Abstract
Detrital zircon ages are presented for 34 early Palaeozoic sandstones from Buller and Takaka terranes, New Zealand, and formerly adjacent parts of Australia–Antarctica. The Buller–Takaka datasets always have two major groups: Ordovician–late Neoproterozoic, 444–700 Ma (but mainly 540–700 Ma), termed ‘Gondwana Assembly’ (GA), and early Neoproterozoic–Mesoproterozoic, 700–1600 Ma (but mainly 900–1200 Ma), termed ‘Rodinia Assembly’ (RA). In both terranes, significant age components within these groups are strikingly similar and also have RA/GA ratios, 0.6–1.8. The Cambrian volcanic arc of the Takaka Terrane has contributed little to the zircon patterns. Proportions of Late Cambrian–Early Ordovician zircons, characteristic of granitoid sources in the Ross–Delamerian Orogen are low. The zircons are predominantly reworked with contemporary zircons only evident in a few Buller datasets. The zircon patterns suggest that two major sources (late Mesoproterozoic and late Neoproterozoic), enduring over 120 Ma, were widely distributed and it is postulated they form Precambrian basement beneath southern Zealandia.
Introduction
The Zealandia continent, comprising New Zealand and offshore plateaux (), was created at the Late Cretaceous (c. 83 Ma) Gondwana breakup, before which it formed part of the eastern margin of Gondwana. It comprises a Western Province of Cambrian–Devonian sedimentary/volcanic rocks intruded by Devonian–Carboniferous and Jurassic–Cretaceous granitoids, and an Eastern Province of Permian–Cretaceous terranes (Bishop et al. Citation1985). The early Palaeozoic rocks have equivalents in southeastern Australia (Victoria, Tasmania) and Northern Victoria Land and Marie Byrd Land, Antarctica (Bradshaw et al. Citation1983, Citation1997, Cooper & Tulloch Citation1992).
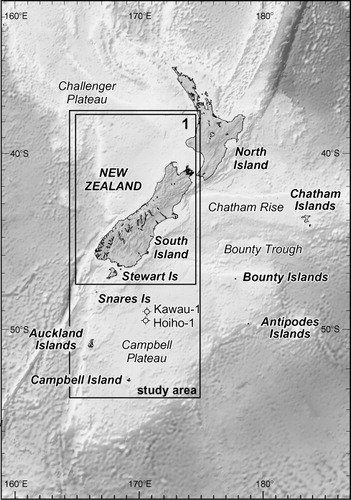
All early Palaeozoic rocks in Zealandia contain significant proportions of Precambrian zircons originating within the Rodinia Supercontinent (c. 700–1600 Ma). In Rodinia, Australia–Antarctic connections are well established but at the eastern margin, the position of a Laurentian block, as in the SWEAT versus AUSWUS models, is less certain (Dalziel Citation1991; Karlstrom et al. Citation1999; Burrett & Berry Citation2000). In South Australia, Rodinia breakup commenced at c. 700–650 Ma, with rifting and deposition of Late Neoproterozoic successions (Preiss Citation2002; Li et al. Citation2008).
In this study, 30 new detrital zircon age patterns from Buller and Takaka terranes of New Zealand, and four from Australia and Antarctica, are used to: (1) test the above correlations between Zealandia and Australia–Antarctica, (2) evaluate features that might be ‘endemic’ to Zealandia, and (3) explore its possible origins within the Rodinia Supercontinent.
Geological outline
The New Zealand Western Province is considered a correlative of the Lachlan Fold Belt of southeastern Australia and continues across the Campbell Plateau into Marie Byrd Land (Bradshaw et al. Citation1983; Adams Citation1986; Beggs et al. Citation1990; Bradshaw et al. Citation1997). The metasedimentary parts of the Western Province comprise Early Palaeozoic rocks which are subdivided into Buller and Takaka terranes (Cooper Citation1989; Cooper & Tulloch Citation1992) (). Recent 1 : 250 000 geological map compilations (Rattenbury et al. Citation1998, Citation2010, Nathan et al. Citation2002, Turnbull et al. Citation2010) provide a comprehensive overview of the stratigraphic nomenclature used here ().
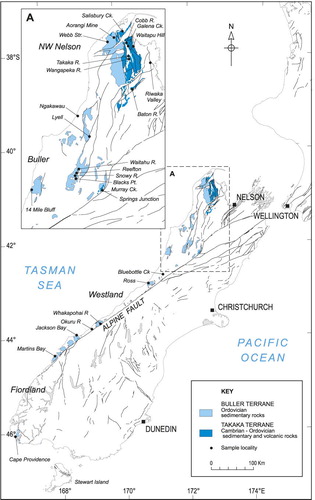
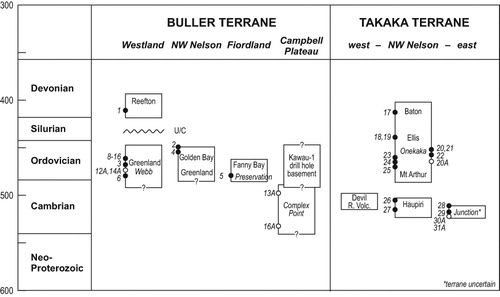
Buller Terrane
The most areally extensive unit within the Buller Terrane is the Greenland Group. It occurs in discontinuous outcrops through Westland and Nelson. The terrane can be traced in South Island, New Zealand (and offshore) for at least 400 km along strike. Early Ordovician (Lancefieldian) graptolites occur at Waitahu River (; Cooper Citation1974). In northwest Nelson, Greenland Group is correlated eastwards with Roaring Lion and Webb Formations. In turn, these pass up into Middle Ordovician, Aorangi Mine Formation of the Golden Bay Group (Cooper Citation1989; Rattenbury et al. Citation1998). In the far south, from near Martins Bay (), siltstones have calcareous concretions and thin (< 1 m) impure marbles (Mutch Citation1964).
The Greenland Group rocks are tightly folded with axial-plane spaced cleavage in sandstones and slaty-cleavage in mudstones. Regional metamorphism is low-greenschist facies (Roser & Nathan Citation1997) but locally, amphibolite facies (Mortimer et al. Citation2013). This is occasionally overprinted by static metamorphism within thermal aureoles of Devonian–Carboniferous and Cretaceous granitoids. K–Ar and Rb–Sr slate ages often reflect this overprint but, in South Westland, c. 440 Ma ages possibly indicate a minimum Late Ordovician age for the earlier regional metamorphism (Adams et al. Citation1975; Adams Citation2004).
Greenland Group rocks are proximal- to mid-fan sandstone-dominated turbidites (Laird Citation1972; Laird & Shelley Citation1974), with 1–5 m graded beds of medium-grained sandstones and siltstones (in places slightly calcareous), and minor slates. Sediment transport directions are from south/southeast to north/northwest (Laird Citation1972). Point-count studies for sandstones (Mortimer Citation2009) indicate lithic, and only moderately quartzose, quartz–arenite compositions (average Q : F : L = 58 : 16 : 26). Lithic–volcanic and lithic–metamorphic clast compositions and vein quartz grains suggest a low-grade metamorphic source environment, while modest K-feldspar contents (< 4%) could be derived from granitoid detritus. The petrographic and bulk-rock geochemical characteristics together indicate a recycled-orogen source at a passive margin (Nathan Citation1976, Roser et al. Citation1996, Mortimer Citation2009).
40Ar/39Ar ages of detrital muscovite and U–Pb SHRIMP ages of detrital zircon have ranges of 470–505 Ma and 470–700 Ma, respectively (Ireland Citation1992a; Adams & Kelley Citation1998; Ireland & Gibson Citation1998). These authors suggested derivations from granitoids in the Ross–Delamerian Orogen of Antarctica–Australia, but regarded minor 1000–1200 Ma zircons as of unknown provenance.
Buller Terrane rocks are also present in Fiordland where graptolitic Early–Middle Ordovician rocks (Fanny Bay Group) occur near Cape Providence in Fiordland (Benson & Keble Citation1935, Turnbull et al. Citation2010) (). Semi-schists resembling Greenland Group metagreywacke occur as basement in the Hoiho-1 and Kawau-1 drillholes (Hunt International Petroleum Citation1977, Citation1978; Cook et al. Citation1999) (). Seafloor samples near the Bounty Islands are quartzose metagreywackes with petrographic, detrital zircon age and Sr-isotope characteristics similar to Greenland Group (Cullen Citation1975; Adams & Cullen Citation1978; Adams Citation2008) (). On Campbell Island, one outcrop of quartzofeldspathic schist (Complex Point Formation, Oliver Citation1950) has detrital zircon age components as young as Early Ordovician. Another has an Early Cambrian Rb–Sr metamorphic age and detrital zircon age components no younger than latest Neoproterozoic, c. 550 Ma (Adams et al. Citation1979, Citation2013, Adams Citation2008).
The Greenland Group has been correlated with Ordovician Robertson Bay Group of Northern Victoria Land and Swanson Formation of Marie Byrd Land (Bradshaw et al. Citation1983; Adams & Ireland Citation2007). The uniform sedimentary style and lithological content of these and other depocentres over a large sector of eastern Gondwana suggest passive margin environments where sediments are derived from continent-scale hinterlands.
Most of the Buller Terrane and its correlatives are of demonstrable or presumed Ordovician age. However, near Reefton, small outliers of quartzites, mudstones and limestones have Early Devonian (Emsian) faunas (Bradshaw & Hegan Citation1983; Bradshaw Citation1995). These successions of Buller Terrane are less deformed than Ordovician rocks and are of shallow-water facies.
Takaka Terrane
The Takaka Terrane is lithologically more varied than the Buller Terrane and structurally complicated by dismemberment into 13 tectonic slices (Münker & Cooper Citation1995, Citation1999; Rattenbury et al. Citation1998; Münker & Crawford Citation2000).
The oldest formation (Junction Formation) is a Middle Cambrian accretionary complex whose terrane attribution is uncertain (Rattenbury et al. Citation1998; Münker & Cooper Citation1999; Fowler Citation2000; Jongens et al. Citation2003).
