ABSTRACT
Carboniferous granitoids in central Stewart Island host two metalliferous hydrothermal systems that formed during emplacement of the c. 306 ± 2 Ma old Euchre Pluton and 303 ± 2 Ma Hill 267 leucogranitoid, respectively. The Ogles Creek–Silvertown Hydrothermal System (OST) on the eastern side of South West Arm, Paterson Inlet is centred on the scheelite-bearing, ilmenite-syenogranite core of the Euchre Pluton. Here pyritic-potassic alteration and related veins contain Mo, Bi, Ag, Co, Cu, W, Pb, Zn, U–Th and rare Earth element (REE) sulphide, telluride, oxide and phosphate minerals. Peripheral quartz-rich veins contain various Ag, Au, Pb and Zn-bearing sulphide, telluride and oxide minerals. The Hill 267 Hydrothermal System (HHS) is centred on the ridge between the Scott Burn and Forked Creek where strongly altered leucogranitoid riddled with miarolitic cavities contains pyrite, W- and Nb-bearing rutile, molybdenite, tourmaline and 2–10 times background Bi, Ag, Te, Cu and Se. More prospective parts of both systems have probably been removed by erosion, but less deeply eroded correlative hydrothermal systems may be present elsewhere in New Zealand near the outboard margin of the Takaka Terrane.
Introduction
Intrusion-related metal deposits globally and in New Zealand
A wide variety of economically important intrusion-related hydrothermal metal deposits such as porphyry Cu (Mo, Au), intrusion-related Au, Climax-type molybdenum, granite-related greisen Sn, W, Mo and Bi, and various types of skarn deposits occur in Phanerozoic convergent margin orogenic belts worldwide (e.g. Titley & Beane Citation1981; White et al. Citation1981; Thompson et al. Citation1999, Blevin Citation2004; Černý et al. Citation2005; Meinert et al. Citation2005; Seedorff et al. Citation2005). Mineral paragenetic relationships, fluid inclusion, isotopic and geochronological data in each deposit type indicate a direct link between crystallisation of a particular intrusion or suite of intrusions and generation of a metalliferous magmatic-hydrothermal fluid.
Plutonic rocks are an important part of the New Zealand basement in the western South Island and Stewart Island (Rattenbury et al. Citation1998, Citation2006; Nathan et al. Citation2002; Turnbull & Allibone Citation2003; Turnbull et al. Citation2010a). However, despite the widespread occurrence of plutonic rocks in southern and western New Zealand mineral exploration since the mid-19th century has only identified a small number of intrusion-related metalliferous mineral occurrences and minor deposits (e.g. Williams Citation1934; Wodzicki Citation1972; Eggers & Adams Citation1979; Pirajno Citation1981, Citation1982; Pirajno & Bentley Citation1985; Braithwaite & Rabone Citation1985; Tulloch & Rabone Citation1993; Grapes & Challis Citation1999; Gollan Citation2006). The Sams Creek gold deposit in northwest Nelson has been cited as an intrusion-related gold deposit (Faure & Braithwaite Citation2006; Angus Citation2014; Phillips Citation2014), but the age of the peralkaline granite dyke which hosts the deposit and source of hydrothermal fluids are still debated (Windle & Craw Citation1991; Tulloch & Dunlap Citation2006). Orogenic Au deposits in the Reefton and Preservation Inlet districts are not thought to be products of magmatic-hydrothermal fluids (Williams Citation1974; Christie & Braithwaite Citation2003; Bierlein et al. Citation2004), although their formation may have been coeval with one or more phases of plutonism in surrounding areas.
Tectono-magmatic setting of plutonic rocks in southern New Zealand
Plutonic, volcanic and metasedimentary rocks which underlie Stewart Island formed during two prolonged episodes of orogenesis on the convergent margin of Gondwana at c. 495–300 Ma and c. 250–106 Ma (Turnbull & Allibone Citation2003; Allibone & Tulloch Citation2004; Tulloch et al. Citation2009; Turnbull et al. Citation2010b) (). Intact early–middle Paleozoic metasedimentary rocks and plutons of S-type granite with affinities to continental Gondwana extend as far north as the Gutter Shear Zone, the southernmost of three major structures that cut the basement rocks of Stewart Island (Allibone & Tulloch Citation2008). Rare xenoliths of similar metasedimentary rock and Carboniferous I and A-type granitoids occur as far north as the Freshwater Fault System (FFS), the northernmost of the three major structures which cut basement rocks on the island. Paleozoic rocks are absent north of the FFS, indicating it marks the outboard margin of continental Gondwana in the late Carboniferous.
