ABSTRACT
Volcaniclastics hosting accretionary lapilli on the Tonga Ridge were sourced from the remnant Lau Ridge, prior to Lau back-arc basin opening. For the ‘Eua occurrences, an atypical dispersal distance of not less than 70 km is estimated, partly arising from the anomalous easterly position of ‘Eua. Dispersal within ocean-surface pyroclastic density currents is supported but strike-slip movement in a fault zone south of ‘Eua, post-middle Miocene but pre-ridge-splitting, can account for part of the dispersal distance by vertical axis block rotation, a tectonic process common on the southern Tonga–Kermadec–Hikurangi trend. In this model, the volcano which sourced the ‘Eua tephra was on a subjacent block, rather than the block which hosts ‘Eua. After deposition but prior to the opening of the Lau Basin, the accretionary lapilli on ‘Eua became displaced by block rotation c. 40 km towards the Tonga trench and away from source.
Introduction
Accretionary lapilli are highly ordered types of ash aggregate typically associated with explosive eruptions, where they may form in the plume itself or in pyroclastic density currents as they interact with the co-ignimbrite ash plume. Dispersal may therefore occur sub-aerially by expansion of the plume, spreading of any atmospheric umbrella cloud and/or by pyroclastic density currents. The ‘Eua accretionary lapilli contain examples typically 10–15 mm in diameter and are accretionary lapilli sensu stricto (Brown et al. Citation2010; Van Eaton & Wilson Citation2013), as distinguished from less-ordered ash pellets and fragile ash aggregates (Brazier et al. Citation1982; Carey & Sigurdsson Citation1982; Wiesner et al. Citation1995; Brown et al. Citation2012) which rarely survive in that form but are detected in sieve analysis of grain size. Accretionary lapilli have been reported from Miocene volcaniclastics which are exposed on the Nomuka group islands (Ballance et al. Citation2004) and on ‘Eua on the Tonga Ridge (Ballance et al. Citation2004; Cunningham & Beard Citation2014). The host volcaniclastics were sourced from volcanoes on the Lau Ridge, prior to the splitting of the Lau–Tonga ancestral arc in the latest late Miocene to form the Lau back-arc basin (Cole et al. Citation1985; Clift et al. Citation1994, Citation1995, Citation1998; Parson & Wright Citation1996). Reconstruction of the ancestral ridge places the Nomuka group islands, which are positioned on the west of the Tonga Ridge, at modest dispersal distances from potential source. However, ‘Eua is the most easterly of the island exposures along the Tonga Ridge by some margin and this contributes to a dispersal distance from source at the limit of most (but not all) documented occurrences of accretionary lapilli. Resolving the two problems presented by the anomalous position of ‘Eua and the exceptional distance from potential source of the accretionary lapilli found on ‘Eua is the focus of this paper. The approach taken to address the two problems is firstly to use data from the Tonga Ridge, the Lau Basin and the Lau Ridge to constrain possible locations for the middle Miocene source volcano which provided the accretionary lapilli on ‘Eua, to consider how the distance between source and destination may have been impacted by post-middle Miocene tectonics including block rotation, and to estimate the minimum actual contemporary distance from source. The paper then examines constraints on possible maximum dispersal distances for the relatively large accretionary lapilli from ‘Eua. Discussion is then focused on whether the anomalous position of ‘Eua and the unusual dispersal distance of the ‘Eua accretionary lapilli from source can be explained by block rotation within the Tonga microplate and/or a dispersal distance enabled by a pyroclastic density current which traversed the ocean surface before depositing the accretionary lapilli on ‘Eua.
Regional setting
Located on the northern part of the Hikurangi–Kermadec–Tonga trend, the Tonga and Lau ridges are dominantly open marine as delineated by the 2000 m contour (A). A number of islands occur; some are large but most are barely emergent and exposures are limited. An exception is the island of ‘Eua on the Tonga Ridge, where an uplifted Eocene basement high and overlying sediments are now exposed sub-aerially. These sediments include deep-marine middle Miocene volcaniclastics. More is known of the Tonga Ridge (B) than the remnant Lau Ridge. Oil industry activity, including five exploration wells on Tongatapu, proved that a deep basin of sediment overlays a presumed volcanic arc basement on the north–central part of the Tonga Platform (Cunningham & Anscombe Citation1985). Scientific cruises (Scholl & Vallier Citation1985; Stevenson et al. Citation1994) confirmed this frontal arc basin extended south and established that the present Tonga Ridge is broken into a number of fault-delineated blocks (B). On the southern platform, depocentres are identifiable on the west of the basin on isopach A–B (which includes the Miocene), with the sediments thickening generally towards the west. Herzer & Exon (Citation1985) suspected that their alignment along the west side of the basin indicated these sediment ‘thicks’ were fed from volcanic centres ‘nearby to the west, outside the mapped area’. The Lau Ridge bathymetry is very similar to the Tonga Ridge, broadly outlined by the 2000 m contour (A), but many more islands with a dominantly volcanic aspect control the geology (Woodhall Citation1985). Basement rocks are not exposed in the islands; the oldest rocks exposed are middle Miocene but volcanism occurred during 14.0–2.5 Ma, so older geology would have been obscured on volcanic islands. The many Lau Islands which have a long-lived volcanic history therefore provide credible candidates for the volcanic centres ‘nearby to the west, outside the mapped area’ of Herzer & Exon (Citation1985). The island-arc andesite character of the Lau Volcanic group (14.0–6.0 Ma) and the age range which includes the middle Miocene, the age of the mafic volcaniclastics on ‘Eua, supports the case. In order to provide a working model for the sourcing of the accretionary lapilli found on the Tonga Ridge, it is now necessary to constrain possible source locations prior to the partition of the ancestral Lau–Tonga Ridge and then consider how the active tectonics in the region may have repositioned source or settlement site.
Figure 1. Regional setting. A, The position of ‘Eua and Nomuka on the Tonga Ridge and Vatoa and Ono-i-Lau on the Lau Ridge. The Tonga frontal arc basin sediments (shaded) are broadly coincident with the 2000 m isobath, after Tappin (Citation1993). B, The Tonga Ridge platform, highlighted by the 1000 m isobath, with the currently active back-arc Tofua volcanic chain. Block margins after Scholl & Vallier (Citation1985), Austin et al. (Citation1989) and Tappin et al. (Citation1994).
