Abstract
Waimumu Quartz Gravels, 8 km southwest of Gore, New Zealand, were deposited in Pliocene river channels, and were derived by recycling of older sediments on nearby uplifting mountain ranges. The high proportion of quartz pebbles (>90%) is a result of the decomposition of clay-altered lithic clasts during sedimentary recycling. Most of the quartz was derived from the Otago Schist Belt to the north, including upper greenschist facies metachert clasts with spessartine and piemontite. The quartz gravels at Waimumu Stream mine contain abundant Mn-rich garnet clasts that were derived from Otago Schist. In addition, chromite clasts constitute >10% of the heavy mineral sand fraction, and this may have been derived from the Dun Mountain Ophiolite Belt although the exact source is unknown. Most detrital gold occurs as flakes that have undergone long-distance transport. Rare grains of crystalline gold occur, some of which are in association with prismatic quartz crystals. Gold flakes have minor evidence for authigenic gold overgrowths in association with authigenic marcasite, and gold mobility may have been enhanced by marcasite precipitation and/or oxidation.
Introduction
Placer gold deposits are locally important economic resources, and numerous deposits have been mined sporadically in southern New Zealand over the past 150 years. Some placer deposits can provide indications of source directions and even sources of primary deposits in basement rocks, and therefore constitute useful mineral exploration indicators (Williams Citation1974; Boyle Citation1979; Craw et al. Citation2013, Citation2015a). Because placer deposits result from progressive deposition, reworking and winnowing of sediments, they contain elevated concentrations of dense (heavy) minerals that can record evidence of source regions that are less well represented in normal limited-cycle fluvial deposits (Boyle Citation1979; Youngson & Craw Citation1993; Garnett & Bassett Citation2005).
The Waimumu Quartz Gravels of Southland are Pliocene fluvial deposits that have been extensively worked for placer gold historically, and they host some small modern mines (MacPherson Citation1937; Clough & Craw Citation1989; Craw Citation1992; Falconer & Craw Citation2009). The sources of gold and other minerals in the gravels have not been defined in detail, although a contribution from veins in the Otago Schist () is generally assumed (MacPherson Citation1937; Craw et al. Citation2003; Craw Citation2013). There is no active fluvial link between potential Otago Schist source areas and present occurrences of Waimumu Quartz Gravels, as any such links have been disrupted and broken by tectonic uplift in the intervening areas (, ; MacPherson Citation1937; Craw Citation2013).
Figure 1 Regional basement geological map (partly after Turnbull Citation2000; Turnbull & Allibone Citation2003) showing the location of the Waimumu placer goldfield in relation to various basement rock types mentioned in the text. Cover sediments are indicated with transparent grey shading.
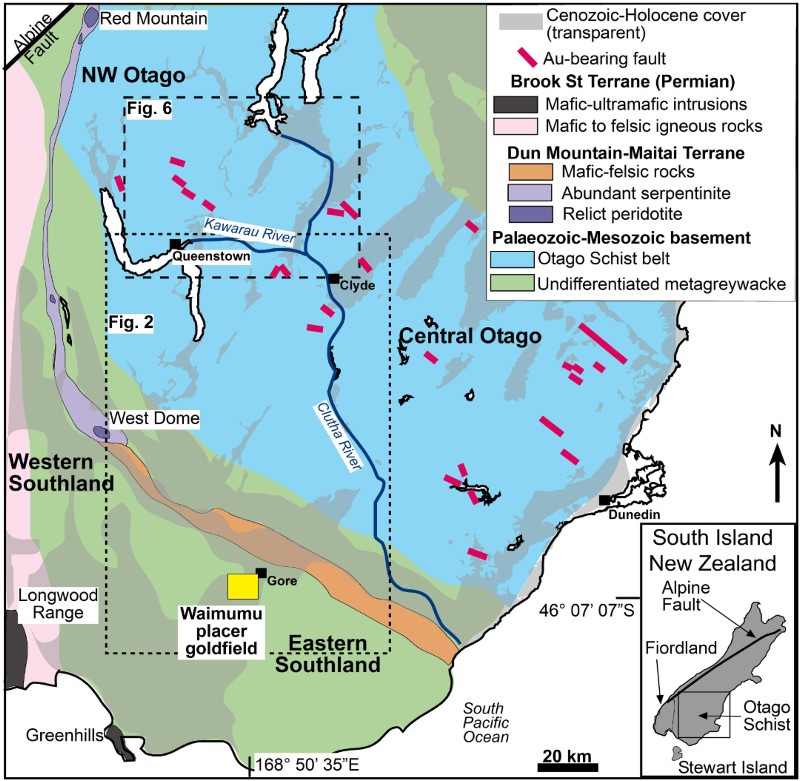
One of the small active mines in Waimumu Quartz Gravels at Waimumu Stream produces relatively large amounts of heavy minerals compared to other nearby mine sites (). Further, the heavy mineral content at another mine, Belle-Brook, is unusual because of the presence of abundant authigenic marcasite (Clough & Craw Citation1989; Falconer et al. Citation2006). The third active mine, Parker Road, has only minor heavy mineral contents apart from gold. In this study, we take advantage of the abundant heavy minerals at the Waimumu Stream mine to investigate the provenances of the sediments. We use these observations to infer the source(s) of the placer gold and associated drainage geometry in the context of the known tectonic history of the region.
General geology
Regional setting
The Waimumu Stream mine occurs in a broad low-relief river valley of the Mataura River in eastern Southland, within the Waimumu placer goldfield (). The broad river valleys in the area occur between ranges of hills that have been rising since the Miocene, and are still rising along active fault zones (; Turnbull & Allibone Citation2003; Stein et al. Citation2011; Craw Citation2013). Most of the uplift has occurred to the north of Waimumu in the Umbrella, Old Man and Garvie ranges (). In addition, there has been uplift in the immediate vicinity of Waimumu, particularly along the Hillfoot and Hedgehope faults (). These uplifted ranges consist largely of Palaeozoic–Mesozoic basement consisting of metagreywacke with interlayered siltstone and argillite (; Turnbull & Allibone Citation2003). Further north, the metagreywackes become progressively more schistose towards the Otago Schist Belt, the core of which lies to the north of a complex set of mountain ranges that culminate in the Old Man Range (, ). A narrow zone containing ultramafic to felsic igneous rocks, the Dun Mountain Ophiolite Belt (Coombs et al. Citation1976; Cawood Citation1986), lies along the southern base of these mountain ranges with poor outcrop (, ).
Fluvial sediments have been accumulating in the Southland region since the late Oligocene and early Miocene, starting with the Gore Lignite Measures that overlie and interdigitate with marine sediments (A; Isaac & Lindqvist Citation1990; Stein et al. Citation2011). Basal parts of the Gore Lignite Measures include locally derived subangular to subrounded, poorly sorted (immature) conglomerates and sandstones dominated by metagreywacke clasts (A; Stein et al. Citation2011). However, a major component of the Gore Lignite Measures clastic material is quartz rich and was derived from Otago Schist as a southern continuation of rivers draining the hills formed by incipient uplift in Central Otago (, A; Isaac & Lindqvist Citation1990; Stein et al. Citation2011).
Figure 3 Stratigraphic and sedimentological setting for the Waimumu Stream mine in the Waimumu Quartz Gravels. A, Summary stratigraphic column, with indications of sediment transport directions (partly after Isaac & Lindqvist Citation1990; Stein et al. Citation2011). B, Photograph of polymict gravels that overlie the mine gravels. C, Gold-bearing Waimumu Quartz Gravels in a channel at the mine.
