ABSTRACT
Sandstone, mudstone and limestone samples dredged in the Reinga and Aotea basins, NW New Zealand during voyage TAN1312 provide age and lithological constraints on the Cretaceous–Neogene succession. A total of 46 micropaleontology and 7 macropaleontology samples were examined along with 84 thin-sectioned petrographical samples. Some were examined by X-ray diffraction and porosity-permeability analyses. Late Cretaceous sandstones are dominated by feldspathic and lithofeldspathic compositions, with mixed granitic plutoniclastic and volcaniclastic provenance; a comparison with Pakawau Group of Taranaki Basin is appropriate. Late Cretaceous–Paleogene mudstones are widespread and display close petrographical and age similarities to the Whangai Formation facies of other parts of New Zealand and fine-grained carbonate facies of the Weber and Amuri formations of eastern North and South Islands, respectively. Cretaceous limestone and Paleogene sandstone were not recovered. Carbonates and mudstones dominate the Neogene succession of Reinga and Aotea basins; rare Neogene sandstones have feldspatholithic compositions and resemble Waitemata Group sandstones of the northern North Island. In terms of petroleum prospectivity, Cretaceous sandstones represent a potential reservoir facies but are lithic with low permeability.
Introduction
New impetus is being given to exploration of the broader New Zealand Exclusive Economic Zone (EEZ) and the Extended Continental Shelf (ECS) for a variety of geo-political reasons (Glasby & Wright Citation1990; http://www.nzpam.govt.nz/cms/investors/our-resource-potential/petroleum/exploration-data-pack) to spur fundamental understanding of sedimentary basins (Uruski et al. Citation2008; Uruski Citation2011; Bland & Uruski Citation2012). The New Zealand Government has in recent years undertaken a series of regional 2D seismic-reflection surveys in several of our ‘frontier’ basins (such as the Great South, Raukumara and Pegasus basins). One of the prime goals of such surveys has been to document the seismic stratigraphy and the tectonic development of these basins and, from this, to determine the prospectivity for oil and gas exploration (Stagpoole et al. Citation2009, Citation2014). Such attempts depend to a large extent on the availability of subsurface well control, but wells are typically lacking or are at best limited in such frontier basins. Dredging to obtain rock samples is a relatively cheap and practical alternative method of obtaining samples for analysis in such frontier regions where tectonic deformation has exposed subsurface geology at the seabed.
In this paper we present descriptions of sedimentary rocks that were dredged in November 2013 from the seabed in Reinga and Aotea basins as part of the TAN1312 research survey using the RV Tangaroa. The area is located in the northwest of New Zealand, a 220,000 km2 frontier region with typical water depths in the range of 500–2500 m. The Reinga Basin lies on the margin of the Zealandia continent adjacent to oceanic crust of the Norfolk Basin (Herzer et al. Citation2009), whereas the Aotea Basin occupies a more internal position and was formerly closer to the interior of Gondwana. The Reinga (75,000 km2) and Aotea (145,000 km2) basins are bounded bathymetrically to the northeast by the South Norfolk Basin, Reinga Ridge and Northland and to the southwest by the Lord Howe Rise and Challenger Plateau (). The Reinga and Aotea basins lack well control, with the only wells drilled being Waka Nui-1 and Romney-1 which are southeast of the study region (). The purpose of the 2013 dredge survey was to collect representative rock samples from a range of geological structures defined by seismic and multibeam swath bathymetry data, from areas where Cretaceous–Cenozoic strata are assumed to exist (Bache et al. Citation2013a, Citation2014c). As a consequence most samples were recovered from structural highs and bathymetric ridges, or seamounts. A diverse range of rock types (including lavas) were dredged during the survey but, for the purposes of this paper, mudstones, sandstones and limestones of Cretaceous–Neogene age are described. Rocks resulting from diagenetic processes (e.g. concretions) as well as basement and igneous samples will be the subject of future studies.
Figure 1. Locations of dredge samples and one core collected during TAN1312 cruise, Reinga and Aotea basins. Each dredge location is prefixed by ‘d’ and a site number along the ship track (see ). Not all dredge sites are described in this paper. The one core site collected during TAN1312 and the locations of DSDP Sites 206, 207, 592 and 593 are also indicated. Parts of the coastline between the northern South Island and Northland is indicated for reference. The bathymetry used is taken from CANZ (Citation2008). Inset map of Zealandia shows the location of our study area.
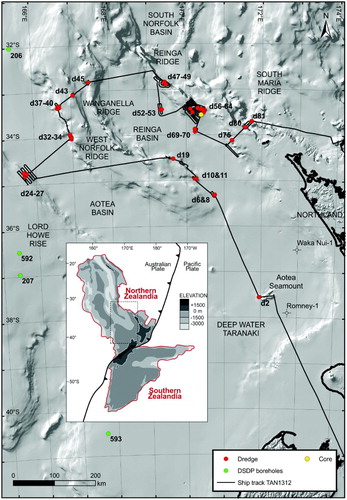
The regional and tectonic history of the area is described elsewhere (Herzer et al. Citation1997, Citation2009; Bache et al. Citation2012a, Citation2012b, Citation2013a, Citation2013b, Citation2014a, Citation2014c). In brief, the Reinga and Aotea basins are underlain by the extrapolated extent of onland New Zealand basement terranes. Based on seismic interpretation, both basins are typical Zealandia sedimentary basins in that they contain strata of the Late Cretaceous–Holocene rift-transgressive-regressive Zealandia Megasequence (King et al. Citation1999). Reinga Basin is one of the few New Zealand basins to have experienced widespread Paleogene contraction, interpreted to record the initiation of subduction at the Zealandia continent–ocean boundary. That subduction margin was later modified by Miocene opening of the South Norfolk Basin.
In this essentially descriptive paper we present the details of analytical work carried out on rock samples collected during the TAN1312 cruise, provide lithological and age control for a subset of samples, integrate these results with pre-existing dredge surveys, and relate these results to the onshore and nearshore geology of mainland New Zealand. The results have important implications for understanding the seismic stratigraphy and petroleum potential of the area because very few dredge samples have been collected previously.
Previous work
Previous work in the Reinga and Aotea basins has included the acquisition and interpretation of widely spaced 2D seismic reflection surveys (Herzer et al. Citation1997; Stagpoole et al. Citation2009; Uruski et al. Citation2010; Bache et al. Citation2012a, Citation2012b, Citation2013a, Citation2013b, Citation2014a, Citation2014c) and a seismic database (Sutherland et al. Citation2012). Lithological and organic source-rock data were previously published for the area, based on two dredge sample sites collected during 1993 (Herzer et al. Citation1997, Citation1999) and by the French Institute of Research for Development and Cooperation (ORSTOM; Herzer et al. Citation1999). The stratigraphies at Deep Sea Drilling Program (DSDP) sites in the Tasman Sea also have relevance to this study. In 1973 the DSDP drilled Sites 206 and 207 and in 1982 Site 592 west of the study area along the Lord Howe Rise and in New Caledonia Basin (Shipboard Scientific Party Citation1973a, Citation1973b, Citation1986a). In 1982, Site 593 was drilled south of the study area on the Challenger Plateau (Shipboard Scientific Party Citation1986b). The present paper is based on data collected during the TAN1312 survey, which included continuous gravity and magnetic data (Stagpoole et al. Citation2014), multibeam swath bathymetry surveys at sample and other sites (Bache et al. Citation2014c), as well as reporting on the source-rock potential of the samples collected (Ventura Citation2014). A full description of the expedition and the initial results of the survey were published by Bache et al. (Citation2014c), Browne et al. (Citation2014) and Lawrence et al. (Citation2014).
Methods
Specific dredge sites were selected on the basis of regional 2D seismic reflection lines available prior to the cruise and from multibeam swath bathymetry charts acquired during the TAN1312 survey (Bache et al. Citation2014c). Dipping strata, anticlines, unconformities and acoustic basement were variously targeted. No new seismic reflection data were acquired during the survey. Dredge locations are presented in and in Table S1. Core collection was attempted with limited success at one site on the eastern Reinga Ridge (). A steel rock dredge with a 0.45 × 1.2 m mouth was used over target locations to collect samples. At some sites, multiple dredge samples were collected at different water depths along the same profile so that multiple stratigraphic horizons could be sampled in discrete dredge recoveries. In all, 45 locations were dredged; of these, 37 localities yielded samples, amounting to approximately 2 m3 of rock. Dredge locations are indicated by a small ‘d’ site number (as shown in and Table S1), as well as a ‘P’ prefix referring to the online New Zealand Petlab database (for details see http://pet.gns.cri.nz).
Once on board the ship, samples were cut using a diamond saw and sorted into manageable portions for hand-specimen description and cataloguing. In the post-expedition phase, subsamples were selected for a range of paleontological, petrographical, X-ray diffraction (XRD) of mudrock samples, porosity and permeability, and source-rock analyses, which are reported either here or in Browne et al. (Citation2014) or Ventura (Citation2014). Care was taken not to analyse obviously weathered or otherwise altered (such as Mn encrusted) samples. A total of 46 micropaleontology samples (11 samples for dinoflagellates, 26 samples for foraminifera, 9 samples for radiolaria) and 7 macropaleontology samples were examined as part of this study, as the prime means to determine sample age. Age control is based mainly on the biostratigraphic datums summarised by Cooper (Citation2004), which have been recalibrated to the 2012 international geological timescale by Raine et al. (Citation2015). A total of 84 samples were thin-sectioned and examined petrographically (Table S1). A subset of 10 sandstone samples were point-counted after staining for potassium feldspar, and counted using the Gazzi–Dickinson method (Ingersoll et al. Citation1984) but without specific note of volcanic texture subtypes. A total of 29 of the thin-sectioned samples were carbonates. These were stained with Alizarin red to enhance calcite identification. Carbonate samples were classified according to their major bioclastic constituents after the classification schemes of Dunham (Citation1962) and Folk (Citation1962). Carbonate components were visually estimated. A total of 16 samples were examined by XRD, 12 of which were mudstones and 4 were concretions. Porosity and permeability measurements were initially made on 9 core plug samples at GNS Science, Lower Hutt, and 5 of these were later re-tested at CoreLabs, New Plymouth.