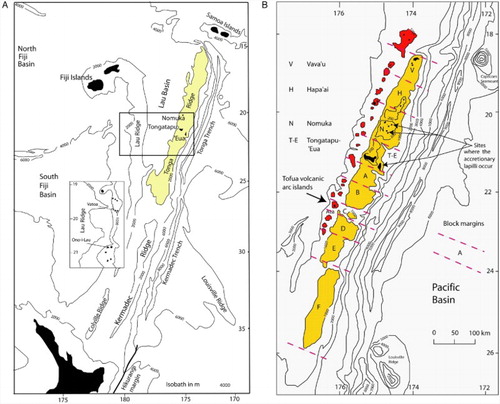
Accretionary lapilli and location of possible volcanic sources
The reported occurrences of accretionary lapilli on the Tonga Ridge are from Miocene volcaniclastics on ‘Eua and on two islands in the Nomuka group (B). The ‘Eua occurrences (A–C) range up to 20 mm in maximum dimension and typically occur unsorted in grain to grain contact as thin beds up to 20 cm in thickness. The matrix is coarse-grained (>500 μm) or absent. The ‘Eua occurrences exhibit characteristics suggesting they settled to pelagic depths (Ballance et al. Citation2004; Cunningham & Beard Citation2014) while some of the Nomuka occurrences may have been reworked from the original settlement site (Ballance et al. Citation2004). The ‘Eua host volcaniclastics are typically granulestone/sandstone in grain size with occasional larger clast sizes, none in excess of 30 mm, and a pelagic planktonic foraminiferal fauna. The fauna are dated at middle Miocene, c. 14 Ma, with sparse reworked slightly earlier fauna (Quinterno Citation1985; Chaproniere Citation1994) indicating depths of deposition are not less than 1600 m. A range of sediment gravity-flow types (Ballance et al. Citation2004) are reflected in the host formation, with rare westwards-dipping cross-beds (D). Tappin & Ballance (Citation1994) reported a WNW-verging flame structure. In contrast, the ‘Eua beds of accretionary lapilli exhibit a narrow size distribution in that they are large, typically 10–15 mm in diameter and the matrix is fines-depleted or absent. These features are applied to terminal velocity calculations by Cunningham & Beard (Citation2014) to argue that these beds were the result of settling to pelagic depths and were not delivered by sediment gravity flows or submarine pyroclastic flows. The upper size constraint of volcanogenic clasts in the ‘Eua volcaniclastics contrasts with the Nomuka host rocks; on Mango in the Nomuka group of islands, middle (?) Miocene volcaniclastics contain indications of the proximity of volcanic edifices such as volcanic boulder-bearing debris-flow deposits (Ballance et al. Citation2004). Further south on the Tongatapu–‘Eua (T–E) block, the detection of volcanic sources is assisted by the availability of close-spaced oil industry data (Gatliff et al. Citation1994). With the high rates of sediment supply implicit in island-arc environments, the problem of distinguishing reef structures from buried volcanic edifices is important and has been reviewed (Alexander Citation1985; Herzer & Exon Citation1985; Pflueger & Havard Citation1994; Tappin et al. Citation1994). Only one volcanic edifice was detected along the Tonga Ridge, in the B–C late Oligocene–early Miocene interval and on block D (Figure 1B). No ambiguous structures at all were identified on the T–E block within the interval which includes the middle Miocene (Gatliff et al. Citation1994) and ‘No volcanic structures sourcing unit A–B have yet been identified on the Tonga Ridge’ (Tappin et al. Citation1994). The seismostratigraphy therefore reveals no obvious local source on the Tonga Ridge for the accretionary lapilli, either for the Nomuka group or the ‘Eua occurrences. The regional setting suggests that sources would be to the west and on the remnant Lau Ridge, where long-lived volcanic islands exist.
Tectonics
The study area of the SW Pacific is a tectonic province with a relatively well-documented geological history, particularly with respect to back-arc extension/basin formation processes (Packham Citation1978; Tappin Citation1993; Sager et al. Citation1994; Tappin et al. Citation1994; Parson & Wright Citation1996; Taylor et al. Citation1996). In the south of the region, on the Tonga–Kermadec–Hikurangi trend, a subducting oceanic plate encounters continental crust on the South Island, New Zealand (Lamb Citation2011). Further north, the environment is oceanic. A more sophisticated model for Lau Basin formation (A, C) replaced a simple mid-oceanic type spreading centre model with a two-phase model (Parson et al. Citation1994; Parson & Wright Citation1996; Taylor et al. Citation1996). The Lau Basin floor geology is asymmetric; patterns of strong positive magnetic intensity are exhibited east of a line running NNW across the Lau Basin at roughly 317°, reflecting the new oceanic crust being created at the central and eastern Lau spreading centres. However, west of that line and east of the 2000 m isobath on the Lau Ridge, an irregular terrain of north-trending horst/grabens occurs where specific magnetisation events were not well delineated, attributed to diffuse spreading to form ‘extended arc crust’. In broad terms, the ancestral Lau–Tonga Ridge arc crust split and experienced extension to the east of the active arc volcanoes on the remnant Lau Ridge by:
graben/half-graben faulting accompanied by intrusive activity which mark the location of repeated ‘failed’ spreading centres (creating the ‘extended arc crust’), before:
formation of new crust occurred continuously at more typical mid-ocean-ridge-type spreading centres (the central Lau spreading centre/east Lau spreading centre, which were initiated in the north of the Lau Basin and propagated southwards.
Figure 3. Lau Basin tectonics. A, Synthesis of data centred on the Lau Basin, after Taylor et al. (Citation1996), with c. 20° easterly rotation of the Tonga Ridge (solid black lines) after Sager et al. (Citation1994). B, Outline reconstruction of the ancestral Lau–Tonga ridge, pre-Lau Basin formation, just after splitting commenced, with bathymetric contours. C, Schematic section of the Lau Ridge, Lau Basin and Tonga Ridge with ODP sites at c. 1.5–1.0 Ma after Clift et al. (Citation1995), modified to reflect the work of Parson & Wright (Citation1996).