Early back-arc successions of Mataki Volcanics and Haupiri Group volcaniclastic sedimentary rocks precede a Middle–Late Cambrian magmatic arc with both volcanic (Benson Volcanics) and plutonic rocks (Cobb Igneous Complex) (Münker & Cooper Citation1999). Within the Haupiri Group are sparsely fossiliferous conglomerates (Salisbury, Christmas, Lockett and Dead Goat conglomerates), greywackes and limestone olistoliths (Rattenbury et al. Citation1998; Münker & Cooper Citation1999; Gutjahr et al. Citation2006). Indications of sediment transport directions are rare but within the Lockett Conglomerate these are from east to west (Pound Citation1993). A Late Cambrian diapiric melange (Balloon Melange) occurs within the volcanic arc, with Junction Formation forming its main parent matrix (Jongens et al. Citation2003). The tectonic slicing of the terrane renders the subduction-polarity of the magmatic arc uncertain (Münker & Cooper Citation1999).
The Cambrian active margin environment was progressively replaced with shallower water, Late Cambrian–Early Ordovician formations (Anatoki, Patriarch) and continued through the Ordovician with passive-margin turbidites (Wangapeka Formation) and major limestones (Arthur Marble). In the Silurian, significant shallow-water quartzites (Hailes Quartzite) occur. At Baton River (), the youngest successions are Early Devonian mudstones (Baton Formation) with minor limestone, sandstone and conglomerate (Willis Citation1965; Bradshaw Citation2000). Amalgamation of the Buller and Takaka terranes took place along the Anatoki Fault and occurred in the mid Palaeozoic either before or during emplacement of Late Devonian–Early Carboniferous granitoids (Jongens Citation2006, Tulloch et al. Citation2009).
Technical details
Coarse sandstones and quartzites were chosen for detrital zircon study and reduced to gravel size at the outcrop. A sample (200–400 g) was crushed for a few seconds in a tungsten carbide mill to < 300 µm size. Mud-size components were removed in water and a heavy mineral concentrate separated in sodium polytungstate liquid at SG 2.98. About 200 zircon grains were then randomly handpicked, and 100 were mounted and polished for U–Pb analysis. Zircon grains, 40–150 µm, are mostly euhedral or, less commonly, moderately abraded. Rounded grains are rare.
U–Pb zircon ages were determined on Agilent 7500 and 7700 LA-ICPMS instruments using 212 nM lasers (40 µm spot size, 5 Hz and approximate power 5 J/cm2 power) at the Australian Research Council (ARC) National Key Centre of Geochemical Evolution and Metallogeny of Continents (GEMOC), Macquarie University, Sydney; techniques (calibration, standardisation, data treatment) are described by Jackson et al. Citation(200). Principal calibration was with GJ zircon standard and secondary Mud Tank (MT) and 91 500 zircon standards were run repeatedly (hourly) during the analysis period. The detrital zircon ages were determined close to crystal terminations and do not include analyses of crystal cores.
U–Pb isotopic ratios and 207Pb/206Pb, 207Pb/235U and 206Pb/238U ages are tabulated in Supplementary file 1 (SF1) with probability density/histogram diagrams of 206Pb/238U ages generated using ISOPLOT 3 software (Ludwig Citation2003). Common-Pb corrections were made following Andersen (Citation2002) but were usually insignificant. Significant zircon age components were derived from probability density diagrams using limiting criteria that all zircons included: (1) had to have concordant 207Pb/235U and 206Pb/238U ages, (2) form an overlapping group of four or more zircon ages at 95% confidence limits, and (3) comprise ≥ 4% total. These 206Pb/238U age components were mostly calculated as weighted averages, but where components overlapped groups were deconvoluted using the ISOPLOT 3 ‘Unmix Ages’ subroutine. Sample U–Pb zircon age data (as ‘accepted sets’) and calculated significant zircon 206Pb/238U age components are tabulated in SF1, and the latter summarised in .
Table 1 Sample details: Buller and Takaka Terranes, South Island, New Zealand, southeastern Australia and Antarctica (Marie Byrd Land and North Victoria Land).
Results
General patterns of total zircon datasets
Probability–density diagrams of amalgamated zircon age datasets are shown in for Buller Terrane (17 localities, 1053 zircons) and Takaka Terrane (13 localities 874 zircons). In both terranes, the great majority of detrital zircon ages (> 90%) are < 1200 Ma and form two major groups: (1) latest Neoproterozoic–Cambrian–Ordovician, 700–450 Ma (informally termed GA, Gondwana assembly); and (2) Mesoproterozoic–Neoproterozoic, 1600–700 Ma (informally termed RA, Rodinia assembly). The frequency minimum at c. 700 Ma used for this definition coincides with the commencement of Rodinia breakup (Li et al. Citation2008). The Buller Terrane RA/GA ratio mode, 1.02, is greater than the Takaka Terrane ratio, 0.82, and is a common (but not exclusive) feature of individual sample datasets (see below). In both terranes, these major groups have broadly similar age maxima/minima (, 0–1200 Ma figures).
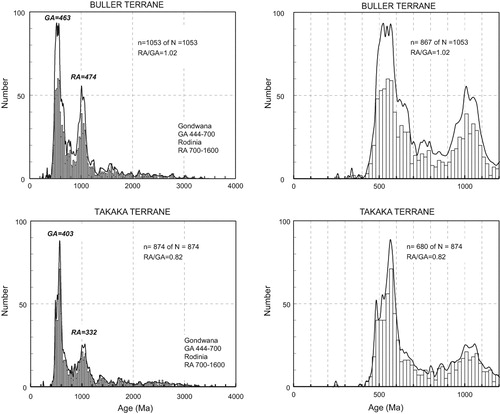
In , all zircon ages carry equal weight and are subdivided into selected geological intervals. The datasets are stacked in order of maximum stratigraphic age of the sample, as indicated by fossil control or, where unavailable, by the youngest zircon component or, in a few cases, the youngest individual zircon ages (). The zircon percentages display major RA and GA groups, with proportions in the range 30–70%. The ranges of RA/GA ratios in both terranes are broadly similar, 0.6–1.8 (). Because 1000–1200 Ma zircon proportions are often higher in the Buller Terrane (> 20%), the distribution of its RA/GA ratios is slightly skewed towards the upper part, 0.8–1.8, whereas the Takaka Terrane ratios are distributed mostly in the lower part, 0.7–1.1. The GA group is commonly dominated by zircons in the late Neoproterozoic, 542–700 Ma, interval ().
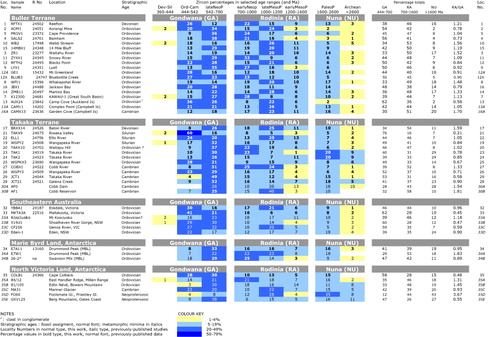
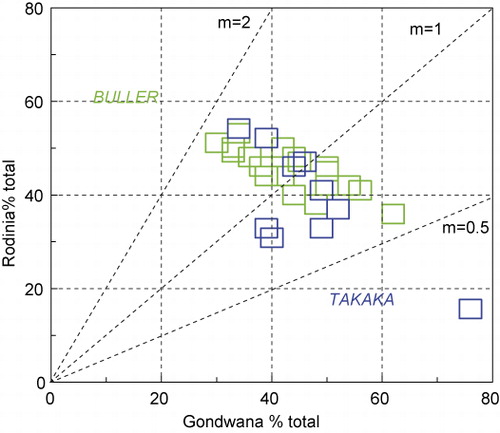
Both terranes have significant Palaeoproterozoic and Archean zircon proportions, up to 20% and 29%, respectively (, informally termed Nuna group, NU). The oldest zircons are Palaeoarchean (3200–3600 Ma). The NU/GA ratio is usually < 0.4 but an interesting extreme occurs in samples TAK1 and TAK2 (Ordovician, Takaka Terrane) with Archean proportions reaching 9% (). Although no Precambrian rocks (whether magmatic, metamorphic or sedimentary) are known in Zealandia, it should be noted that > 80% of Buller–Takaka terrane zircons are Precambrian. Even in the GA group, Precambrian (late Neoproterozoic) zircons predominate (mostly > 25%) and only a small proportion (often < 15%) fall in the Cambrian–Ordovician interval.
Variations between individual Buller–Takaka terrane datasets
In , selected typical Buller Terrane zircon patterns are from Middle and Early Ordovician fossil localities (top and middle rows) and unfossiliferous western localities, probable Early Ordovician. In the Takaka Terrane diagram (), the selection is of mostly fossiliferous formations: Silurian (top), Ordovician (middle) and Cambrian (bottom). In the Buller datasets, the RA/GA ratios vary slightly: < 1 at the Late Ordovician fossil localities (AOM1, SALS2) to > 1 in the Early Ordovician fossil localities (ZR1, PROV1). From younger to older samples, there is a small increase in ‘Nuna’ (> 1600 Ma) ages. In Fiordland and on the Campbell Plateau, the zircon age patterns of PROV1 (SW Fiordland Fanny Bay Group), CAM13 (Campbell Island) and K12300 (Kawau-1 drillhole) are similar to Buller Terrane patterns. In the Takaka Terrane datasets (), no geographic trends can be ascertained. Although representing a broader, Late Cambrian–Silurian timespan, the range of RA/GA ratios, 0.7–1.3, is similar to Buller Terrane values. An exception is the northeasternmost sample (TAKX9) in Onekaka Schist (Grindley Citation1971), which is almost unimodal, with a very low RA/GA ratio, 0.2. Also, the Late Ordovician Wangapeka Formation quartzites (TAK1, 2) have very high proportions of ‘Nuna’ zircons (up to 29% > 2000 Ma with the oldest > 3500 Ma).