Figure 1. A, Stewart Island is underlain by Western Province Paleozoic rocks with affinities to continental Gondwana and Mesozoic arc plutonic rocks of the Median Batholith. B, Metalliferous hydrothermal systems described in this paper are located on the northern, outboard limit of Carboniferous plutonic rocks on Stewart Island, immediately south of the FFS.
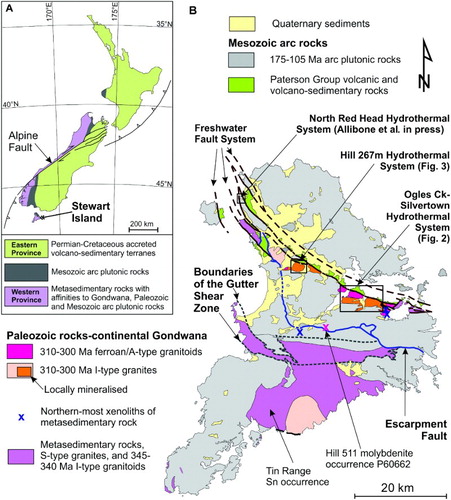
Calc-alkaline, arc-related, I-type gabbroic to granitic (Darran Suite) rocks were emplaced widely throughout the northern half of Stewart Island during c. 170–130 Ma (Kimbrough et al. Citation1994; Allibone & Tulloch Citation2004). After c. 130 Ma the focus of plutonism migrated into the southern half of the island, where further dioritic to granitic (Separation Point Suite) rocks with an alkali-calcic, high Sr/Y chemistry were emplaced during c. 125–106 Ma.
Plutons of A-type granite and quartz syenite associated with both Paleozoic and Mesozoic orogenesis are present along the southern margin of the FFS, suggesting the proto-FFS was a focus for extension and associated high heat flow during both the late Carboniferous and Cretaceous.
This study
The discovery of scattered mineralised granite outcrops during various mapping projects between 1986 and 2002 (Allibone Citation1991; Allibone & Tulloch Citation1997, Citation2004, Citation2008; Turnbull & Allibone Citation2003) provided the impetus for this investigation, which resulted in the recognition of three kilometre-scale intrusion-related hydrothermal systems in north and central Stewart Island (). The largest and paragenetically most complex system is developed in c. 7 km2 of the Euchre, Freds Camp and Silvertown plutons on the east side of South West Arm in Paterson Inlet (). It includes particularly intensely altered and mineralised rocks in Ogles Creek and the mineralised quartz vein in the historic Silvertown adit at the mouth of South West Arm (1218381 E, 4790446 N). Accordingly this hydrothermal system is referred to as the Ogles Creek–Silvertown Hydrothermal System (OSH).
Figure 2. Geological map of the Ogles Creek–Silvertown Hydrothermal System (OSH). Mineralised rocks occur in a northeast-trending zone c. 7 km2 long on the eastern side of South West Arm. The most strongly altered and mineralised rocks are located within the ilmenite-rich granitic core of the Euchre Pluton. No mineralised rocks have been found to date within nearby parts of the adjacent Jurassic and Cretaceous units.
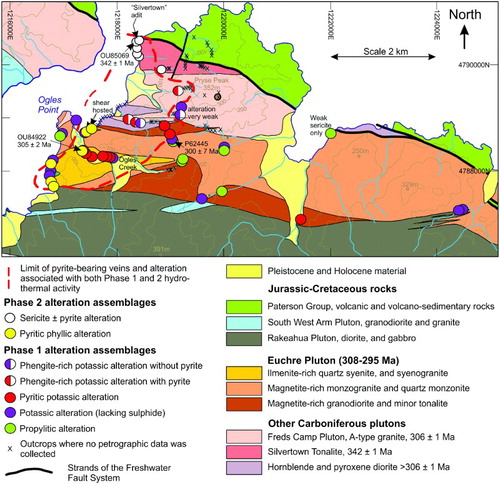
Strongly altered and partially oxidised leucogranite riddled with miarolitic cavities crops out over c. 3 km2 between the Scott Burn and Forked Creek in the vicinity of spot height 267 m, immediately south of the FFS in central Stewart Island (, ). Altered rocks are largely restricted to the ridge crest between the Scott Burn and Forked Creek, implying they are remnants of an eroded carapace within the top of the underlying Hill 267 leucogranitoid. Largely unaltered, massive, unmineralised, homogenous, equigranular, and medium- to coarse-grained leucogranite and leuco-granodiorite forms surrounding parts of Hill 267. Accordingly, this hydrothermal system is referred to as the Hill 267 Hydrothermal System (HHS).