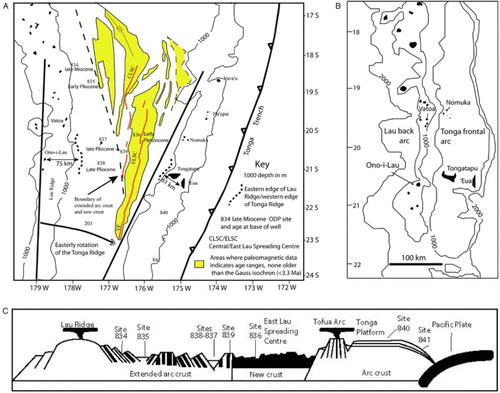
During these processes, Lau Ridge and intra-basin volcanism occurred and eventually ceased, before restoration of back-arc volcanism on the currently active Tofua Arc. The net effect is that of an apparent rotation of the Tonga Ridge, the current active arc, some 20° clockwise away from the remnant Lau Ridge segment of the ancestral arc. With no compelling evidence to support a source on the Tonga Ridge, ‘Eua appears to be at a considerable distance from a source which must have existed further to the west on the ancestral Lau-Tonga ridge. Using present sea-bed depth contours at 1000 and 2000 m to estimate the width of the ancestral arc elements, an outline reconstruction (B) is achieved by rotating the Tonga Ridge in the horizontal plane back to the west by the c. 20° estimated by Sager et al. (Citation1994). On block T–E, the distance between the western edge of the Tonga Ridge and ‘Eua, where it thins against the proto-‘Eua submergent high, is c. 61 km (A) before correction for extension due to post-middle Miocene faulting. Post-middle Miocene subvertical fault patterns on the Tonga Ridge segment do not suggest this will be material when compared with pre-middle Miocene graben/half-graben faulting which may be listric at depth. However, the threat of underestimation of extension due to unidentified small faults (Twiss & Moores Citation2007) supports the application of a non-trivial provision, say 10%, which would bring the 61 km estimate down to c. 55 km pre-fault extension. The Tonga frontal arc basin segment terminates abruptly on the west with down-to-Tofua faulting (Herzer & Exon Citation1985). The footprint of any volcanic source on the remnant Lau Ridge segment requires estimation. The profile of the currently active Tofua arc volcanoes provides possible analogues of Lau Ridge volcanic sources. At base, these range up to c. 30 km in width, excluding composite structures which are wider (Chase Citation1985, figure 1). On this basis, 55 + 15 km = 70 km is indicative of the minimum distance from a source on the eastern edge of the remnant Lau Ridge segment. If the source volcano was originally in what is now the extended arc crust of the western Lau Basin this figure is increased, but no data are available from the Ocean Drilling Program (ODP) sites in the Lau Basin to constrain this possibility as none of these reached the middle Miocene (A). A much higher figure is required if a structure in the position of Ono-i-Lau is considered. In the Lau Basin at the longitude under study, c. 105 km of extended arc crust exists and the distance from Ono-i-Lau to the eastern edge of the Lau Ridge is 75 km.
The more local effects of individual block rotation are now considered. During reprocessing of oil industry data on the block T–E, it was noted that a number of physiographic features of the block would be explained if it had rotated 30° anticlockwise (Gatliff et al. Citation1994). One feature is the atypical triangular shape of the Tongatapu–‘Eua block as a whole (B), as reflected at the 1000 m isobath. ‘Eua is closer to the eastern margin of the frontal arc basin than any other basement high and, as an emergent island with an elevation of 912 m, is much higher. To further explore whether there is seismostratigraphic/geophysical support for the rotation proposition, a number of sources of data were superimposed on blocks A, B and T–E (). There are clearly a number of departures from the Tonga Ridge NNE–SSW ridge-parallel structural trend, localised to the southern margin of block T–E. On block T–E, a trend in total magnetic intensity highs broadly coincident with basement highs (Gatliff et al. Citation1994) departs from trend and is deflected east of ‘Eua. Further south on blocks A and B, a trend of magnetic intensity anomalies (Stevenson & Childs Citation1985) coincident with ridge-parallel gravity/basement highs is abruptly curtailed as the southern margin of the T–E block is encountered. The ‘Eua Channel Fault, a major structural feature on the southern Tonga Ridge, disappears north of the T–E block southern margin, where the Tongatapu/‘Eua Channel depocentre was identified (Herzer & Exon Citation1985; Gatliff et al. Citation1994).
Figure 4. Discontinuity of trends across the boundary between tectonic blocks A, B and T–E. The trend of gravity and arc basement highs on blocks A and B is superimposed on residual magnetic anomaly data from Stevenson & Childs (Citation1985), determined by subtracting the 1975 International Geomagnetic Reference Field (IAGA Citation1976) from the observed total field measurements. The trend of basement highs on block T–E is superimposed on total magnetic intensity data from Gatliff et al. (Citation1994).
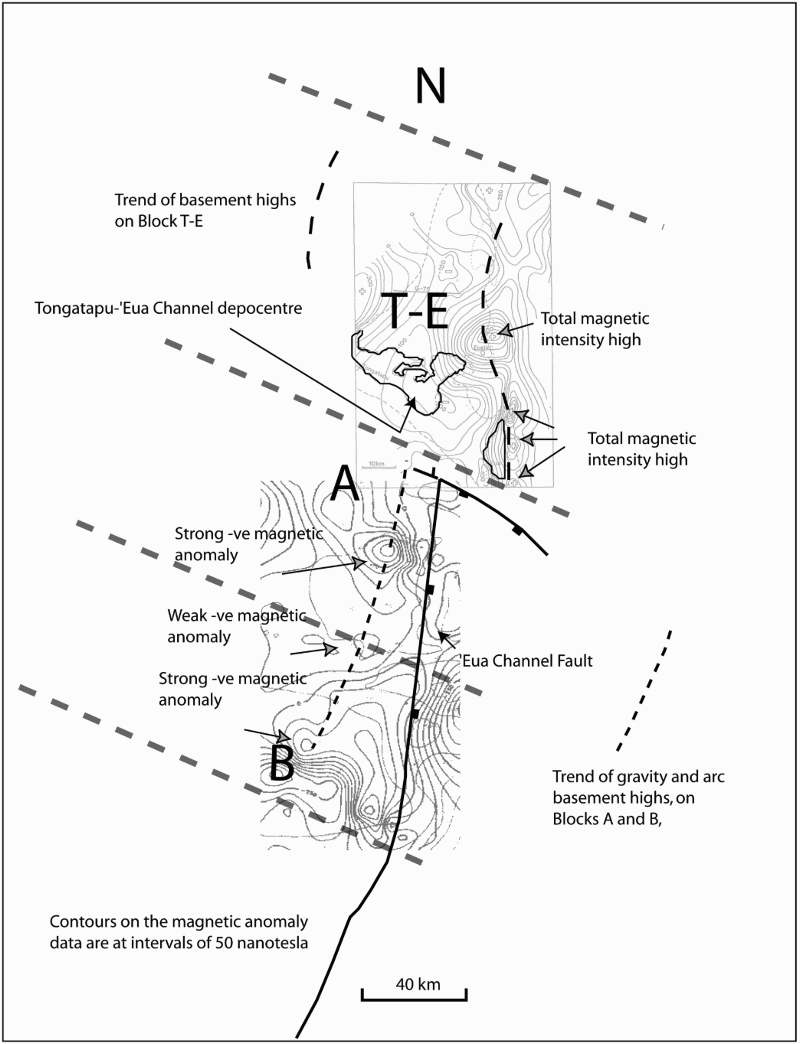
The three total magnetic intensity highs immediately east of ‘Eua on the Tongatapu-‘Eua block appear to be displaced by a strike-slip fault c. 40 km to the east of the trend of the magnetic intensity anomalies on blocks A and B. This would have the effect of anticlockwise rotation sensu Gatliff et al. (Citation1994). Further south on the Tonga–Kermadec–Hikurangi trend, Lamb (Citation2011) reviews the tectonics and kinetics of faulting in the leading Australian plate continental crust which accommodates the effects of non-orthogonal subduction. The distinctive faulting styles described include those which could explain features on the T–E block (Cunningham & Anscombe Citation1985, figure 2) by inverting the rotation effect of strike-slip faulting on arcuate faults (Lamb Citation2011, figure 18A), combined with dextral strike-slip faulting on a curved strike-slip fault ‘hinge’ (Lamb Citation2011, figure 18F). Block rotation may be contemporaneous with or post-date block formation. Block formation by ridge-traverse faults may have begun ‘long before the block geometry became so prominent after Late Miocene time’ (Scholl & Herzer Citation1994). Since the western margin of the T–E block has a down-to-Tofua NNE–SSW fault pattern consistent with the other blocks, any rotation must have occurred before the ancestral Lau–Tonga arc splitting commenced in the latest late Miocene (5.6 Ma), as noted by Gatliff et al. (Citation1994). An event at c. 10 Ma was detected by sediment backstripping analysis on the Tonga Ridge at ODP 841 (Clift et al. Citation1994) and hence in the early late Miocene. We now propose a model by which block rotation may have contributed towards the dispersal distance anomaly. The model crucially suggests that, pre-ancestral Lau–Tonga Ridge splitting, a middle Miocene volcano on what would become subjacent block A sourced the ‘Eua accretionary lapilli found on what would become block T–E. Anticlockwise block rotation after deposition, but before Lau Basin opening commenced in the latest late Miocene, affects block T–E but not A or N. After rotation of this block, the Nomuka Group islands maintain their distance from source volcano but ‘Eua has been displaced tectonically 40 km eastwards from the tephra source. The distance between source and resting place for the accretionary lapilli has been increased by 40 km even before ridge splitting in the latest late Miocene carries ‘Eua further east.