Stratigraphy
The key to improving the stratigraphy of the Reinga and Aotea basins has been the paleontological dating of samples and the use of a range of taxonomic groups to determine sample age. These results are summarised in . The taxonomic group selected for a given sample was based on lithology, using the most likely fossil group to yield an age and a paleoenvironmental determination for a given lithology type. During dredge sampling a range of lithologies is often collected. This is reflected in the paleontologically determined ages, with a mix of ages recorded for several samples at some sites. Multiple ages possibly result from mass-wasting of younger formations downslope, these rocks becoming intermixed in the dredge with older samples. Potentially, a range of lithologies could also be collected during the time (several minutes) when the dredge is on the seabed. In this paper we have used the oldest recorded age as the adopted age of each sample, and these are shown in .
Figure 2. A, Locations of dredge samples from Reinga and Aotea basins with their paleontologically determined ages (by epoch). Further details on sample location and age determinations are provided in . Squares indicate TAN1312 cruise samples (this study), while pre-existing dredge sample ages are depicted as triangles (from Herzer et al. Citation1997, Citation1999). Latitude and longitude coordinates are in NZTM2000 projection. B, Interpreted seismic reflection profile (line REI09-14) through the West Norfolk Ridge and Reinga Basin (location of line shown in A). Seismic units shown relate to nomenclature presented in . The line shows how dredge sites were selected to sample older stratigraphies, in this case, an anticline of folded seismic unit U4 strata (dredge locations d6 and d8). Figure from Bache et al. (Citation2012b).
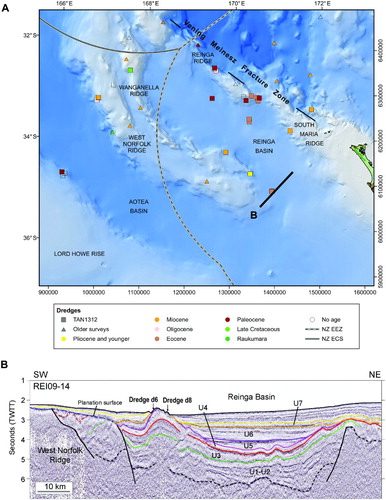
Table 1. Summary of paleontological results.
Past seismic interpretations have defined a series of stratigraphic units (U1–8; Bache et al. Citation2012a, Citation2012b), although the age and lithology of most of these are poorly constrained. These stratigraphic units are inferred to relate to major tectonically driven basin-filling phases (Phases 1–6 of Bache et al. Citation2012a, Citation2012b) during Cretaceous–Holocene time (). This stratigraphic hierarchy has been adopted here. We also relate the succession to the recently published high-level lithostratigraphy of Mortimer et al. (Citation2014). This paper presents the first set of integrated, dated, lithologically described samples to constrain the stratigraphy of the Reinga and Aotea basin region. We describe these rock types below, arranged by age.
Figure 3. Seismo-stratigraphic units in the Reinga and Aotea basins and wider Tasman Frontier (the area between New Caledonia and New Zealand), showing corresponding local and regional geological phases (after Bache et al. Citation2012a, Citation2012b) and regional lithostratigraphic correlatives (after Mortimer et al. Citation2014). The age distribution of sandstones, mudstones and limestones (this study) are also shown.
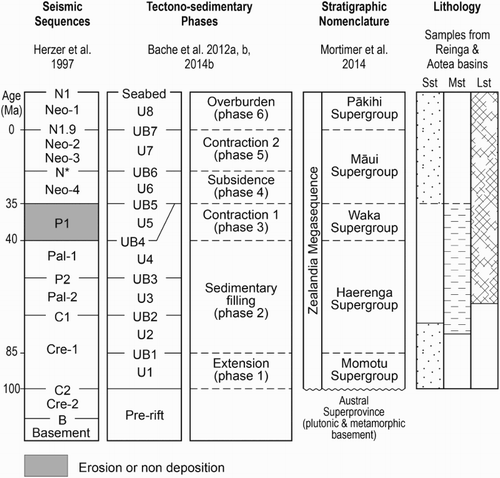
Cretaceous
Sandstones
Only one sample, P83211 (SE32169/f4), was dated (Haumurian) in our survey (), and we have inferred Late Cretaceous ages for other sandstone samples based on the Late Cretaceous (Raukumara) age provided by Herzer et al. (Citation1997) and Herzer et al. (Citation1999) for samples which these authors collected from nearby geographic sites (our sites d45 and d34 on the West Norfolk Ridge). For our petrographic analysis, we have used samples collected in the TAN1312 survey as well as one sample (P57038) collected from the West Norfolk Ridge by Herzer et al. (Citation1999) (Table S2).
Raukumara-aged sandstones (five samples) are pale olive-grey to grey, moderately well to poorly sorted fine-grained to pebbly arkosic sandstones (; Table S2). Compositionally, these samples are mostly feldspathic and lithofeldspathic sandstones (). Quartz grains make up 20–27% of the rock, and feldspar comprises over 38% in modal analyses (). The ratio of potassium feldspar to total feldspar is variable (0.17–0.54), while sample P57038 contains the highest potassium feldspar/total feldspar ratio of any sandstone in our dataset (0.54). In terms of rock fragments (lithics), the samples are rich in volcanic grains (A, B). Based on cross-hatch twinning of K-feldspar, perthitic textures, composite quartz-feldspar grains and abundant coarse micas, the sandstones have a clear granitic plutoniclastic as well as a volcaniclastic provenance. Volcanic lithics commonly have a felsitic texture indicative of silicic volcanism. In some samples, the porosity has been accentuated by the dissolution of plagioclase and by the alteration of detrital biotite grains along cleavage planes (C, D). All these sandstone samples were collected from the West Norfolk Ridge, and hence may not be representative of other sandstones of this age in the region. Studies in Taranaki Basin have shown that the mineralogy of Cretaceous sandstones strongly reflects the mineralogy of the local basement terranes from which they were derived (Mortimer et al. Citation1997; Browne et al. Citation2008; Higgs et al. Citation2010).
Figure 4. QFL (quartz–feldspar–lithics) ternary plot for sandstones of Reinga and Aotea basins plotted according to age. The Gazzi–Dickinson method was used in these point counts (see – for details).
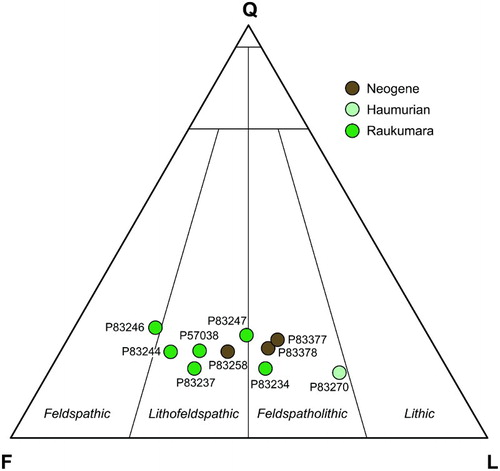
Figure 5. Thin-sections of typical sandstones. Abbreviations: Bt, biotite; Cp, primary carbonate; Cs, secondary carbonate; Glc, glauconite; Pl, plagioclase feldspar; Kf, potassium-feldspar; Ls, sedimentary lithic; Lv, volcanic lithic; Qz, quartz; V, void space (porosity). A, Inferred mid-Cretaceous age moderately sorted lithofeldspathic sandstone. Note the large volcanic lithic grain (dashed line, upper left). Sample P83244, West Norfolk Ridge. Plane-polarised light. B, Mid-Cretaceous age sandstone that is a moderately well-sorted lithofeldspathic sandstone. Sample P57038 (dredge RE9302-5-1 of Herzer et al. Citation1999), West Norfolk Ridge. Plane-polarised light. C, Same view of sample P57038 under cross-polarised light. D, Moderately well-sorted feldspatholithic sandstone of Late Cretaceous age. This sample includes primary carbonate macrofossils and secondary carbonate in the form of sparry calcite. No detrital biotite is present. Sample P83270, Wanganella Ridge. Plane-polarised light. E, Same view of sample P83270 under cross-polarised light. F, Higher magnification of sample P83270 with an example of locally present, well-rounded glauconite. Plane-polarised light. G, Same view of sample P83270 under cross-polarised light.
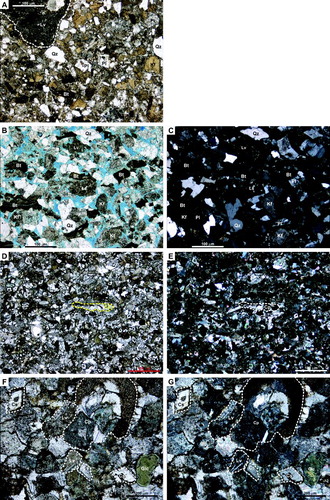
Table 2. Point count data for ten sandstone samples using the Gazzi–Dickinson method.
The Haumurian sandstone sample collected in this study (P83270) from the Wanganella Ridge is a yellowish moderately sorted, sub-angular to sub-rounded calcareous fine-grained feldspatholithic sandstone, almost classified as a lithic sandstone (; , 5E–H). Detrital potassium feldspar is low (potassium feldspar: total feldspar ratio is 0.17; ), significantly lower than the more westerly (and inferred older) sandstones from the West Norfolk Ridge (Herzer et al. Citation1999). This sample includes a small proportion (6%) of primary carbonate microfossils and 38% secondary sparry carbonate. Volcanic lithics are common and have vitric rather than holocrystalline textures, indicating that they result from basaltic volcanism. Detrital mica is absent, but rounded glauconite pellets are present (E–G).
