ABSTRACT
Quartz arenites are a very common geological feature on the South Island of New Zealand. The provenance of the quartz grains that form these arenites is not well constrained because standard point-counting techniques do not provide enough detailed information. In this study we distinguish different quartz types using scanning electron microscopy–cathodoluminescence combined with standard optical microscopy on the same individual quartz grains. This is done on a suite of samples collected from shallow marine quartz arenites of the Paleocene–Eocene Blondin Sand Member of the Broken River Formation (Eyre Group) at Mt Somers in the Canterbury basin on the South Island of New Zealand. Our results show that the volcanic quartz grains were derived from local volcanic source rocks, whereas metamorphic quartz grains may have been derived from either local source rocks of the Torlesse Supergroup or from distant metamorphic sources rocks via longshore transport. Plutonic quartz grains were exclusively derived by long-distance transport. Warm and humid climatic conditions and the presence of humic acids in the associated coal-forming depositional environment probably increased the proportion of quartz in the sampled rocks.
Introduction
Quartz arenites are very pure quartz sandstones with more than 95% quartz framework grains. They are regarded as enigmatic with respect to their occurrence, their conditions of formation and their provenance (Chandler Citation1988) because source-rock composition, climate, relief, vegetation cover and characteristics of the depositional environment are all important factors controlling the composition of clastic sediment (Blatt Citation1967). The classic view is that quartz arenites are texturally mature, multi-cyclic sediments with grains that show high degrees of rounding and sorting (Pettijohn et al. Citation1987). Nonetheless, other studies found evidence for first-cycle quartz arenites with angular and poorly sorted quartz grains in settings favouring chemical weathering (Johnsson et al. Citation1988, Citation1991).
We present here a petrologic study using the approach of combined scanning electron microscopy–cathodoluminescence (SEM-CL) and optical microscopy (OM) analysis on single quartz grains (Bernet & Bassett Citation2005). The aim of this study is to determine the quartz types that make up the Paleocene–Eocene Blondin Sand Member of the Broken River Formation (Eyre Group) in the Mount Somers area on the South Island of New Zealand (). The SEM-CL/OM data of this study were originally published in Bernet & Bassett (Citation2005), where we explained the technical aspects of this provenance analysis technique. Here we present our geological interpretation of these data and their implications for understanding the provenance of the quartz arenites in the Mount Somers area. In the following sections we briefly review the formation of quartz-rich sediments and the geological setting of the study area. We then present our results using the combined SEM-CL/OM approach and discuss potential sources and processes that led to the formation of these quartz arenites.
Formation of quartz arenites
The formation of sand or sandstone of quartz arenite composition with 95% or more of quartz grains has two basic requirements: (1) sediment sources need to contain sand- or gravel-sized quartz crystals; and (2) mechanical and more likely chemical processes must selectively enrich quartz while depleting the sediment in lithic fragments, feldspars and other components. Quartz is a very common mineral in many crustal rocks. Sediment sources for quartz arenite can therefore cover a wide range of rocks including felsic plutonic rocks (e.g. granites, granodiorites), acidic volcanic rocks (rhyolites, dacites, ignimbrites, etc.) and the metamorphic equivalents of such rock types (e.g. ortho-gneiss and meta-volcanic rocks, etc.). Vein quartz may volumetrically not be as significant as other lithologies, but can in some cases be very important on a local scale because of its purity and commonly large crystal size. Quartz grains derived from such sources are regarded as first-cycle sediment (Pettijohn et al. Citation1987; Chandler Citation1988). However, additional sources of sand-sized quartz grains are coarse-grained siliciclastic sedimentary rocks (conglomerates and sandstones) and their low-grade metamorphic equivalents. Because quartz grains from such sources have already been through at least one quartz-enriching sedimentary cycle, they are regarded as recycled or multi-cycle sediment (Pettijohn et al. Citation1987; Chandler Citation1988).
Early studies found that quartz arenites are commonly texturally mature with high degrees of sorting and rounding (Chandler Citation1988). Such textural maturity indicates extended mechanical reworking of sediment during which unstable lithic fragments and feldspars break down and disappear, while more durable quartz grains are relatively enriched. The primary depositional environments of quartz arenites were therefore identified as aeolian and marine shelf environments, which allowed prolonged reworking and sorting of sediment (Selley Citation1982).
Nonetheless, studies by Suttner et al. (Citation1981) and Johnsson et al. (Citation1988, Citation1991) demonstrated the formation of texturally immature fluvial quartz arenite under specific climatic and sedimentological conditions. The influence of climate and weathering on sediment composition has been elaborated in a variety of studies using modern river sediments in regions with tropical to subtropical climates, characterised by high mean annual temperatures and high precipitation rates (Suttner et al. Citation1981; Basu Citation1985; Suttner & Dutta Citation1986; Johnsson et al. Citation1988, Citation1991). Weathering and chemical breakdown starts right at the source rock (White et al. Citation2001) and continues during sediment transport and temporary storage in point bars, floodplains and soils within the fluvial system (Johnsson et al. Citation1988, Citation1991; Johnsson & Meade Citation1990; McLaren & Cameron Citation1996; Powrie Citation2004; Bouchez et al. Citation2012), and even after ‘final’ deposition (McBride Citation1985; De Ros Citation1998). Chemical breakdown of unstable lithic fragments and feldspars, plagioclase in particular, is the main factor for relative quartz enrichment, whereas mechanical destruction of non-quartz components plays only a secondary role under tropical climatic conditions. In more temperate regions both mechanical and chemical processes are at work to enrich sediment in quartz content, but chemical processes are enhanced under warm and/or humid conditions (Mitchell Citation1993; Allen Citation1997). Localised acidic conditions under coal-forming peat bogs can enhance chemical weathering in temperate climates (Allen Citation1997; Curran et al. Citation2002). Climate and weathering conditions can vary considerably between sources and sinks in large drainage systems. The weathering response of sediment can change because of compositional change in the sediment during temporary storage in floodplains, with progressive removal of reactive material as well as variability in pore fluid pH and fluid flux (Johnsson & Meade Citation1990; Johnsson et al. Citation1991; Bouchez et al. Citation2012).