Constraining dispersal distances for accretionary lapilli
The evidence for final deposition of the ‘Eua accretionary lapilli by settling through a marine column of not less than 1600 m, as presented by Cunningham & Beard (Citation2014), has been summarised earlier. The processes by which they could have reached the point of settlement are now reviewed. The present Tofua active volcanic arc (B) is composed of emergent, barely emergent and submarine volcanic edifices at modest depths and may be a good proxy for the middle Miocene ancestral active volcanic arc, given the dominantly volcanic insular geology as described earlier for the remnant Lau Ridge. The ash clouds within which ash aggregates form (Brown et al. Citation2012) are typically associated with sub-aerial explosive volcanic eruptions, although shallow marine eruptions can also be contenders if they breach water depths (McBirney Citation1963; Wright & Gamble Citation1999; White et al. Citation2003) with the creation of an atmospheric ash cloud. The ‘Eua accretionary lapilli may therefore have formed during an explosive volcanic eruption initiated sub-aerially from an emergent volcanic edifice or at shallow depths. In addition, proximity of the ocean surface permits the possibility of formation of accretionary lapilli in secondary ash-rich steam clouds as pyroclastic density currents enter the sea (Dufek et al. Citation2007). Dispersal may take place sub-aerially within the eruption plume/umbrella cloud or as pyroclastic density currents travel across the sea surface (Carey et al. Citation1996; Allen & Cas Citation2001; Maeno & Taniguchi Citation2007). The substantial distances by which less-ordered ash aggregates can be dispersed from source sub-aerially are well established; ash aggregates dispersed in the eruption plume at Mt St Helens were detected at 200 km from source (Carey & Sigurdsson Citation1982). In contrast, the dispersal of relatively large and dense accretionary lapilli within the eruption plume must be restricted by their significant mass to more modest dispersal distances from the source volcano, as constrained in tephra dispersal models (Walker et al. Citation1971; Walker Citation1981; Carey & Sparks Citation1986; Pfeiffer et al. Citation2005; Folch Citation2012). Accretionary lapilli are technically lapilli falling within the 2–64 mm range (Schmid Citation1981; Fisher & Schmincke Citation1984). Lapilli-sized tephra can be dense juvenile/country-rock clasts, mafic scoria or vesicular silicic pumice clasts. Reported specific gravities of accretionary lapilli, which are dominantly silicic, are in the range of 1200–1500 kg m−3 (Sparks et al. Citation1997). The ‘Eua examples are mafic in composition and should therefore be at the upper end of this spectrum or slightly exceed it. Isopleths for 16 mm sized lapilli for known eruptions show maximum dispersal distance in the range of 20–30 km (Carey & Sparks Citation1986), for tephra at densities of 2500 kg m−3, and ‘larger centimetric and millimetric fragments typically settle in minutes to few hours at distances of the order of tens of km from the volcano’ (Folch Citation2012). Grain size directly influences terminal velocity of descent of a particle. This varies significantly with height in the atmosphere and departure from sphericity (Dellino et al. Citation2005). These parameters are accommodated in most tephra transport and dispersal models. provides indicative terminal velocities over a range of heights (Pfeiffer et al. Citation2005) for particles of = −4 (=16 mm), density of 1500 kg m−3 and departure from sphericity. These particles are close to the typical size of the ‘Eua accretionary lapilli. The density of 1500 kg m−3 is appropriate (advanced palagonitisation obscures the original density of the constituent glass particles). These figures would however underestimate terminal velocity for the notably spheroidal ‘Eua accretionary lapilli. Contemporary prevailing wind speeds in the Lesser Antilles range from 5.55 m s−1 in the stratosphere up to 25 m s−1 in the upper troposphere (Sigurdsson et al. Citation1980). Based on input of the 16 mm clast isopleth for Cotopaxi layer 3, Burden et al. (Citation2011) estimate plume height between 26 km and 32.5 km with a wind speed of 35 m s−1. If these wind speeds were applicable to the SW Pacific in the middle Miocene, the effects of wind advection should be modest for tephra the size of the ‘Eua accretionary lapilli. Complexity is introduced by the formation of aggregates during plume development whether in the form of accretionary lapilli or less-ordered ash aggregates, as this is complex to model (Costa et al. Citation2010). Accretionary lapilli often occur in phreatomagmatic eruptions where phase changes involving latent heat release might increment the upwards convection vector and counter the dominant role, in most models, of the downwards terminal (‘settling’) velocity of descent. Modelling of the phreatomagmatic 25.4 ka Oruanui event (Van Eaton et al. Citation2012), an ultra-Plinian event, instead of a simple plume/high-level umbrella cloud with lower-level co-ignimbrite ash clouds, produced ‘hybrid’ ash clouds generated both from the plume and from buoyant co-ignimbrite ash clouds which rise to plume heights. Concentrically layered accretionary lapilli similar to those in ‘Eua were dispersed at distances of 120 km from source (Van Eaton & Wilson Citation2013) in this event. The 25.4 ka Oruanui event is statistically unusual; only 156 (2.3%) such events are reported from a total of 6736 in the Smithsonian Institute database (Siebert & Simkin