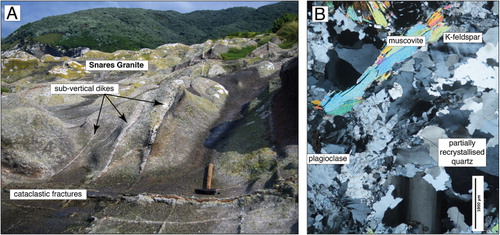
On Broughton Island, Snares Granite is megacrystic near the intrusive contact with Broughton Granodiorite and dykes of megacrystic Snares Granite intrude the granodiorite (). The megacrystic facies extends along the west coast of Broughton Island and is between 100 and 200 m wide. The facies contains distinctively coarse K-feldspar grains that reach up to 4 cm in diameter. These megacrysts have myrmekitic margins and are set within a finer matrix composed of plagioclase, biotite and quartz. A 2 × 2 m raft of porphyritic granite that occurs within Snares Granite at Station Cove on the east coast of North East Island is probably a different lithology.
A striking feature of Snares Granite is the occurrence of cross-cutting granitic dykes (). On North East Island these dykes rarely exceed 10 cm in width, but have a very consistent north-striking and steeply dipping orientation. Some are pegmatitic (composed of quartz, K-feldspar, coarse muscovite and rare garnet), whereas others are aplitic. Offsets on these dykes preserve a good record of the brittle fracturing and cataclasis that has affected North East Island (, ). Pegmatitic and aplitic dykes, often occurring together, are common on the Western Chain. Some dykes on Rua are over a metre in width.
Basaltic dykes
Two 20 cm thick unmetamorphosed basaltic dykes were discovered on cliff tops of the southwest side of Broughton Island (, ). They are both moderate to steeply dipping and intrude the megacrystic facies of Snares Granite. Another basaltic dyke was tentatively identified (from a boat) at sea level on the southeast point of Broughton Island. The cliff-top dykes are extremely altered and now largely composed of clay; however, relict euhedral feldspar laths up to c. 1 mm in length and opaque oxides set in a trachyitic matrix confirm a basaltic origin (). Alteration is too advanced to allow comparison with the volcanic pebbles described from North East Island by Reed (Citation1953). Unlike the granitoids and schists, the dykes show no sign of a metamorphic fabric and must therefore postdate deformation of the granitoids and schists.
Faults and joints
Most outcrops on the islands show some form of brittle fracturing. This is most obvious where the cataclasite-bearing fractures cut offset pegmatite/aplite dykes (). The fractures have a systematic east–west orientation and steep dip. Both sinistral and dextral apparent offsets have been observed, generally in the order of only a few centimetres, in agreement with Fleming (Citation1953). Two larger east-striking faults, through Sinkhole Gut and the Sinkhole, have gently east- and west-plunging slickensides respectively, indicating strike-slip movement although the relative offsets are unknown. Most major indentations on the coastline of North East Island, such as those on the southern promontory extending towards Broughton Island (), are fault zones and have abundant fractures. These fault zones strongly influence the shape of the islands’ coastlines.
Snares Granite is strongly jointed, with joint spacing generally c. 1–2 m but ranging down to a few centimetres locally near faults. The predominant sets trend north–south and east–west (see also Fleming Citation1953); however, joints on many other orientations are locally developed. Subhorizontal joints are also prominent. The latter are particularly well-developed on Rua and Toru on the Western Chain, at spacings of 1–2 m.
Gravel/gastrolith deposits
Fleming (Citation1951, Citation1953) described ‘scattered well-rounded water-worn pebbles’ in the bush across North East Island. These pebble deposits are widespread at about 20 m above sea level on the eastern side of North East Island in bare penguin colonies and in the Olearia forest nearby (), mainly on the tops of spurs or in flattish areas such as the head of Penguin Creek. The pebbles are predominantly locally derived Snares Granite, with rare basalt (also reported by Reed Citation1953) and even rarer diorite and thinly laminated rock that may be metasedimentary. Clasts range from sand-sized to 6–8 cm across () and range from subrounded to very well rounded. Gravel was found at only one spot on Fleming's lower terrace (see fig. 8 in Fleming Citation1953), in a penguin colony immediately north of the huts. The only modern pebble beach known on North East Island, at the mouth of Mutton Bird Creek, comprises well-sorted and well-rounded granite gravel with clasts averaging 2–3 cm in diameter.