Mudstones
The single directly dated Cretaceous mudstone (sample P83271) from the Wanganella Ridge is Haumurian in age and is a well-laminated, very fine sandy quartzose and micaceous siltstone (A). The laminae are caused by slight textural changes in grain size and sorting and by sub-parallel-oriented carbonaceous debris lying on bedding planes. Quartz grains are up to fine sand size, typically angular to sub-angular grains, and make up about 5–10% of the rock. Mica (muscovite) grains tend to be no larger than very fine sand size. Benthic and planktic foraminifera with tests infilled with micrite and foraminiferal fragments make up <1% of the thin-section. Plagioclase and glauconite are accessory minerals. Brown, semi-circular structures up to 6 mm diameter infilled with mud occur in parts of the thin-section and are interpreted as burrows. Bulk XRD results for this sample indicate the presence of montmorillonite, kaolinite, muscovite, quartz, calcite and plagioclase.
Figure 6. Thin sections of typical mudstones. A, Haumurian-aged mudstone showing well-laminated, very fine sandy quartzose and micaceous texture. Sample P83271, Wanganella Ridge. Plane-polarised light. B, Late Teurian mudrock displaying a bioturbated fabric comprising ‘sub-horizontal’ or broadly parallel to bedding burrow structures (darker areas). The dark brown patches are possibly Mn-oxides. Sample P83279, north Reinga Ridge. Plane-polarised light. C, Bedded very fine sandy bioturbated non-calcareous Waipawan (early Eocene) siltstone. The sample has very fine-grained detrital quartz, and green and brown glauconite as well as fine-scale Mn contamination. Bioturbation is indicated by the paler colour mottling. Sample P83330, south Reinga Ridge. Plane-polarised light.
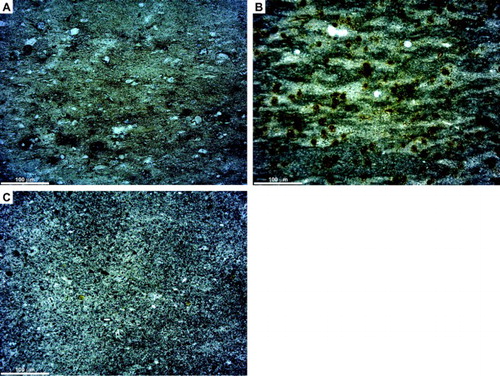
Foraminifera in this sample (SE32167/f7; P83271) include the planktic species Rugoglobigerina macrocephala and Globigerinelloides volutus, and the benthic species Bulimina rakauroana, Bolivina incrassata, Bolivinoides dorreeni, Frondicularia rakauroana and Planulina rakauroana, indicating a late Haumurian age (c. 80–66 Ma). The benthic species Stensioina beccariiformis and Rzehakina epigona indicate a deep upper bathyal (400–600 m) paleodepth. Planktic species are rare, reflecting a sheltered inner neritic water mass.
Limestones
No Cretaceous limestones were recovered in the survey, but we note that chalk of Late Cretaceous (Haumurian) age was recovered from the base of DSDP Site 208 north of the study area (Shipboard Scientific Party Citation1973c).
Paleogene
Sandstones
No Paleogene sandstones were recovered in our survey. This may simply reflect a sampling bias of our dredge locations, or it may indicate the region was not receiving sand from landmasses capable of providing sand. Sedimentary rocks of this age are represented by fine-grained mudstones (Stagpoole et al. Citation2014).
Mudstones
Several mudstone samples are confirmed as Paleocene–early Eocene in age (). Those examined petrographically include samples P83230 (from the same dredge as sample P83231; early Teurian), P83279 (late Teurian; B) and P83330 (Waipawan–Heretaungan; C). The oldest of these, sample P83230 from the Lord Howe Rise, is a very fine sandy micaceous non-calcareous siltstone. Detrital grains comprise angular quartz (up to very fine sand size), muscovite and brown biotite. Mica is common, and grains are roughly aligned parallel to bedding. Brown-stained burrows up to 2 mm diameter are infrequent, and are infilled with mudstone. Bulk XRD results for this sample indicate the presence of montmorillonite and kaolinite.
The late Teurian sample (P83279) from the northern Reinga Ridge is a micro-bedded, very fine non-calcareous sandy claystone (B). Manganese encrustation has been pervasive and gives the rock a mottled, streaky appearance. Some of the manganese alteration may be following original burrows, but this is not always clear. Detrital sand grains make up <1% of the rock, and comprise muscovite and quartz. Planktic foraminifera are common and are infilled with sparry calcite. Brown mottling is common throughout. Bulk XRD results for this sample indicate the presence of montmorillonite, kaolinite and muscovite/illite clays as well as quartz and calcite.
The youngest sample of this group (P83330) from the southern Reinga Ridge is a laminated very fine bioturbated non-calcareous sandy siltstone with a strong overprint of manganese encrustation (C). Detrital grains are dominated by very-fine-grained quartz (up to 120 µm diameter) that make up <10% of the rock, as well as green and brown very-fine-grained glauconite sand (<5%). The sediment is strongly mottled by semi-circular burrows up to 4 mm diameter, infilled with mud. Rare mud-filled planktic foraminifera are present throughout, and are partially altered by the manganese overgrowth.
Four of the mudstone samples submitted for palynological analysis yielded excellent dinoflagellate assemblages with well-constrained ages ranging from early Teurian to middle Teurian (dinoflagellate zone NZDP5 to NZDP7 of Crouch et al. Citation2014). The oldest, sample SE34165/f2, is early Teurian in age (dinoflagellate zones NZDP1 to NZDP2 probably the early part of the latter) based on the presence of Trithyrodinium evittii and Palaeoperidinium pyrophorum, and the absence of Alterbidinium pentaradiatum. Sample SE33169/f3 is dated less certainly by the presence of Alterbidinium pentaradiatum and Cerodinium striatum, which indicate an early Teurian age (dinoflagellate zones NZDP2 to NZDP5). Sample SE33170/f19 is probably from the early part of the late Paleocene (dinoflagellate zone NZDP6), based on the co-occurrence of Isabelidinium cingulatum and Deflandrea foveolata, although the latter identification is tentative, and the absence of Alterbidinium pentaradiatum. The final sample, SE33170/f14 is also early late Teurian (zones NZDP5 to NZDP7) based on the presence of Deflandrea foveolata.
Of particular note is the presence of an unidentified and probably new taxon Apteodinium ‘trifolliculum’ (manuscript name), which unusually appears to possess three wall layers. It occurs in sample SE33170/f14 and is presently known from only two other localities: the Waipawa Formation outcrop at Triangle Farm, northern Wairarapa, and from an apparently gradational interval encompassing the Upper Calcareous Member of the Whangai Formation and the Waipawa Formation in the lower part of the Taylor White Section, upper Angora Road, southern Hawke’s Bay (GNS Science, unpublished records). In both cases, the age is considered to be early late Teurian.
Sample SE33170/f19 (P83344) also yielded some taxonomic novelties, Impagidinium agremon and Pyxidinopsis epakros, both formerly known from a single study of late Haumurian–Teurian sedimentary rocks from the Clarence Valley, Marlborough (Willumsen Citation2011). The stratigraphical range of Impagidinium agremon straddles the Haumurian–Teurian boundary, and that of Pyxidinopsis epakros includes at least the earliest Teurian. However, neither range is well constrained at present.
The 11 foraminifera-bearing mudstone samples that yielded Eocene ages are too numerous to detail here (further details provided in Browne et al. Citation2014). Generalisations that can be made are that seven of the ages determined from foraminifera often have widely bracketed Waipawan to Porangan (early–middle Eocene) ages and generally indicate bathyal depositional conditions, either upper bathyal (200–400 m) or deep middle bathyal (800–1000 m). Only sample SE33170/f17 (P83338) from the north side of the Reinga Ridge indicates a shallow-water depositional setting (middle shelf) based on the foraminiferal data. Unlike the Paleocene samples, Eocene foraminiferal assemblages are dominated by planktic species which comprise over 95% of many samples, indicating open-oceanic–water-mass conditions during deposition. Typical planktic species include Morozovella crater, Morozovella lensiformis, Acarinina primitiva, Globanomalina australiformis and Subbotina spp. in the early–middle Eocene and Globigerinatheka index, Acarinina primitiva and Acarinina collactea in the middle–late Eocene.
Limestones
Ten limestone samples dated as Paleogene in age were recovered in the survey; most were dated using foraminifera and one was dated radiolarian (, ).
Figure 7. Thin-sections of typical Paleogene limestones. All samples have been stained to reveal calcite and all images are in plane-polarised light. A, Packed biomicrite comprising a mixture of intact and fragmentary foraminifera in a micrite matrix. In this example, foraminiferal tests dominantly contain micrite, although sparry calcite occurs in some. The bivalve fragment (Bi) displays evidence of boring and is coated with a greenish-brown material that is possibly Mn-oxide. Sample P83287, North Reinga Ridge. B, Detailed view of sparry calcite fill in an intact foraminiferal test in a packed biomicrite. Sample P83331, South Reinga Ridge. C, Coarse, bioclastic grainstone comprising almost exclusively large (>millimetre scale) bryozoan skeletal debris. Sample P83197, Wanganella Ridge. D, Coarse bioclastic grainstone comprising predominantly foraminiferal material and what appear to be micritic intraclasts (Mi). Variably sized sparry calcite (arrows) is locally present. Note the rounded glauconite grain (Glc). Sample P83338, South Reinga Ridge. E, Sections through bryozoa displaying intra-particle porosity along with possible partial geopetal micrite infill. Sparry cements (arrows) either partially or completely fill intra-particle pores. The micrite matrix has a pelloidal appearance. Packed biomicrite. Sample P83183, Volcano ‘A’. F, Slightly thick slide displaying echinoderm debris (Ec), planktic foraminifera (Pf), rryozoa (Br) and a bivalve fragments (Bi) that have dissolved leaving large unconnected, mouldic pores. Packed biomicrite. Sample P83170, Anticline ‘A’. G, Typical example of a sparse biomicrite comprising scattered foraminifera (mainly planktic) in a micrite matrix. The dark irregular masses (arrows) are probably Mn-oxides. Sample P83303, Anticline ‘C’. H, Detail of micrite in a fossiliferous micrite with remnant planktic foraminiferal material (Pf). The micrite is relatively coarse grained compared to other micrite examples in , suggesting possible recrystallisation to microspar. Sample P83347, Anticline ‘D’.