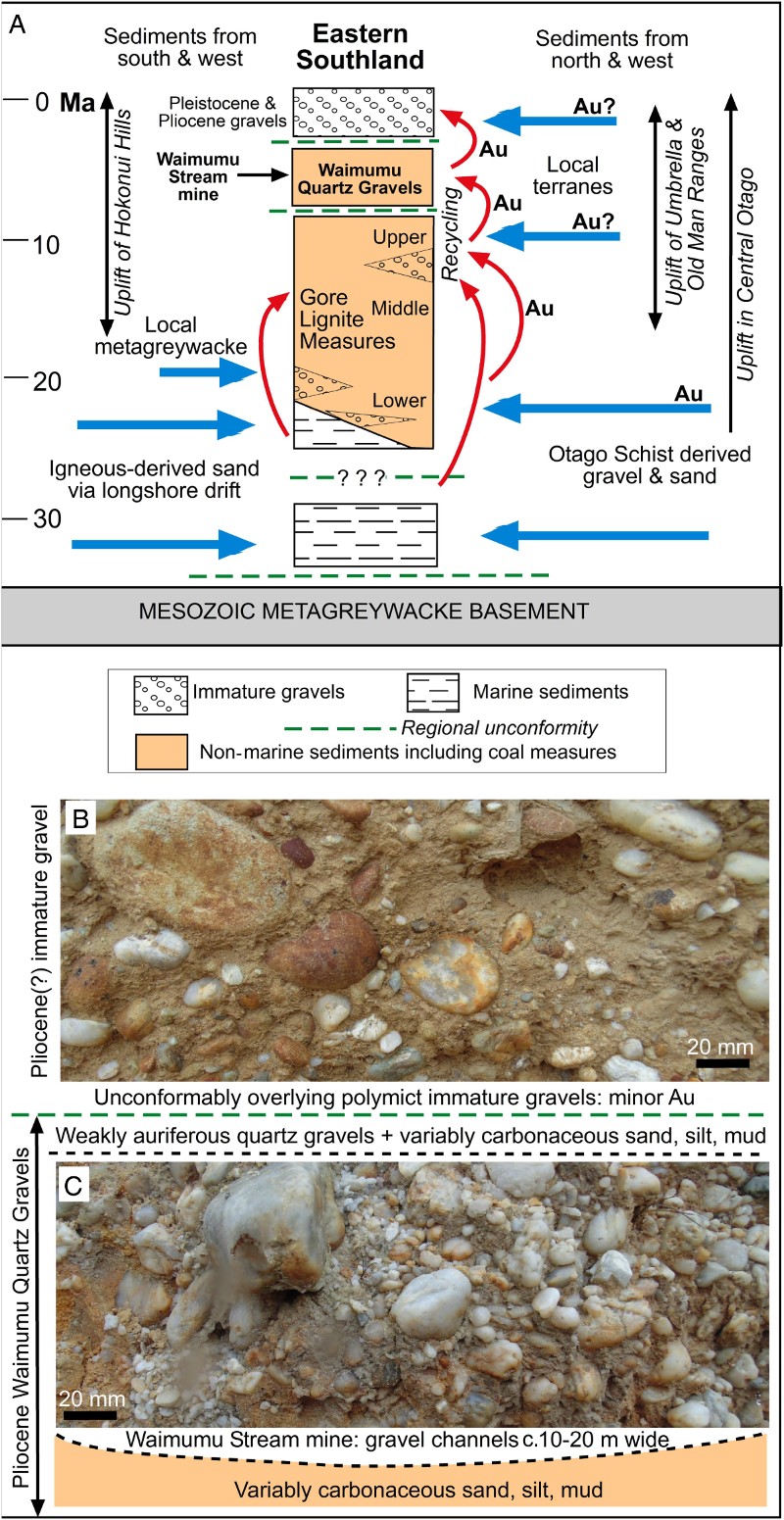
The Pliocene Waimumu Quartz Gravels unconformably overlie the Gore Lignite Measures (A) and this unconformity is locally angular, reflecting the ongoing fault-related deformation of the area (MacPherson Citation1937; Clough & Craw Citation1989; Falconer et al. Citation2006; Youngson et al. Citation2006). The quartz gravels are in turn unconformably overlain by immature Pliocene gravels and a range of immature Pleistocene gravels. These younger gravels are generally dominated by metagreywacke clasts derived from the surrounding ranges, with a variable quartz component (, A, B; Wood Citation1956; Isaac & Lindqvist Citation1990; Turnbull & Allibone Citation2003).
Sedimentary recycling
The high proportion of quartz clasts in the Waimumu Quartz Gravels (C) is a result of sedimentary recycling during erosion of older sediments that have been uplifted on the flanks of rising mountain ranges (Youngson et al. Citation2006). Labile minerals such as micas and feldspars in metagreywacke clasts in early-formed fluvial sediments were altered to clay by post-depositional groundwater interaction (Clough & Craw Citation1989; Youngson et al. Citation2006; Stein et al. Citation2011). As these altered sediments were uplifted, re-exposed and eroded the clay was removed, leaving a quartz-rich residue (Clough & Craw Citation1989; Youngson et al. Citation2006; Stein et al. Citation2011).
This recycling and quartz concentration process was particularly important for formation of the Waimumu Quartz Gravels (C) but has occurred numerous times in the history of the late Cenozoic sedimentation, starting with recycling during formation of the Upper Gore Lignite Measures and continuing to the present (A; Clough & Craw Citation1989; Isaac & Lindqvist Citation1990; Youngson et al. Citation2006). Localised Pliocene erosion of Waimumu Quartz Gravels yielded polymict conglomerates with more distally derived metagreywacke clasts (A). Subsequent alteration and erosion of these deposits yielded quartz-rich sediments again (Clough & Craw Citation1989; Youngson et al. Citation2006), and this process is continuing along active faults in the area.
In addition to recycling of quartz, there has been ongoing recycling of heavy minerals, including gold, that are resistant to the chemical degradation processes that affected labile minerals (A; Clough & Craw Citation1989; Falconer et al. Citation2006; Youngson et al. Citation2006; Stein et al. Citation2011). Heavy minerals from igneous sources such as Fiordland and Stewart Island () were initially incorporated via longshore drift into middle Cenozoic marine sediments, and these have been recycled into younger sediments (A; Clough & Craw Citation1989; Youngson et al. Citation2002; Falconer et al. Citation2006). Likewise, heavy minerals from sources such as the Otago Schist have been recycled into progressively younger sediments (A; Clough & Craw Citation1989; Youngson et al. Citation2002; Falconer et al. Citation2006).
Methods
This study was conducted with observations and material collected from the active Waimumu Stream mine (Grid ref: NZTM 1278986 4884849), which has created fresh exposures of Waimumu Quartz Gravels in an area of generally poor exposure. In addition to gold the mine is extracting quartz pebbles for decorative purposes, so material from the whole exposed section of Waimumu Quartz Gravels is processed rather than just narrow gold-rich zones. This extensive processing is yielding abundant heavy mineral concentrates that are discarded at the mine as impurities, and those concentrates were sampled for the present study. In addition, processing of the quartz gravels has yielded piles of cleaned cobbles and pebbles from which a suite of distinctive and unusual clasts were collected for this study for determining provenance (sample OU85058).
Three groundwater samples were collected where water emanates from the channelised Waimumu Quartz Gravels at the Waimumu Stream mine. These waters had probably also passed through the overlying polymict gravels (B). An additional surface water sample was collected from the adjacent Waimumu Stream for comparison to the groundwater compositions (). Water samples were collected and analysed by methods described in Craw et al. (Citation2015a).
Table 1 Major ion analyses of waters at the Waimumu Stream mine. All values except pH are in ppm (mg L–1); alkalinity (Alk) is presented as
.
Heavy minerals and distinctive clasts were examined with standard petrographic techniques in polished thin-section and via stereoscopic microscopy to identify principal minerals and textures. For this purpose, the internal structure of clasts and the textures of mineral grains within those clasts was of particular interest. A sample of the heavy mineral sand concentrate (OU85059) was further investigated in a carbon-coated polished thin-section using a Zeiss Sigma FEG scanning electron microscope (SEM) with Oxford Instruments XMax 20 Si Drift energy dispersion X-ray detector (EDS) at the Otago Centre for Electron Microscopy, University of Otago. EDS maps of mineral particles were obtained at 15 keV and made over several hours of automated scanning across the whole section. Mineral analyses were performed on the digitised SEM images using the already captured element mapping data, with variable analytical spot diameters from 10 to 50 µm, taking care to avoid inclusions and cracks where possible. Analytical uncertainties (1σ) range from ±0.1 to 0.6 wt% (see Table S1). These analyses focused on spinel and garnet clasts, as they provided the most distinctive compositional variations relevant to our provenance studies. Minor zoning of these minerals was detected, but irregular particle shapes and variable degrees of rounding and edge abrasion precluded detailed definition of zoning patterns.
Waimumu Quartz Gravels at Waimumu Stream mine
Historic mining (late nineteenth century to 1930s) in the Waimumu Stream area initially involved dredge recovery of gold that had been naturally eroded from Waimumu Quartz Gravels into the adjacent modern Waimumu Stream (Falconer et al. Citation2006). Subsequent hydraulic mining occurred in the Waimumu Quartz Gravels themselves, in outcrops immediately above the eastern bank of the modern stream. Historic miners also extracted gold that had been recycled from the Waimumu Quartz Gravels into the immediately overlying polymict Pliocene gravels (B). The historic hydraulic mining, and that of the modern mine, occur in channels of quartz gravel cut into fine-grained carbonaceous sediments (C). The channels are c. 10–20 m wide and trend approximately east–west at a high angle to the modern stream that flows southwards.
The quartz clasts are rounded to subrounded, and range in diameter from 0.5 cm to 10 cm (C). The gravel is clast-supported with a matrix of quartz sand, silt and kaolinite clay. Most clasts are broadly equant pebbles and cobbles of white, massive quartz, commonly with irregular cavities in rounded surfaces where some other mineral has apparently been removed. The internal structure of quartz clasts is dominated by granoblastic anhedral grains, 50–200 µm across, typical of metamorphic quartz. Many clasts are tabular with lineated and rodded mineral alignment on their flat upper and lower surfaces. Tabular clasts typically have a foliation, locally with relict muscovite grains up to 150 µm across. Patches of relict chlorite (centimetre scale) are preserved in some depressions in clast surfaces between quartz rods, although partial dissolution of chlorite also leaves irregular -shaped cavities in some clasts. Red jasper-like clasts are rare but prominent in outcrop and mine products.
Some tabular quartz clasts contain pale-coloured spessartine garnet (A). The spessartine occurs in wispy layers within a relict foliation in these clasts, which is locally complexly folded (A). In addition, one piemontite-bearing quartz clast was found (A). This latter clast is strongly lineated and metamorphically segregated (A). The spessartine- and piemontite-bearing clasts are highly distinctive in the southern South Island context, and these minerals occur in manganese-rich metachert horizons in the upper greenschist facies core of the Otago Schist Belt (, B; Craw Citation1984; Turnbull Citation2000). No other basement outcrops of such distinctive rocks are known in the southern South Island.