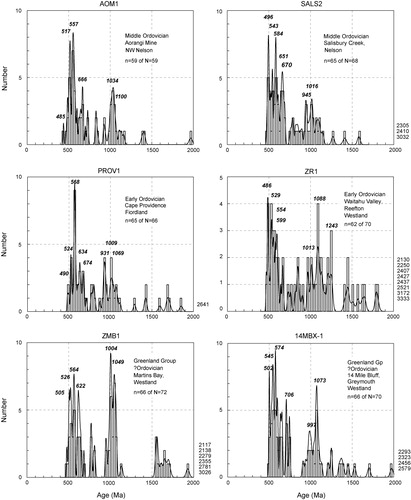
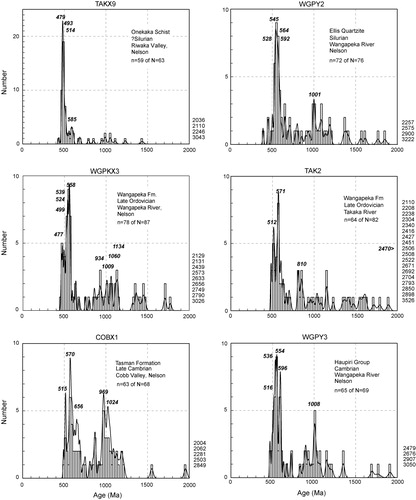
Taken together, the Buller and Takaka terrane data show the same persistent RA and GA groupings without any consistently distinctive feature (e.g. RA/GA ratios, or age maxima) attributable to either terrane.
Middle Cambrian Junction Formation datasets (JCT1 and JCT21) are shown separately in . The youngest age components for JCT1 and JCT21, 555 ± 5 Ma and 541 ± 7 Ma respectively, establish Early Cambrian maximum depositional ages. Although the age patterns are similar to both Buller and Takaka terranes (–), their respective RA/GA ratios 0.6 and 0.9, are perhaps more typical of the Takaka Terrane ().
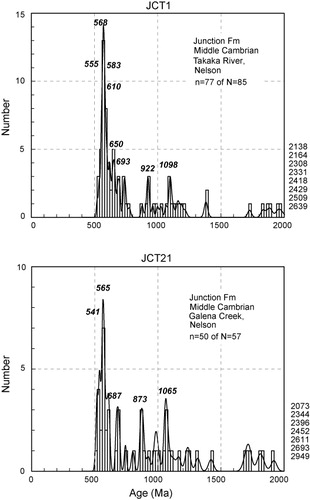
Detrital zircon age data are shown in for Early Devonian sandstones: (1) Reefton Group (RFTX1) in the Buller Terrane, and (2) Baton Group (BRXX14) in the Takaka Terrane. RFTX1 is a quartzite whose zircon age pattern is similar to Buller Terrane basement nearby (cf. dataset RFTH2). However, there is a distinctive, youngest age component at 453 ± 5 Ma (latest Ordovician–Early Silurian). Sample BRXX14 represents the youngest part of a continuous Takaka succession from the Early Ordovician and its RA/GA ratio, 1.6, is at the upper limit of the Takaka Terrane range.
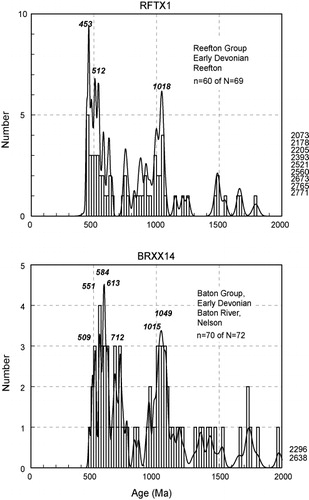
Zircon age patterns of Australia–Antarctica correlatives
To compare the Buller–Takaka data with adjacent areas of Gondwana, new detrital zircon age data are shown in for four sandstones from Antarctica and southeast Australia. The Australian samples are late Early–Middle Ordovician fossil localities at Eskdale, Victoria (YBBA1) (Kilpatrick & Fleming Citation1980) and Mallakoota (MKTA4) but the Antarctic samples (COLB1, E7A11), from unfossiliferous localities, are of presumed Early Ordovician age. Technical procedures followed those of New Zealand samples and allow confident comparison. Their RA/GA ratios, MKTA4 = 0.45, YBBA = 0.96, E7A11 = 0.95, COLB1 = 0.48 are in the lower part of the Buller–Takaka range.
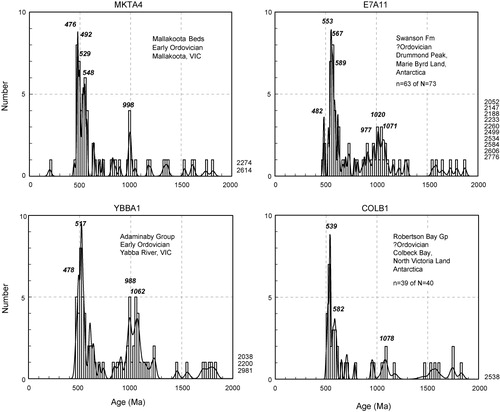
Significant age components in individual sample datasets
Within the Buller–Takaka datasets, the youngest significant age components set maximum depositional ages. Strictly speaking, the youngest individual detrital zircon age in each sample dataset provides this maximum depositional age. However, in rare cases where an individual zircon age is younger than a plausible depositional age, these are anomalous and unless a substantial metamorphic overprint is suspected, there has been unexplained Pb loss (WB2, and ZYXX1, SF1). It is preferable to take a conservative approach and rely on youngest significant age components (as defined in ‘Technical details’ and ) to supply a maximum depositional age. For individual Buller Terrane samples these range between 480 and 530 Ma (excluding Devonian sample RFTX1) with the majority 480–500 Ma (Late Cambrian–earliest Ordovician). Where Early–Middle Ordovician fossil age control is available (e.g. AOM1, SALS2, PROV1), the youngest age components are significantly older (Late Cambrian) than depositional age. Only sample ZR1 (Early Ordovician fossil locality) has the youngest zircon component, 486 ± 6 Ma, concordant with the depositional age, although this is possibly the case in samples LYX1, WB2, ZYXX1 and RFTH2 (all without fossil age control).
Table 2 Detrital zircon U–Pb significant age components: Buller and Takaka Terranes, Southern Zealandia.
In the Takaka Terrane datasets, the majority of samples have Cambrian to Devonian fossil age control and the youngest significant age components, mostly 510–555 Ma, are commonly older than indicated depositional ages. However, unlike the Buller Terrane, there are occasionally a few (3–5) individual ages that are younger than the interpreted fossil age, e.g. Middle Cambrian JCT1, three ages 521–538 Ma, JCT21, three ages 511–522 Ma; Late Cambrian WGPY3, five ages 467–501 Ma; and Early Ordovician WGPKX3, three ages 458–465 Ma (see SF1).
All the significant age components are summarised in , which also includes previously published detrital zircon ages from New Zealand, southeast Australia, North Victoria Land (East Antarctica) and Marie Byrd Land (West Antarctica) recalculated with similar protocols (Supplementary file 2, SF2). On the vertical axis, the datasets are in order of maximum stratigraphic age. The vertical heights of individual databoxes reflect the proportion (% total) of a zircon component and tall databoxes thus reflect components with most individual age values (indicated on the horizontal axis) and best precision (width of databoxes; the wider they are the less precision). In both Buller and Takaka terrane datasets, the 444–700 Ma and 900–1200 Ma significant age components are clearly visible.
![Figure 12 Detrital zircon age data patterns in sandstones and quartzites from A, early Palaeozoic, Buller and Takaka Terranes, New Zealand; and B, correlatives in adjacent eastern Australia and Antarctica. Significant detrital zircon 238U/ 206Pb age components are derived from probability diagrams (tabulated in Table 2 and Supplementary file 2 [Table S2]) and individual sample data are stacked vertically from top to bottom in order of ascending maximum biostratigraphic age or, where uncertain, the youngest significant zircon age component. Each data box represents a significant zircon age component of n ≥ 4 analyses and ≥ 4% of total dataset (usually N = 50–100), whose position and width on the horizontal axis represent the component age and uncertainty, and whose height on the vertical axis shows the proportion of that component as a percentage of the total dataset (see 0–50% scale bar at right).](/cms/asset/1aa0d2ee-474f-46e5-8f6c-2190867652ab/tnzg_a_1025798_f0012_c.jpg)
Frequency of significant age components
The broad groups of significant age components show a considerable fine structure (). In Buller and Takaka terrane datasets, the frequency of particular age components is strikingly similar. For example, in the Buller Terrane: RA group c. 1011 and 1060 Ma and GA group c. 500, 522, 558 and 586 Ma; and in the Takaka Terrane: RA group c. 1012, 1059 Ma and GA group c. 513, 556 and 585 Ma. The Buller Terrane has eight age components in the Late Cambrian–Early Ordovician interval, whereas the Takaka Terrane has only four. Although derived from a smaller database (15 in total), the Australia/Antarctica datasets () also have their most frequent significant age components in the RA group c. 1067 Ma and the GA group at c. 479, 495, 553 and 590 Ma.
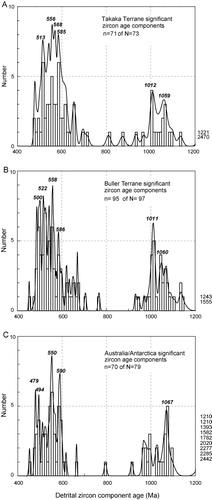
Campbell Plateau
The last piece of new data in this article is an age for granodiorite S100A (R6365), dredged from the southwesternmost edge of the Campbell Plateau. This is important because if in situ, it is a potential source for late Mesoproterozoic zircons in Buller–Takaka rocks. A new zircon age, 1170 ± 9 Ma () confirms previous K–Ar dating (Challis et al. Citation1982).