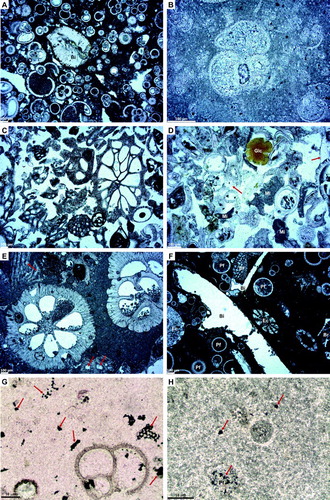
Table 3. Summary of limestone samples.
The oldest limestones (P83287, Paleocene–early Eocene; P83331: early Eocene) are fine-grained, bioclastic packstones (FBP) dominated by intact or fragmentary foraminifera (A). Planktic foraminifera are significantly more abundant than benthic foraminifera. Other skeletal components typically constitute less than 10% of the rock and comprise scattered, fragmentary bryozoa, echinoderm plates and spines, bivalves and rare algal material. Detrital material is typically rare to absent. Diagenetic alteration in FBP samples comprises sparry calcite filling of some foraminiferal chambers (B) and local micritisation of foraminiferal chamber walls. Scattered, small glauconite grains were also observed in sample P83287.
The only limestone sample that yielded radiolaria was sample SE35170/f1 (P83167), a chalky micritic limestone that contained a rich, moderately well-preserved assemblage. Its age is Mangaorapan–early Heretaungan (late early Eocene), based on the co-occurrence of Lychnocanium bellum (First Occurrence 53 Ma), Buryella tetradica (Last Common Occurrence 51 Ma), Phormocyrtis striata striata and Lithochytris archaea (Last Occurrence 48 Ma).
Eocene–Oligocene limestones dredged on TAN1312 display the complete range of grain sizes from coarse to fine. Coarse bioclastic grainstone to packstone (CBGp) samples (e.g. samples P83197 and P83338) are characterised by large (>millimetre scale) skeletal debris that includes material derived from bryozoans, bivalves, echinoderms, gastropods, foraminifera, algae and rare sponge spicules (C). The size of skeletal debris distinguishes CBGp samples from FBP samples. Bryozoan material is generally most abundant with echinoderm and bivalve material being subordinate, except in sample P83338 which is dominated by foraminifera (D). Detrital sand-sized grains constitute up to 10% of the rock volume (2–5% in most samples) and predominantly comprise quartz, but can also include feldspar plus minor chert and volcanic rock fragments. Detrital grains are typically sub-angular to sub-rounded. Glauconite occurs as rounded grains in interparticle pores (D), but can also occur within framework pores.
Coarse, bioclastic packstone (CBP) samples (P83170, P83175, P83183) have similar faunal assemblages to the CBGp samples (E, F) described above, but may contain common foraminifera (F). The amount of micrite can be significant and in some samples appears pelloidal. In one sample (P83170) skeletal material has been altered to micrite. Wackestone (WST) samples (P83303 and P83347) are fossiliferous micrites to sparse biomicrites (G, H) with skeletal material restricted to planktic foraminifera. Benthic foraminifera are generally rare or absent. There is local evidence of pelloidal micrite, bioturbation and some of the micrite grains are recrystallised microspar (H).
Neogene
Sandstones
Three sandstones of Neogene age were recovered in the survey. Sample P83258 from West Norfolk Ridge is poorly dated as Miocene–Holocene (using bivalves and gastropods), whereas samples P83377 and P83378 from North Maria Ridge are of latest Oligocene–early Miocene age (using foraminifera; ). Sample P83258 is a breccia with a limestone matrix. Breccia clasts comprise pale-brown, moderately well-sorted, sub-angular, very-fine-grained lithofeldspathic sandstone, but the rock is intensely penetrated by opaque manganese oxides (). Quartz, plagioclase, potassium feldspar and volcanic lithics are present. Samples P83377 and P83378 are pale olive-grey, weakly laminated, moderately to well sorted, sub-rounded to sub-angular, fine-grained feldspatholithic sandstones from the same dredge (A). The main detrital grains are quartz, plagioclase, potassium feldspar, sedimentary lithics (mudstone), lath-textured volcanic lithics and glauconite. Rare but conspicuous green and brown amphibole grains are present. Biotite is present but rare in sample P83377, and absent from sample P83378. An andesitic volcanic provenance is inferred, based on the mineralogy and rock fragment composition. In thin-section the sandstone is moderately porous with sparry calcite filling in some, but by no means all, of the pore space.
Figure 8. Thin-sections of typical Neogene sandstone and mudstone and two Cretaceous sandstones; all images in plane-polarised light. Abbreviations: Bt, biotite; Glc, glauconite; Pl, plagioclase feldspar; Kf, potassium-feldspar; Ls, sedimentary lithic; Lv, volcanic lithic; Qz, quartz; Pf, planktic foraminifera; M, mud matrix. A, Waitakian (latest Oligocene–earliest Miocene) sandstone comprising quartz, plagioclase, K-feldspar, sedimentary lithics (mudstone), lath-textured volcanic lithics and glauconite. Rare but conspicuous green and brown amphibole grains are present. Sample P83377, South Maria Ridge. B, Waitakian (latest Oligocene–earliest Miocene) very fine sandy bioturbated calcareous siltstone. Detrital grains include angular quartz, green biotite and rare glauconite. Planktic foraminifera are relatively common throughout. Sample P83380, South Maria Ridge. C, Fine-grained Cretaceous sandstone with a fine-grained mud matrix. This sample had a measured porosity of 27.5% and permeability of 1.1 mD, the low permeability thought to be due to the matrix. Sample P83234, West Norfolk Ridge. D, Cretaceous sample with a measured porosity of 23.8 and 27% and permeability of 3.4 mD. Although the sample has porosity, the result of both depositional sorting and its coarse-grained nature, and secondary porosity due to partial dissolution of feldspar grains and bending of biotite, it has a low permeability, probably because the pore spaces are not well interconnected. Sample P83246, West Norfolk Ridge.
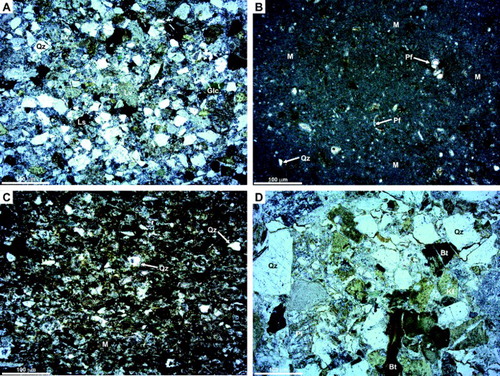
Mudstones
Only one dated Neogene (late Waitakian) mudstone sample from North Maria Ridge was examined petrographically (P83380). It is a laminated very fine sandy bioturbated calcareous siltstone (B). Detrital grains include angular quartz grains (very fine sand) comprising <2% of the rock, as well as green biotite and glauconite. Planktic foraminifera are relatively common throughout, their tests typically filled with micritic mud or less commonly by calcite spar. Bioturbation takes the form of brown-stained circular structures up to 8 mm in diameter, infilled with siltstone, giving a general mottled appearance throughout the slide. Some burrows are infilled with fine-grained calcite. Dark grains are likely to be fine-grained carbonaceous debris, with some grains preferentially aligned parallel to bedding. Fractures are generally rare, but when present display partial filling by sparry calcite. Bulk XRD results for this sample indicate the presence of montmorillonite, kaolinite and muscovite/illite clays as well as quartz, titanomagnetite and calcite.
Foraminifera were identified in eight Neogene samples (). One of these sites (SE34169/f2; P83202) indicates inner to mid-shelf (20–100 m) water depths, but the remainder indicate upper bathyal (200–400 m) to deep lower bathyal water depths (1500+ m) and range in age between Oligocene (Whaingaroan) and Holocene. Planktic taxa such as Globigerina sp., Globorotalia sp. and Globoquadrina are much more abundant than benthics such as Amphistegina, Ehrenbergina, Elphidium and Operculina in most samples, and suggest fully open-oceanic conditions (details in Browne et al. Citation2014).
Limestones
Four dated Neogene limestone samples were recovered in the survey (), and range in age from early Miocene to early Pliocene. They comprise CBGp and FBP limestone types (two samples of each), similar to the Paleogene limestone types described above (A, B) with a few differences. Unlike the Paleogene limestones, sample P83364 contains micrite (A) and P83202 has noticeably more algal material than any other sample (B). Sample P83253 is a tightly packed micrite not unlike the Paleogene limestones (C). Sample P83257 is the only Pliocene limestone, has additional skeletal components that are not present in equivalent Paleogene FBP types and also contains a solitary sponge spicule (D). Sample P83364 (SE34171/f3–6, 10, 13, 14) has a diverse mid-shelf macrofauna, including aragonitic bivalves and gastropods now preserved as moulds, and well-preserved specimens of the large scallop Serripecten yahliensis (formerly known in New Zealand as S. hutchinsoni; Beu & Darragh Citation2001) of early–late Miocene age (Altonian–Tongaporutuan). It also includes warm-water epifaunal bivalves (Spondylus, Chama) and warm-water gastropods (Cypraea, Morum). Common moulds of Cucullaea are considerably smaller than New Zealand species, and resemble Australian Neogene species. The fauna has as much in common with southern Australian Miocene fauna as with New Zealand fauna.