Figure 4 Otago Schist metachert. A, Large clasts of upper greenschist facies piemontite and spessartine metachert from the Waimumu Quartz Gravels at Waimumu Stream mine. B, Principal metachert localities in the core of the Otago Schist Belt (; partly after Turnbull Citation2000). Locations of nearby swarms of orogenic gold veins and remnants of Miocene gold-bearing quartz gravels are also indicated . Distance and direction to Waimumu are indicated with blue arrow.
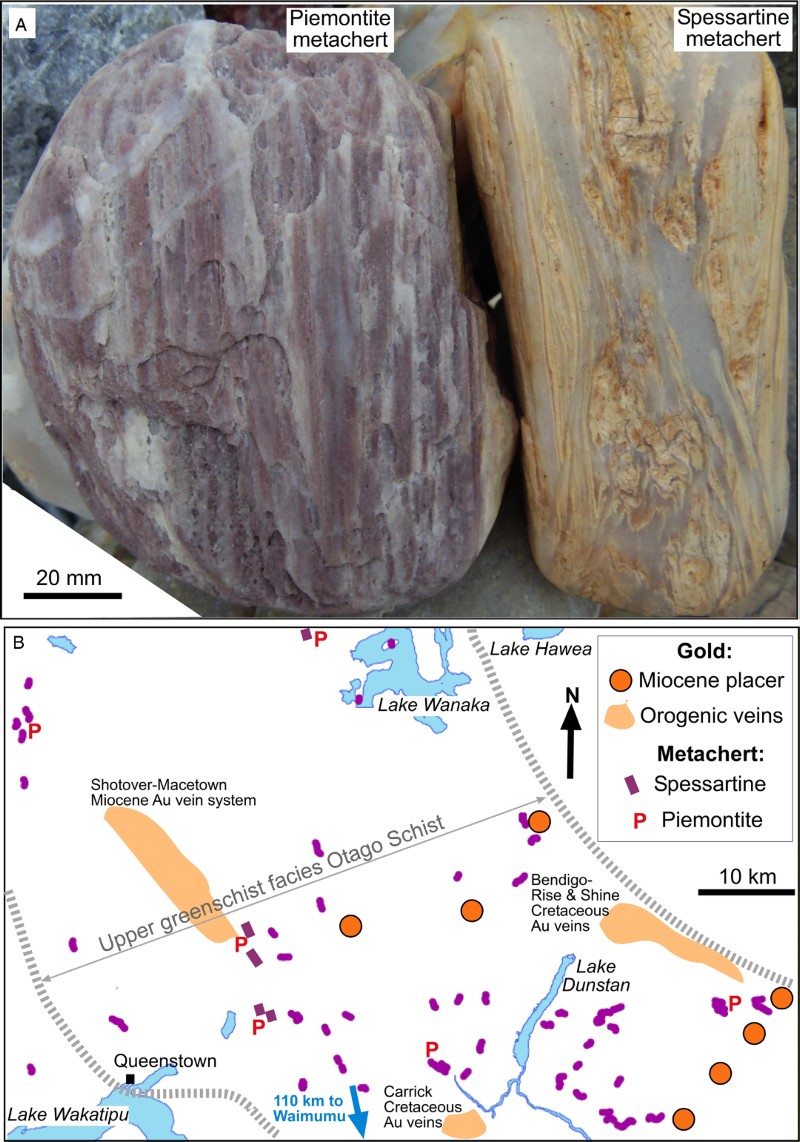
Rare grey quartz clasts are different from the white clasts in having evidence for prismatic quartz crystals (A), locally protruding into open cavities. Some prismatic quartz crystals are clear, or creamy in colour, but most are darkened to some extent by the presence of abundant fluid inclusions and fine-grained mineral inclusions (rutile and pyrite in A). Grey quartz clasts are generally tabular in shape, despite extensive rounding of edges, and some such clasts have weak layering of quartz crystals parallel or subparallel to the flat tabular faces. These clasts are clearly of hydrothermal rather than metamorphic origin, derived from quartz veins in the source region(s).
Figure 5 Heavy minerals from the Waimumu Stream mine. A, Thin-section view (plane polarised light) of a clast (from sample OU85058) consisting of prismatic quartz containing inclusions of dusty rutile (circled) and rare pyrite. Dark seams rich in fluid inclusions define zoning in the quartz crystals. B, Scanning electron microscope image of a polished section of heavy mineral sand concentrate (OU85059), with false-colour overlays reflecting differing elemental compositions. Principal minerals are zircon (Zir), chromite (Chr), garnet (Gt) and ilmenite (Ilm), with small fragments of Mn oxides (purple; indicated with white arrows).
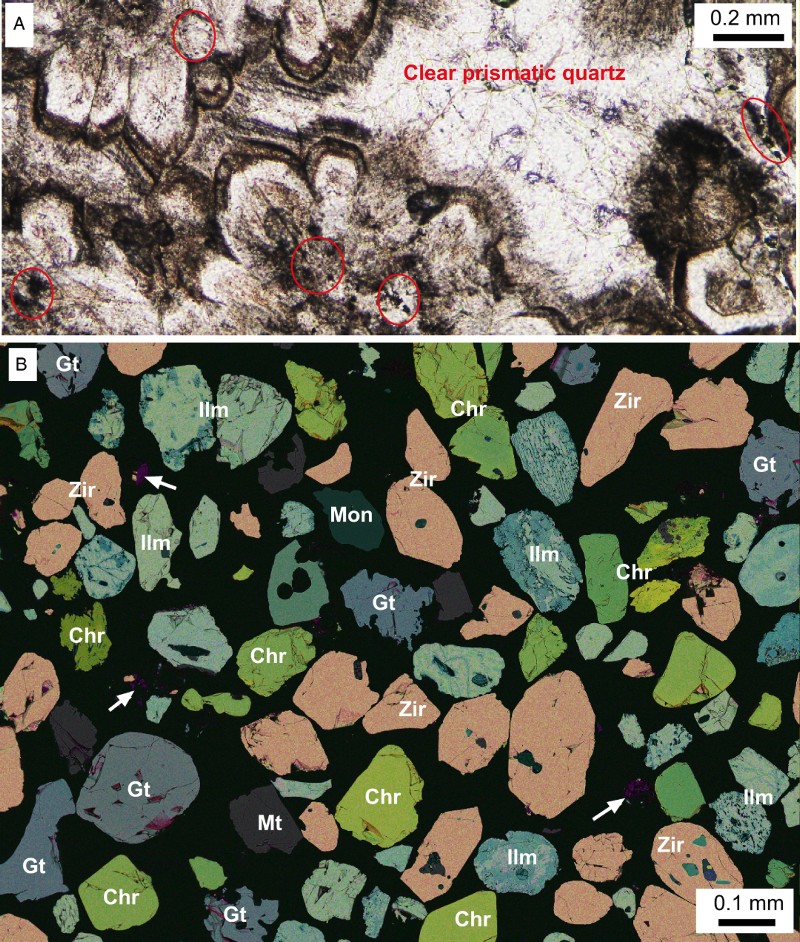
Rounded, smooth cobbles of recycled quartz sediments occur scattered through the quartz gravels, and are most common near to the gold-bearing basal unconformity. These clasts consist of quartz pebbles that have been cemented by quartz, and typically have a brown iron oxyhydroxide coating on their outer surfaces.
Heavy mineral suite
The heavy mineral suite that is separated by the mining process consists of dark-coloured clasts that range in size from very fine sand to medium sand, but are mostly c. 100–200 µm (B). The clasts are subangular to rounded and generally equant, some with remnants of crystal shapes (B). Many clasts have internal fractures and some have broken during transport, possibly including the mine separation process. The suite is dominated by zircons (20–40%) and ilmenite (10–30%), with abundant chromite (10–15%) and garnet (5–10%) and minor magnetite, monazite and Mn oxides (B).
The garnet clasts are generally manganoan and most lie near the centre of the grossular-almandine-spessartine compositional range, with some compositions spreading toward spessartine (A). The latter compositions are predictable from the presence of large spessartine-bearing chert clasts in the gravels (A). The overall garnet compositional range falls mainly within that of typical Otago Schist metamorphic garnet (A, B). However, a few low-Ca garnet clasts fall into the compositional range of almandine and manganoan almandine of high-grade metamorphic rocks of Fiordland and Stewart Island (A, B; Youngson et al. Citation2002).
Figure 6 Garnet compositions in terms of proportions of Ca, Fe2+ and Mn2+ components. A, Compositions of garnet clasts from the Waimumu Quartz Gravels at the Waimumu Stream mine, determined by analytical SEM. These compositions are compared to garnet clasts from nearby Belle-Brook gold mine, also in Waimumu Quartz Gravels (), and regional basement sources (dashed lines, as shown in B). B, Garnet compositions in basement rocks of Fiordland, Stewart Island and Otago Schist, as compiled by Youngson et al. (Citation2002) (and also outlined in A) in comparison to garnet clasts from the Nokomai gold mine ().