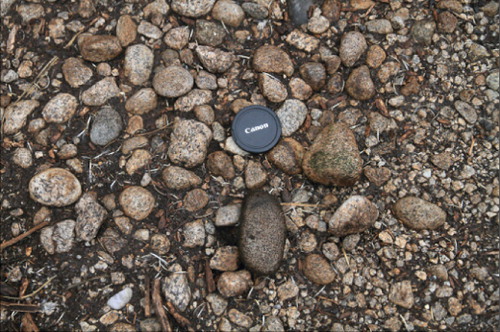
The origin of these pebbly deposits is controversial. Fleming (Citation1953) interpreted them as sea lion gastroliths, originally thinly distributed throughout the peat and since winnowed out, especially in old (and existing) penguin colonies where a lag deposit of pebbles has accumulated as the peat has eroded away. Part of Fleming's argument is based upon the occurrence of basalt cobbles that he thought must originate from the closest volcanic centre, which at the time was thought to be the Auckland Islands c. 270 km to the south. However, the discovery of basaltic dykes on Broughton Island means that a local source (perhaps not exposed at the surface) cannot be ruled out. Alternatively, the pebbles could represent uplifted beach deposits that have been redistributed into the peat. The presence of the pebble deposits at a similar height across the eastern part of North East Island supports an interpretation of them representing the relicts of an uplifted beach. Even if the pebbles are gastroliths, sea lions tend to congregate on beaches; this also supports a raised beach origin.
Isotopic dating
U–Pb zircon
Zircon grains were dated from three samples: Broughton Granodiorite, Snares Granite and a schist raft occurring in Snares Granite on Broughton Island. The analyses were conducted by laser ablation attachment on an Agilent 7500 quadrupole inductively coupled plasma mass spectrometer at the University of Otago. The method for obtaining a U–Pb zircon date is described by Scott & Palin (Citation2008).
Zircon grains in Broughton Granodiorite (OU84689) range from stubby to elongate prisms that have c. 100–300 μm long axes (). Many zircon grains have irregular-shaped cores that are surrounded by oscillatory-zoned and prismatic crystal terminations. Forty-seven dates from 45 spot analyses range from 100 to 1128 Ma (). The isotope profiles in analyses 4 and 37 could be subdivided into two separate dates; see Scott et al. (Citation2009) for an example of this workflow. Of these 45 analyses, 40 yielded dates between 101 and 128 Ma; all older dates were from the irregularly shaped zircon cores. Thirty of the Early Cretaceous dates give an error-weighted mean age of 114.2 ± 1.2 Ma (MSWD = 1.5, 2σ error), which excludes the four youngest and the two oldest Early Cretaceous dates ().
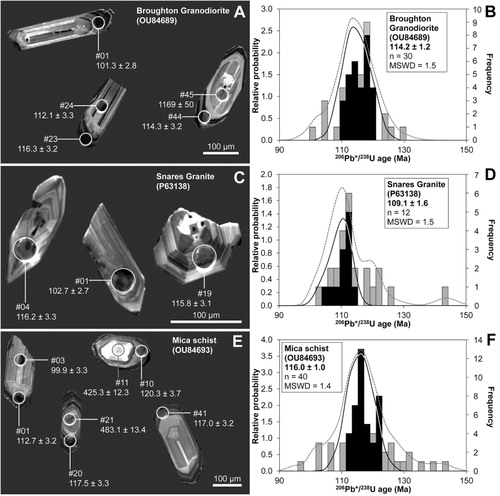
Table 1 Rb–Sr and Sm–Nd data for the Snares Granite.
The zircon grains from Snares Granite (P63138) have stubby prismatic morphologies and exhibit faint oscillatory zoning under cathodoluminescence (). Some grains have distinct cores with broad or convoluted zoning. Thirty analyses yielded 31 dates ranging from 101 to 1965 Ma (). Twenty-five of the dates are Cretaceous (101–144 Ma), of which 12 give a pooled age of 109.1 ± 1.6 Ma (MSWD = 1.5, 2σ error; ). Dates outside of this population are considered to be from inherited zircon or the result of sub-solidus Pb loss, and are not considered for the pooled age.