Figure 9. Thin sections of typical Neogene limestones. All samples have been stained to reveal calcite; all images in plane-polarised light. A, Bioclasts comprise echinoderm (Ec), bryozoan (Br) and bivalve (Bi) material. Intra- and interparticle porosity may be filled with fine to coarse sparry calcite (red arrows). A mould, probably of a bivalve, is partially filled with smaller sparry calcite crystals (green arrows) but still retains some porosity (Po). Note that some of the matrix is micritic in this view, although this is not the case elsewhere in the section. A solitary, angular quartz grain is also present. Measured porosity is in the range 6.4–23.5% and probably results from a mixture of intra-particle and dissolution pores. High permeability (580 mD) probably reflects local connectivity between dissolution pores. Packed biomicrite. Sample P83364, southwestern side of the South Maria Ridge. B, Bryozoa (Br) with common intra-particle porosity (Po) and a large fragment of algal material (Alg). Poorly washed biosparite. Sample P83202, Wanganella Scarp. C, Abundant clast-supported planktic foraminifera with scattered benthic foraminifera in a packed biomicrite. Foraminiferal chambers are commonly empty. Sample P83253, West Norfolk Ridge. D, Packed biomicrites displaying matrix- to clast-supported planktic foraminifera plus a solitary sponge spicule (Sp). Sample P83257, West Norfolk Ridge.
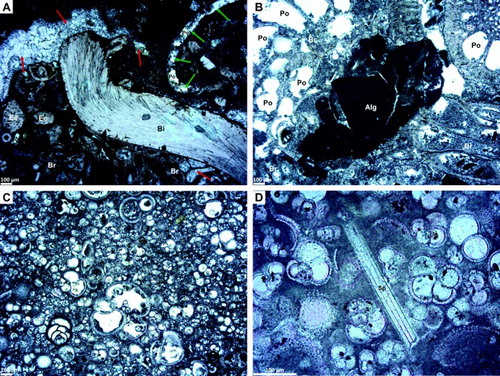
Porosity and permeability results
Five sandstones and two limestones were analysed for porosity and permeability properties at GNS, and five of these analyses were replicated at CoreLabs in New Plymouth (). Although these data are limited, porosity values are up to 27% for sandstones and 26% for limestones, with an average of 18% for both. Porosity values are comparable between the two labs, though the values determined are slightly lower in the GNS Science analyses. This might be expected given that the individual samples were different, and because the orientations at which the core plugs were cut possibly differed with respect to bedding or other rock defects. The exception is sample P83364 from Reinga Ridge, which has a porosity of 6.4% in the GNS Science analyses and 23.5% in those from CoreLabs. The most likely reason for this is the vuggy (porous) nature of the limestone, the sample tested at GNS Science being less vuggy than the sample tested at CoreLabs. Visual estimates of porosity made from thin-sections are typically lower than the measured porosity values (), and may relate to intragranular porosity not being fully recognised in the thin-sections.
Table 4. Porosity and permeability measurements for sandstones and limestones, Reinga and Aotea basins. Analyses were performed at CoreLabs, New Plymouth (using He) and GNS Science, Lower Hutt (using air).
Even greater variability is apparent in the permeability measurements taken at GNS Science and CoreLabs. This variability may be partly due to different lab techniques and different rock specimens being used in the two labs, and partly to orientation of the core plugs with respect to rock defects, principally bedding. In some cases the core plug may be a measure of the horizontal (kh) or the vertical (kv) permeability; as these are dredge samples, it is not always possible to determine whether kh or kv is being measured. All the permeability values are low (<10.5 mD), the exception being sample P83364 from the Reinga Basin (also the sample mentioned above with low porosity). The high permeability in this sample most likely relates to the porous nature of the limestone sample, that is, it is a vuggy limestone.
Discussion
Lithological constraints for seismic interpretation
Previous workers have described the seismic stratigraphy of Reinga Basin (e.g. Herzer et al. Citation1997; Bache et al. Citation2012a, Citation2012b). Our TAN1312 dredge results provide the first opportunity to interpret this seismic stratigraphy in the context of a suite of seabed samples with known lithology and age. Our new samples and analyses corroborate lithostratigraphic and chronostratigraphic correlations made by earlier workers to their seismic-based stratigraphy (, 3).
Seismic unit U1 (Bache et al. Citation2012a, Citation2012b; ) contains high-amplitude continuous to discontinuous seismic reflections that fan outwards against normal faults and onlap basement. The unit is found in the deepest parts of the basin. It is confirmed by our new samples to contain rock units that include petrographically immature Late Cretaceous sandstones. Based on sample descriptions of Herzer et al. (Citation1999), the unit also includes coal measures or organic facies although such rocks were not recovered in our survey. Cretaceous rocks are the oldest Zealandia Megasequence and lie stratigraphically above the Austral Superprovince (Mortimer et al. Citation2014) which includes Mesozoic plutonic (Median Batholith) and metamorphic basement (Murihiku Supergroup) in the region (Bache Citation2012a; Stagpoole et al. Citation2014). Our new paleontological ages indicate that Cretaceous rocks crop out on the southern flank of the West Norfolk Ridge and Wanganella Ridge. Both these areas were part of the Zealandia sector involved in Cretaceous break-up of Gondwana. We predict from seismic interpretation that Cretaceous sedimentary rocks also exist on the northeastern flank of the Lord Howe Rise.
Seismic units U2–5 () contain parallel reflectors of moderate or high amplitude, or discontinuous reflections of low amplitude. The correlative lithostratigraphic unit is confirmed by our samples to be composed of fine-grained carbonates or siliciclastic sedimentary rocks (the Haerenga Supergroup of Mortimer et al. Citation2014). The prominent seismic reflector at the top of this unit, reflector UB5 of Bache et al. (Citation2012a, Citation2012b), is a late Eocene–early Oligocene unconformity.
Seismic units U6–8 () contain parallel reflectors with variable amplitude and continuity that onlap the UB5 surface of Bache et al. (Citation2012a, Citation2012b). Our samples of Neogene sandstone, mudstone and carbonate are correlative lithostratigraphic units (the Māui Supergroup of Mortimer et al. Citation2014).
Stratigraphic correlations with onshore Zealandia successions
Many authors have commented on the affinity of dredged or drilled samples from northwest New Zealand with mainland New Zealand geology. For example, comparisons have been made between dredged alkaline basalt lava collected from a seamount on the Lord Howe Rise and Neogene intraplate basalts across Zealandia (Mortimer Citation2004). The general stratigraphic themes recognised in Zealandia’s sedimentary basins as expanded by King et al. (Citation1999) and Mortimer et al. (Citation2014) can be applied to the Reinga and Aotea basins. Affinities with New Zealand geology include the lack of carbonates during Cretaceous time, in large part because siliciclastic sediments derived from erosion of continental areas overwhelmed any contribution from carbonate sediment sources (King et al. Citation1999). Similarly, Paleogene sandstones are lacking from our sample set which, if representative of the basin as a whole, reflects the largely passive drift phase of tectonic development through Paleocene and early–middle Eocene time when the basin was experiencing subsidence and a general waning of sediment supply. DSDP Sites 206, 207, 592 and 593 all show similar lithologies to the Paleogene samples reported here (). At Sites 206 and 592, Middle Paleocene claystones and Eocene nannofossil and calcareous ooze is recorded (Shipboard Scientific Party Citation1973a, Citation1986a), and Site 207 on the Lord Howe Rise penetrated Late Cretaceous non-calcareous mudstone, a condensed calcareous mudstone of Paleocene age, and early–late middle Eocene foraminiferal nannofossil ooze (Shipboard Scientific Party Citation1973b). Site 593 on the Challenger Plateau drilled into late Eocene nannofossil chalk (Shipboard Scientific Party Citation1986b). There is a clear case for folding and inversion in late Eocene and Oligocene time as described by Bache et al. (Citation2012a, Citation2012b) to produce the anticlinal structures such as shown in . In the case of the Reinga and Aotea basins Neogene sedimentation appears to be dominated by carbonates and mudstone, reflecting a general lack of sediment supply from the continent.
The tectonostratigraphic phases established for Reinga Basin (Bache et al. Citation2012a, Citation2012b), reproduced in , are broadly consistent with the timing of sedimentary basin fill in other parts of Zealandia. We can now add that the lithologies present in the Reinga and Aotea basins are also broadly comparable with those in many other basins around New Zealand (Bache et al. Citation2014c). For example, late Cretaceous sandstones and mudstones of Reinga and Aotea basins seem to be broadly similar to age-equivalent rift-related rocks in Marlborough and Canterbury–Great South basins (unit Zealandia-2 of Bache et al. Citation2014c or Momotu Supergroup of Mortimer et al. Citation2014), and the Late Cretaceous sandstones (U2 in ) collected in our survey are broadly comparable with Pakawau Group sediments of the adjacent Taranaki Basin (Zealandia-2 of Bache et al. Citation2014c or lower Haerenga Supergroup of Mortimer et al. Citation2014). This comparison extends to the feldspathic and lithofeldspathic composition of the sandstones in the different basins (, Higgs et al. Citation2010). The Late Cretaceous mudstones collected in our survey are comparable lithologically with the early passive margin (U3 in ) Whangai Formation of eastern New Zealand. The micritic limestones (WST and FPB) collected in our survey are equivalent petrographically and temporally to the Amuri Limestone and possibly Mead Hill Formation facies of the Marlborough and Canterbury regions (Field & Browne Citation1989; Reay Citation1993; Lawrence Citation1994; Rattenbury et al. Citation2006). Younger equivalents of late Eocene–Whaingaroan age (U4–5) appear similar to the Whaingaroa Siltstone or Mahurangi Limestone of south Waikato and Northland, respectively, or to the Weber Formation of East Coast North Island (Kear Citation1966; Nelson & Hume Citation1987; Isaac et al. Citation1994; Edbrooke Citation2005; Edbrooke & Brook Citation2009; Morgans Citation2016). These formations are part of the upper Haerenga and the Waka supergroups of Mortimer et al. (Citation2014). Broad similarities also exist with the Late Cretaceous (argillaceous siltstone) and Paleocene–Eocene siliceous and calcareous micrite stratigraphies of New Caledonia (Cluzel et al. Citation2012).