Discussion
The Buller–Takaka terrane detrital zircon data are always dominated by the RA and GA age groups in substantial proportions, > 30%, a pattern repeated extensively across present-day southern Zealandia and enduring for > 100 Ma (Middle Cambrian to Early Devonian). To supply extensive depocentres with RA and GA detritus for this length of time, the source areas must have had sufficient magnitude (areal extent and depth/volume), for example, craton-size igneous/metamorphic complexes and/or Cordilleran-size granitoid batholiths. Variations in distribution and exhumation would explain local changes in RA/GA ratios (). Reworking of zircons throughout early Palaeozoic deposition, from older into younger levels, is also possible, effectively maintaining the RA/GA patterns. However, if juvenile zircon sources were introduced during deposition, then one might expect progressively greater proportions of younger zircons, but this does not appear to be the case ( In general, the high proportions of 900–1200 Ma and 540–700 Ma zircon groups, and low proportions of 485–510 Ma zircons are difficult to reconcile with any then-active magmatic sources at the contemporary Australian–Antarctic sector of the Gondwana margin and in particular the Ross–Delamerian Orogen.
Provenance of the Buller and Takaka terrane sediments
Previous workers have placed a general source for Buller–Takaka terrane sedimentary rocks to the west at the adjacent Gondwana continental margin (e.g. Adams Citation2004). Petrographic and geochemical studies (Nathan Citation1976; Roser et al. Citation1996; Mortimer Citation2009) indicate that Greenland Group sources are a mixture of granitoids, volcanics and low-grade metasediments. In , it is seen that as the 444–542 Ma age subset of the GA group increases, the proportion of the 542–700 Ma subset in the GA group relative to the 700–1600 Ma RA group remains essentially unchanged. This suggests that basement complexes of late Neoproterozoic and late Mesoproterozoic age are geographically related.
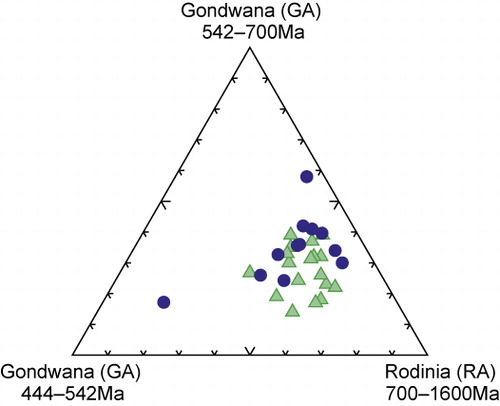
The same broad detrital zircon age features that we have identified in our low metamorphic grade Buller Terrane dataset are also evident in amphibolite facies metamorphic rocks (Ireland Citation1992a; Hiess et al. Citation2010). A similar situation is seen in two amphibolite facies gneissic sample datasets in the present study, from higher metamorphic grades than most of the other datasets: OKZ12 Okuru River, Westland and AG86 Ngakawau Gorge (SF1). The latter is mapped as Karamea Suite granite but has a zircon pattern resembling Greenland Group. Both samples have RA and GA zircon age groups but also some anomalously young ages < 470 Ma (SF1), probably related to the later overprints.
The Takaka Terrane seemingly represents a different tectonic and depositional environment from the Buller Terrane by virtue first, of its Cambrian volcanic arc and associated volcano-sedimentary rocks at an active margin, and second, of its Ordovician–Devonian history of passive-margin deposition, including massive limestones and quartzites (Rattenbury et al. Citation1998). Despite these differences, the present study shows that the Takaka Terrane zircon patterns have significant age components strikingly similar to the Buller Terrane (). There are no secular trends in Takaka RA/GA ratios, or significant age component ratios through the entire Cambrian to Devonian depositional interval sampled (, ). The Middle–Late Cambrian volcanism in the Takaka Terrane has contributed surprisingly little to detrital zircon populations. Indeed, none of the youngest Takaka zircon age components is contemporaneous with deposition (). Some of the youngest Takaka Terrane zircon components may be related to a 515 ± 7 Ma granite in the Cobb Igneous Complex and/or 496 ± 6 Ma granitoid clasts that occur in the Lockett Conglomerate and Dead Goat Conglomerate (Gutjahr et al. Citation2006). The few Early Ordovician zircon components, c. 470–485 Ma in the Ordovician–Silurian Takaka samples, e.g. WGPX3 (), must derive from granitoid sources similar to those in the Ross–Delamerian Orogen.
New detrital zircon age data for the Junction Formation (JCT1 and JCT21, ) do not resolve its terrane status. The general pattern, with RA/GA ratios of 0.6–0.9, resembles that commonly found in both Takaka and Buller terrane datasets, for example, in the Cambrian, Haupiri Group sample WGPY3 (), but only more rarely in Buller Terrane patterns, for example, AOM1 (). The present Junction Formation zircon age data (zircon rims) are not easily compared with the Junction Formation dataset of AF0 (SF2) of Fowler (Citation2000)—although broadly similar, it is not stated whether Fowler’s data were core or rim ages.
In Fiordand, amphibolite-facies Takaka Terrane rocks are present (Turnbull et al. Citation2010), but the substantial metamorphic overprints potentially introduce complications to interpretations of detrital zircon age patterns (Gibson & Ireland Citation1996; Allibone et al. Citation2009; Ireland & Gibson Citation1998; Scott et al. Citation2009). Detrital zircons as young as c. 480 Ma (Lake Roe Schist, Gibson & Ireland Citation1996) constrain a maximum Early Ordovician depositional age. Also, a minimum age for some metasediments is constrained by a TIMS zircon age, c. 493 Ma, for local intrusions (Jacquiery Granitoid Gneiss and Pandora Gneiss) (Ramezani and Tulloch, unpublished, in Allibone et al. Citation2009; Turnbull et al. Citation2010). The Lake Roe Schist, Mt Gladstone Schist, Russet Formation and Deep Cove Gneiss age patterns show the typical broad GA and RA age groups (Ireland & Gibson Citation1998; Scott et al. Citation2009) and have all been tentatively included in the Takaka Terrane by Turnbull et al. (Citation2010). Most schists and paragneisses have relatively low RA/GA ratios (< 0.9). The complexity of the structural situation of these high-grade metasediments and the overall similarity of low-grade Buller and Takaka detrital zircon spectra means that the Buller and Takaka terrane correlations of Turnbull et al. (Citation2010) while plausible, cannot be corroborated by detrital zircon data.
Collectively, detrital zircons provisionally identify three main kinds of likely source areas for the Buller and Takaka terrane sandstones ():
A minor source of Early Ordovician–Middle Cambrian granitoids (younger part of GA group). In the Buller Terrane, these are 480–530 Ma (with significant components at c. 500 and 522 Ma), whereas in the Takaka Terrane these are 470–510 Ma (with a significant component at c. 513 Ma).
Major sources of Early Cambrian–late Neoproterozoic age c. 540–700 Ma (older part of GA group). These could be from plutonic and/or volcanic arcs with significant age components at c. 558 and 586 Ma in the Buller Terrane, and c. 556, 568 and 585 Ma in the Takaka Terrane. In both terranes, there is a subset (22 samples) of common significant age components in a 600–700 Ma age range that is exceptionally rare (one sample only) in Australia–Antarctica datasets ().
A major source in Rodinia basement, mainly late Mesoproterozoic, c. 900–1200 Ma (RA group), with significant age components at c. 1011 and 1060 in both terranes ().
Nature of the Ross–Delamerian basement in southern Zealandia
Cambrian magmatism, metamorphism and deformation in Fiordland and NW Nelson have been cited as evidence for Ross–Delamerian orogenesis (Gibson & Ireland Citation1996; Jongens et al. Citation2003; Gutjahr et al. Citation2006; Bradshaw Citation2007; Scott et al. Citation2008; Allibone et al. Citation2009). The Ross–Delamerian Orogen formed at the late Neoproterozoic–Ordovician continental Gondwana margin as a major feature 100–200 km wide and continuing 4000 km along the Transantarctic Mountains (Ross Orogen) into South Australia (Delamerian Orogen). Within the orogen, in South Australia, Victoria, Northern Victoria Land and the Transantarctic Mountains, there are Cambrian–Early Ordovician granitoid and metamorphic complexes (Stump Citation1995; Foden et al. Citation2002; Birch Citation2003). The earliest magmatism (and metamorphism) is probably Early Cambrian c. 540 Ma (Adams & Höhndorf Citation1991; Stump et al. Citation2006), followed by main-phase (post-tectonic) plutonism in the Middle Cambrian to Early Ordovician (510–470 Ma) (Stump Citation1995). Rapid exhumation of the Ross–Delamerian Orogen provided voluminous sediment detritus for the Ordovician–Silurian flysch to the east, widespread across the Lachlan Orogen in Victoria and New South Wales (VandenBerg & Stewart Citation1992; Turner et al. Citation1996; Ireland et al. Citation1998) and also in Northern Victoria Land (Robertson Bay Terrane) and Marie Byrd Land (Swanson Formation). On the east flank of the orogen, in the Bowers Terrane, Northern Victoria Land, a major Middle–Late Cambrian volcanic arc and associated sedimentary successions have been correlated with the Takaka Terrane (Weaver et al. Citation1984; Münker & Cooper Citation1999; Bradshaw et al. Citation2009).
Compared with the large U–Pb, Rb–Sr and 40Ar/39Ar age datasets for plutonic rocks, metamorphic rocks and deformation events in the main Ross–Delamerian Orogen and Lachlan Orogen, the equivalent datasets for co-eval rocks in New Zealand are meagre and comprise: Jacquiery/Pandora Granitoid Gneiss (one c. 493 Ma U–Pb TIMS zircon age; Allibone et al. Citation2009); Kellard Point Orthogneiss (an older 481 ± 8 Ma subset with a range of Cambrian–Devonian U–Pb zircon ages; Gibson & Ireland Citation1996); Dead Goat Conglomerate granitoid clast (U–Pb zircon age of 496 ± 6 Ma; Gutjahr et al. Citation2006); and a granite in the Cobb Igneous Complex (U–Pb zircon age 515 ± 7 Ma, without details, quoted by Münker & Cooper Citation1999). There are no isotopic age data relating to Late Cambrian deformation (Jongens et al. Citation2003).