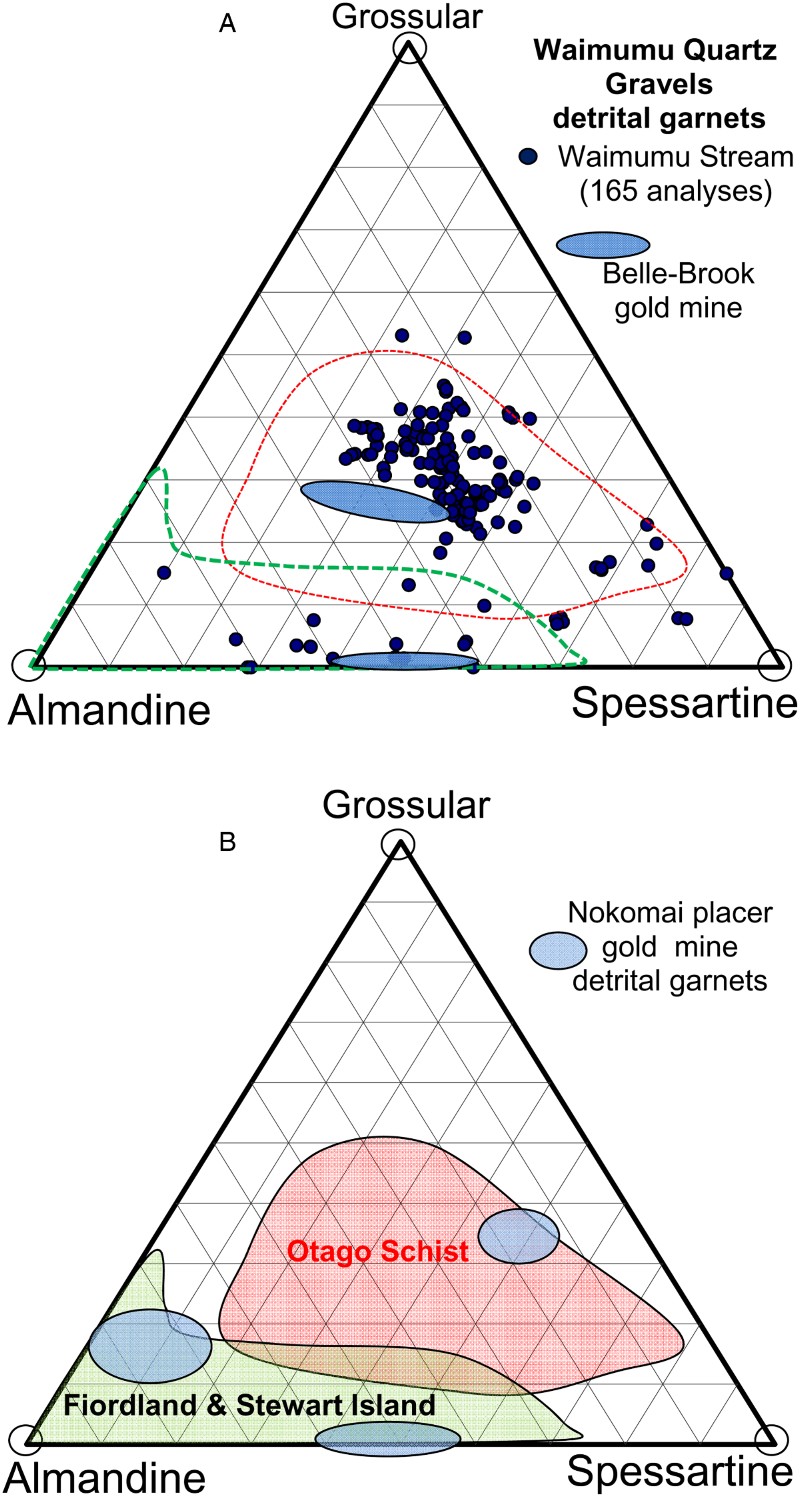
Chromite clasts have high Mg content, with Mg/Fe2+ near to 1 (A). The chromite clasts also have high Al content, with Al/Cr up to 2 (B). Magnetite clasts have negligible Cr content. These chromite clasts compositionally resemble those from the Dun Mountain Ophiolite Belt (C, D; Hyden Citation1980; Sinton Citation1980 ). However, there is some overlap with chromium-bearing spinel compositions in nearby mafic to ultramafic intrusions (C, D). These latter intrusions, of possible Ural-Alaska type, occur within metagreywacke basement (Brook Street Terrane) to the south and west of Waimumu (; Spandler et al. Citation2003; Ashley et al. Citation2012).
Figure 7 Chromium-bearing spinel-group mineral compositions, recalculated to ideal formulae. A, B, Divalent and trivalent cations, respectively, for chromite clasts in Waimumu Quartz Gravels at Waimumu Stream mine, compared to Cr-bearing spinel-group mineral compositions in Dun Mountain Ophiolite Belt and Brook Street terrane rocks, as in C & D. Data in C & D from Hyden (Citation1980) and Sinton (Citation1980).
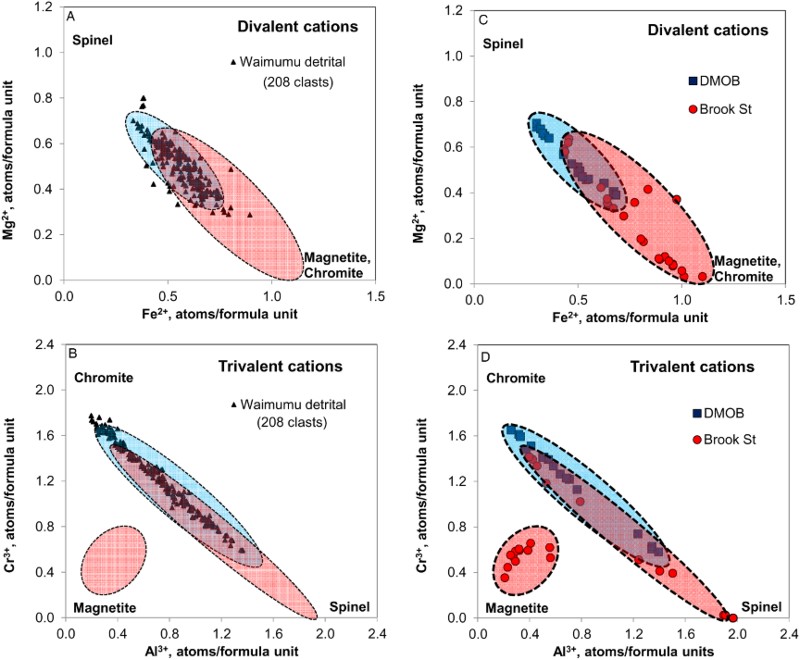
Detrital gold
Nearly all the gold in the quartz gravels occurs as flakes that range from 50 to 700 µm across (A). The flakes are thin (<50 µm) although they are commonly folded and locally thickened by this folding, especially on their rims (A). Some flakes have been folded, refolded and reflattened. One small nugget has been found, highly irregular in shape with local preservation of straight edges of what may be gold crystals at the 100 µm scale (B). The nugget gold is intimately intergrown with clear prismatic quartz that has not been rounded by sedimentary transport, to form a very delicate specimen (B). Either this nugget was derived from an unknown vein source close to Waimumu Stream or the nugget was liberated when a clast of gold-bearing hydrothermal vein quartz (e.g. A, A) broke during transport, possibly during mine processing.
Figure 8 Detrital gold from Waimumu Quartz Gravels at Waimumu Stream mine. A, Detrital flakes (lying flat), with locally folded rims (indicated with red arrows). B , Small nugget with crystalline gold intergrown with prismatic quartz crystals.