Citation2002–Citation2014). Occurrences from more modest events are reported from dispersal within the Soufriere St Vincent plume at 36 km from source (Brazier et al. Citation1982) and dispersed within pyroclastic density currents at Mt St Helens at c. 25 km (Fisher et al. Citation1987); these are closer to ash pellets as defined (Brown et al. Citation2010; Van Eaton & Wilson Citation2013), rather than accretionary lapilli. Surface dispersal over the ocean surface is now considered. Pyroclastic density currents can partition into a coarse, dense-clast-rich submarine flow and a dilute pyroclastic surface flow running at the surface on entering the sea, as seen from experiments and simulations referred to observed/inferred events and their deposits (Freundt Citation2003; Trofimovs et al. Citation2006, Citation2008; Dufek & Bergantz Citation2007; Dufek et al. Citation2009). Observations of the deposits of the Kos Plateau Tuff (Allen & Cas Citation2001) supported this model with the loss of the coarsest vent and conduit-derived lithic clasts over the sea due to sinking, while over land saltation was considered to have preserved the coarser element in the resulting ignimbrites. Saltation may also occur over water and be accentuated by the occurrence of pumice rafts (Fiske et al. Citation1998); conversely, transport capacity will be influenced by areal dilution as momentum transfer between large and small particles is diminished (Dufek & Bergantz Citation2007; Dufek et al. Citation2009). Such surface flows have travelled for considerable distances (), carrying bomb and lapilli-sized clasts in addition to ash and hot gas. In conclusion, for plume/umbrella cloud dispersal within the atmosphere, the ‘tens of kilometres’ metric is supported. For pyroclastic density-current-enabled dispersal over land, only a statistically unlikely ultra-Plinian event is capable of providing dispersal via the atmosphere for the minimum 70 km dispersal scenario (considering the source was close to the eastern edge of the remnant Lau Ridge segment). In contrast, for pyroclastic density-current-enabled dispersal across the ocean surface, there is some evidence that relatively modest-magnitude events could provide dispersal distances which contribute significantly to the scenario.
Table 1. Values for Ut, vertical terminal velocity at height, for particles of diameter 16 mm and density of 1500 kg m−3, after Pfeiffer et al. (Citation2005).
Table 2. Dispersal of larger tephra by pyroclastic density currents travelling over the ocean surface (Ui Citation1973; Carey et al. Citation1996; Allen & Cas Citation2001; Maeno & Taniguchi Citation2007). DRE: dense-rock equivalent.
Discussion and conclusions
The accretionary lapilli on ‘Eua, Tonga occur in middle Miocene pelagic volcaniclastic sediments with no evidence for a proximal volcanic source. A contemporary distance which is unlikely to be less than 70 km (and may be much more) from a source on the Lau segment of the ancestral Lau–Tonga Ridge is estimated from seismostratigraphic and other data. This is much further than would be expected for dispersal of these spheroids of significant mass, unless an exceptional ultra-Plinian source is invoked. Tephra fall associated with an ultra-Plinian event on the scale of the Oruanui at 25.4 ka (Van Eaton & Wilson Citation2013) could appear to resolve the dispersal distance problem, since the dispersal distances of accretionary lapilli in the atmosphere by the eruption plume and pyroclastic density currents in that event were substantial. However, there is no field evidence in the area under study for an ultra-Plinian event in the middle Miocene. At 530 km3 dense-rock equivalent (DRE), the Oruanui event is exceptional. Unit 8, which contains the highly dispersed occurrences, exhibits characteristics which suggest that the eruption produced an extremely high-mass eruption rate (≥109 kg s−1); numerical simulations (Van Eaton et al. Citation2012) imply the potential for transportation of tephra to stratospheric heights. Explosive volcanic events of a much more modest magnitude, but driving pyroclastic density currents over the ocean surface, have dispersed tephra to considerable distances () with larger tephra being carried as far as 65 km. The restriction of upper size carried, depending on mass flux during the event, has significance for the Tongan insular Miocene where the absence of clasts exceeding 30 mm has been attributed to some trapping mechanism elsewhere (Ballance et al. Citation2004) for clasts of greater size. Delivery by sediment gravity flows is probable for most of the volcaniclastics on the ‘Eua high (Tappin & Ballance Citation1994; Ballance et al. Citation2004). However, for any component of the ‘Eua volcaniclastics delivered by ocean surface pyroclastic density currents, an alternative process by which upper grain size is restricted is suggested. Furthermore, the rare westwards-dipping cross-beds in the ‘Eua volcaniclastics (D) may be attributable to sediment overloading on the ‘Eua high by periodic ocean surface pyroclastic density currents and consequential westwards backflow.
While delivery by pyroclastic density current over the ocean surface may explain all or part of the dispersal distance issue, it does not explain the anomalous position of the ‘Eua high; ‘Eua is positioned much further from the western edge of the Tonga Ridge than any other island. The discontinuities in trends at the southern T–E block margin, interpreted as block rotation of a particular type, provides a tectonic explanation for this anomaly. The relative thickness of sediment in the Tongatapu/‘Eua Channel depocentre () fits well within this model. With block rotation occurring in the late Miocene, but pre-splitting, the Tongatapu-‘Eua Channel basin would have been 40 km closer to the source volcanoes to the west for part of the interval 14–5.6 Ma, thus only 30 km from source on the minimum 70 km scenario.
For the rotation event to have contributed 40 km to the ‘Eua accretionary lapilli dispersal distance, a number of conditions must apply. First, it must pre-date splitting of the ancestral Lau–Tonga Ridge which commenced in latest late Miocene (5.6 Ma), second it must post-date the deposition of the accretionary lapilli on proto-‘Eua at 14 Ma, and third the accretionary lapilli must have been sourced from a volcano on the ancestral Lau–Tonga Ridge segment which became block A.