The abundance of planktic foraminifera making up the bulk of the bioclastic material in this U3–5 grouping suggests a deep-marine depositional setting. The presence of minor detrital material and larger skeletal fragments in FPB samples (e.g. bryozoa) from Reinga Basin suggest that deposition was not completely pelagic and that some detrital material may have been reworked from adjacent highs or from older stratigraphic units. The majority of the carbonate samples analysed are of early–middle Eocene age, equivalent in terms of age and lithology to parts of the Amuri Limestone of Marlborough and the SE North Island (see Browne Citation1987; Lawrence Citation1994; Rattenbury et al. Citation2006; Uruski & Bland Citation2011; Hines et al. Citation2013).
The Waipawa Formation and equivalents are thin (typically less than 50 m thick; Schiøler et al. Citation2010; Hollis et al. Citation2014) so the likelihood of encountering that formation during dredging is low. Based on the regional extent of this formation in other parts of New Zealand, however, as well as at ODP Site 1172 offshore eastern Tasmania (Hollis et al. Citation2014), the Waipawa Formation is likely to occur in the Reinga and Aotea basins, although we have not been able to confirm this. An unconformity at this time (Paleocene) is present in DSDP Site 206 in the New Caledonia Basin and at DSDP Sites 207 and 208 on Lord Howe Rise (Burns et al. Citation1973; Edwards Citation1973) and this could be an additional reason why the Waipawa Formation was not recovered in our dredge samples.
Lithologically, the Neogene rocks appear similar to some mixed volcanic-lithic and sedimentary-lithic clastic units such as the Waitemata Group of Northland-Auckland (samples P83377, P83378; Carter Citation1971) or, in the case of sample P83380, with Neogene mudstones of Wanganui-Taranaki (the Wai-iti and Mahoenui groups) and Raukumara basin sediments (Tolaga Group). Some of the limestones, albeit younger, have compositional affinities to the Te Kuiti Group, all the above being part of the Māui Supergroup of Mortimer et al. (Citation2014).
Implications for petroleum reservoir development
In terms of reservoir rocks, clear potential exists in the Cretaceous for non-marine and paralic sandstone facies in the areas around the northern flanks of Lord Howe Rise and Wanganella and West Norfolk ridges, thought to be emergent land masses during Cretaceous time and comprising igneous and metasedimentary basement, capable of producing quartzofeldspathic sands (Stagpoole et al. Citation2009, Citation2014; Uruski et al. Citation2010). Although limited, our Cretaceous sandstone samples are plutoniclastic feldspathic, lithofeldspathic and feldspatholitic sandstones in which the lithics are dominated by volcanic grains (). The relatively low percentage of quartz, the proportion of lithics, the dissolution of feldspar and the alteration of biotite suggests that their reservoir quality may not be particularly good and their composition variable, but more samples are required to substantiate this. Cretaceous sandstones are thought to be the most likely reservoir rock we sampled, although the potential exists that some of the Neogene carbonates may also be reservoir facies (Stagpoole et al. Citation2009, Citation2014; Bache et al. Citation2013a).
The porosity and permeability values for the five Cretaceous sandstones have been plotted in against published data from Cretaceous sandstones in the adjacent Taranaki Basin (data derived from Higgs et al. Citation2012 and the CoreLabs measurements). Our porosity measurements used fluid saturation, and for permeability we used air (in the GNS Science analyses) or helium (in the case of the CoreLab results). displays data only from the five CoreLabs measurements shown in (using helium), to be consistent with data from Higgs et al. (Citation2012). For Taranaki Cretaceous sandstones, typical porosities are below 18% with permeabilities below c. 100 mD; more deeply buried sandstones have lower porosities and permeabilities. Although the Higgs et al. (Citation2012) plot is based on a range of analytical methods and is a plot of horizontal permeability (kh), and the samples in both their study and our study spans a wide period of the Cretaceous, the broad trends of their data can be compared with the data describing the Reinga and Aotea basins. In general, the porosity-permeability values are broadly similar between the Cretaceous samples from Taranaki Basin, and those for Reinga and Aotea basins. Although data are sparse, indicates that the Reinga and Aotea basin samples have a higher porosity than their typical Taranaki counterparts for a given permeability. For each sample, these relationships need to be examined on a case-by-case basis, but the following observations cover the range of explanations. Sample P83234 is a moderately sorted, fine-grained sandstone, with abundant fine-grained mud matrix (C; for compositional data see ). It also displays a preferred fabric shown by the preferred alignment of mica grains. This sample had a measured porosity of 27.5% reflecting intergranular pore space, but a permeability of only 1.1 mD; the fine-grained nature and the preferred alignment likely reduced the permeability, especially in the vertical sense. Sample P83246 is a much coarser-grained quartzofeldspathic sandstone with a lot of both primary (intergranular) and secondary porosity (through feldspar dissolution and biotite alteration and deformation) based on measured values of 23.8% and 27% porosity in the two sets of lab analyses, but measured permeability of only 3.4 mD possibly because the pore spaces are not interconnected (D). Overall, the Reinga and Aotea basin samples have not been greatly buried, given that their porosity values are high and perhaps represent values akin to their original depositional porosity. However, they have experienced diagenetic modifications that have reduced the connectedness between grains or between secondary pore spaces, reflected in their lower-than-expected permeability based on comparison with their Taranaki Basin counterparts.
Figure 10. Porosity–permeability values for four Cretaceous sandstones (five analyses) in Reinga and Aotea basins, plotted against typical Cretaceous sandstones in Taranaki Basin (from Higgs et al. Citation2012 and data presented in Table 5). Note that the data were derived by different methods; Taranaki data represent horizontal permeability based on well samples (typically core plugs), whereas our data (also core plugs of the dredged sample) have no particular orientation. Only data from the five analyses undertaken at CoreLabs are shown here. The Reinga and Aotea basin samples have a higher porosity for a given permeability than their Taranaki Basin counterparts.
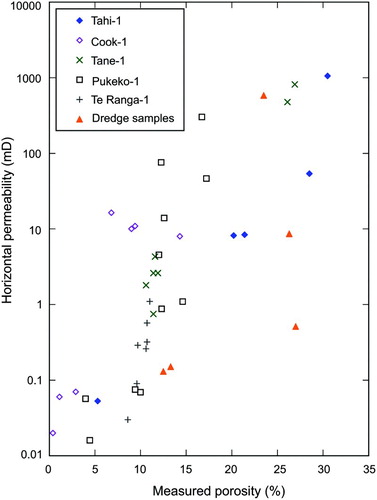
Neogene limestones (samples P83183 and P83364) have porosities of around 25% (excluding the GNS Science measurement for sample P83364 mentioned above) and permeabilities between c. 10 and 580 mD (). Petrographical examination showed that these samples have carbonate cement or biospar, and this likely reduces the permeability in these samples although porosity could be retained within bioclasts such as bryozoans, echinoids, bivalves and foraminifera (as in C–E, 9A, C).
Summary
Rock dredging carried out on the TAN1312 voyage, together with previous dredge samples, is providing a more complete picture of the sedimentary rock succession in the Aotea and Reinga basins. Rocks of mid-Cretaceous–Neogene age have been recovered and their ages, depositional settings, textures and compositions are reported here. Cretaceous rocks are not as well represented as Cenozoic rocks, in large part due to their depth of burial and the small number of anticlines or tilted blocks that expose these rocks at the seabed. Our survey has confirmed their existence in areas flanking the West Norfolk and Wanganella ridges and, together with information from seismic interpretations, they provide evidence that the Cretaceous rocks are part of the rift succession formed during Gondwana break-up (Bache et al. Citation2012a, Citation2012b). They are a potential petroleum reservoir facies which were interpreted by Stagpoole et al. (Citation2014) as fluvial and shallow-marine (including deltaic) depositional settings. Although we cannot confirm these environments of deposition, we have found them to be dominated by feldspathic and lithofeldspathic sandstones. The relatively low proportion of quartz and the abundance of lithics may compromise reservoir quality but their plutoniclastic composition is a favourable attribute in terms of their reservoir quality. More samples are needed to fully assess reservoir quality in the region. It may be that the Cretaceous rocks in eastern Reinga Basin are lithic-rich sandstones derived from the Eastern Province greywackes, whereas more plutonic-derived sandstones may exist further west, more like the sandstones of the Western Platform of Taranaki Basin (Higgs et al. Citation2012). We know from studies in the Taranaki Basin that the composition of Cretaceous sandstones is strongly affected by provenance and reflects the local source rocks, so these compositions (which are largely only from the West Norfolk and Wanganella ridges) may not be representative of Cretaceous rocks elsewhere in the Reinga and Aotea basins. We suspect that reservoir quality varies considerably for these Cretaceous sandstones and, based on our knowledge from elsewhere, we think it likely they will be controlled by local provenance, depositional facies and diagenetic modifications.
Porosity and permeability analyses, although limited in number, suggest these rocks have high porosity (12–27.5%) but relatively low permeabilities (0.15–3.4 mD). Although the sandstones have not been greatly buried (as indicated by their relatively high porosity), they have undergone diagenetic changes in the form of alteration of feldspar and biotite grains which has reduced their permeability. Much more work (and additional sample collection) needs to be undertaken to determine reservoir quality in the region.