Nevertheless, recognition of these rocks of Ross–Delamerian age in New Zealand has resulted in several models attempting to explain the large separation from the parent orogen (> 1000 km on Gondwana reconstructions). For example, Bradshaw (Citation2007) and Bradshaw et al. (Citation2009) recognised similarities between the Middle Cambrian Sledgers Group of Northern Victoria Land and Devil River Volcanics Group (and Haupiri Group) of New Zealand, and placed the Cambrian Takaka Terrane much closer (and far south of its present position), adjacent to the contemporary proto-Pacific margin and with a west-dipping subduction zone. The Greenland Group might have been formerly adjacent to the repositioned Takaka Terrane and thus shared the latter’s sediment sources. Alternatively, it might have been to the north, sharing sediment sources with the Ordovician flysch widespread across the Lachlan Fold Belt (e.g. MKTA4, YBBA1 samples). Most of the Ordovician flysch shows west-to-east current directions, consistent with western sources in the Delamerian Orogen (Birch Citation2003), but Adaminaby Group in easternmost Victoria, has unusual south-to-north current directions (Fenton et al. Citation1982).
Comparison of Australian/Antarctic data, principally SHRIMP ages, with our New Zealand data, principally ICPMS ages, requires caution because SHRIMP ages include both zircon inner (core) and outer zone determinations, and have common-Pb correction and 207Pb/235U, 206Pb/238U discordance criteria that are different from our ICPMS datasets (outer zone ages only). However, a provisional comparison raises several points:
(1) In the southeast Australia Lachlan datasets (i.e. closest to the Ross–Delamerian Orogen), the proportions of Cambrian–Ordovician zircons are frequently highest (usually > 20%) with significant age components at c. 479 and 494 Ma (, ). This is the basis for prevailing models that indicate Lachlan Orogen flysch derivation from the Ross–Delamerian Orogen (e.g. Turner et al. Citation1996). It is possible (but uncertain) that accompanying late Neoproterozoic and late Mesoproterozoic zircon components originate in (fairly distant) primary and reworked sources within the Australian craton, e.g. Musgrave Block (White et al. Citation1999). In contrast, the Buller–Takaka detrital zircon patterns show surprisingly little evidence for a major Ross–Delamerian input as Late Cambrian–Early Ordovician detrital zircons represent only a very small proportion of the total ().
(2) Primary (magmatic) zircon sources in the Late Neoproterozoic age range (542–700 Ma) are very rare in the Ross–Delamerian Orogen but Early Cambrian granite (545 ± 7 Ma), and diorite (531 ± 7 Ma), do occur in the central Transantarctic Mountains (Stump et al. Citation2006) and rhyolite (545 ± 33 Ma) is recorded from Iselin Bank, Ross Sea (Mortimer et al. Citation2011). Despite this, late Neoproterozoic (542–600 Ma) zircons form one of the largest zircon subsets (the greater part of the GA group) in both Buller and Takaka terranes and some Australia/Antarctica datasets (). One explanation might be that such zircons are actually recycled from latest Neoproterozoic–Early Cambrian sedimentary rocks that contain significant 542–600 Ma zircons, such as samples FO60 and GXV125 () in Antarctica (Adams et al. Citation2013) or Early Cambrian Kanmantoo Group, in South Australia (Ireland et al. Citation1998). These sediments would then be supplied to depocentres, but without contributions downstream from Cambrian–Ordovician (Ross–Delamerian) granitoid sources.
(3) Significant primary magmatic zircon sources in the age range 900–1200 Ma are unknown in the Ross–Delamerian Orogen, yet they form a large group in nearly all Buller–Takaka datasets. However, there are significant detrital zircons in this range in the Kanmantoo Group of South Australia (Ireland et al. Citation1998). A 900–1200 Ma group is an enigmatic component that frequently appears, without an obvious ultimate igneous source, in numerous Early Palaeozoic detrital zircon patterns from around the Pacific margin of Gondwana: South and eastern Australia, New Zealand, Northern Victoria Land, Marie Byrd Land and northwest Argentina (Ireland Citation1992b, Citation1998; Pankhurst et al. Citation1998; Adams et al. Citation2011, Citation2013).
(4) As mentioned above, the Buller and Takaka datasets have a distinctive subset of 600–700 Ma age components in the older GA group (22 in total, ). These are also apparent in the oldest Takaka Terrane sampled (Middle Cambrian, Junction Formation). In Late Cambrian–Early Ordovician rocks, the 600–700 Ma zircons are accompanied by a late Mesoproterozoic, c. 1000–1100 Ma group (). The proportions of 600–700 Ma zircon age components decline in frequency in Middle and Late Ordovician rocks. These features are not seen in Antarctic and southeast Australia datasets but are restricted to Zealandian rocks.
There is an alternative potential source area that may explain some of the source constraints and anomalies discussed above. Outboard of the Ross–Delamerian Orogen, there are large areas of continental plateaux, now submerged, that might have Precambrian foundations sufficient to provide sediment sources for the Buller–Takaka depocentres. These include South Tasman Rise, West Tasman Rise and East Tasman Plateau in Australia, and Campbell Plateau and the western Challenger Plateau in Zealandia. Together, these constitute a substantial area > 1 000 000 km2. The Tasmanian examples are a southern extension of the Precambrian–Cambrian Selwyn Block () that includes a Palaeoproterozoic–early Mesoproterozoic terrain that may extend northwards beneath Victoria (Cayley et al. Citation2002, Cayley Citation2011). Within Tasmania itself, the Precambrian Tyennan and Rocky Cape rocks are mainly metasediments with distinctive late Palaeoproterozoic c. 1750 Ma, and early Mesoproterozoic c. 1400 Ma zircon components, and in Bass Strait, unique late Neoproterozoic, c. 750 Ma granitoids (Black et al. Citation1997; Adams et al. Citation2013). These rocks are thus unlikely sources for Buller and Takaka rocks. Cryptic microcontinental blocks, formerly offshore of the Rodinia continental margin, have also been suggested (Borg et al. Citation1990; Stump et al. Citation2006). These authors postulated a ‘Beardmore microcontinent’ offshore of the central Transantarctic Mountains region, which at c. 670 Ma, was separated from it by a zone of rifting. With the onset of Cambrian subduction, this was incorporated westwards into the Ross Orogen.
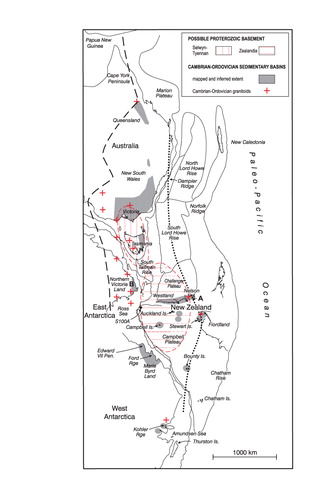
Zealandia in Rodinia
Without very selective erosion of, and transport from, rather local sources, the Ross–Delamerian Orogen does not provide adequate zircon sources, in terms of age and extent, to supply the zircons now found in Buller and Takaka terranes. Indeed, some features of the latter, such as 600–700 Ma detrital zircon components, seem almost ‘endemic’ to Zealandia.
The possibility that Zealandia might have Precambrian basement was postulated by Adams & Griffin (Citation2012). They noted that Late Cretaceous fluviatile sandstones, deposited directly upon Buller Terrane basement in southern Zealandia after separation (< 83 Ma) from Antarctica–Australia, had very high detrital zircon RA/GA ratios > 2.0, higher than any known from the Western or Eastern Provinces (this current study included). They suggested that late Mesoproterozoic (1000–1200 Ma) Rodinian sources are presently hidden beneath southern Zealandia () but were uplifted and briefly eroded at rift margins during Late Cretaceous separation from Gondwana. In this respect, the 1170 ± 9 Ma granodiorite dredged from the southernmost edge of the Campbell Plateau (–) is important. Although Challis et al. (Citation1982) regarded most of dredge S100 as ice-transported erratics originating in East Antarctica, their location 150 m down-slope of probable seafloor outcrop, angular character and a fresh broken face on S100A could indicate a local origin. Similarly, on South Tasman Rise, formerly close to this region (), a quartz-syenite dredge sample has a late Mesoproterozoic zircon age, 1119 ± 9 Ma (Fioretti et al. Citation2005).
On the basis of the new data and interpretation discussed above, we postulate a new model invoking Precambrian basement under the southwestern and western edge of (present-day) Zealandia. This would be in an analogous tectonic position to the South Tasman Rise and Selwyn Block of Tasmania, outboard from the main Ross–Delamerian orogen (). Because of the persistent association of late Mesoproterozoic and late Neoproterozoic zircon groups in the early Palaeozoic detrital zircon age patterns, we further suggest that the Precambrian basement would comprise late Mesoprotozoic granitoid and metamorphic complexes and late Neoproterozoic–Early Cambrian magmatic arcs. The proportions would vary across the block to satisfy the range of RA/GA ratios, 0.6–1.8, and persistence of the 600–700 Ma subgroup in Buller–Takaka zircon patterns.