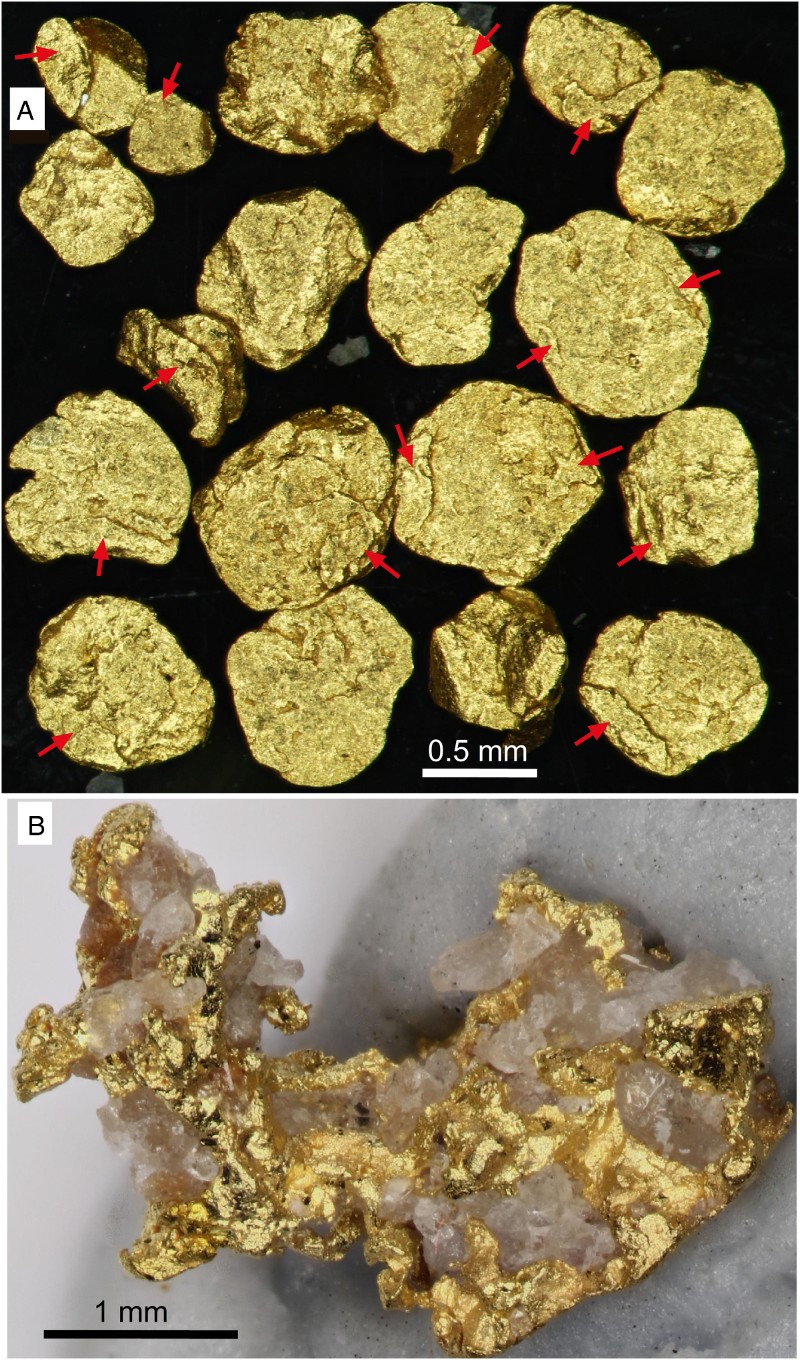
One large grey hydrothermal quartz cobble hosts numerous irregularly shaped gold encrustations on its surface and within cavities exposed to the surface (A–F). The exterior gold encrustations consist of clusters of coarse (30–200 µm) crystals and finer-grained masses, with no evidence for rounding or flattening during sedimentary transport (A–F). Some gold occurs in cavities with prismatic quartz crystals (C), some in recesses in the cobble (B, D) and some on outer surfaces coating rounded quartz (E, F). It is notable that the interior of this quartz clast, as seen on three broken surfaces (A), does not have visible gold.
Figure 9 Gold-bearing quartz clast from Waimumu Quartz Gravels at Waimumu Stream mine. A, View of whole clast, showing variable grey seams in hydrothermal vein quartz. Locations of images B–F are indicated. B, Crystalline gold in a cavity. C, Crystalline gold intergrown with prismatic quartz in a cavity. D, Large gold particle on clast surface, with white detrital kaolinite. E, F, Irregular and rough gold particles on smooth, rounded clast surfaces.
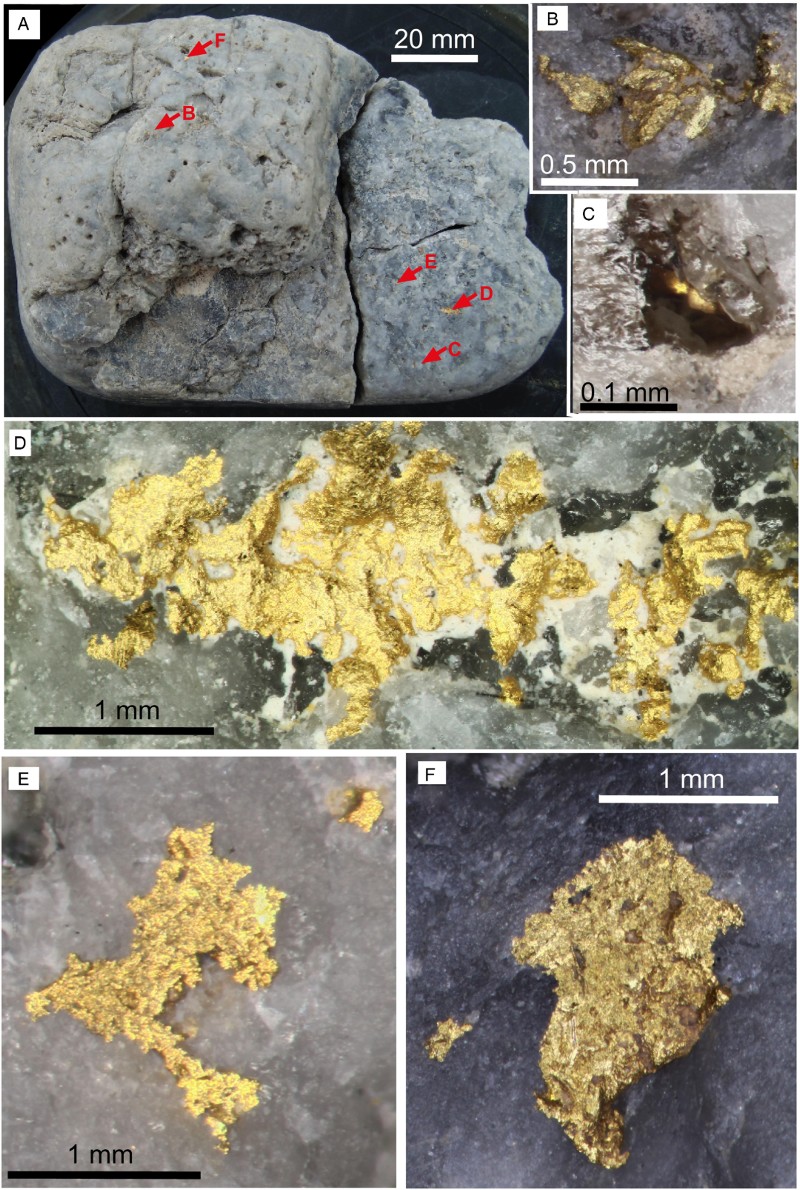
Authigenic gold and marcasite
There is abundant evidence from other mines in Waimumu Quartz Gravels (Parker Road, Belle-Brook; ) for precipitation of authigenic gold after sedimentary deposition (Clough & Craw Citation1989; Falconer et al. Citation2006; Falconer & Craw Citation2009). This process occurred in conjunction with abundant authigenic marcasite precipitation at Belle-Brook mine (Clough & Craw Citation1989). There is also evidence for similar authigenic gold precipitation at the Waimumu Stream mine (Craw Citation1992). In the present study, we specifically sampled the base of a channel at Waimumu Stream mine to identify key components of the authigenic gold precipitation system (A–E).
Figure 10 Authigenic minerals in Waimumu Quartz Gravels at Waimumu Stream mine. A, Outcrop view of quartz gravels in the base of a channel, with a large woody clast at the base. B, Authigenic marcasite in the woody material shown in A. C, Sphere of authigenic marcasite in a heavy mineral concentrate from the quartz gravels. D, SEM electron backscatter image of a folded rim of a detrital gold flake, extracted from the base of the channel in A. Authigenic gold plates coat the detrital flake surface. E, Authigenic gold protrusions partially fill a cavity in the flake in D.
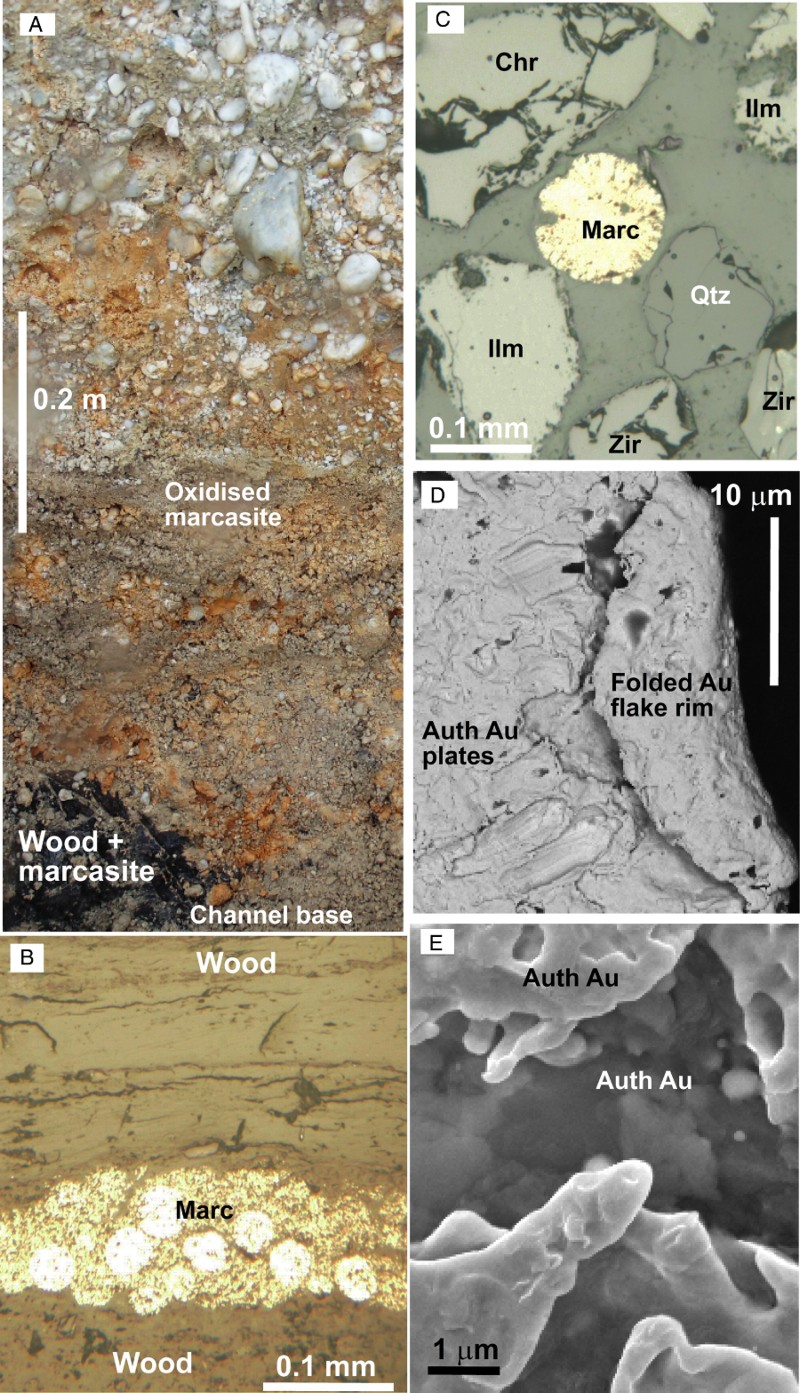
Authigenic marcasite is a minor component of the quartz gravels near the base of the sampled channel, and mainly occurs as spherical clusters of grains (B, C). These clusters have formed in the matrix of the quartz gravels, where they are now being oxidised by groundwater to form rusty stains in the lower part of the channel (A, C). In addition, marcasite has formed within and adjacent to woody material hosted in the quartz gravels at the base of the channel (A, B).