We favour a model where the accretionary lapilli on ‘Eua finally settled through a marine column of not less than 1600 m. Their delivery to the final resting site was most likely achieved by transport within a pyroclastic density current travelling over the ocean surface which, even in the case of those initiated by small/moderate explosive volcanic events, have delivered relatively large tephra considerable distances from source. A dual model comprising block rotation and dispersal by ocean surface pyroclastic density currents can explain the anomalies described and accommodate a large range of possible dispersal distances from a source of modest magnitude. The dating of block formation and of subsequent movement is however problematic; ridge-normal faulting is only strongly expressed in displacement of the A–B isopach, implying that it post-dated latest late Miocene. Only detailed paleomagnetic studies of the host middle Miocene volcaniclastics on ‘Eua could increase precision in this regard; the ubiquity of magnetite in thin hemipelagites which occur in these rocks would make such studies worthwhile.
Acknowledgements
JKC acknowledges the many in Tonga and on ‘Eua who assisted during 2 years spent there and during more recent visits. Shell International kindly provided copies of data sheets and their final report. Discussion, help and encouragement from Peter Ballance was crucial in framing the objectives of this paper. Subsequent assistance from Rick Hoblitt, Sharon Allen, Ben Ellis and Alexa Van Eaton greatly improved the execution. The detailed review points of Martin Jutzeler and an anonymous reviewer were crucial in achieving the final draft. The editors are thanked for their support.
Associate Editor: Professor Shane Cronin.
Disclosure statement
No potential conflict of interest was reported by the authors.
Additional information
Funding
References
- Alexander C. 1985. 2-D gravity and magnetic modelling of subsurface domical structure 11/14: Volcanic episodes in ‘Eua, Tonga. In: Scholl DW, Vallier TL editors. Geology and offshore resources of Pacific island arcs—Tonga region. Earth Science Series 2. Houston, TX: Circum-Pacific Council for Energy and Mineral Resources; p. 197–202.
- Allen SR, Cas RAF. 2001. Transport of pyroclastic flows across the sea during the explosive rhyolitic eruption of the Kos Plateau Tuff, Greece. B Volcanol. 62(6-7):441–456. doi: 10.1007/s004450000107
- Austin J, Taylor FW, Cagle CD. 1989. Seismic stratigraphy of the central Tonga Ridge. Mar Petrol Geol. 6:71–92. doi: 10.1016/0264-8172(89)90077-9
- Ballance PF, Tappin DR, Wilkinson IP. 2004. Volcaniclastic gravity flow sedimentation on a frontal arc platform: the Miocene of Tonga. New Zeal J Geol Geop. 47:567–587. doi: 10.1080/00288306.2004.9515076
- Brazier S, Davis AN, Sigurdsson H, Sparks RSJ. 1982. Fall-out and deposition of volcanic ash during the 1979 explosive eruption of the Soufriere of St. Vincent. J Volcanol Geoth Res. 14:335–359. doi: 10.1016/0377-0273(82)90069-5
- Brown RJ, Bonadonna C, Durant AJ. 2012. A review of volcanic ash aggregation. Phys Chem Earth. 45-46:65–78. doi: 10.1016/j.pce.2011.11.001
- Brown RJ, Branney MJ, Maher C, Harris PD. 2010. Origin of accretionary lapilli within ground-hugging density currents: evidence from pyroclastic couplets on Tenerife. B Geol Soc Am. 122:305–320. doi: 10.1130/B26449.1
- Burden RE, Phillips JC, Hincks TK. 2011. Estimating volcanic plume heights from depositional clast size. J Geophys Res. 116:B11206. doi:10.1029/2011JB008548.
- Carey SN, Sigurdsson H. 1982. Influence of particle aggregation on deposition of distal tephra from the May 18, 1980, eruption of Mount St. Helens volcano. J Geophys Res. 87:7061–7072. doi: 10.1029/JB087iB08p07061
- Carey SN, Sigurdsson H, Mandeville C, Bronto S. 1996. Pyroclastic flows and surges over water: an example from the 1883 Krakatau eruption. B Volcanol. 57:493–511. doi: 10.1007/BF00304435
- Carey SN, Sparks RSJ. 1986. Quantitative models of the fallout and dispersal of tephra from volcanic eruption columns. B Volcanol. 48:109–125. doi: 10.1007/BF01046546
- Chaproniere GCH. 1994. Middle and Late Eocene, Neogene, and Quaternary foraminiferal faunas from ‘Eua and Vava'u Islands, Tonga group, In: Stevenson AJ, Herzer RH, Ballance PF, editors. Geology and submarine resources of the Tonga-Lau-Fiji Region. SOPAC Technical Bulletin 8. Suva, Fiji: South Pacific Applied Geoscience Commission; p. 21–44.
- Chase TE. 1985. Submarine topography of the Tonga-Fiji Region and the southern Tonga platform area. In: Scholl DW, Vallier TL, editors. Geology and offshore resources of Pacific island arcs—Tonga region. Earth Science Series 2. Houston, TX, Circum-Pacific Council for Energy and Mineral Resources; p. 21.
- Clift PD, Bednarz UB, Bøe R, Rothwell RG, Hodkinson RA, Ledbetter JK, Pratt CE, Soakai S. 1994. Sedimentation on the Tonga forearc related to arc rifting, subduction erosion, and ridge collision: a synthesis of results from sites 840 and 841. In: Hawkins JW, Parson LM, Allan JF, editors. Proceedings of the Ocean Drilling Program, Scientific Results, Vol. 135. College Station, TX: p. 843–873.
- Clift PD and ODP. Leg 135 Scientific Party 1995. Volcanism and sedimentation in a rifting island arc terrain: an example from Tonga, SW Pacific. In: Smellie JL, editor. Volcanism associated with extension at consuming plate margins. Special Publication 88. London: Geological Society of London; p. 29–52.
- Clift PD, McCleod CJ, Tappin DR, Wright DJ, Bloomer SH. 1998. Tectonic controls on sedimentation and diagenesis in the Tonga Trench and forearc, southwest Pacific. Geol Soc Am Bull. 110:483–496. doi: 10.1130/0016-7606(1998)110<0483:TCOSAD>2.3.CO;2
- Cole JW, Gill JB, Woodhall D. 1985. Petrological history of the Lau Ridge, Fiji. In: Scholl DW, Vallier TL, editors. Geology and offshore resources of Pacific island arcs—Tonga region. Earth Science Series 2. Houston, TX: Circum-Pacific Council for Energy and Mineral Resources; p. 379–391.
- Costa A, Folch A, Macedonio G, Durant A. 2010. Modelling transport and aggregation of volcanic ash particles. EGU General Assembly 2–7 May 2010, Vienna, Austria. 8965.