At Rodinia break-up at c. 700–650 Ma, a proto-Zealandia block, along with the South Tasman Rise and Selwyn Block, was close to the Australian–Antarctic margin, at the eastern side of a rift centre receiving late Neoproterozoic successions (Adelaidean of South Australia, Beardmore Group of Antarctica; Goodge et al. Citation2002). This proto-Zealandia block may have been adjacent to, or contiguous with, the cryptic ‘Beardmore microcontinent’ of Stump et al. (Citation2006). The Ross–Delamerian Orogen developed at the main continental margin and culminated in widespread Middle Cambrian–Early Ordovician granitoid plutonism. In Middle–Late Cambrian time, major volcanic arcs formed in Tasmania and Victoria and in the Bowers Terrane (B, ) in Northern Victoria Land. Bradshaw (Citation2007), Bradshaw et al. (Citation2009) placed the Takaka Terrane Cambrian arc close to the Bowers Terrane, citing similarities in volcanic associations and back-arc sedimentary successions containing conglomerates with granitoid clasts originating in the Ross Orogen. However, Nd-isotopic comparison of Dead Goat Conglomerate clasts (Gutjahr et al. Citation2006) with a range of possible Ross–Delamerian values is unconvincing and the trace element discriminants (Y, Zr) show that they are different.
Bradshaw et al. (Citation2009) suggested that the Takaka Terrane then migrated to its present position, in conjunction with rollback of the Ordovician–Devonian subduction zone responsible for the Lachlan Fold Belt. We propose here a modification of this model in which the Takaka Terrane Cambrian magmatic arc was formed instead at the margin of the proto-Zealandia block as the latter became detached from both the Australian–Antarctic margin and the Selwyn Block (). This explains the commonality of Rodinia (RA) detrital zircon patterns across the whole region and also the increasing endemism of Zealandia zircon patterns after 700 Ma, when potential sediment sources in the Ross–Delamerian Orogen and Selwyn/Tasmania Block were severed. Both the Cambrian volcanism (and minor plutonism) and the Buller and Takaka terrane sediment sources are thus all indigenous to the proto-Zealandia block itself. Sediment transport from easterly and southerly directions in the Buller Terrane (Greenland Group; Laird Citation1972) and the Takaka Terrane (Haupiri Group, Lockett Conglomerate; Pound Citation1993) would thus reflect sources solely within proto-Zealandia.
Palaeoproterozoic and Archean zircons in the Takaka Terrane
The Nuna (NU) group of Palaeoproterozoic and Archean zircons frequently occur (c. 5% total, rarely > 20%) in Palaeozoic rocks in Zealandia, Australia and Antarctica. They are especially prominent in shallow-water quartzites (Hickey Citation1986) TAK1 and TAK2 from Ordovician Wangapeka Formation (, ) with, respectively, 7% and 10% Palaeoproterozoic, and 13% and 12% Archean zircons. This occurrence is well outboard from Palaeoproterozoic and Archean rocks in Australia–Antarctica (). The reason for such high > 2000 Ma zircon proportions is uncertain but might also indicate a hitherto unsuspected, more local source.
Conclusions
Detrital zircon ages in Early Palaeozoic sandstones from both Buller and Takaka terranes define two major groups, each 30–50% of the total zircon populations: (1) an early Palaeozoic–late Neoproterozoic, ‘Gondwana’ (GA) group (444–700 Ma, but mainly 540–700 Ma), and an early Neoproterozoic–Mesoproterozoic, ‘Rodinia’ (RA) group (700–1600 Ma, but mainly 900–1200 Ma). The remainder are of Palaeoproterozoic and Archean age (a ‘NUNA’ NU group), each age usually 5–10%, but in the Takaka Terrane together reaching 20%, with ages as old as 3560 Ma.
Over the 120 Ma Cambrian–Devonian depositional record, the RA/GA ratios in both terranes have similar ranges, 0.6–1.8, across the entire region, suggesting that the two major zircon sources were widely distributed, mixed and voluminous, and represent enduring major complexes of Cordilleran-style granitoids and regional metamorphic complexes.
Significant zircon age components in Buller and Takaka terrane sandstones are strikingly similar: within the GA group these are c. 515, 555 and 585 Ma; and in the RA group c. 1010 and 1060 Ma. These are present in most Cambrian–Devonian samples. Exceptions are late Neoproterozoic, 600–700 Ma, age components, possibly ‘endemic’ to Zealandia, that are common in Middle Cambrian–Early Ordovician samples. These are extremely rare in southeast Australian (Victoria) and Antarctica (Northern Victoria Land).
The detrital zircons in both Buller and Takaka sandstones are overwhelmingly reworked and there is only minor evidence (in the Buller Terrane alone), for juvenile zircon inputs demonstrably synchronous with deposition (i.e. from contemporary volcanism).
The prominence of the GA and RA zircon groups, and the relatively low proportion of Late Cambrian–Early Ordovician zircons cannot easily be reconciled with Buller and Takaka terrane sediment sources originating at the contemporary Gondwana margin in southeast Australia and Antarctica, in particular from within the Ross–Delamerian Orogen.
We propose a model in which a proto-Zealandia block of early Neoproterozoic–late Mesoproterozoic (900–1200 Ma) and Early Cambrian–late Neoproterozoic (540–700 Ma) rocks became detached, commencing in Early Cambrian time, from both the Australia–Antarctica Rodinia margin and the Selwyn Block, and which is now preserved as Precambrian basement beneath southwestern and western Zealandia. In Middle Cambrian to Devonian time, this block drifted progressively away from Australia–Antarctica, with Cambrian felsic plutonism, arc volcanism and deformation, and Ordovician–Devonian passive-margin sedimentation developing at its margin.
Associate Editor: Dr Richard Jongens.
Supplementary data
Supplementary file 1: Table S1. Tabulation of full U–Pb isotopic age data for all new analyses, presented herein, of detrital zircons from 34 early Palaeozoic sandstone samples derived from Buller Terrane and Takaka Terrane sequences in southern New Zealand.
Supplementary file 2: Table S2. Tabulation of detrital zircon U–Pb age components derived from previously published data from within Zealandia, Australia and Antarctica.
Table S2. Tabulation of detrital zircon U-Pb age components derived from previously published data from within Zealandia, Australia and Antarctica.
Download MS Excel (47 KB)Table S1. Tabulation of full U-Pb isotopic age data for all new analyses, presented herein, of detrital zircons from 34 early Palaeozoic sandstone samples derived from Buller Terrane and Takaka Terrane sequences in southern New Zealand.
Download MS Excel (2.6 MB)Acknowledgements
The authors thank GNS Science colleagues Simon Nathan, Malcolm Laird, Mark Rattenbury, Ian Turnbull and Richard Jongens for sharing their extensive knowledge of Western Province geology. Earlier versions of the manuscript were improved by comments from three anonymous referees and by editor Richard Jongens. The Ministry of Business, Innovation and Employment, Wellington, kindly provided access to core samples from Great South Basin oil-exploration drillholes. Norman Pearson and Will Powell at GEMOC, Macquarie University, Sydney, provided valuable technical assistance throughout the programme. This is contribution no. 529 of the ARC Centre of Excellence for Core to Crust Fluid Systems at Macquarie University (http://www.ccfs.mq.edu.au), and contribution no. 972 in the GEMOC Key Centre (http://www.gemoc.mq.edu.au). Philip Carthew at GNS Science created many of the diagrams. The analytical data were obtained using instrumentation funded by Department of Education Science and Training (DEST) Systemic Infrastructure Grants (now Department of Education, Employment and Workplace Relations), ARC Linkage Infrastructure Equipment and Facilites (LIEF), National Collaborative Research Infrastructure Strategy (NCRIS), industry partners and Macquarie University.
References
- Adams CJ 1986. Geochronological studies of the Swanson formation of Marie Byrd land, West Antarctica, and correlations with Northern Victoria Land, East Antarctica, and South Island, New Zealand. New Zealand Journal of Geology and Geophysics 29: 345–358. 10.1080/00288306.1986.10422157
- Adams CJ 2004. Rb-Sr age and strontium isotope characteristics of the Greenland Group, Buller Terrane, New Zealand, and correlations at the East Gondwanaland margin. New Zealand Journal of Geology and Geophysics 47: 189–200. 10.1080/00288306.2004.9515047
- Adams CJ 2008. Geochronology of Paleozoic terranes at the Pacific Ocean margin of Zealandia. Gondwana Research 13: 250–258. 10.1016/j.gr.2007.07.001
- Adams CJ, Bradshaw JD, Ireland TR 2013. Provenance connections between late Neoproterozoic and early Paleozoic sedimentary basins of the Ross Sea region, Antarctica, southeast Australia and southern Zealandia. Antarctic Science 26: 173–182.
- Adams CJ, Cullen DJ 1978. Potassium-argon ages of granites and metasediments from the Bounty Islands area, southwest Pacific Ocean. Journal of the Royal Society of New Zealand 8: 127–132.
- Adams CJ, Griffin WL 2012. Rodinian detrital zircons in Late Cretaceous sandstones indicate a possible Precambrian basement under southern Zealandia. Precambrian Research 212–213: 13–20.
- Adams CJD, Harper CT, Laird MG 1975. K-Ar ages of low-grade metasediments of the Greenland and Waiuta Groups in Westland and Buller, New Zealand. New Zealand Journal of Geology and Geophysics 18: 39–48.
- Adams CJ, Höhndorf A 1991. Age of the metamorphic basement of the Salamander and Lanterman Ranges, Northern Victoria Land, Antarctica. In: Thomson MRA, Crame JA, Thomson JW eds. Geological evolution of Antarctica. Cambridge, Cambridge University Press. Pp. 149–153.
- Adams CJ, Ireland TR 2007. Provenance connections between Late Neoproterozoic and early Paleozoic sedimentary basins of the Ross Sea region, Antarctica, southeast Australia and southern Zealandia. In: Cooper AK, Raymond CR, 10th ISAES Editorial Team eds. Antarctica: a keystone in a changing world. Online Proceedings for the 10th International Symposium on Antarctic Earth Sciences. US Geological Society Open File Report 2007–1047, Extended Abstract 064. Reston, VA, US Geological Survey. http://pubs.usgs.gov/of/2007/1047/ea/of2007-1047ea064.pdf (accessed 21 March 2015).
- Adams CJ, Kelley S 1998. Provenance of Permian–Triassic and Ordovician metagreywacke terranes in New Zealand; evidence from 40Ar/39Ar dating of detrital micas. Geological Society of America Bulletin 110: 422–432.