Table 2 Whole-rock geochemical data for Snares Granite and Broughton Granodiorite.
Most zircon grains from the mica-rich schist raft (OU84693) in Snares Granite form stubby prisms that measure c. 150–200 μm in their longest direction (). Generally well-formed zircon terminations mantle pale interiors of oscillatory zoned, homogeneous or broadly zoned cores. Sixty-seven dates were obtained from 60 analyses (Table S3). Sixty-three of the dates are Cretaceous, with four distinctly older dates. Forty analyses, which exclude the 10 youngest and 12 oldest Cretaceous dates on the basis that represent Pb loss or subtle inheritance, respectively, yield an age of 116.0 ± 1.0 Ma (MSWD = 1.5, 2σ error; ). Although this sample was initially thought to have a metasedimentary origin, the essentially unimodal Cretaceous age population, the similarity to the Broughton Granodiorite zircon data and the lack of zircon rounding suggest that the schist has an igneous protolith.
Rb–Sr and Sm–Nd whole-rock
Rb–Sr and Sm–Nd concentrations and Sr and Nd isotopic compositions were measured on a whole-rock sample of Snares Granite (P63138) from North East Island using methods similar to those described by Scott et al. (Citation2014). The resulting data, together with recalculated Sr isotope data of Denison & Coombs (Citation1977) (from samples OU11960, OU11961 and OU11966), scatter roughly between isochrons of 125 and 100 Ma (). The lack of an isochron may be the result of disturbance of the Rb–Sr system in the white micas, which are typically deformed ().
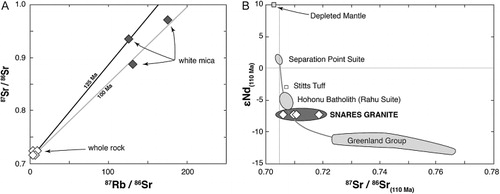
Initial isotope compositions for a single sample of Snares Granite (P63138) are 87Sr/86Sr(110Ma) = 0.71849 and εNd(110 Ma) = −7.2 (). Previously published data of Denison & Coombs (Citation1977) yields 87Sr/86Sr(110Ma) of 0.70593–0.71050. This wide range likely reflects some post-crystallisation disturbance of Rb–Sr ratios, plus error magnification as a consequence of the relatively large whole-rock 87Rb/86Sr (ranging from 3 to 9). Plotting all Sr isotope data at the single εNd(110 Ma) value of sample P63138 shows that the Snares Granite has a relatively evolved isotopic composition, suggesting the magma has either interacted with, or was derived from, a moderately radiogenic component such as continental crust ().
In comparison with other broadly contemporaneous Cretaceous granitoids from the Western Province, it can be seen that the Snares Granite has compositions similar to the 115–107 Ma Rahu Suite (Waight et al. Citation1997) but with somewhat less radiogenic Nd, and is distinct from the typically slightly older Separation Point Suite granitoids (Muir et al. Citation1997, Citation1998; ). This conclusion is supported by the similarity in zircon U–Pb ages of the Broughton Granodiorite and Snares Granite with Rahu Suite plutons found in Westland (Muir et al. Citation1997; Waight et al. Citation1997; Ritchie et al. Citation2015). However, although Snares Granite has similar major element concentrations to Rahu Suite Buckland Granite at equivalent SiO2 (), it has distinctly lower Sr and Ba and higher Y, Nb and Rb. Correlation with Rahu Suite is therefore considered tentative at best.
Discussion
Petrographic observations show that the granitoids and schists have been ductilely deformed. Many Snares Granite specimens have ductile deformation textures in which quartz forms strained polycrystalline aggregates. The schist rafts on Broughton Island contain quartz with prominent recrystallisation textures and muscovite that forms mica fish textures. The emplacement age of Snares Granite, 109 Ma, provides a maximum older time constraint on deformation, and the Rb–Sr muscovite ages of c. 103–93 Ma and muscovite K–Ar ages of 97–94 Ma provide a rough younger constraint (ages recalculated with modern decay constants from data presented in Denison & Coombs Citation1977).