Cenozoic sedimentary rocks are widespread and are dominated by mudstones and micritic limestones in the Paleogene, and by mudstones, carbonates and sandstones in the Neogene. We see many parallels to the stratigraphy of mainland New Zealand for these rocks as well as with samples drilled in DSDP Sites 206, 207, 592 and 593. Samples principally resemble the Whangai, Amuri, Mead Hill, Mahurangi, Wanstead and Weber formations of latest Cretaceous–Oligocene age, and Waitemata and Wai-iti groups of Miocene age, all of which seem to be widespread and thick throughout the Reinga and Aotea basins.
Supplementary data
Table S1. List of samples collected in the TAN1312 survey and analyses performed on these samples in this study (samples used for the various types of analyses are indicated). Latitude and longitude are ship positions when the dredge first touched the seabed. Rock type abbreviations: Clyt, claystone; Cgl, conglomerate; Lst, limestone; Mst, mudstone; Zst, siltstone; Sst, sandstone. Igneous samples are not described here but will be the subject of a subsequent publication. In addition, Mn-crusts and cherts are not discussed in this paper.
Table S2. Point counts.
Table S2. Point counts.
Download MS Excel (38.5 KB)Table S1. List of samples collected in the TAN1312 survey and analyses performed on these samples in this study (samples used for the various types of analyses are indicated).
Download MS Word (171.5 KB)Acknowledgements
We acknowledge the valuable assistance of the captain and crew of RV Tangaroa, and also Arne Pallentin, Susi Woelz and Steve Wilcox (NIWA) who were part of the science crew on the TAN1312 cruise. In addition, the shipboard assistance of Ben Hines, Sarah Jury and Heidi Roop (Victoria University of Wellington), Pierrick Rouillard (ADECAL, Agence de Développement Economique de la Nouvelle-Calédonie) and Malcolm Flowers (New Zealand Petroleum and Minerals (NZP&M), Wellington) is greatly appreciated. Vaughan Stagpoole, Richard Herzer, Stuart Henrys, Todd Ventura, David Try, Randall McDonnell, Roger Tremain, Sonja Bermudez, Debra Chappell, Peter Barker and Barbara Lyndsell (all GNS Science), Helen Neil and Els Maas (NIWA), Mike Aitkenhead (MPI) and Brad Ilg, Nancy Davies and Chris Duncan (all NZP&M) helped with various post-cruise discussions, data, maps, analyses or sample processing. Assistance with graphics was provided by Philip Carthew (GNS Science) and Imogen Browne (University of Otago). Ben Durrant (GNS Science) provided excellent quality thin-sections, and CoreLabs, New Plymouth provided petrophysical measurements. The manuscript benefitted from thoughtful reviews by Malcolm Arnot, Kyle Bland and Brad Field (GNS Science) and Kathie Marsaglia (California State University, Northridge).
Associate Editor: Dr Kari Bassett.
Disclosure statement
No potential conflict of interest was reported by the authors.
ORCiD
N Mortimer http://orcid.org/0000-0002-6812-3379
Additional information
Funding
References
- Bache F, Mortimer N, Sutherland R, Collot J, Rouillard P, Stagpoole V, Nicol A. 2014a. Seismic stratigraphic record of transition from Mesozoic subduction to continental breakup in the Zealandia sector of eastern Gondwana. Gondwana Res. 26:1060–1078. doi: 10.1016/j.gr.2013.08.012
- Bache F, Stagpoole V, Sutherland R. 2012a. Seismic stratigraphy of the Reinga Basin, NW New Zealand: tectonic and petroleum implications. In: Rosen NC, Weimer P, Coutes dos Anjos SM, Henrickson S, Marques E, Mayall M, Fillon R, D'Agostino T, Saller A, Campion K, et al., editors. Proceedings: New Understanding of the Petroleum Systems of Continental Margins of the World. GCSSEPM Foundation 32nd Annual Bob F. Perkins Research Conference; 2012 Dec 2–5; Houston, Texas. Houston, TX: GCSSEPM Section; p. 221–252.
- Bache F, Stagpoole V, Sutherland R. 2013a. The Reinga Basin, NW New Zealand: seismic stratigraphy, tectonic evolution and implications for hydrocarbon exploration. Paper presented at: Advantage NZ: New Zealand Petroleum Conference; 2013 Apr 28–May 1; Auckland, New Zealand.
- Bache F, Stagpoole V, Sutherland R, Barker D, Black J, Sahoo T, Bull S. 2013b. Tasman Frontier petroleum prospectivity study. GNS Science Consultancy Report 2013/305. Lower Hutt: GNS Science.
- Bache F, Sutherland R, King PR. 2014b. Use of ancient wave-ravinement surfaces to determine palaeogeography and vertical crustal movements around New Zealand. New Zeal J Geol Geophys. 57:459–467. doi: 10.1080/00288306.2014.975254
- Bache F, Sutherland R, Mortimer N, Browne G, Lawrence MJF, Black J, Flowers M, Rouillard P, Pallentin A, Woelz S, et al. 2014c. Tangaroa TAN1312 voyage report: dredging Reinga and Aotea basins to constrain seismic stratigraphy and petroleum systems (DRASP), NW New Zealand. GNS Science Report 2014/05. New Zealand Petroleum Report PR4916. Lower Hutt: GNS Science.
- Bache F, Sutherland R, Stagpoole V, Herzer R, Collot J, Rouillard P. 2012b. Stratigraphy of the southern Norfolk Ridge and the Reinga Basin: a record of initiation of Tonga-Kermadec-Northland subduction in the southwest Pacific. Earth Planet Sci Lett. 321–322:41–53. doi: 10.1016/j.epsl.2011.12.041
- Beu AG, Darragh TA. 2001. Revision of southern Australian Cenozoic fossil Pectinidae (Mollusca: Bivalvia). Proc Roy Soc Vic. 113:1–205.
- Bland KJ, Uruski CI. 2012. Pegasus Basin: a little-deformed window into an old friend, the East Coast Basin. In: Proceedings of the 34th International Geological Congress 2012; 2012 Aug 5-10; Brisbane, Australia. Brisbane, QLD: Australian Geosciences Council; p. 3945.
- Browne GH. 1987. In situ and intrusive sandstone in Amuri facies limestone at Te Kaukau Point, southeast Wairarapa, New Zealand. New Zeal J Geol Geophys. 30:363–374. doi: 10.1080/00288306.1987.10427541
- Browne GH, Kennedy EM, Constable RM, Raine JI, Crouch EM, Sykes R. 2008. An outcrop based study of the economically significant Late Cretaceous Rakopi Formation, northwest Nelson, Taranaki Basin, New Zealand. New Zeal J Geol Geophys. 51:295–315. doi: 10.1080/00288300809509867
- Browne GH, Lawrence MJF, Mortimer N, Ventura GT, Clowes C, Morgans HEG, Seward D, Barker PB, Lyndsell BM, Beu AG, Hollis CJ. 2014. Analysis of rock samples collected during the TAN1312 dredging survey (DRASP) of Reinga and Aotea basins, NW New Zealand. GNS Science Consultancy Report 2014/267, 124 p. Reproduced as New Zealand Petroleum Report PR5042. Lower Hutt: GNS Science.
- Burns RE, Andrews JE, van der Lingen GJ. 1973. Initial reports of the Deep Sea Drilling Project 21. Washington, DC: US Government Printing Office.
- CANZ (Charting Around New Zealand). 2008. New Zealand region bathymetry, 1:4 000 000. 2nd ed. NIWA Chart. Miscellaneous Series No. 85. Auckland: NIWA.
- Carter L. 1971. Stratigraphy and sedimentology of the Waitemata Group, Puketotara Peninsula, Northland. New Zeal J Geol Geophys. 14: 169–191. doi: 10.1080/00288306.1971.10422469
- Cluzel D, Maurizot P, Collot J, Sevin B. 2012. An outline of the geology of New Caledonia; from Permian-Meozoic southeast Gondwanaland active margin to Cenozoic obduction and supergene evolution. Episodes. 35:1–15.
- Cooper RA. 2004. The New Zealand geological timescale. Institute of Geological and Nuclear Sciences Monograph 22. Lower Hutt: Institute of Geological and Nuclear Sciences.
- Crouch EM, Willumsen PS, Kulhanek D, Gibbs S. 2014. A revised Palaeocene (Teurian) dinoflagellate cyst zonation from eastern New Zealand. Rev Palaeobot Palynol. 202:47–79. doi: 10.1016/j.revpalbo.2013.12.004
- Dunham RJ. 1962. Classification of carbonate rocks according to depositional texture. In: Ham WE, editor. Classification of carbonate rocks. Memoir of the American Association of Petroleum Geologists 1. Tulsa, OK: American Association of Petroleum Geologists; p. 108–121.
- Edbrooke SW. 2005. Geology of the Waikato area. Scale 1:250,000. Institute of Geological and Nuclear Sciences Geological Map 13. Lower Hutt: Institute of Geological and Nuclear Sciences.
- Edbrooke SW, Brook FJ. 2009. Geology of the Whangarei area: scale 1:250 00. GNS Science Geological Map 2. Lower Hutt: GNS Science.
- Edwards, AR. 1973. Calcareous nannofossils from the southwest Pacific, Deep Sea Drilling Project, Leg 21. Initial Reports of the Deep Sea Drilling Project. 21:641–691.
- Field BD, Browne GH. 1989. Cretaceous and Cenozoic sedimentary basins and geological evolution of the Canterbury region. South Island, New Zealand: New Zealand Geological Survey Basin Studies 2.
- Folk RL. 1962. Spectral subdivisions of limestone types. In: Ham WE, editor. Classification of carbonate rocks. Memoir of the American Association of Petroleum Geologists 1. Tulsa, OK: American Association of Petroleum Geologists; p. 62–84.
- Glasby GP, Wright IC. 1990. Marine mineral potential in New Zealand’s exclusive economic zone. Mar Min. 9:403–427.