- Cunningham JK, Anscombe KJ. 1985. Geology of ‘Eua and other islands, Kingdom of Tonga. In: Scholl DW, Vallier TL, editors. Geology and offshore resources of Pacific Island Arcs–Tonga Region island arcs—Tonga region. Earth Science Series 2. Houston, TX: Circum-Pacific Council for Energy and Mineral Resources; p. 221–257.
- Cunningham JK, Beard AD. 2014. An unusual occurrence of mafic accretionary lapilli in deep-marine volcaniclastics on ‘Eua, Tonga: palaeoenvironment and process. J Volcanol Geoth Res. 274:139–151. doi: 10.1016/j.jvolgeores.2014.01.012
- Dellino P, Mele D, Bonasia R, Braia G, La Volpe L, Sulpizio R. 2005. The analysis of the influence of pumice shape on its terminal velocity. Geophys Res Lett. 32:L21306. doi:10.1029/2005GL023954.
- Dufek J, Bergantz GW. 2007. Dynamics and deposits generated by the Kos Plateau Tuff eruption: controls of basal particle loss on pyroclastic flow transport. Geochem Geophy Geosy. 8, Q12. doi:10.1029/2007GC001741.
- Dufek J, Manga M, Staedter M. 2007. Littoral blasts: Pumice-water heat transfer and the conditions for steam explosions when pyroclastic flows enter the ocean. J Geophys Res. 112:B11201. doi:10.1029/2006JB004910.
- Dufek J, Wexler J, Manga M. 2009. Transport capacity of pyroclastic density currents: experiments and models of substrate-flow interaction. J Geophys Res. 114:B11203. doi:10.1029/2008JB006216.
- Fisher RV, Glicken H, Hoblitt RP. 1987. May 18, 1980, Mount St. Helens Deposits in South Coldwater Creek, Washington. J Geophys Res—Solid Earth. 92(B10):10267–10283. doi: 10.1029/JB092iB10p10267
- Fisher RV, Schmincke H-U. 1984. Pyroclastic rocks. Berlin: Springer-Verlag.
- Fiske RS, Cashman KV, Shibata A, Watanabe K. 1998. Tephra dispersal from Myojinsho, Japan, during its shallow submarine eruption of 1952–1953. B Volcanol. 59:262–275. doi: 10.1007/s004450050190
- Folch A. 2012. A review of tephra transport and dispersal models: evolution, current status, and future perspectives. J Volcanol Geoth Res. 235-236:96–115. doi: 10.1016/j.jvolgeores.2012.05.020
- Freundt A. 2003. Entrance of hot pyroclastic flows into the sea: experimental observations. B Volcanol. 65:144–164. doi: 10.1007/s00445–002–0250–1.
- Gatliff RW, Pflueger JC, Havard KR, Helu SP. 1994. Structure, seismic stratigraphy and petroleum potential of the Tongatapu -‘Eua area of the Kingdom of Tonga. In: Stevenson AJ, Herzer RH, Ballance PF, editors. Geology and submarine resources of the Tonga-Lau-Fiji Region. SOPAC Technical Bulletin 8. Suva, Fiji: South Pacific Applied Geoscience Commission; p. 107–119.
- Herzer RH, Exon NF. 1985. Structure and basin analysis of the southern Tonga forearc. In: Scholl DW, Vallier TL, editors. Geology and offshore resources of Pacific island arcs—Tonga region. Earth Science Series 2. Houston, TX: Circum-Pacific Council for Energy and Mineral Resources; p. 55–74.
- IAGA Division 1 Study Group. 1976. International geomagnetic reference field 1965. J Geophys Res. 74:4407–4408.
- Lamb S. 2011. Cenozoic tectonic evolution of the New Zealand plate-boundary zone: a paleomagnetic perspective. Tectonophysics. 509:135–164. doi: 10.1016/j.tecto.2011.06.005
- Maeno F, Taniguchi H. 2007. Spatiotemporal evolution of a marine caldera-forming eruption,generating a low-aspect ratio pyroclastic flow, 7.3 ka, Kikai caldera, Japan: Implication from near-vent eruptive deposits. J Volcanol Geoth Res. 167:212–238. doi: 10.1016/j.jvolgeores.2007.05.003
- McBirney AR. 1963. Factors governing the nature of submarine volcanism. B Volcanol. 26(Pt. 2):455–469. doi: 10.1007/BF02597304
- Packham, GH. 1978. Evolution of a simple island arc: The Lau-Tonga Ridge. B Aust Soc Explor Geophysicists. 9:133–140. doi: 10.1071/EG978133
- Parson LM, Rothwell RG, MacLeod CJ. 1994. Tectonics and sedimentation in the Lau Basin (southwest Pacific. In: Hawkins JW, Parson LM, Allan JF editors. Proceedings of the Ocean Drilling Program: scientific results. Vol. 135. College Station, TX: Texas A&M University. p. 9–22.
- Parson LM, Wright IC. 1996. The Lau-Havre-Taupo back-arc basin: A southward-propagating, multi-stage evolution from rifting to spreading. Tectonophysics. 263:1–22. doi: 10.1016/S0040-1951(96)00029-7
- Pfeiffer T, Costa A, Macedonio G. 2005. A model for the numerical simulation of tephra deposits. J Volcanol Geoth Res. 140:273–294. doi: 10.1016/j.jvolgeores.2004.09.001
- Pflueger JC, Havard KR. 1994. A re-examination of the line 11/14 anomaly on the Southern Tonga Platform. In: Stevenson AJ, Herzer RH, Ballance PF, editors. Geology and submarine resources of the Tonga-Lau-Fiji Region. SOPAC Technical Bulletin 8. Suva, Fiji: South Pacific Applied Geoscience Commission; p. 107–119.
- Quinterno PJ. 1985. Cenozoic planktonic foraminifers and coccoliths from ‘Eua, Tongatapu and Nomuka Islands, southwest Pacific Ocean. In: Scholl DW, Vallier TL, editors. Geology and offshore resources of Pacific island arcs—Tonga region. Earth Science Series 2. Houston, TX: Circum-Pacific Council for Energy and Mineral Resources; p. 259–267.
- Sager WW, MacLeod CJ, Abrahamsen N. 1994. Palaeomagnetic constraints on Tonga Arc tectonic rotation from sediments drilled at Sites 840 and 841. In: Hawkins JW, Parson LM, Allan JF, editors. Proceedings of the Ocean Drilling Program: scientific results. Vol. 135. College Station, TX: Texas A&M University; p.763–783.