Gold flakes from the quartz gravels (e.g. A) all have slightly rough surfaces at the 10 µm scale, with some globular amorphous gold protrusions and irregular gold plates. Gold collected by panning from the least oxidised rocks at the base of the channel, adjacent to the marcasitic woody material, has some clearly defined elongate crystal plates, as well as thin irregular overgrowths (D). In addition, cavities within the flake surfaces, especially adjacent to folded rims (D), have fine-grained (micron-scale) irregularly shaped overgrowths, some with spheroidal or globular bud-like forms (E) similar to those seen at Parker Road and Belle-Brook mines (Falconer et al. Citation2006; Falconer & Craw Citation2009).
Groundwater from the gold-bearing channel (A; ) was sampled to quantify the general geochemical system in which the authigenic gold occurs. The resultant water compositions are typical of nearby Southland groundwater, in that they are dominated by Na and Cl from marine aerosols in rain (Craw et al. Citation2015a). This seawater component typically contributes dissolved sulphate in a proportion that is c. 5% of the seawater Cl (Craw & Beckett Citation2004). The observed Na and Cl contents imply that c. 1 ppm of the dissolved sulphate comes from marine aerosols (). The moderate sulphate contents of the groundwater samples (up to 9 ppm; ) presumably come mainly from oxidation of sulphide minerals in the rocks, including the authigenic marcasite (A). The pH is weakly alkaline (c. 7.5) in all waters, suggesting that any acid generated by sulphide oxidation has been neutralised by the high calcite-generated alkalinity (), possibly derived from the overlying greywacke-bearing polymict gravels (B). The groundwater compositions are very similar to the Waimumu Stream surface water () that has also been in contact with calcite-bearing and sulphide-bearing rocks ().
Discussion
Ultimate source: the Otago Schist
Textures and mineralogy of quartz clasts in the Waimumu Quartz Gravels at Waimumu Stream mine strongly support ultimate derivation of much of the sediment from the Otago Schist Belt to the north (). The 1–10 cm size of most of the metamorphic quartz fragments suggest derivation from highly segregated schist in the core of the schist belt and this is supported by the presence of coarse-grained (>100 µm) muscovite grains and centimetre-scale chlorite segregations (Turnbull et al. Citation2001). The distinctive Mn-rich metachert clasts (A) also support derivation from the core of the schist belt (B). Likewise, most of the garnet clasts in the quartz gravels were derived from the schist belt (A, B). The highly flattened and folded gold flakes attest to long-distance transport, on the order of tens to hundreds of kilometres (Youngson & Craw Citation1999). Derivation of most of the gold from Otago Schist hydrothermal vein systems (, B) is therefore highly likely.
Despite the strong petrographic evidence for an Otago Schist source for the clasts in the quartz gravels, direct transport of this material from Central Otago in the Pliocene is unlikely. Intervening mountain ranges began rising in the middle Miocene, cutting off the direct drainage system from Otago to Southland that was initiated in the late Oligocene and early Miocene (; Isaac & Lindqvist Citation1990; Stein et al. Citation2011; Craw Citation2013). Erosion during the Miocene uplift shed debris southwards from the rising intervening ranges (Old Man and Umbrella ranges; , ) to contribute coarse gravel debris to the Upper Gore Lignite Measures (Isaac & Lindqvist Citation1990). Ongoing uplift and recycling of this debris occurred in the Pliocene, on the flanks of the Old Man and Umbrella ranges, and also the Hokonui Hills nearer to Waimumu (). This recycling, combined with disaggregation of clay-altered lithic clasts, led to formation of the Waimumu Quartz Gravels (Clough & Craw Citation1989; Youngson et al. Citation2006). Because the debris from the Otago Schist was quartz-rich, it was largely chemically resilient so this material remained to dominate the resultant deposits. While many of the clasts in the Waimumu Quartz Gravels were derived from distant sources, the actual transport distance of the Waimumu Quartz Gravels from their immediate sources of pre-existing sediments may therefore be small as indicated in .
Figure 11 Hillshade regional map showing topography for a similar area to , and the principal modern rivers. Inferred palaeodrainage directions relevant to the Waimumu Quartz Gravels are indicated (see text).
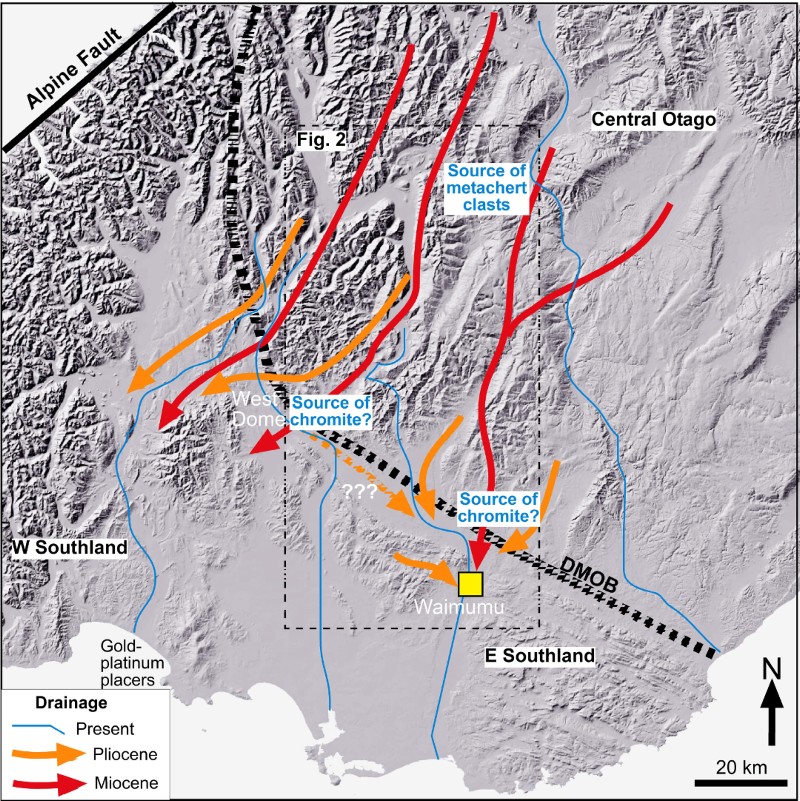
Garnet clasts derived from high-grade metamorphic rocks of Fiordland and/or Stewart Island are relatively rare in the quartz gravels at Waimumu Stream mine, compared to the abundance of garnet clasts derived from the Otago Schist (A, B). High-grade, mainly almandine-rich, garnet clasts from Fiordland and Stewart Island occur in middle Cenozoic marine sediments (A), and have since been recycled into various younger sediments in Southland including the Gore Lignite Measures. These garnet clasts from the south and west appear in heavy mineral concentrates in other Southland gold mines, including the Waimumu Quartz Gravels at Belle-Brook and Pleistocene gravels at Nokomai to the northwest (A, B; Youngson et al. Citation2002; Falconer et al. Citation2006). The paucity of high-grade garnet clasts at Waimumu Stream mine suggests that the marine sediments that constitute their principal local source, via recycling, had already been eroded before deposition of the Waimumu Quartz Gravels. Likewise, since high-grade garnet clasts are common in the Gore Lignite Measures, the Gore Lignite Measures may also have been eroded before deposition of the Waimumu Quartz Gravels at Waimumu Stream.