- Adams CJ, Miller H, Acenolaza, FG, Toselli AJ, Griffin WL 2011. The Pacific Gondwana margin in the late Neoproterozoic–early Paleozoic: detrital zircon U-Pb ages from metasediments in northwest Argentina reveal their maximum age, provenance and tectonic setting. Gondwana Research 19: 71–83. 10.1016/j.gr.2010.05.002
- Adams CJ, Morris PA, Beggs JM 1979. Age and correlation of volcanic rocks of Campbell Island and metamorphic basement of the Campbell Plateau, South-west Pacific Ocean. New Zealand Journal of Geology and Geophysics 22: 679–671.
- Allibone AH, Jongens R, Scott JM, Tulloch AJ, Turnbull IM, Cooper AF et al. 2009. Plutonic rocks of the Median Batholith in eastern and central Fiordland, New Zealand: field relations, geochemistry, correlation and nomenclature. New Zealand Journal of Geology and Geophysics 52: 101–148.
- Andersen T 2002. Correction of common lead in U-Pb analyses that do not report 204Pb. Chemical Geology 192: 59–79.
- Beggs JM, Challis GA, Cook RA 1990. Basement geology of the Campbell Plateau: implications for correlation of the Campbell Magnetic Anomaly system. New Zealand Journal of Geology and Geophysics 33: 401–404.
- Benson WN, Keble RA 1935. The Geology of the Regions adjacent to Preservation and Chalky Inlets, Fiordland, New Zealand. Part IV. Stratigraphy and paleontology of the fossiliferous Ordovician rocks. Transactions of the Royal Society of New Zealand 65: 244–294.
- Birch WD ed. 2003. Geology of Victoria. Special Publication 23. Melbourne, Geological Society of Australia (Victoria Division). 842 p.
- Bishop DG, Bradshaw JD, Landis CA 1985. Provisional terrane map of South Island, New Zealand. In: Howell DG ed. Tectonostratigraphic Terranes. Houston, Texas, Circum-Pacific Council for Energy and Mineral Resources Earth Science Series 1: Pp. 515–521.
- Black LP, Seymour DH, Corbett KD, Cox SE, Streit JE, Bottril RS et al. 1997. Dating Tasmania’s oldest events. AGSO record 1997/15. Canberra, Australian Geological Survey Organisation. 57 p.
- Borg SG, DePaolo DJ, Smith BM 1990. Isotopic structure and tectonics of the central Transantarctic Mountains. Journal of Geophysical Research 95: 6647–6667.
- Bradshaw MA 1995. Stratigraphy and structure of the Lower Devonian rocks of the Waitahu and Orlando Outliers near Reefton, New Zealand. New Zealand Journal of Geology and Geophysics 38: 81–92.
- Bradshaw MA 2000. Base of the Devonian Baton Formation and the question of a pre-Baton tectonic event in the Takaka Terrane, New Zealand. New Zealand Journal of Geology and Geophysics 43: 601–610.
- Bradshaw JD 2007. The Ross Orogen and the Lachlan Fold Belt in Marie Byrd Land, northern Victoria land and New Zealand: implications for the tectonic setting of the Lachlan Fold Belt in Antarctica. In: Cooper AK, Raymond CR, 10th ISAES Editorial Team 2007 eds. Antartica: a keystone in a changing world. Online proceedings for the 10th International Symposium on Antarctic Earth Science. US Geological Society Open File 2007-1047, Short research paper 059. Reston, VA, US Geological Survey. http://pubs.usgs.gov/of/2007/1047/srp/srp059/of2007-1047srp059.pdf (accessed 4 March 2015).
- Bradshaw JD, Andrews PB, Field BD 1983. Swanson Formation and related rocks of Marie Byrd Land and a comparison with the Robertson Bay Group of Northern Victoria Land. In: Oliver RL, James PR, Jago JB eds. Antarctic earth science. Canberra, Australian Academy of Sciences. Pp. 177–189.
- Bradshaw JD, Gutjahr M, Weaver SD, Bassett KN 2009. Cambrian intra-oceanic arc accretion to the austral Gondwana: constraints on the location of proto-New Zealand. Australian Journal of Earth Sciences 56: 587–594.
- Bradshaw MA, Hegan BD 1983. Stratigraphy and structure of the Devonian rocks of the Inangahua outlier, Reefton, New Zealand. New Zealand Journal of Geology and Geophysics 26: 325–344.
- Bradshaw JD, Pankhurst RJ, Weaver SD, Storey BC, Muir RJ, Ireland TR 1997. New Zealand Superterranes recognised in Marie Byrd Land and Thurston Island. In: Ricci CA ed. The Antarctic region: geological evolution and processes. Proceedings of the Seventh International Symposium on Antarctic Earth Sciences. Siena, Terra Antartica Publication. Pp. 429–436.
- Burrett C, Berry R 2000. Proterozoic Australia-Western United States (AUSWUS) fit between Laurentia and Australia. Geology 28: 103–106.
- Cayley RA 2011. Exotic crustal block accretion to the eastern Gondwanaland margin in the Late Cambrian—Tasmania, the Selwyn Block and implications for the Cambrian–Silurian evolution of the Ross, Delamerian, and Lachlan orogens. Gondwana Research 19: 628–649.
- Cayley RA, Taylor DH, VandenBerg AHM, Moore DH 2002. Proterozoic–Early Paleozoic rocks and the Tyennan orogeny in central Victoria: the Selwyn Block and its tectonic implications. Australian Journal of Earth Sciences 49: 225–254.
- Challis GA, Gabites J, Davey FJ 1982. Precambrian granite and manganese nodules dredged from the southwestern Campbell Plateau (Note). New Zealand Journal of Geology and Geophysics 25: 493–497.
- Cook RA, Sutherland R, Zhu R 1999. Cretaceous–Cenozoic geology and petroleum systems of the Great South Basin, New Zealand. Institute of Geological and Nuclear Sciences Monograph 20. Lower Hutt, Institute of Geological & Nuclear Sciences Ltd. 190 p
- Cooper RA 1974. Age of the Greenland and Waiuta Groups, South Island, New Zealand (Note). New Zealand Journal of Geology and Geophysics 17: 955–962.
- Cooper RA 1989. Early Paleozoic terranes of New Zealand. Journal of the Royal Society of New Zealand 19: 73–112.
- Cooper RA, Tulloch AJ 1992. Early Paleozoic terranes in New Zealand and their relationship to the Lachland Fold belt. Tectonophysics 214: 129–144.
- Cullen DJ 1975. Autochthonous rocks of the Bounty Islands region southwest Pacific Ocean. New Zealand Journal of Geology and Geophysics 18: 767–785.
- Dalziel IWD 1991. Pacific margin of Laurentia and east Antarctica–Australia as a conjugate rift pair: evidence and implications for an Eocambrian supercontinent. Geology 19: 598–601.
- Fenton MW, Keene JB, Wilson CJL 1982. Sedimentology and environment of deposition of the Mallacoota Beds, eastern Victoria. Journal of the Geological Society of Australia 29: 107–114.
- Fergusson CL, Fanning CM 2002 Late Ordovician stratigraphy, zircon provenance and tectonics, Lachlan Fold Belt, southeastern Australia. Australian Journal of Earth Sciences 49: 423–436.
- Fioretti AM, Black LP, Foden J, Visona D 2005. Grenville age magmatism at the South Pacific Rise: a new piercing point for the reconstruction of Rodinia. Geology 33: 769–772.
- Foden JD, Elburg MA, Turner SP, Sandiford M, O’Callaghan J, Mitchell S 2002. Granite production in the Delamerian Orogen, South Australia. Journal of the Geological Society of London 159: 557–575.
- Fowler A 2000. Age, genesis, provenance and correlation of the Junction Formation and the Balloon Melange, northwest Nelson, New Zealand. Unpublished BSc Honours project. Christchurch, University of Canterbury. 67 p.
- Gibson GM, Ireland TR 1996. Extension of the Delamerian (Ross Orogen) into western New Zealand: evidence from zircon ages and implications for crustal growth along the Pacific margin of Gondwana. Geology 24: 1087–1090.
- Goodge JW, Myrow P, Williams IS, Bowring SA 2002. Age and provenance of the Beardmore Group, Antarctica: constraints on Rodinia Supercontinental breakup. Journal of Geology 110: 393–406.
- Grindley GW 1971. Takaka sheet S8. Geological map of New Zealand 1: 63, 360. Wellington, Department of Scientific and Industrial Research. 19 p. 1 map + booklet.
- Gutjahr M, Bradshaw JD, Weaver S, Münker C, Ireland T 2006. Provenance of Cambrian conglomerates from New Zealand: implications for the tectonomagmatic evolution of the SE Gondwana margin. Journal of the Geological Society of London 163: 997–1010.
- Hickey KA 1986. Geology of Palaeozoic and Tertiary rocks between Upper Takaka and the Waingaro River, North-west Nelson. MSc thesis. Auckland, University of Auckland 146 p.
- Hiess J, Ireland T, Rattenbury M 2010. U-Th-Pb zircon and monazite geochronology of Western Province gneissic rocks, central–south Westland, New Zealand. New Zealand Journal of Geology and Geophysics 53: 241–269.
- Hunt International Petroleum 1977. Final report Kawau 1A. Ministry of Economic Development unpublished petroleum report 716. Wellington, Ministry of Economic Development. 412 p.
- Hunt International Petroleum 1978. Hoiho-1C well completion report. Ministry of Economic Development unpublished petroleum report 730. Wellington, Ministry of Economic Development. 249 p.
- Ireland TR 1992a. Crustal evolution of New Zealand: evidence from age distributions of detrital zircons in Western Province paragneisses and Torlesse greywacke. Geochimica et Cosmochimica Acta 56: 911–920.