- Schmid R. 1981. Descriptive nomenclature and classification of pyroclastic deposits and fragments: recommendations of the International Union of Geological Sciences Subcommission on the Systematics of Igneous Rocks. Geology. 9:41–43. doi: 10.1130/0091-7613(1981)9<41:DNACOP>2.0.CO;2
- Scholl DW, Vallier TL, editors. 1985. Geology and offshore resources of the Pacific island arcs—Tonga region. Earth Science Series 2. Houston, TX: Circum-Pacific Council for Energy and Mineral Resources.
- Scholl DW, Herzer RH. 1994. Geology and resource potential of the southern Tonga–Lau region. In: Stevenson AJ, Herzer RH, Ballance PF editors. Geology and submarine resources of the Tonga-Lau-Fiji Region. SOPAC Technical Bulletin 8. Suva, Fiji: South Pacific Applied Geoscience Commission; p. 329–335.
- Siebert L, Simkin T. 2002–2014. Volcanoes of the world: an illustrated catalog of Holocene volcanoes and their eruptions. Global Volcanism Program Digital Information Series, GVP–3. Washington, DC: Smithsonian Institution.
- Sigurdsson H, Sparks RSJ, Carey SN, Huang TC. 1980. Volcanogenic sedimentation in the Lesser Antilles Arc. J Geol. 88(5):523–540. doi: 10.1086/628542
- Sparks RSJ, Bursik MI, Carey SN, Gilbert JE, Glaze L, Sigurdsson H, Woods AW 1997. Particle aggregation in plumes. In: Sparks RSJ, Bursik MI, Carey SN, Gilbert JE, Glaze L, Sigurdsson H, Woods AW, editors. Volcanic plumes. Chichester, UK: John Wiley & Sons; p. 431–462.
- Stevenson AJ, Childs JR. 1985. Single channel seismic and geopotential data collection and processing. In: Scholl DW, Vallier TL editors. Geology and offshore resources of Pacific island arcs—Tonga region. Earth Science Series 2. Houston, TX: Circum-Pacific Council for Energy and Mineral Resources; p. 27–29.
- Stevenson AJ, Herzer RH, Ballance PF. 1994. Geology and submarine resources of the Tonga-Lau-Fiji region. SOPAC Technical Bulletin 8. Suva, Fiji: South Pacific Applied Geoscience Commission.
- Tappin DR. 1993. The Tonga frontal arc basin. In: Ballance PF, editor. South Pacific sedimentary basins. Sedimentary Basins of the World 2. Amsterdam: Elsevier; p. 157–176.
- Tappin DR, Ballance PF. 1994. Contributions to the sedimentary geology of ‘Eua Island, Kingdom of Tonga: reworking in an oceanic forearc. In: Stevenson AJ, Herzer RH, Ballance PF editors. Geology and submarine resources of the Tonga-Lau-Fiji Region. SOPAC Technical Bulletin 8. Suva, Fiji: South Pacific Applied Geoscience Commission; p.1–20.
- Tappin DR, Herzer RH, Stevenson AJ. 1994. Structure and history of an oceanic forearc- The Tonga Ridge – 22° to 26° south. In: Stevenson AJ, Herzer RH, Ballance PF, editors. Geology and submarine resources of the Tonga-Lau-Fiji Region. SOPAC Technical Bulletin 8. Suva, Fiji: South Pacific Applied Geoscience Commission; p. 81–99.
- Taylor B, Zellmer K, Martinez F, Goodliffe A. 1996. Sea-floor spreading in the Lau back-arc basin. Earth Planet Sci Lett. 144:35–40. doi: 10.1016/0012-821X(96)00148-3
- Trofimovs J, Amy L, Boudon G, Deplus C, Doyle E, Fournier N, Hart MB, Komorowski JC, Le Friant A, Lock EJ, et al. 2006. Submarine pyroclastic deposits formed at the Soufriere Hills Volcano, Montserrat (1995–2003): what happens when pyroclastic flows enter the ocean? Geology. 34:549–552. doi: 10.1130/G22424.1
- Trofimovs J, Sparks, RSJ, Talling, PJ. 2008. Anatomy of a submarine pyroclastic flow and associated turbidity current: July 2003 dome collapse, Soufriere Hills volcano, Montserrat, West Indies. Sedimentology. 55:617–634. doi: 10.1111/j.1365-3091.2007.00914.x
- Twiss RJ, Moores EM. 2007. Structural geology. New York: Freeman.
- Ui T. 1973. Exceptionally far-reaching, thin pyroclastic flow in Southern Kyushu, Japan. Bull Volcanol Soc Japan. 2(18):153–168.
- Van Eaton AR, Herzog M, Wilson CNJ, McGregor J. 2012. Ascent dynamics of large phreatomagmatic eruption clouds: the role of microphysics. J Geophys Res - Solid Earth. 117(B3). doi:10.1029/2011JB008892.
- Van Eaton, AR, Wilson, CNJ. 2013. The nature, origins and distribution of ash aggregates in a large-scale wet eruption deposit: Oruanui, New Zealand. J Volcanol Geoth Res. 250:129–154. doi: 10.1016/j.jvolgeores.2012.10.016
- Walker GPL, Wilson L, Bowell ELG. 1971. Explosive volcanic eruptions I: the rate of fall of pyroclasts. Geophys J Roy Astron Soc. 22:377–383. doi: 10.1111/j.1365-246X.1971.tb03607.x
- Walker GPL. (1981). Plinian eruptions and their products. B Volcanol. 144:223–240. doi: 10.1007/BF02600561
- White JDL, Smellie JL, Clague DA. 2003. Introduction: a deductive outline and overview of subaqueous explosive volcanism. In: White JDl, Smellie JL, Clague DA, editors. Explosive subaqueous volcanism. Geophysical Monograph Series 140. Washington, DC: American Geophysical Union. p. 1–14.
- Wiesner MG, Wang Y, Zheng L. 1995. Fallout of volcanic ash to the deep South China Sea induced by the 1991 eruption of Mount Pinatubo. Geology. 23:885–888. doi: 10.1130/0091-7613(1995)023<0885:FOVATT>2.3.CO;2
- Woodhall D. 1985. Geology of the Lau Ridge. In: Scholl DW, Vallier TL, editors. Geology and offshore resources of Pacific island arcs—Tonga region. Earth Science Series 2. Houston, TX: Circum-Pacific Council for Energy and Mineral Resources; p. 351–378.
- Wright IC, Gamble JA. 1999. Southern Kermadec submarine caldera arc volcanoes (SW Pacific): caldera formation by effusion and pyroclastic eruption. Mar Geol. 161:207–227. doi: 10.1016/S0025-3227(99)00040-7