Geological setting
The Mount Somers area is located in the Canterbury basin to the southwest of Christchurch between the Ashburton and Rakaia rivers on the South Island of New Zealand (). In this region the Canterbury basin is largely a passive continental margin basin, with the Eyre Group representing a non-marine–marine transgression sequence resting on Triassic basement rocks of the Torlesse Supergroup and Late Cretaceous volcanic rocks of the Mount Somers Volcanic Group (Field et al. Citation1989). The Torlesse Supergroup consists of prehnite–pumpellyite facies meta-sedimentary rocks, marked by the occurrence of quartz and zeolite veins (Oliver et al. Citation1982). The Mount Somers Volcanic Group (92–98 Ma) rocks are calc-alkaline, ranging in composition from high-alumina basalt to rhyolite erupted in domes, flows, tuffs and ignimbrites (Adams & Oliver Citation1979; Oliver & Keene Citation1989; Tappenden Citation2003). The rhyolites and ignimbrites are relatively quartz-rich (Tappenden Citation2003).
On the South Island, the Torlesse Supergroup and the Mount Somers Volcanic Group are generally stratigraphically succeeded by latest Cretaceous–earliest Oligocene transgressive sedimentary units of the Broken River Formation (e.g. Raine & Wilson Citation1988; van der Lingen Citation1988; Field et al. Citation1989). As shown in , the stratigraphy of the rocks in the study area at Mt Somers consists of the Torlesse Supergroup, Mount Somers volcanic rocks and saprolite (kaolinised rhyolite), which is separated by a stratigraphic hiatus from overlying clay deposits and coal measures of Paleocene–early Eocene age (van der Lingen Citation1988; Field et al. Citation1989). The coal measures are succeeded by shallow-marine tidal siltstone to fine-sandstone (Unit I), shoreface sandstone (Unit II), lagoonal black clay and quartz sandstone with fossil wood (Unit III), a second shoreface sandstone deposit (Unit IV), and nearshore marine glauconite-bearing quartz-pebble sandstone (Unit V; ; ). This succession is overlain by Quaternary fluvio-glacial conglomerates. Units I, II and IV are generally poorly consolidated and very friable sandstones with local iron-stained horizons and have a nearly pure quartz composition, such that they are mined for glass production. These sandstone deposits are typically cross-bedded on a decimetre to metre scale (; Browne & Field Citation1985; Oliver & Keene Citation1989). These sediments were never deeply buried. They are highly porous with little to no cement; no evidence for secondary porosity, such as overly large pores, was observed.
Figure 2. Mount Somers area sand quarry stratigraphic column (modified after van der Lingen Citation1988).
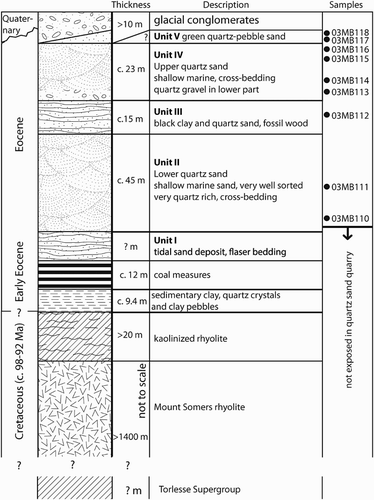
Figure 3. A, Outcrop of the Blondin Sand Member of the Broken River Formation in the glass sand quarry at Mount Somers, South Island New Zealand, showing the middle to upper part of the section and overlying Quaternary fluvio-glacial conglomerates. B, Fine-grained sandy conglomerate deposits in unit IV (; ). C, Large-scale cross-bedding in unit II (; ). The different units were defined by van der Lingen (Citation1988).
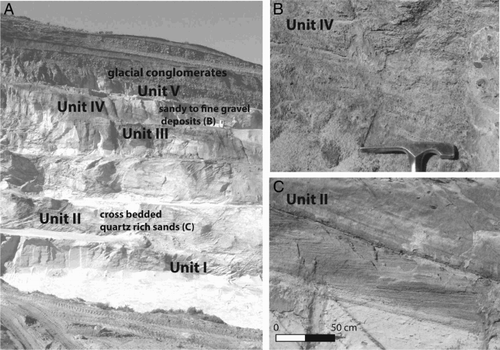
Table 1. Description of Mount Somers quartz sand quarry samples.
Methods
For this study we analysed nine samples collected from the glass sand quarry (GPS coordinates 43.64598°S, 171.29720°E) to the NW of Mount Somers (). Thin-sections of all samples were first described petrographically and point counted using standard techniques, counting 300 grains per thin-section. The grain size of the analysed samples ranged from fine to coarse sand.
Using highly polished thin–sections, about 100 randomly selected individual quartz grains per sample were analysed with the combined SEM-CL/OM technique as outlined by Bernet & Bassett (Citation2005). The aim of this type of analysis is to distinguish on the basis of optical and cathodoluminescence characteristics between quartz grains derived from volcanic, plutonic or metamorphic rocks () and, if possible, quartz grains recycled from sedimentary rocks. Volcanic quartz grains appear in CL images as either zoned or homogeneous while maintaining straight extinction in OM images. In addition, volcanic quartz grains commonly have embayments. Quartz grains of plutonic origin are characterised by healed microcracks and occasional zoning in CL images, and straight to weak undulose extinction in OM images. Polycrystalline quartz grains with dark CL and strong undulose extinction in OM are interpreted as grains derived from low- to high-grade metamorphic sources or from quartz veins. Recycled grains can only be identified if they carry certain features that can be related to previous deposition and diagenesis, such as inherited iron-oxide ‘dust’ rims as seen in OM, or inherited quartz overgrowth which can be identified more reliably with SEM-CL or colour CL analysis (e.g. Zinkernagel Citation1978; Sanderson Citation1984; Hogg et al. Citation1992; Bernet et al. Citation2007).
Figure 4. Examples of volcanic, plutonic and metamorphic quartz source-rock lithologies in SEM-CL images. A, B, Plutonic quartz from the Deutgam granodiorite, Hohonu batholith, South Island, New Zealand, showing microcracks and healed microfractures. C, D, Volcanic quartz in Mount Somers rhyolite samples, South Island, New Zealand. E, F, Metamorphic quartz of the Alpine/Otago Schist from the Haast Pass, Southern Alps, South Island, New Zealand.
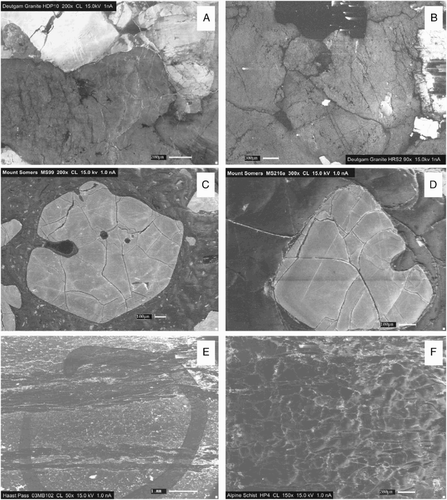
All thin-sections for combined SEM-CL/OM analysis were carbon coated and analysed with a Leica Stereoscan 440 SEM equipped with an Oxford MiniCL cathodoluminescence detector. Digital panchromatic images were acquired at 300 pA to 2.0 nA beam current and 15–25 kV acceleration voltage at a working distance of 19–22 mm. Images were taken at 4096–8192 pixel-average, corresponding to 5–10 minutes of scan-time. Optical microscopy was performed with a Leitz petrographic microscope.