- Herzer RH, Chaproniere GCH, Edwards AR, Hollis CJ, Pelletier B, Raine JI, Scott GH, Stagpoole V, Strong CP, Symonds P, Wilson GJ, Zhu H. 1997. Seismic stratigraphy and structural history of the Reinga Basin and its margins, southern Norfolk Ridge system. New Zeal J Geol Geophys. 40:425–451. doi: 10.1080/00288306.1997.9514774
- Herzer RH, Davy BW, Mortimer N, Quilty P, Chaproniere GCH, Jones CM, Crawford AJ, Hollis CJ. 2009. Seismic stratigraphy and structure of the Northland Plateau and the development of the Vening Meinesz transform margin, SW Pacific Ocean. Mar Geophys Res. 30:21–60. doi: 10.1007/s11001-009-9065-1
- Herzer RH, Sykes R, Killops SD, Funnell RH, Burggraf DR, Townend J, Raine JI, Wilson GJ. 1999. Cretaceous carbonaceous rocks from the Norfolk Ridge system, Southwest Pacific; implications for regional petroleum potential. New Zeal J Geol Geophys. 42:57–73. doi: 10.1080/00288306.1999.9514831
- Higgs KE, Arnot MJ, Browne GH, Kennedy EM. 2010. Reservoir potential of Late Cretaceous terrestrial to shallow marine sandstones, Taranaki Basin, New Zealand. Mar Petrol Geol. 27:1849–1871. doi: 10.1016/j.marpetgeo.2010.08.002
- Higgs KE, Strogen D, Griffin A, Ilg B, Arnot M. 2012. Reservoirs of the Taranaki Basin, New Zealand. GNS Science Data Series No. 2012/13a. Lower Hutt: GNS Science.
- Hines BR, Kulhanek DK, Hollis CJ, Atkins CB, Morgans HEG. 2013. Palaeocene-Eocene stratigraphy and paleoenvironment at Tora, southeast Wairarapa, New Zealand. New Zeal J Geol Geophys. 56:243–262. doi: 10.1080/00288306.2013.836112
- Hollis CJ, Tayler MJS, Andrew B, Taylor KW, Lurcock P, Bijl PK, Kulhanek DK, Crouch EM, Nelson CS, Pancost RD, et al. 2014. Organic-rich sedimentation in the South Pacific Ocean associated with Late Palaeocene climatic cooling. Earth-Sci Rev. 134:81–97. doi: 10.1016/j.earscirev.2014.03.006
- Ingersoll RV, Bullard TF, Ford RL, Grimm JP, Pickle JD, Sares SW. 1984. The effect of grain size on detrital modes: a test of the Gazzi-Dickinson point-counting method. J Sediment Petrol. 54:103–116.
- Isaac MJ, Herzer RH, Brook FJ, Hayward BW. 1994. Cretaceous and Cenozoic sedimentary basins of Northland, New Zealand. Institute of Geological and Nuclear Sciences Monograph 8. Lower Hutt: Institute of Geological and Nuclear Sciences.
- Kear D. 1966. Sheet N55-Te Akau. Geological map of New Zealand 1:63,360. Wellington: Department of Scientific and Industrial Research.
- King PR, Naish TR, Browne GH, Field BD, Edbrooke SW. 1999. Cretaceous to recent sedimentary patterns in New Zealand. GNS Science Folio Series 1. Lower Hutt: GNS Science.
- Lawrence MJF. 1994. Conceptual model for early diagenetic chert and dolomite, Amuri Limestone Group, north-eastern South Island, New Zealand. Sedimentology. 41:479–498. doi: 10.1111/j.1365-3091.1994.tb02007.x
- Lawrence MJF, Browne GH, Bache F, Sutherland R, Morgans HEG, Clowes C, Mortimer N. 2014. Petroleum prospectivity of Reinga Basin, NW New Zealand: initial laboratory analyses of samples from the TAN1312 Survey. GNS Science Consultancy Report 2014/58. Reproduced as New Zealand Petroleum Report PR4916. Lower Hutt: GNS Science.
- Morgans HEG. 2016. The Weber Formation at its type locations in Southern Hawke’s Bay, and its distribution through the east Coast, North Island, New Zealand. GNS Science Report 2015/38. Lower Hutt: GNS Science.
- Mortimer N. 2004. Basement gabbro from the Lord Howe Rise. New ZealJ Geol Geop. 47:501–507. doi: 10.1080/00288306.2004.9515072
- Mortimer N, Rattenbury MS, King PR, Bland KJ, Barrell DJA, Bache F, Beggs JG, Campbell HJ, Cox SC, Crampton JS, et al. 2014. High-level stratigraphic scheme for New Zealand rocks. New Zeal J Geol Geoph. 57:402–419. doi: 10.1080/00288306.2014.946062
- Mortimer N, Tulloch AJ, Ireland TR. 1997. Basement geology of Taranaki and Wanganui Basins, New Zealand. New Zeal J Geol Geophys. 40:223–236. doi: 10.1080/00288306.1997.9514754
- Nelson CS, Hume TM. 1987. Paleoenvironmental controls on mineral assemblages in a shelf sequence: Te Kuiti, South Auckland, New Zealand. New Zeal J Geol Geophys. 30:343–362. doi: 10.1080/00288306.1987.10427540
- Raine JI, Beu AG, Boyes AF, Campbell HJ, Cooper RA, Crampton JS, Crundwell MP, Hollis CJ, Morgans HEG. 2015. Revised calibration of the New Zealand geological timescale: NZGT2015/1. GNS Science Report 2012/39. Lower Hutt: GNS Science.
- Rattenbury MS, Townsend DB, Johnston MR. 2006. Geology of the Kaikoura area. Scale 1:250,000. Institute of Geological and Nuclear Sciences Geological Map 13. Lower Hutt: Institute of Geological and Nuclear Sciences.
- Reay MB. 1993. Geology of the middle Clarence Valley. Scale 1:50,000. Institute of Geological and Nuclear Sciences Geological Map 10. Lower Hutt: Institute of Geological and Nuclear Sciences.
- Schiøler P, Rogers KM, Sykes R, Hollis CJ, Ilg BR, Meadows D, Roncaglia L, Uruski CI. 2010. Palynofacies, organic geochemistry and depositional environment of the Tartan Formation (Late Palaeocene), a potential source rock in the Great South Basin, New Zealand. Mar Petrol Geol 27:351–369. doi: 10.1016/j.marpetgeo.2009.09.006
- Shipboard Scientific Party. 1973a. Site 206. In: Initial reports of the Deep Sea Drilling Project, Volume XXI. Part I. Site reports. Washington, DC: Government Printer; p. 103–195. doi:10.2973/dsdp.proc.21.106.1973.
- Shipboard Scientific Party. 1973b. Site 207. In: Initial reports of the Deep Sea Drilling Project, Volume XXI. Part I. Site reports. Washington, DC: Government Printer; p. 197–269. doi:10.2973/dsdp.proc.21.107.1973.
- Shipboard Scientific Party. 1973c. Site 208. In: Initial reports of the Deep Sea Drilling Project, Volume XXI. Part I. Site reports. Washington, DC: Government Printer; p. 271–331. doi:10.2973/dsdp.proc.21.108.1973.
- Shipboard Scientific Party. 1986a. Site 592: Lord Howe Rise, 36° S. In: Initial reports of the Deep Sea Drilling Project, Volume XC. Part II. Site reports. Washington, DC: Government Printer; p. 487–550. doi:10.2973/dsdp.proc.90.108.1986.
- Shipboard Scientific Party. 1986b. Site 593: Challenger Plateau. In: Initial reports of the Deep Sea Drilling Project, Volume XC. Part II. Site reports. Washington, DC: Government Printer; p. 551–651. doi:10.2973/dsdp.proc.90.109.1986.
- Stagpoole VM, Neil H, Black J, Bland KJ, Bowden D, Browne GH, Clark M, Crutchley G, Dunkin M, Gorman R, et al. 2014. Reinga Basin petroleum prospectivity screening report. GNS Science Consultancy Report 2014/269. Reproduced as New Zealand Petroleum Report PR5042. Lower Hutt: GNS Science.
- Stagpoole VM, Reid E, Browne GH, Bland KJ, Ilg BR, Griffin AG, Herzer RH, Uruski, CI. 2009. Petroleum prospectivity of the Reinga Basin, New Zealand. GNS Science Consultancy Report 2009/251. Reproduced as New Zealand Petroleum Report PR4037. Lower Hutt: GNS Science.
- Sutherland R, Viskovic P, Bache F, Stagpoole V, Collot J, Rouillard P, Hashimoto T, Hackney R, Higgins K, Rollet N, Patriat M, Roest WR. 2012. Compilation of seismic reflection data from the Tasman Frontier region, southwest Pacific. GNS Science Report 2012/01. Lower Hutt: GNS Science.
- Uruski CI. 2011. Exploring New Zealand’s marine territory. APPEA Journal. 51:549–565.
- Uruski CI, Bland KJ. 2011. Pegasus Basin and the prospects for oil and gas. New Zealand Petroleum Report PR4326. GNS Science Consultancy. Report 2010/291. Lower Hutt: GNS Science.
- Uruski CI, Kennedy C, Stagpoole VM, Sutherland R, Henrys SA. 2008. The discovery of a new sedimentary basin: offshore Raukumara, East Coast, North Island, New Zealand. APPEA Journal. 48:53–68.
- Uruski CI, Reid E, Stagpoole VM, Herzer RH, Griffin AG, Bland KJ, Ilg BR, Browne GH. 2010. The Reinga Basin, North Island, New Zealand. APPEA Journal 50:287–308.
- Ventura GT. 2014. Organic Geochemical Study of Dredge Samples Collected During the TAN1312 Dredging Survey (DRASP) of the Reinga and Aotea Basins, Northwest New Zealand. GNS Science Consultancy Report 2014/265. Reproduced as New Zealand Petroleum Report PR5042. Lower Hutt: GNS Science.
- Willumsen PS. 2011. Maastrichtian to Palaeocene dinocysts from the Clarence Valley, South Island, New Zealand. Alcheringa 35:199–240. doi: 10.1080/03115518.2010.494484