Cretaceous ductile deformation is a common feature of New Zealand basement rocks and represents a time of crustal extension preceding eventual opening of the Tasman Sea at c. 85 Ma. Cretaceous crustal extension has been documented from shear zones across South Island (e.g. Gibson et al. Citation1988; Tulloch & Kimbrough Citation1989; Gray & Foster Citation2004; Scott & Cooper Citation2006), as well as on Stewart Island (Kula et al. Citation2007) 150 km to the north of Snares Islands/Tini Heke. Rahu Suite magmatism has also been linked to crustal extension (Waight et al. Citation1998). The orientation of a swarm of subvertical north-striking pegmatite dykes intruding Snares Granite on North East Island potentially supports the concept of the area having been affected by extension. These dykes are deformed and were therefore emplaced after 111 Ma and before 103–93 Ma. However, their notably similar subvertical and north-striking orientations indicate that there was a strong structural influence on their emplacement. Andersonian fault theory suggests that dykes should open perpendicular to σ3, the smallest principal stress. Assuming this to be the case, the steep orientation of the dykes means that σ3 was horizontal and implies that the regional stress regime at the time of dyke emplacement was extensional or strike-slip.
Conclusions
The first comprehensive geological map is provided for the Sub-Antarctic Snares Islands/Tini Heke. The oldest rocks are schists that crop out on parts of the Western Chain. Some of the schists are likely igneous in origin, whereas the protolith to mica-rich aluminosilicate-bearing lithologies is less clear. The schists are enveloped by c. 109 Ma muscovite-rich Snares Granite that also makes up most of North East Island and most of Broughton Island. Snares Granite on Broughton Island has a distinctive megacrystic facies that intrudes biotite-hornblende-bearing Broughton Granodiorite. Zircon dates indicate the granodiorite was emplaced at c. 114 Ma. Similarities in age and Nd isotope composition suggest tentative correlation of Snares Granite with Rahu Suite plutons elsewhere in the Western Province of New Zealand. Zircons extracted from a mica-rich schist raft in Snares Granite are prismatic and have an age spectrum that is strikingly similar to the granodiorite; they therefore may have a meta-igneous origin. Basaltic dykes intrude Snares Granite on Broughton Island. The dykes are strongly altered but retain an igneous texture. The discovery of these dykes means that all New Zealand's Sub-Antarctic island groups now contain unmetamorphosed mafic volcanic-hyperabyssal rocks. Pebbly deposits lying in the thick peat across North East Island represent lag deposits of sea lion gastroliths or are remnant raised beach deposits.
Broughton Granodiorite, Snares Granite and associated schist rafts have been deformed and contain variably developed ductile recrystallisation textures. The zircon ages coupled with published K–Ar age constraints indicate that deformation was a Cretaceous event. Time constraints indicate deformation could have been synchronous with extensional shear zones active on Stewart Island, 150 km to the north.
Supplementary data
Supplementary file 1: Table S1. Broughton Granodiorite (OU84689) U-Th-Pb zircon isotopic data.
Supplementary file 2: Table S2. Granite, North East Island (P63138) U-Th-Pb zircon isotopic data.
Supplementary file 3: Table S3. Mica schist (OU84693) U-Th-Pb zircon isotopic data.
Table S3. Mica schist (OU84693) U-Th-Pb zircon isotopic data.
Download MS Word (35.3 KB)Table S2. Granite, North East Island (P63138) U-Th-Pb zircon isotopic data.
Download MS Word (28.5 KB)Table S1. Broughton Granodiorite (OU84689) U-Th-Pb zircon isotopic data.
Download MS Word (32.4 KB)Acknowledgements
We thank Paul Sagar of NIWA for organising the two visits to the Snares Islands/Tini Heke, allocating JMS and IMT space on R/V Tiama and for sharing his vast knowledge of the islands. Henk Haazen and his Tiama crews transported us to and from the island group and ferried us comfortably onto Broughton Island and the Western Chain. The Department of Conservation permitted access and collection of material. The University of Otago Geology Department and GNS Science covered the costs of isotope analyses. Nick Mortimer's editorial comments improved the manuscript.
Associate Editor: Dr Nick Mortimer.
References
- Adams GJ, Cullen DJ 1978. Potassium-argon ages of granites and metasediments from the Bounty Islands area, southwest Pacific Ocean. Journal of the Royal Society of New Zealand 8: 127–132.