Results
Point counts of framework grains from the same samples show on average 99.1% quartz, 0% feldspar and 0.9% lithic fragments (a mixture of volcanic, sedimentary and chert) with occasional muscovite and glauconite grains. Following the sandstone classification of McBride (Citation1963), all samples in this study can therefore be considered as quartz arenites (A). The vast majority of grains observed in thin-sections are sub-angular to well-rounded grains. Combined SEM-CL/OM analysis shows the occurrence of volcanic, plutonic and metamorphic quartz in all samples (, ; ). No recycled quartz grains with inherited quartz overgrowths were identified. Detailed data tables of individual quartz type determination for each sample presented in this study are available in Repository S1.
Figure 5. A, Sandstone classification after McBride (Citation1963) based on standard point-counting. B, SEM-CL/OM quartz-type results of correlative Broken River, Iron Creek, Waipara Greensand and Conway Formations at the six sampling locations. Metamorphic quartz includes all low-grade to high-grade metamorphic recrystallised and vein quartz.
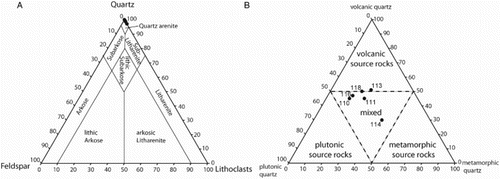
Figure 6. Example of SEM-CL images of sand samples A, 03MB110, B, 03MB113, and C, 03MB114. All samples show volcanic, plutonic and metamorphic quartz grains. Volcanic, angular quartz grains with a homogeneous CL response are more common in the finer-grained fraction. Plutonic quartz grains can be recognised by the ‘spider webs’ of microcracks. The classification as different quartz types is based on SEM-CL images and optical analysis (extinction behaviour etc.) of the same quartz crystals. See for SEM-CL images of examples of all three quartz types from potential source rocks.
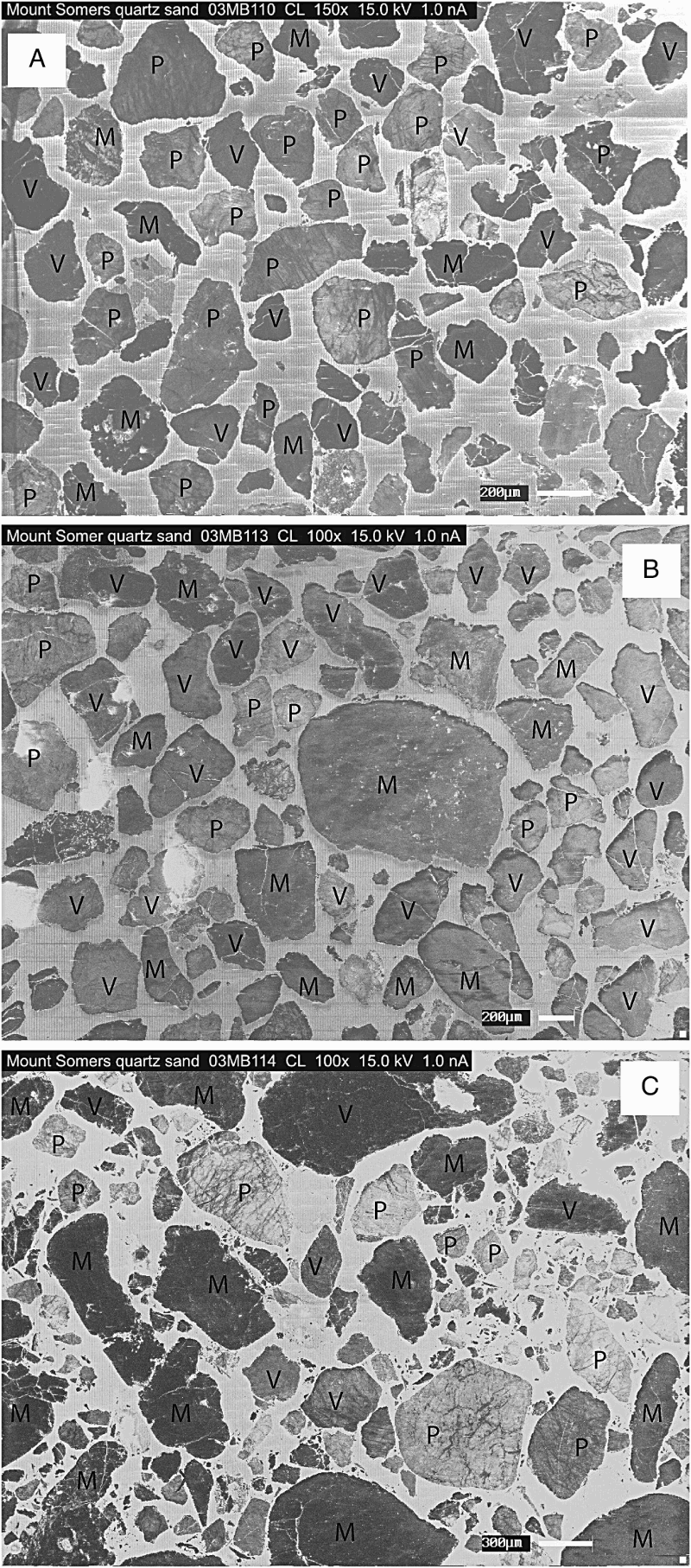
Table 2 Mount Somers SEM-CL/OM quartz type counts.
On average, the samples contain 44.7% volcanic quartz, 32.7% plutonic quartz and 22.7% metamorphic quartz, but individual samples may vary by up to 10–15% from these values (). The samples were collected from well- to very-well-sorted-, mainly fine-sand-sized quartz arenite, with the exception of sample 03MB114 which has poor sorting with coarse- to fine-sand-sized grains ().