- Ireland TR 1992b. Correlation and provenance of sedimentary units around the southwestern Pacific margin. Geological Society of New Zealand, 1992 Annual Conference, Programme and Abstracts. Geological Society of New Zealand Miscellaneous Publication 63A, 84 p.
- Ireland TR, Flöttman T, Fanning CM, Gibson GM, Preiss WV 1998. Development of the early Paleozoic Pacific margin of Gondwana from detrital zircon ages across the Delamerian Orogen. Geology 26: 243–246.
- Ireland TR, Gibson GM 1998. SHRIMP monazite and zircon geochronology of high-grade metamorphism in New Zealand. Journal of Metamorphic Geology 16: 149–167.
- Jackson SE, Pearson NJ, Griffin WL, Belousova EA 2004. The application of laser ablation-inductively coupled plasma-mass spectrometry (LA-ICP-MS) to in situ U-Pb zircon geochronology. Chemical Geology 211: 47–69.
- Jongens R 2006. Structure of the Buller and Takaka Terrane rocks adjacent to the Anatoki Fault, northwest Nelson, New Zealand. New Zealand Journal of Geology and Geophysics 49: 443–461.
- Jongens R, Bradshaw JD, Fowler AP 2003. The Balloon Melange, northwest Nelson: Origin structure, and emplacement. New Zealand Journal of Geology and Geophysics 46: 437–448.
- Karlstrom KE, Harlan SS, Williams ML, McClelland J, Geissman JW, Ahall KI 1999. Refining Rodinia: geologic evidence for the Australia-western U.S. connections in the Proterozoic. GSA Today 9: 1–7.
- Kilpatrick DJ, Fleming PD 1980. Lower Ordovician sediments in the Wagga Trough: discovery of early Bendigonian graptolites near Eskdale, Victoria. Journal of the Geological Society of Australia 27: 69–73.
- Laird MG 1972. Sedimentology of the Greenland group in the Paparoa range, West Coast, South Island. New Zealand Journal of Geology and Geophysics 15: 372–393.
- Laird MG, Shelley D 1974. Sedimentation and early tectonic history of the Greenland Group, Reefton, New Zealand. New Zealand Journal of Geology and Geophysics 17: 839–854.
- Li ZX, Bogdanovab SV, Collinsc AS, Davidsond A, De Waelea B, Ernst RE, et al. 2008. Assembly, configuration and break-up history of Rodinia: a synthesis. Precambrian Research 160: 179–210. 10.1016/j.precamres.2007.04.021
- Ludwig KR 2003. User’s manual for ISOPLOT 3.00: a geochronological toolkit for Microsoft Excel. Berkeley Geochronology Centre Special Publication 4. Berkeley, CA, BGC. 74 p.
- Mortimer N 2009. Zealandia’s Early Paleozoic greywackes: detrital mineralogy of the Greenland and Reefton Groups. Australasian Institute of Mining and Metallurgy, New Zealand Branch Annual Conference 42: 305–313.
- Mortimer N, Nathan S, Jongens R, Kawachi Y, Ryland C, Cooper AF, et al. 2013. Regional metamorphism of the early Paleozoic Greenland Group, South Westland, New Zealand. New Zealand Journal of Geology and Geophysics 56: 1–15.
- Mortimer N, Palin JM, Dunlap WJ, Hauff F 2011. Extent of Ross Orogen in Antarctica: new data from DSDP 270 and Iselin Bank. Antarctic Science. 10.1017/S0954102010000969
- Münker C, Cooper RA 1995. The island arc setting of a New Zealand Cambrian volcano-sedimentary sequence: implications for the evolution of the SW Pacific Gondwana margin. Journal of Geology 103: 687–700.
- Münker C, Cooper RA 1999. The Cambrian arc complex of the Takaka Terrane, New Zealand: an integrated stratigraphical, paleontological and geochemical approach. New Zealand Journal of Geology and Geophysics 42: 415–445.
- Münker C, Crawford A 2000. Cambrian arc evolution along the SE Gondwana active margin: a synthesis from Tasmania–New Zealand–Australia–Antarctica correlations. Tectonics 19: 415–432.
- Mutch AR 1964. Martins Bay sheet S105. 1st ed. Geological Map of New Zealand 1: 63, 360. Wellington, Department of Scientific and Industrial Research. 1 map.
- Nathan S 1976. Geochemistry of the Greenland Group (early Ordovician), New Zealand. New Zealand Journal of Geology and Geophysics 19: 683–706.
- Nathan S, Rattenbury MS, Suggate RP (compilers) 2002. Geology of the Greymouth area. Institute of Geological & Nuclear Sciences 1: 250 000 geological map 12. Lower Hutt, Institute of Geological & Nuclear Sciences Ltd. 58 p. 1 map + 1 book.
- Oliver RL 1950. Preliminary report on the geology of Campbell Island. In: Oliver RL, Finlay HJ, Fleming CA. The geology of Campbell Island. Wellington, Department of Scientific and Industrial Research. Pp 7–44.
- Pankhurst RJ, Weaver, SD, Bradshaw, JD, Storey BC, Ireland TR 1998. Geochronology and geochemistry of pre-Jurassic superterranes in Marie Byrd Land, Antarctica. Journal of Geophysical Research 103B: 2529–2547.
- Pound KS 1993. Geology of the Lower Paleozoic Haupiri Group rocks, Cobb Valley area, Northwest Nelson, New Zealand. Unpublished PhD thesis. Dunedin, New Zealand. University of Otago. Pp. 122–124.
- Preiss WV 2002. The Adelaide Geosyncline in South Australia and its significance in Neoproterozoic continental reconstructions. Precambrian Research 100: 21–63.
- Rattenbury MS, Cooper RA, Johnston MR (compilers) 1998. Geology of the Nelson area. Institute of Geological & Nuclear Sciences 1: 250 000 geological map 9. Lower Hutt, Institute of Geological & Nuclear Sciences Ltd. 1 map + 67 p.
- Rattenbury MS, Jongens R, Cox SC (compilers) 2010. Geology of the Haast area. Institute of Geological & Nuclear Sciences 1: 250 000 geological map 14. Lower Hutt, GNS Science. 1 map + 58 p.
- Roser BP, Nathan S 1997. An evaluation of elemental mobility during metamorphism of a turbidite sequence (Greenland Group, New Zealand). Geological Magazine 134: 219–234.
- Roser BP, Cooper RA, Nathan S, Tulloch AJ 1996. Reconnaisance sandstone geochemistry, provenance and tectonic setting of the lower Paleozoic terranes of the West Coast and Nelson, New Zealand. New Zealand Journal of Geology and Geophysics 39: 1–16.
- Scott JM, Cooper AF, Palin JM, Tulloch AJ, Kula J, Jongens R, et al. 2009. Tracking the influence of continental margin on growth of a magmatic arc in Fiordland, New Zealand using thermobarometry, thermochronology and zircon U–Pb and Hf isotopes. Tectonics 28: TC6007. 10.1029/2009TC002489
- Scott JM, Turnbull IM, Ewing TA, Allibone AH, Palin JM, Cooper AF 2008. Petrology and geochronology of the volcaniclastic and volcanogenic Mesozoic Loch Burn Formation in eastern Fiordland, New Zealand. New Zealand Journal of Geology and Geophysics 51: 89–103.
- Stump E 1995. The Ross Orogen of the Transantarctic mountains. Cambridge, Cambridge University Press. 285 p.
- Stump E, Gootee B, Talarico F 2006. Tectonic models for the development of the Byrd Glacier discontinuity and the surrounding regions of the Transantarctic Mountains during the Neoproterozoic-early Paleozoic. In: Futterer DK, Damaske D, Kleinschmidt G, Miller H, Tessensohn F eds. Antarctic contributions to global earth science. Berlin, Springer. Pp. 181–194.
- Sutherland R 1999. Basement geology and tectonic development of the greater New Zealand region: an interpretation from regional magnetic data. Tectonophysics 308: 341–362.
- Tulloch AJ, Ramezani J, Kimbrough DL, Faure K, Allibone AH 2009. U-Pb geochronology of Paleozoic plutonism in western New Zealand: implications for S-type granite generation and growth of the east Gondwana margin. Geological Society of America Bulletin 121: 1236–1261.
- Turnbull IM, Allibone AH, Jongens R (compilers) 2010. Geology of the Fiordland area. Institute of Geological & Nuclear Sciences 1: 250 000 geological map 17. Lower Hutt, GNS Science. 1 map + 97 p.
- Turner SP, Kelley SP, VandenBerg AHM, Foden JD, Sandiford M, Flöttman T 1996. Source of the Lachlan fold belt flysch linked to convective removal of the lithospheric mantle and rapid exhumation of the Delamerian–Ross fold belt. Geology 24: 941–944.
- VandenBerg AHM, Stewart JR 1992. Ordovician terranes of the southeastern Lachlan Fold Belt: stratigraphy, structure and paleogeographic reconstruction. Tectonophysics 214: 159–176. 10.1016/0040-1951(92)90195-C
- Weaver SD, Bradshaw JD, Laird MG 1984. Geochemistry of Cambrian volcanics of the Bowers Supergroup and implications for the early Paleozoic tectonic evolution of northern Victoria Land, Antarctica. Earth and Planetary Science Letters 68: 128–140.
- White RW, Clarke G, Nelson DR 1999. SHRIMP U-Pb dating of Grenville-age events in the western part of the Musgrave Block, central Australia. Journal of Metamorphic Geology 17: 465–481.
- Williams IS, Chen Y, Chappell BW, Compston W 1988. Dating sources of the Bega batholith granites by ion microprobe. Geological Society of Australia Abstract Series 21: 424.
- Willis I 1965. Stratigraphy and structure of the Devonian strata at Baton River, New Zealand. New Zealand Journal of Geology and Geophysics 8: 35–48.
- Wysoczanski RJ, Gibson GM, Ireland TR 1997. Detrital zircon age patterns and provenance in late Paleozoic–early Mesozoic New Zealand terranes and development of the paleo-Pacific Gondwana margin. Geology 25: 939–942.