- Beggs JM 1978. Geology of the metamorphic basement and late cretaceous to Oligocene sedimentary sequence of Campbell Island, Southwest Pacific Ocean. Journal of the Royal Society of New Zealand 8: 161–177.
- Beggs JM, Challis GA, Cook RA 1990. Basement geology of the Campbell Plateau: implications for correlation of the Campbell Magnetic Anomaly System. New Zealand Journal of Geology and Geophysics 33: 401–404.
- Challis GA, Gabites J, Davey FJ 1982. Precambrian granite and manganese nodules dredged from southwestern Campbell Plateau, New Zealand. New Zealand Journal of Geology and Geophysics 25: 493–497.
- Chappell BW, White AJR 2001. Two contrasting granite types: 25 years later. Australian Journal of Earth Sciences 48: 489–499.
- Cullen DJ 1975. Autochthonous rocks of the bounty islands region, south-west Pacific ocean. New Zealand Journal of Geology and Geophysics 18: 767–785.
- Denison RE, Coombs DS 1977. Radiometric ages for some rocks from Snares and Auckland Islands, Campbell Plateau. Earth and Planetary Science Letters 34: 23–29.
- Fleming CA 1951. Sea lions as geological agents. Journal of Sedimentary Research 21: 22–25.
- Fleming CA 1953. General geology. In: Fleming CA, Reed JJ, Harris WF eds. The geology of the Snares Islands. Cape Expedition Series Bulletin 13. 42 p.
- Gamble JA, Adams CJ 1985. Volcanic geology of Carnley volcano, Auckland Islands. New Zealand Journal of Geology and Geophysics 28: 43–54.
- Gibson GM, McDougall I, Ireland TR 1988. Age constraints on metamorphism and the development of a metamorphic core complex in Fiordland, southern New Zealand. Geology 16: 405–408.
- Gray DR, Foster DA 2004. 40Ar/39Ar thermochronologic constraints on deformation, metamorphism and cooling/exhumation of a Mesozoic accretionary wedge, Otago Schist, New Zealand. Tectonophysics 385: 181–210.
- Harris WF 1953. Peat samples. In: Fleming CA, Reed JJ, Harris WF eds. The geology of the Snares Islands. Cape Expedition Series Bulletin 13. 42 p.
- Herzer RH, Challis GA, Christie RHK, Scott GH, Watters WA 1989. The Urry Knolls, late Neogene alkaline basalt extrusives, southwestern Chatham rise. Journal of the Royal Society of New Zealand 19: 181–193.
- Kula J, Tulloch A, Spell TL, Wells ML 2007. Two-stage rifting of Zealandia-Australia-Antarctica: evidence from 40Ar/39Ar thermochronometry of the Sisters shear zone, Stewart Island, New Zealand. Geology 35: 411–414.
- Marshall P, Browne R 1909. The geology of Campbell Island and the Snares. In: Charles C ed. The Subantarctic Islands of New Zealand. Vol. 2. Christchurch, Philosophical Institute of Canterbury. Pp. 680–701.
- McGlone MS 2002. The Late Quaternary peat, vegetation and climate history of the Southern Oceanic Islands of New Zealand. Quaternary Science Reviews 21: 683–707.Morris PA 1984. Petrology of the Campbell Island volcanics, southwest Pacific Ocean. Journal of Volcanology and Geothermal Research 21: 119–148.
- Muir RJ, Ireland TR, Weaver SD, Bradshaw JD, Waight TE, Jongens R et al. 1997. SHRIMP U-Pb geochronology of Cretaceous magmatism in northwest Nelson-Westland, South Island, New Zealand. New Zealand Journal of Geology and Geophysics 40: 453–463.
- Muir RJ, Ireland TR, Weaver SD, Bradshaw JD, Evans JA, Eby GN et al. 1998. Geochronology and geochemistry of a Mesozoic magmatic arc system, Fiordland, New Zealand. Journal of the Geological Society 155: 1037–1053.
- Oliver RL, Finlay HJ, Fleming CA 1950. The geology of Campbell Island. Cape Expedition Series Bulletin 3. Wellington, Department of Scientific & Industrial Research. 62 p.