Discussion
Provenance
Volcanic source rocks
Given that volcanic quartz types (, D, 6; ) are overall the most important component of the quartz arenites in this study, it is likely that the quartz grains of the Blondin Sand Member of the Broken River Formation in the Mount Somers area were for the most part derived locally from volcanic source rocks. The glass sand quarry is located less than a kilometre from the rhyolite domes of the Mt Somers Volcanic Group. Using more conventional cold-cathode colour CL analysis, Field (Citation1989) concluded that quartz crystals with strong blue luminescence found in Paleocene–Oligocene sedimentary rocks of the Canterbury basin were derived from the Mount Somers volcanic rocks. This is supported by Zinkrenagel (Citation1978) who described rapidly cooled plutonic, and particularly volcanic quartz, as having blue-violet luminescence colours.
Metamorphic source rocks
The source of metamorphic or recrystallised quartz grains (, F, 5, 6) is a bit more complicated to identify as the CL response varies with the degree of metamorphism and subsequent cooling history of the quartz crystals (Zinkrenagel Citation1978). Furthermore, vein quartz can appear very similar to metamorphic quartz as vein quartz has a very variable CL response and is not always dark or mottled; it may even show concentric zoning (Bernet & Bassett Citation2005, figure 1). Nonetheless, zoned vein quartz is relatively rare and was not observed in the quartz grains of this study. Because of the large overlap in patterns and CL response, with panchromatic SEM-CL/OM analysis we cannot easily distinguish between quartz grains derived from metamorphic rocks or quartz veins within such rocks.
So what are the potential source rocks of metamorphic quartz on the South Island? The first source to be considered is the locally abundant Torlesse Supergroup, which consists of very-low-grade to low-grade metasedimentary rocks and greywackes (Shelley Citation1975; Oliver & Keene Citation1989; Mortimer Citation1993). It is the likely source of sedimentary lithic fragments identified in the pebbly layers of Unit V of the sampled section (, 3), and is therefore also a potential local source of metamorphic quartz. Using conventional colour CL analysis, Field (Citation1989) found that the Torlesse Supergroup is locally of such low metamorphic grade that it retains a wide range of luminescence colours in its quartz grains, more typical of a sedimentary source. With our current analysis we did not find evidence for recycled quartz grains from a sedimentary/metasedimentary source, such as dust rims or preserved quartz overgrowths around detrital grains (e.g. Bernet et al. Citation2007). We cannot rule out some contribution of metamorphic quartz from the local very-low-grade metamorphic Torlesse greywackes, but we think that the fine grain size of these rocks makes it unlikely to impossible that they can provide medium-sand-sized quartz grains for the Broken River Formation (). However, the quartz veins that can be found in the Torlesse Supergroup could be a likely source of at least some of the quartz grains that we described as metamorphic or recrystallised quartz types with dark CL or patchy/mottled CL (Bernet & Bassett Citation2005).
As proposed by Van der Lingen (Citation1988) and Field et al. (Citation1989), the Otago Schist is also a likely source for the metamorphic quartz grains; well-rounded mica-schist pebbles can occasionally be found in Unit V of the studied section, as well as abundant detrital muscovite in our sandstone samples. The Otago Schist, a geographic subset of the Haast Schist, is the end result of increasing metamorphism from north to south from the Torlesse Supergroup into the volcanic Caples Terrane (; Turnbull et al. Citation2001) and consists mainly of greenschist–subgreenschist-facies metasandstone alternating with argillite beds (Mortimer Citation1993; Turnbull et al. Citation2001). It is today exposed in the south and west of the Southern Alps. Field (Citation1989) stated that the Otago Schist only contains non-luminescent to reddish-brown luminescent quartz typical of low-grade to medium-grade metamorphic quartz described by Zinkrenagel (Citation1978). The non-luminescent or dark CL quartz grains, as we call them in this study, may therefore have been transported first by rivers and then by longshore currents along the east coast of the South Island for 200–300 km. Present-day ocean currents around the South Island of New Zealand are fully capable of such transport (e.g. Bowen et al. Citation2004) and such longshore transport is not unusual, as observed in the Gulf of Mexico (e.g. Cipriani & Stone Citation2001; Ellis & Stone Citation2006). A longshore transport model is further supported by the higher degree of rounding of the metamorphic quartz crystals, which is more typical of rounding by wave than by river transport (e.g. Blatt Citation1967).
Figure 8. Paleogeographic map of the South Island during the Eocene–Oligocene, showing the location of potential volcanic, plutonic and metamorphic source rocks with respect to the Mount Somers (Mt S) area before initiation of the Alpine Fault (after Landis and Coombs Citation1967; Bradshaw Citation1993; Muir et al. Citation1995; King Citation2000).
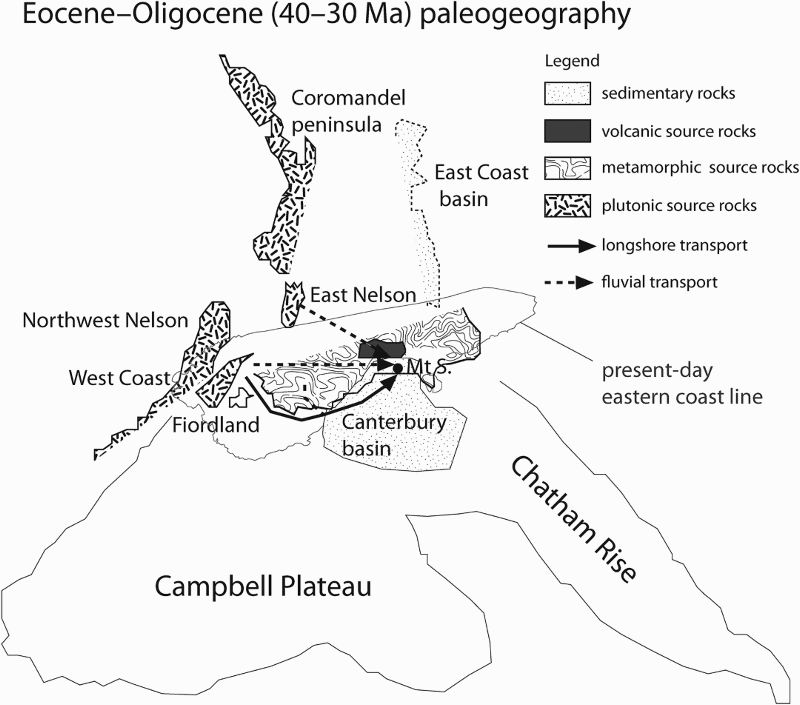
Our preliminary data suggest that two populations of metamorphic quartz may be present: one that is polycrystalline with dark CL and the other that has high undulose extinction with dark CL. Unfortunately, at this stage it is not possible to distinguish between vein quartz derived from the Torlesse Supergroup or metamorphic quartz from the Otago Schist, but this may be possible with detailed trace element or isotopic analyses (Götze & Zimmerle Citation2000).