Source and transport of chromite
Chromite is relatively rare in southern South Island basement rocks and is found in only a few mafic to ultramafic bodies. Despite this regional rarity, chromite forms a surprisingly high proportion of the heavy mineral concentrate at the Waimumu Stream mine (B). Chromite does not occur in the Otago Schist, where the few constituent ultramafic rocks have been extensively metasomatised with associated replacement of chromite to magnetite, serpentine and/or fuchsite (Cooper Citation1976; Craw Citation1984). Rare detrital chromite occurs in Gore Lignite Measures, possibly derived from detrital chromite in nearby metagreywacke clasts that have decomposed after clay alteration (Falconer et al. Citation2006; Stein et al. Citation2011). However, no concentrations of chromite are known in these older rocks, which could have formed a local source for the Waimumu Stream chromite.
Chromium-bearing spinel group minerals with a wide range of compositions occur in mafic-ultramafic intrusions in the Brook Street Terrane to the south and southwest of Waimumu (, A–D; Spandler et al. Citation2003; Ashley et al. Citation2012). The Waimumu Stream chromite clasts have a relatively tight compositional cluster that overlaps only part of the range of Brook Street Terrane Cr-bearing spinel group minerals (A–D), suggesting that the Brook Street Terrane is not the source of the chromite clasts. Further, it would require significant north- to north-eastwards transport to carry Brook Street Terrane spinel clasts to the Waimumu area, a process that only occurred during marine incursion in the Oligocene (A). While this may have contributed to the scattered chromite in the Gore Lignite Measures (Falconer et al. Citation2006), it is unlikely to have produced a localised concentration in recycled sediments that became the Waimumu Quartz Gravels at Waimumu Stream, but not in other parts of the Waimumu Quartz Gravels. The comparative rarity in Waimumu Stream quartz gravels of high metamorphic grade garnet clasts (, A, B) also suggests that derivation of other heavy minerals from the south and southwest is unlikely.
The other known regional source of chromite is the Dun Mountain Ophiolite Belt, although that mineral is rare in current outcrops and is restricted to remnants of peridotite (). Chromite is abundant at Red Mountain in the far northwest (; Sinton Citation1980); this area currently drains to the west however, and may not even have been exposed in the Miocene or Pliocene in the early stages of uplift of the mountains along the Alpine Fault. The nearest known Dun Mountain Ophiolite Belt outcrop of chromite to Waimumu is at West Dome (, ; Coombs et al. Citation1976; Hyden Citation1980). Most of the chromite-bearing peridotite in that locality has been altered to magnetite and serpentine, so chromite is rare (Hyden Citation1980). However, the compositional range of Waimumu Stream chromite clasts is very similar to that at known Dun Mountain Ophiolite Belt localities (A–D). Hence, we conclude that the chromite clasts at Waimumu Stream were derived from the ophiolite belt.
Miocene and Pliocene drainage from the Southern Alps in northwest Otago was primarily toward the southwest (; Craw et al. Citation2012; Upton et al. Citation2014). This southwestwards drainage system has been inferred to have transported gold and platinum from the Dun Mountain Ophiolite Belt into Western Southland (; Craw et al. Citation2013). Hence, Pliocene transport of chromite southeast from West Dome to Waimumu () appears unlikely, although not impossible. Given the rarity of chromite at West Dome, it is also unlikely that high concentrations of chromite could be transported c. 80 km without undergoing dilution by input of heavy minerals in sediments derived from the uplifting ranges to the north and south of that transport path, including metagreywacke basement and overlying Cenozoic sediments (). While we cannot completely discount West Dome area as a source for the chromite, we therefore consider it implausible. An alternative possibility, which we prefer, is that Miocene and Pliocene uplift of the Dun Mountain Ophiolite Belt on the southern slopes of what is now the Umbrella Range immediately north of Waimumu () may have exposed chromite-bearing ultramafic rocks. These rocks may have since been completely removed by erosion or fault excision, or have been covered with younger material at the base of the rising mountains (). These hypothetical rocks could have provided a nearby source for the chromite that was localised at Waimumu Stream ().
Authigenic gold mobility
The close association between authigenic marcasite and authigenic gold mobilisation that has been observed elsewhere in the Waimumu Quartz Gravels (Clough & Craw Citation1989; Falconer et al. Citation2006) also appears to have developed at Waimumu Stream (A–E). This association implies gold mobility under reduced conditions, as would be expected in a channel containing woody material and incised into carbonaceous sediments (, A). Dissolved sulphur in groundwater is thought to have fed the marcasite precipitation, facilitated by bacterial reduction (Falconer et al. Citation2006). Present groundwater has dissolved sulphur (currently as sulphate) up to 9 ppm, which is typical of Southland groundwater compositions (Craw et al. Citation2015a). Gold is soluble in reduced sulphur-bearing waters as bisulphide complexes at near-neutral pH, and as thiosuphate-bearing waters derived from oxidation of sulphides (Webster Citation1986; Heinrich Citation2015). The marcasite-forming environment and also the marcasite-oxidation environment (A) were therefore conducive to gold mobilisation and reprecipitation, again possibly mediated by bacteria (Reith et al. Citation2012).
Textures of gold encrustations on a quartz vein sample from the Waimumu Stream mine (A–F) may give an indication of scale of gold mobilisation. Some of the gold in this specimen may have a primary hydrothermal origin, or undergone subsequent supergene enrichment at source (Craw et al. Citation2015b). The gold crystals in cavities in this specimen, especially those associated with prismatic quartz (B, C) could have travelled with the clast from a distant source (e.g. Central Otago; ). However, some of the gold adheres to rounded exterior surfaces of the clast, yet is apparently undeformed (E, F). This latter gold may have been added after sedimentary deposition, in a similar manner to authigenic gold reprecipitation described above (D, E). The close spatial relationships between these different gold textures on an unusually gold-rich clast suggest that there is a genetic link between them. From these observations, we infer that gold could have been chemically mobilised and then reprecipitated on the same clast, on a scale of centimetres (A).
Conclusions
Most of the clasts in the Pliocene Waimumu Quartz Gravels were derived primarily from the Otago Schist Belt, particularly the highest grade (upper greenschist facies) core of the belt. Some cobbles, and the heavy mineral suite, at the Waimumu Stream mine are distinctive and/or different from those at other studied localities for Waimumu Quartz Gravels. Distinctive metachert cobbles with spessartine and piemontite were probably derived from the northwestern part of Central Otago, between Clyde and Queenstown. Abundant Mn-rich garnet clasts in the heavy mineral sand fraction also support this Otago Schist provenance. Gold occurs in this source area as auriferous quartz veins and as detrital particles in Miocene quartz gravels. The gold at Waimumu Stream was therefore probably mainly derived from Central Otago and transported with the quartz clasts. Detrital gold at Waimumu Stream is mostly highly flattened and flaky, consistent with long-distance (>100 km) transport implied by the quartz and garnet clasts.
The long-distance fluvial drainage link between Central Otago and Southland was severed in the Miocene by uplift of mountain ranges, dominated by greywacke and low-grade schist, across that palaeodrainage system. The fluvial system that led to the channelised Waimumu Quartz Gravels may therefore have been short, related to localised uplift in the Pliocene with associated recycling of older sediments. The high proportion of quartz (>90%) in the Waimumu Quartz Gravels has resulted from these recycling processes. Pre-existing (Miocene?) sediments with lithic clasts became clay-altered after deposition so that only quartz-rich clasts survived Pliocene recycling, while the clay component was mainly transported further away.
Clasts of hydrothermal quartz occur in the Waimumu Quartz Gravels at Waimumu Stream mine, some with attached gold that is locally crystalline and angular. It is possible that this gold was derived from a local source nearer than Central Otago, although no such source is known. Alternatively, this gold may have been transported in clasts of quartz vein material that have broken up to liberate the gold, either during the recycling process in the Pliocene or in the modern gold mine processing system. In addition, there is evidence for authigenic gold mobility and deposition associated with detrital gold flakes that has also led to irregular and rough gold particle shapes, and authigenic gold deposition may have occurred on the outside of one rounded quartz clast. The gold mobility and redeposition may be related to deposition and/or oxidation of authigenic marcasite that has formed in and near carbonaceous debris in the quartz gravels.
Chromite is a distinctive, anomalous and abundant component (>10%) of the heavy mineral sand fraction of Waimumu Quartz Gravels at the Waimumu Stream mine. The compositions of the chromite clasts resembles chromite from the Dun Mountain Ophiolite Belt to the northwest, although there is some compositional overlap with chromite from Brook Street Terrane rocks to the south and southwest. The lack of garnet clasts indicative of sedimentary source from high metamorphic grade rocks to the south or southwest helps to preclude the Brook Street Terrane as a source of the chromite. The nearest known chromite-bearing outcrops in the Dun Mountain Ophiolite Belt are at West Dome, c. 80 km to the northwest of Waimumu. Previous research suggests that drainage past West Dome was towards the southwest during the Pliocene, not to the southeast. Derivation of the Waimumu detrital chromite from West Dome is therefore unlikely and a nearby but unknown source, possibly now eroded or buried by sediments, is inferred instead. The source and transport of the abundant detrital chromite at Waimumu has yet to be fully resolved.
Supplementary data
Table S1. SEM-EDS analyses of detrital minerals from the Waimumu Quartz Gravels at the Waimumu Stream mine, Southland. Typical 1σ uncertainties are indicated. Location: Grid ref NZTM 1278986 4884849. Sample: Placer gold mine processing system, heavy mineral concentrate. Sample archive: University of Otago, OU85059.