- Reed JJ 1953. Petrology. In: Fleming CA, Reed JJ, Harris WF eds. The geology of the Snares Islands. Cape Expedition Series Bulletin 13. Pp. 28–32.
- Ritchie DD, Turnbull IM, Beu AG, Scott GH 1985. Cenozoic sedimentary rocks at Carnley Harbour, Auckland Islands, Campbell Plateau. New Zealand Journal of Geology and Geophysics 28: 23–41.
- Ritchie TW, Scott JM, Muhling JR, Kennedy AK 2015. Cretaceous metamorphism, magmatism and shearing in the Waipuna Valley, directly south of the Reefton Goldfield. New Zealand Journal of Geology and Geophysics. doi:10.1080/00288306.2014.992444
- Scott JM, Cooper AF 2006. Early Cretaceous extensional exhumation of the lower crust of a magmatic arc: evidence from the Mount Irene Shear Zone, Fiordland, New Zealand. Tectonics 25: 15.
- Scott JM, Palin JM 2008. LA-ICP-MS U-Pb zircon ages from Mesozoic plutonic rocks in eastern Fiordland, New Zealand. New Zealand Journal of Geology and Geophysics 51: 105–113.
- Scott JM, Palin JM, Cooper AF, Fagereng Å, King RP 2009. Polymetamorphism, zircon growth and retention of early assemblages through the dynamic evolution of a continental arc in Fiordland, New Zealand. Journal of Metamorphic Geology 27: 281–294.
- Scott JM, Turnbull IM, Auer A, Palin JM 2013. The sub-Antarctic Antipodes Volcano: a < 0.5 Ma HIMU-like Surtseyan volcanic outpost on the edge of the Campbell Plateau, New Zealand. New Zealand Journal of Geology and Geophysics 56: 134–153.
- Scott JM, Waight TE, van der Meer QHA, Palin JM, Cooper AF, Muenker C 2014. Metasomatized ancient lithospheric mantle beneath the young Zealandia microcontinent and its role in HIMU-like intraplate magmatism. Geochemistry, Geophysics, Geosystems 15. 10.1002/2014GC005300
- Speight R, Finlayson AM 1909. Physiography and geology of the Auckland, Bounty, and Antipodes Islands. In: Chilton C ed. The Subantarctic Islands of New Zealand. Vol. 2. Wellington, Government Printer. Pp. 705–744.
- Timm C, Hoernle K, Werner R, Hauff F, van den Bogaard P, White J et al. 2010. Temporal and geochemical evolution of the Cenozoic intraplate volcanism of Zealandia. Earth-Science Reviews 98: 38–64.
- Tulloch AJ, Kimbrough DL 1989. The Paparoa metamorphic core complex, New Zealand: cretaceous extension associated with fragmentation of the pacific margin of Gondwana. Tectonics 8: 1217–1234.
- Tulloch AJ, Ramezani J, Mortimer N, Mortensen J, van den Bogaard P, Maas R 2009. Cretaceous felsic volcanism in New Zealand and Lord Howe Rise (Zealandia) as a precursor to final Gondwana break-up. London. Geological Society, Special Publications 321: 89–118.
- Waight TE, Weaver SD, Ireland TR, Maas R, Muir RJ, Shelley D 1997. Field characteristics, petrography, and geochronology of the Hohonu Batholith and the adjacent Granite Hill Complex, North Westland, New Zealand. New Zealand Journal of Geology and Geophysics 40: 1–17.
- Waight TE, Weaver SD, Muir RJ, Maas R, Eby GN 1998. The Hohonu Batholith of North Westland, New Zealand: granitoid compositions controlled by source H2O contents and generated during tectonic transition. Contributions to Mineralogy and Petrology 130: 225–239.
- Watters WA, Fleming CA 1975. Petrography of rocks from the Western Chain of the Snares Islands (Note). New Zealand Journal of Geology and Geophysics 18: 491–499.
- Wright JB 1966. Contributions to volcanic succession and petrology of Auckland Islands, New Zealand. I. West coast section through Ross Volcano. Transactions of the Royal Society of New Zealand 3: 215–229.
- Wright JB 1967. Contributions to volcanic succession and petrology of Auckland Islands. 2. Upper parts of Ross Volcano. Transactions of the Royal Society of New Zealand 5: 71–87.