Plutonic source rocks
The most interesting provenance question concerns the origin of the plutonic quartz grains that make up one-third of the grains analysed in this study. The most obvious source is recycled quartz from very low-grade Torlesse Supergroup rocks. However, as already discussed the fine grain size of the Torlesse Supergroup make this an unlikely local source of medium-sand-sized, plutonic-type quartz grains; such grains must therefore have been derived from more distant sources. We think the most likely plutonic sources include the Devonian and Cretaceous granitoids of the Karamea batholith (e.g. Muir et al. Citation1996), the Cretaceous Separation Point and Hohonu batholiths (e.g. Muir et al. Citation1995; Waight et al. Citation1997) or the Carboniferous and Cretaceous granitic rocks of Fiordland (e.g. Muir et al. Citation1998), all on the South Island of New Zealand (). At least some of these plutonic sources were exposed at the time of deposition of the Broken River Formation and were not too distant prior to displacement along the Alpine Fault, located to the WSW of the Canterbury basin during the Paleocene–Eocene (; Field et al. Citation1989). Despite the proposed somewhat longer distance of transport of a few hundred kilometres, the plutonic quartz grains do not show a higher degree of textural maturity and most are sub-angular to sub-rounded. This is typical of fluvial transport rather than alongshore current transport (e.g. Blatt Citation1967), in contrast to the metamorphic quartz crystals that tend to show more rounding.
Using conventional heavy mineral analysis, Smale (Citation1987) reported that Cretaceous–Paleocene sediments in the Mount Somers area were most likely derived from local sources based on the presence of semi-opaque debris and epidote. However, a closer inspection shows that the Eocene–Oligocene sedimentary rocks in the Mount Somers area are dominated by hornblende, magnetite, ilmenite and zircon, which are rare in the Torlesse Supergroup rocks (Smale Citation1987). We agree with Smale (Citation1987) that the hornblende and magnetite grains were most like derived from the Mount Somers volcanic rocks. However, we think that the ilmenite and particularly the zircon may have come from more distant sources than the Torlesse Supergroup. For a more detailed provenance scenario of the zircon crystals, independent provenance information is needed such as from detrital geo-thermochronology and double-dating of detrital zircon (e.g. Rahl et al. Citation2003; Bernet et al. Citation2006; Jourdan et al. Citation2013). This would be an interesting exercise as many of the granitic rocks on the South Island have been dated with U–Pb analysis and the detrital zircon fission-track ages of the Southern Alps provide a very distinct signal (Garver & Kamp Citation2002).
Weathering and paleoclimate
Warm and humid conditions are favourable for weathering of unstable silicates (e.g. Suttner & Dutta Citation1986). In fact, chemical weathering, and to that effect also diagenesis, seems to be so important that mechanical destruction of non-quartz components plays only a secondary role under tropical climatic conditions (Johnsson et al. Citation1988; Milliken Citation1988; Yang et al. Citation2003). Strong kaolinitisation of rhyolite and other underlying basement rocks in the Mount Somers area (van der Lingen Citation1988) is an indication of humid and warm climatic conditions on the South Island of New Zealand during the Late Cretaceous–Eocene (Hornibrook Citation1971; Raine & Wilson Citation1988; Pocknall Citation1989; Hollis et al. Citation2009, Citation2012).
In addition, peat swamps are highly acidic environments that locally enrich quartz in the floodplain deposits (Staub & Gastaldo Citation2003). It is well known that quartz and muscovite are residual minerals after prolonged chemical weathering in a soil profile (Mitchell Citation1993). Peat bogs are more acidic and rapidly create leached soil profiles in underlying sediments (Staub & Gastaldo Citation2003). Quartz arenites are often associated with coal deposits, particularly in the Eocene of New Zealand, and the silica sand quarry of our study directly overlies thick coal deposits. Such climatic and depositional conditions with enhanced chemical weathering of source rocks and the breakdown of unstable lithic fragments and feldspars, plagioclase in particular, likely contributed to quartz enrichment.
Conclusions
The quartz sandstones of the Blondin Sand Member of the Broken River Formation in the Mount Somers area on the South Island of New Zealand consist of volcanic, metamorphic and plutonic quartz grains based on their internal structures as seen with SEM-CL analysis and their optical properties. Volcanic quartz is most likely derived from the local Mount Somers Volcanic Group. Metamorphic (or recrystallised) quartz could be derived from quartz veins of the local low-grade metamorphic Torlesse Supergroup or from the more distal higher-grade metamorphic Otago Schist via longshore transport along the east coast of the South Island or both. The Torlesse Supergroup metasedimentary rocks are not considered as a main source of metamorphic quartz, as these rocks are in general too fine-grained to provide medium-sand-size quartz crystals. The one-third of plutonic quartz grains are most likely derived by fluvial transport from the west coast batholiths.
Intense chemical alteration during weathering and diagenesis that started at the source rock and continued during transport, reworking, temporary storage in floodplains with numerous acidic peat bogs and perhaps even after deposition, seems to have been very efficient under humid and warm climatic conditions during the formation of the Blondin Sand Member quartz arenites. The removal of weathering products and mechanical breakdown of non-quartz grains occurred during transport and reworking in the shallow-marine depositional environment along the east coast of the South Island of New Zealand.
Supplementary data
Repository S1. Photographs and data tables of quartz samples.
Sample 03MB110
B110_01 SEM-CL. Image of plutonic, volcanic and metamorphic detrital quartz from the Mount Somers quartz sand quarry, Unit II.
B110_02 SEM-CL. Image of plutonic, volcanic and metamorphic detrital quartz from the Mount Somers quartz sand quarry, Unit II.
B110_03 SEM-CL. Image of plutonic, volcanic and metamorphic detrital quartz from the Mount Somers quartz sand quarry, Unit II.
B110_04 SEM-CL. Image of plutonic, volcanic and metamorphic detrital quartz from the Mount Somers quartz sand quarry, Unit II.
B110_05 SEM-CL. Image of plutonic, volcanic and metamorphic detrital quartz from the Mount Somers quartz sand quarry, Unit II.
B110_06 SEM-CL. Image of plutonic, volcanic and metamorphic detrital quartz from the Mount Somers quartz sand quarry, Unit II.
B110_07 SEM-CL. Image of plutonic, volcanic and metamorphic detrital quartz from the Mount Somers quartz sand quarry, Unit II.
B110_08 SEM-CL. Image of plutonic, volcanic and metamorphic detrital quartz from the Mount Somers quartz sand quarry, Unit II.