Acknowledgements
The study would have been impossible without the enthusiasm and logistical support of Tony Baker and Shane Le Masurier of Nomad Mining. Dave Prior provided expert help with SEM data gathering and manipulation. Able technical support was provided by Lisa Craw, Brent Pooley, Alice Waterman and Damian Walls. Discussions with John Smith, JohnYoungson and Noel Becker were helpful in developing our ideas on Southland placer deposits. Thorough reviews by Mark Rattenbury and an anonymous referee improved the presentation.
Associate Editor: Dr Kari Bassett.
Funding
This research was funded by the Marsden Fund administered by the Royal Society of New Zealand, New Zealand Ministry of Business, Innovation and Employment and the University of Otago.
Disclosure statement
No potential conflict of interest was reported by the authors.
References
- Ashley P, Craw D, MacKenzie D, Rombouts M, Reay A 2012. Mafic and ultramafic rocks, and platinum mineralisation potential, in the Longwood Range, Southland, New Zealand. New Zealand Journal of Geology & Geophysics 55: 3–19. doi: 10.1080/00288306.2011.623302
- Boyle RW 1979. The geochemistry of gold and its deposits. Geological Survey of Canada Bulletin 280. 579 p.
- Cawood PA 1986. Stratigraphic and structural relations of the southern Dun Mountain Ophiolite Belt and enclosing strata, northwestern Southland, New Zealand. New Zealand Journal of Geology & Geophysics 29: 179–203. doi: 10.1080/00288306.1986.10427534
- Clough DM, Craw D 1989. Authigenic gold-marcasite association- evidence for nugget growth by chemical accretion in fluvial gravels, Southland, New Zealand. Economic Geology 84: 953–958. doi: 10.2113/gsecongeo.84.4.953
- Coombs DS, Landis CA, Norris RJ, Sinton JM, Borns DJ, Craw D 1976. The Dun Mountain Ophiolite Belt, New Zealand. American Journal of Science 276: 561–603. doi: 10.2475/ajs.276.5.561
- Cooper AF 1976. Concentrically zoned ultramafic pods from the Haast Schist Zone, South Island, New Zealand. New Zealand Journal of Geology & Geophysics 19: 603–623. doi: 10.1080/00288306.1976.10426310
- Craw D 1984. Lithologic variations in Otago Schist, Mt. Aspiring area, northwest Otago, New Zealand. New Zealand Journal of Geology and Geophysics 27: 151–166. doi: 10.1080/00288306.1984.10422524
- Craw D 1992. Growth of alluvial gold particles by chemical accretion and reprecipitation, Waimumu, New Zealand. New Zealand Journal of Geology & Geophysics 35: 157–164. doi: 10.1080/00288306.1992.9514510
- Craw D 2013. River drainage reorientation during placer gold accumulation, southern New Zealand. Mineralium Deposita 48: 841–860. doi: 10.1007/s00126-013-0464-5
- Craw D, Beckett S 2004. Water and sediment chemistry of Sutton Salt Lake, east Otago, New Zealand. New Zealand Journal of Marine and Freshwater Research 38: 315–328. doi: 10.1080/00288330.2004.9517240
- Craw D, Falconer D, Youngson JH 2003. Environmental arsenopyrite stability and dissolution: theory, experiment, and field observations. Chemical Geology 199: 71–82. doi: 10.1016/S0009-2541(03)00117-7
- Craw D, Kerr G, Reith F, Falconer D 2015a. Pleistocene paleodrainage and placer gold redistribution, western Southland, New Zealand. New Zealand Journal of Geology and Geophysics 58: 137–153. doi: 10.1080/00288306.2015.1007462
- Craw D, MacKenzie DJ, Grieve P 2015b. Supergene gold mobility in orogenic gold deposits, Otago Schist, New Zealand. New Zealand Journal of Geology & Geophysics 58: 123–136. doi: 10.1080/00288306.2014.997746
- Craw D, Mitchell M, McCann R, Reay A 2013. Compositional variations and morphological evolution in platinum beach placers, southern New Zealand. Mineralium Deposita 48: 81–97. doi: 10.1007/s00126-012-0417-4
- Craw D, Upton P, Walcott R, Burridge C, Waters J 2012. Tectonic controls on the evolution of the Clutha River catchment, New Zealand. New Zealand Journal of Geology & Geophysics 55: 345–359. doi: 10.1080/00288306.2012.709184
- Falconer DM, Craw D 2009. Supergene gold mobility: a textural and geochemical study from gold placers in southern New Zealand. Economic Geology Special Publication 14: 77–93.
- Falconer DM, Craw D, Youngson JH, Faure K 2006. Gold and sulphide minerals in Tertiary quartz pebble conglomerate gold placers, Southland, New Zealand. Ore Geology Reviews 28: 525–545. doi: 10.1016/j.oregeorev.2005.03.009
- Garnett RHW, Bassett NC 2005. Placer Deposits. Economic Geology 100th Anniversary Volume: 813–843.
- Heinrich CA 2015. Witwatersrand gold deposits formed by volcanic rain, anoxic rivers and Archaean life. Nature Geoscience. doi:10.1038/NGEO2344.
- Hyden G 1980. Geology of the west dome sector of the Dun mountain ophiolite belt. Unpublished PhD thesis, Dunedin, New Zealand, University of Otago. 178 p.
- Isaac M, Lindqvist JK 1990. Geology and lignite resources of the Eastern Southland Group. New Zealand, New Zealand Geological Survey Bulletin 101. 202 p.
- MacPherson EO 1937. The geology of the Waimumu alluvial goldfield and notes on quartz conglomerates of Southland. New Zealand Journal of Science & Technology 18: 772–778.
- Reith F, Stewart L, Wakelin SA 2012. Supergene gold transformation: secondary and nanoparticulate gold from southern New Zealand. Chemical Geology 320: 32–45. doi: 10.1016/j.chemgeo.2012.05.021
- Sinton JM 1980. Equilibration history of the basal Alpine-type peridotite, Red Mountain, New Zealand. Journal of Petrology 18: 216–246. doi: 10.1093/petrology/18.2.216
- Spandler C, Arculus RJ, Eggins SM, Mavrogenes JA, Price RC, Reay AJ 2003. Petrogenesis of the Greenhills Complex, Southland, New Zealand: magmatic differentiation and cumulate formation at the roots of a Permian island-arc volcano. Contributions to Mineralogy and Petrology 144: 703–721. doi: 10.1007/s00410-002-0424-z
- Stein J, Craw D, Pope J 2011. Initial sedimentation and subsequent diagenesis in the Eastern Southland Lignite Basin, southern New Zealand. New Zealand Journal of Geology & Geophysics 54: 167–180. doi: 10.1080/00288306.2010.515599
- Turnbull IM 2000. Geology of the Wakatipu area. Institute of Geological & Nuclear Sciences 1:250 000 geological map 18. 1 sheet + 72p. Lower Hutt, Institute of Geological and Nuclear Sciences.
- Turnbull IM, Allibone AH 2003. Geology of the Murihiku Area: Institute of Geological & Nuclear Sciences 1:250 000 geological map 20, Institute of Geological & Nuclear Sciences Limited, Lower Hutt, New Zealand.
- Turnbull IM, Mortimer N, Craw D 2001. Textural zones in the Haast Schist: a reappraisal. New Zealand Journal of Geology and Geophysics 44: 171–183. doi: 10.1080/00288306.2001.9514933
- Upton P, Craw D, Walcott R 2014. Far-field deformation resulting from rheologic differences interacting with tectonic stresses: An example from the Pacific/Australian plate boundary in southern New Zealand. Geosciences 4: 93–113. doi: 10.3390/geosciences4030093
- Webster JG 1986. The solubility of Au and Ag in the system Au–Ag–S–O2–H2O at 25 °C and 1 atm. Geochimica et Cosmochimica Acta 50: 245–255. doi: 10.1016/0016-7037(86)90242-5
- Williams GJ 1974. Economic geology of New Zealand. Australasian Institute of Mining and Metallurgy Monograph 4.
- Wood BL 1956. The geology of the Gore Subdivision. New Zealand Geological Survey Bulletin 53. 129 p.
- Youngson JH, Craw D 1993. Gold nugget growth during tectonically induced sedimentary recycling, Otago, New Zealand. Sedimentary Geology 84: 71–88. doi: 10.1016/0037-0738(93)90046-8
- Youngson JH, Craw D 1999. Variation in placer style, gold morphology, and gold particle behavior down gravel bed-load rivers: an example from the Shotover/Arrow-Kawarau-Clutha river system, Otago, New Zealand. Economic Geology 94: 615–633. doi: 10.2113/gsecongeo.94.5.615
- Youngson JH, Craw D, Falconer DM 2006. Evolution of Cretaceous-Cenozoic quartz pebble conglomerate gold placers during basin formation and inversion, southern New Zealand. Ore Geology Reviews 28: 451–474. doi: 10.1016/j.oregeorev.2005.02.004
- Youngson JH, Wopereis P, Kerr LC, Craw D 2002. Au-Ag-Hg and Au-Ag alloys in Nokomai and Nevis valley placers, northern Southland and Central Otago, New Zealand, and their implications for placer-source relationships. New Zealand Journal of Geology & Geophysics 45: 53–69. doi: 10.1080/00288306.2002.9514959