Sample 03MB111
B111_01 SEM-CL. Image of plutonic, volcanic and metamorphic detrital quartz from the Mount Somers quartz sand quarry, Unit II.
B111_02 SEM-CL. Image of plutonic, volcanic and metamorphic detrital quartz from the Mount Somers quartz sand quarry, Unit II.
B111_03 SEM-CL. Image of plutonic, volcanic and metamorphic detrital quartz from the Mount Somers quartz sand quarry, Unit II.
B111_04 SEM-CL. Image of plutonic, volcanic and metamorphic detrital quartz from the Mount Somers quartz sand quarry, Unit II.
B111_05 SEM-CL. Image of plutonic, volcanic and metamorphic detrital quartz from the Mount Somers quartz sand quarry, Unit II.
B111_06 SEM-CL. Image of plutonic, volcanic and metamorphic detrital quartz from the Mount Somers quartz sand quarry, Unit II.
Sample 03MB113
B113_01 SEM-CL. Image of plutonic, volcanic and metamorphic detrital quartz from the Mount Somers quartz sand quarry, Unit IV.
B113_02 SEM-CL. Image of plutonic, volcanic and metamorphic detrital quartz from the Mount Somers quartz sand quarry, Unit IV.
B113_03 SEM-CL. Image of plutonic, volcanic and metamorphic detrital quartz from the Mount Somers quartz sand quarry, Unit IV.
B113_04 SEM-CL. Image of plutonic, volcanic and metamorphic detrital quartz from the Mount Somers quartz sand quarry, Unit IV.
B113_05 SEM-CL. Image of plutonic, volcanic and metamorphic detrital quartz from the Mount Somers quartz sand quarry, Unit IV.
B113_06 SEM-CL. Image of plutonic, volcanic and metamorphic detrital quartz from the Mount Somers quartz sand quarry, Unit IV.
Sample 03MB114
B114_01 SEM-CL. Image of plutonic, volcanic and metamorphic detrital quartz from the Mount Somers quartz sand quarry, Unit IV.
B114_02 SEM-CL. Image of plutonic, volcanic and metamorphic detrital quartz from the Mount Somers quartz sand quarry, Unit IV.
B114_03 SEM-CL. Image of plutonic, volcanic and metamorphic detrital quartz from the Mount Somers quartz sand quarry, Unit IV.
B114_04 SEM-CL. Image of plutonic, volcanic and metamorphic detrital quartz from the Mount Somers quartz sand quarry, Unit IV.
Sample 03MB116
B116_01 SEM-CL. Image of plutonic, volcanic and metamorphic detrital quartz from the Mount Somers quartz sand quarry, Unit IV.
B116_02 SEM-CL. Image of plutonic, volcanic and metamorphic detrital quartz from the Mount Somers quartz sand quarry, Unit IV.
B116_03 SEM-CL. Image of plutonic, volcanic and metamorphic detrital quartz from the Mount Somers quartz sand quarry, Unit IV.
B116_04 SEM-CL. Image of plutonic, volcanic and metamorphic detrital quartz from the Mount Somers quartz sand quarry, Unit IV.
B116_05 SEM-CL. Image of plutonic, volcanic and metamorphic detrital quartz from the Mount Somers quartz sand quarry, Unit IV.
B116_06 SEM-CL. Image of plutonic, volcanic and metamorphic detrital quartz from the Mount Somers quartz sand quarry, Unit IV.
B116_07 SEM-CL. Image of plutonic, volcanic and metamorphic detrital quartz from the Mount Somers quartz sand quarry, Unit IV.
B116_08 SEM-CL. Image of plutonic, volcanic and metamorphic detrital quartz from the Mount Somers quartz sand quarry, Unit IV.
B116_09 SEM-CL. Image of plutonic, volcanic and metamorphic detrital quartz from the Mount Somers quartz sand quarry, Unit IV.
B116_10 SEM-CL. Image of plutonic, volcanic and metamorphic detrital quartz from the Mount Somers quartz sand quarry, Unit IV.
Sample 03MB118
B118_01 SEM-CL. Image of plutonic, volcanic and metamorphic detrital quartz from the Mount Somers quartz sand quarry, Unit V.
B118_02 SEM-CL. Image of plutonic, volcanic and metamorphic detrital quartz from the Mount Somers quartz sand quarry, Unit V.
B118_03 SEM-CL. Image of plutonic, volcanic and metamorphic detrital quartz from the Mount Somers quartz sand quarry, Unit V.
B118_04 SEM-CL. Image of plutonic, volcanic and metamorphic detrital quartz from the Mount Somers quartz sand quarry, Unit V.
B118_05 SEM-CL. Image of plutonic, volcanic and metamorphic detrital quartz from the Mount Somers quartz sand quarry, Unit V.
B118_06 SEM-CL. Image of plutonic, volcanic and metamorphic detrital quartz from the Mount Somers quartz sand quarry, Unit V.
B118_07 SEM-CL. Image of plutonic, volcanic and metamorphic detrital quartz from the Mount Somers quartz sand quarry, Unit V.
Data tables
Data tables with individual grain counts based on combined SEM-CL image and optical microscopy analysis for samples 03MB110, 03MB111, 03MB113, 03MB114, 03MB116, and 03MB118.
Repository S1. Photographs and data tables of quartz samples.
Download Zip (12.3 MB)Acknowledgements
We thank Kitty Milliken and Brad Field, as well as Senior Editor Nick Mortimer, for their constructive and helpful reviews and suggestions for improvements of the manuscript. We also gratefully acknowledge the support by Gary Webb of the Silica Sand Processing Plant, who granted us access to a Mount Somers sample location. We also thank Neil Andrews from the School of Biological Sciences, University of Canterbury, for his help with using the scanning electron microscope, and Robert Spiers from the Department of Geological Sciences for thin-section preparation.
Associate Editor: Dr Nick Mortimer.
Disclosure statement
No potential conflict of interest was reported by the authors.
Additional information
Funding
References
- Adams CJD, Oliver PJ. 1979. Potassium-argon dating of Mt Somers Volcanics, South Island, New Zealand: limitations in dating Mesozoic volcanic rocks. New Zeal J Geol Geophys. 22:455–463. doi: 10.1080/00288306.1979.10424153
- Allen PA. 1997. Earth surface processes. London: Blackwell Science Ltd.
- Basu A. 1985. Influence of climate and relief on compositions of sands released at source areas. In: Zuffa GG, editor. Provenance of arenites. Dordrecht: Reidel; p. 1–18.
- Bernet M, Bassett K. 2005. Provenance analysis by single-quartz-grain SEM-CL/optical microscopy. J Sediment Res. 75:492–500. doi: 10.2110/jsr.2005.038
- Bernet M, Kapoustos D, Bassett K. 2007. Diagenesis and provenance of Silurian quartz arenites in south-eastern New York State. Sediment Geol. 201:43–55. doi: 10.1016/j.sedgeo.2007.04.006
- Bernet M, van der Beek P, Pik R, Huyghe P, Mugnier JL, Labrin E, Szulc A. 2006. Miocene to Recent exhumation of the central Himalaya determined from combined detrital zircon fission-track and U/Pb analysis of Siwalik sediments, western Nepal. Basin Res. 18:393–412. doi: 10.1111/j.1365-2117.2006.00303.x
- Blatt H. 1967. Provenance determinations and recycling of sediments. J Sediment Petrol. 37:1031–1044.
- Bouchez J, Gaillardet J, Lupker M, Louvat P, France-Lanord C, Maurice L, Armijos E, Moquet J. 2012. Floodplains of large rivers: weathering reactors or simple silos? Chem Geol. 332:166–184. doi: 10.1016/j.chemgeo.2012.09.032
- Bowen M, Richardson K, Pinkerton M, Korpela A, Uddstrom M. 2004. Squeezing information from an elusive ocean: surface currents from satellite imagery. Water & Atmos. 12:26–27.
- Bradshaw JD. 1993. A review of the Median Tectonic Zone: terrane boundaries and terrane amalgamation near the Median Tectonic Line. New Zeal J Geol Geophys. 36:117–125. doi: 10.1080/00288306.1993.9514559
- Browne GH, Field BD. 1985. The lithostratigraphy of Late Cretaceous to early Pleistocene rocks of northern Canterbury, New Zealand. New Zealand Geological Survey Record 6. Lower Hutt: Department of Scientific and Industrial Research.
- Chandler FW. 1988. Quartz arenites: review and interpretation. Sediment Geol. 58:105–126. doi: 10.1016/0037-0738(88)90065-6
- Cipriani LE, Stone GW. 2001. Net longshore sediment transport and textural changes in beach sediments along the Southwest Alabama and Mississippi Barrier Islands, U.S.A. J Coast Res. 17:443–458.
- Curran J, Smith B, Warke P. 2002. Weathering of igneous rocks during shallow burial in an upland peat environment: observations from the Bronze Age Copney Stone Circle Complex, Northern Ireland. Catena. 49:139–155. doi: 10.1016/S0341-8162(02)00023-1
- De Ros LF. 1998. Heterogeneous generation and evolution of diagenetic quartz arenites in the Silurian–Devonian Furnas Formation of the Paraná Basin, southern Brazil. Sediment Geol. 116:99–128. doi: 10.1016/S0037-0738(97)00081-X
- Ellis J, Stone GW. 2006. Numerical simulation of net longshore sediment transport and granulometry of surficial sediments along Chandeleur Island, Louisiana, USA. Mar Geol. 232:115–129. doi: 10.1016/j.margeo.2006.05.001
- Field BD. 1989. Cathodoluminescence of quartz in Paleozoic to Mesozoic basement rocks and Cretaceous-Cenozoic sedimentary rocks, Canterbury and north Otago, New Zealand. New Zealand Geological Survey Report SL21.
- Field BD, Browne GH, Davy BW, Herzer, RH, Hoskins, RH, Raine JI, Wilson GJ, Sewell RJ, Smale D, Watters WA. 1989. Cretaceous and Cenozoic sedimentary basins and geological evolution of the Canterbury Region, South Island, New Zealand. New Zeal Geol Survey Basin Stud 2:1–94.
- Garver JI, Kamp PJJ. 2002. Integration of zircon color and zircon fission-track zonation patterns in orogenic belts: application to the Southern Alps, New Zealand. Tectonophysics. 349:203–219. doi: 10.1016/S0040-1951(02)00054-9
- Götze J, Zimmerle W. 2000. Quartz and silica as guide to provenance in sediments and sedimentary rocks. Contributions to Sediment Geol. 21:1–91.
- Hogg AJC, Seller E, Jourdan AJ. 1992. Cathodoluminescence of quartz cements in Brent Group sandstones, Alwyn South, UK North Sea. In: Morten AC, Haszeldine RS, Miles MR, Brown S, editors. Geology of the Brent Group. Geological Society [London] Special Publication. 61:421–440.
- Hollis CJ, Handley L, Crouch EM, Morgans HEG, Baker JA, Creech J, Collins KS, Gibbs SJ, Huber M, Schouten S, Zachos JC, Pancost RD. 2009. Tropical sea temperatures in the high-latitude South Pacific during the Eocene. Geology. 37:99–102. doi: 10.1130/G25200A.1
- Hollis CJ, Taylor KWR, Handley L, Pancost RD, Huber M, Creech JB, Hines BR, Crouch EM, Morgans HEG, Crampton JS, Gibbs S, Pearson PN, Zachos JC. 2012. Early Paleogene temperature history of the Southwest Pacific Ocean: reconciling proxies and models. Earth Planet Sci Lett. 349–350:53–66. doi: 10.1016/j.epsl.2012.06.024
- Hornibrook NdB. 1971. New Zealand Tertiary climate. New Zealand Geological Survey Report 47.
- Johnsson M, Stallard RF, Lundberg N. 1991. Controls on composition of fluvial sands from a tropical weathering environment: sands of the Orinoco River drainage basin. Geol Soc Am Bull. 103:1622–1647. doi: 10.1130/0016-7606(1991)103<1622:COTCOF>2.3.CO;2
- Johnsson MJ, Meade RH. 1990. Chemical weathering of fluvial sediments during alluvial storage: the Macuapanim Island point bar, Solimões River, Brazil. J Sediment Petrol. 60:827–842.
- Johnsson MJ, Stallard RF, Meade RH. 1988. First-cycle quartz arenites in the Orinoco river basin, Venezuela and Colombia. J Geol. 96:263–277. doi: 10.1086/629219
- Jourdan S, Bernet M, Tricart P, Hardwick E, Paquette JL, Guillot S, Dumont T, Schwartz S. 2013. Short-lived fast erosional exhumation of the internal Western Alps during the late Early Oligocene: constraints from geo-thermochronology of pro- and retro-side foreland basin sediments. Lithosphere. 5:211–225. doi: 10.1130/L243.1
- King PR. 2000. Tectonic reconstructions of New Zealand: 40 Ma to the Present. New Zeal J Geol Geophys. 43:611–638. doi: 10.1080/00288306.2000.9514913
- Landis CA, Coombs DS. 1967. Metamorphic belts and orogenesis in southern New Zealand. Tectonophysics. 4:501–518. doi: 10.1016/0040-1951(67)90014-5
- van der Lingen GJ. 1988. Lower Tertiary transgressive sediments of the Broken River Formation, Mt Somers area, Canterbury, New Zealand. New Zeal J Geol Geophys. 31:287–304. doi: 10.1080/00288306.1988.10417778
- McBride EF. 1963. A classification of common sandstones. J Sediment Petrol. 33:664–669.
- McBride EF. 1985. Diagenetic processes that affect provenance determinations in sandstone. In: Zuffa GG, editor. Provenance of arenites. Dordrecht: Reidel; p. 95–113.
- McLaren RG, Cameron KC. 1996. Soil science: sustainable production and environmental protection. Oxford: Oxford University Press.
- Milliken KL. 1988. Loss of provenance information through subsurface diagenesis, Plio-Pleistocene sandstones, northern Gulf of Mexico. J Sediment Petrol. 58:992–1007.
- Mitchell JK. 1993. Fundamentals of soil behavior. 3rd ed. Hoboken, NJ: John Wiley and Sons.
- Mortimer N. 1993. Geology of the Otago Schist and adjacent rocks. IGNS Geological Map 7, scale 1:500,000. Lower Hutt: GNS Science.
- Muir RJ, Ireland TR, Weaver SD, Bradshaw JD, Evans JA, Eby GN, Shelley D. 1998. Geochronology and geochemistry of Mesozoic magmatic arc systems, Fiordland, New Zealand. J Geol Soc London. 155:1037–1053. doi: 10.1144/gsjgs.155.6.1037
- Muir RJ, Weaver SD, Bradshaw JD, Eby GN, Evans JA. 1995. The Cretaceous Separation Point batholith, New Zealand: granitoid magmas formed by melting of mafic lithosphere. J Geol Soc. 152:689–701. doi: 10.1144/gsjgs.152.4.0689
- Muir RJ, Weaver SD, Bradshaw JD, Eby GN, Evans JA, Ireland TR. 1996. Geochemistry of the Karamea Batholith, New Zealand and comparisons with the Lachlan Fold Belt granites of SE Australia. Lithos. 39:1–20. doi: 10.1016/S0024-4937(96)00017-5
- Oliver PJ, Campbell JD, Speden IG. 1982. The stratigraphy of the Torlesse rocks of the Mt Somers area (S81) mid-Canterbury. J Roy Soc New Zeal. 12:243–271. doi: 10.1080/03036758.1982.10415348
- Oliver PJ, Keene HW. 1989. Sheet K36AC and part sheet K35, Mt Somers. Geological map of New Zealand 1:50,000. Wellington: Department of Scientific and Industrial Research.
- Pettijohn FJ, Potter PE, Siever R. 1987. Sand and sandstone. 2nd ed. New York: Springer-Verlag.
- Pocknall DT. 1989. Late Eocene to Early Miocene vegetation and climate history of New Zealand. J Roy Soc New Zeal. 19:1–18. doi: 10.1080/03036758.1989.10426451
- Powrie W. 2004. Soil mechanics: concepts and applications. 2nd ed. London: Spon Press.
- Rahl JM, Reiners PW, Campbell IH, Nicolescu S, Allen CM. 2003. Combined single-grain (U-Th)/He and U/Pb dating of detrital zircons from the Navajo Sandstone. Utah. Geology. 31:761–764. doi: 10.1130/G19653.1
- Raine JI, Wilson GJ. 1988. Palynology of the Mt Somers (South Island, New Zealand) early Cenozoic sequence. New Zeal J Geol Geophys. 31:385–390. doi: 10.1080/00288306.1988.10417785
- Sanderson ID. 1984. Recognition and significance of inherited quartz overgrowth in quartz arenites. J Sediment Petrol. 54:473–486.
- Selley RC. 1982. An introduction to sedimentology. 2nd ed. San Diego, CA: Academic Press.
- Shelley D. 1975. Metamorphic belt and volcanic arc migration in New Zealand. Nature. 258:668–672. doi: 10.1038/258668a0
- Smale D. 1987. Heavy minerals in Cretaceous sandstones in Canterbury. New Zealand Geological Survey Report SL17.
- Staub JR, Gastaldo RA. 2003. Late Quaternary sedimentation and peat development in the Rajang River delta, Sarawak, east Malaysia. In: Sidi FH, Nummedal D, Imbert P, Darman H, Posamentier HW, editor. Tropical deltas of southeast Asia—sedimentology, stratigraphy, and petroleum geology. SEPM Special Publication 76. Tulsa, OK: Society for Sedimentary Geology; p. 71–87.
- Suttner LJ, Basu A, Mack GH. 1981. Climate and the origin of quartz arenites. J Sediment Petrol. 51:1235–1246.
- Suttner LJ, Dutta PK. 1986. Alluvial sandstone composition and paleoclimate, I Framework Mineralogy. J Sediment Petrol. 56:329–345.
- Tappenden VE. 2003. Magmatic response to the evolving New Zealand margin of Gondwana during the mid-late Cretaceous [Unpublished PhD thesis]. Christchurch: University of Canterbury.
- Turnbull IM, Mortimer N, Craw D. 2001. Textural zones in the Haast Schist—a reappraisal. New Zeal J Geol Geophys. 44:171–183. doi: 10.1080/00288306.2001.9514933
- Waight TE, Weaver SD, Ireland TR, Maas R, Muir RJ, Shelley D. 1997. Field characteristics, petrography, and geochronology of the Hohonu Batholith and the adjacent Granite Hill complex, North Westland, New Zealand. New Zeal J Geol Geophys. 40:1–17. doi: 10.1080/00288306.1997.9514736
- White AF, Bullen TD, Schulz MS, Blum AE, Huntington TG, Peters NE. 2001. Differential rates of feldspar weathering in granitic regoliths. Geochim Cosmochim Ac. 65:847–869. doi: 10.1016/S0016-7037(00)00577-9
- Yang SY, Li CX, Yang DY, Li XS. 2003. Chemical weathering of the loess deposits in the lower Changjiang Valley, China, and paleoclimatic implications. Quater Inter. 117:27–34. doi: 10.1016/S1040-6182(03)00113-7
- Zinkrenagel U. 1978. Cathodoluminescee of quartz and its application to sandstone petrology. Contributions to Sedimentology 8. Stuttgart, Germany: E. Schweizerbart'sche Verlagsbuchhandlung.