Figure 3. Geological map of the Hill 267 Hydrothermal System (HHS). Strongly altered and mineralised granitoid, which contains miarolitic cavities, caps parts of the ridge between Forked Creek and the Scott Burn.
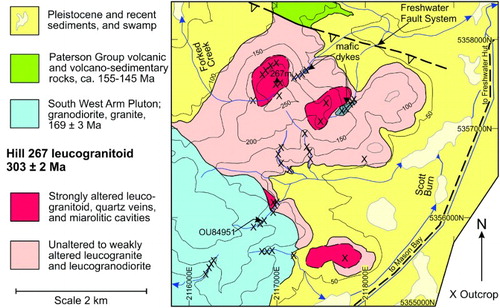
A third metalliferous hydrothermal system associated with A-type granite at North Red Head on the west coast of Stewart Island () has a different metal assemblage and age (Allibone et al. Citation2016).
This paper describes the OSH and HHS granitoid-hosted metalliferous hydrothermal systems in central Stewart Island (). Both are orders of magnitude larger than the minor Sn occurrence at the southern end of the Tin Range (Williams Citation1934). Mineralised rocks described here lack economically viable metal grades over mineable rock volumes. However, the paucity of outcrop and partially oxidised character of much of the available outcrop means the economic potential of the OSH and HHS remain unclear. Both are part of a previously unrecognised metallogenic event in the basement rocks of southern and western New Zealand that may include more significant metal deposits elsewhere, implying plutonic rocks in New Zealand may be more prospective than previously thought. U–Pb zircon emplacement ages were acquired for each mineralised granitoid to confirm the age of this metallogenic event.
Identification of potentially fertile granitoid plutons is an especially difficult problem confronting geologists exploring for intrusion-related metal deposits. Textural features commonly used to distinguish fertile and unprospective granitoid plutons (e.g. Thompson et al. Citation1999; Mustard Citation2001) are absent from large parts of both mineralised plutons described here, suggesting prospective plutonic rocks are more difficult to recognise than generally appreciated. This is one key reason why both the OSH and HHS remained unrecognised until the early 21st century. This paper therefore includes descriptions of both mineralised and unmineralised parts of the plutons that drove the OSH and HHS to highlight the unremarkable character of each pluton where unaffected by obvious hydrothermal activity.
Methods
Field work was undertaken during 2009 and 2013. Unmineralised and mineralised samples were analysed from both systems to provide data on the background metal contents and chemistry of the mineralised rocks (Table S1).
A variety of analytical methods were utilised to ensure the complete metal assemblages were fully defined. The mineralised character of both systems was confirmed in 2004 when samples collected between 1996 and 2002 were analysed for Au, Ag, As, Bi, Cu, Mo, Sb, Te and W by the fire assay F650 (Au) and M104 (4 acid digest and ICP-MS finish) at SGS laboratories in Townsville and Perth, respectively. Larger sample suites were subsequently analysed for a wide range of elements in 2009, 2013 and 2014 by the Au-AA21 fire assay (Au), ME-MS81 (lithium metaborate fusion followed by an acid digest and inductively coupled plasma-mass spectroscopy or ICP-MS finish) and ME-MS61/62 (4 acid digest followed by ICP-MS finish) methods at ALS laboratories in Brisbane. The ME-MS81 method was employed to gain definitive data on the concentration of especially refractory metals such as W and Sn and the rare Earth elements (REE), whereas the ME-MS61/62 method is adequate to quantify the amounts of many other less refractory trace elements and ore metals. Major-element and additional trace-element analyses were obtained by XRF at University of Otago in 2009 and CRL Energy Ltd in 2013. The concentrations of some trace elements have been measured by three different methods, allowing the results of each method to be compared (Table S1).
The identity of many especially fine-grained opaque minerals was confirmed with the Zeiss Sigma VP variable-pressure scanning electron microscope (fitted with a HKL INCA Premium Synergy Integrated EDS/EBSD system) running at the University of Otago.
Zircon U–Pb dating was undertaken at the Centre for Trace Element Analysis, University of Otago, by laser ablation inductively coupled plasma mass spectrometry (LA-ICPMS). Mineral separation was undertaken at GNS Science using standard crushing, heavy liquid and magnetic susceptibility techniques. Zircon grains were imaged by the SEM facility at the University of Otago to assess grain complexity and guide analysis. LA-ICPMS analytical operating procedures follow those outlined in Mortimer et al. (Citation2015). LA-ICPMS data were referenced to the zircon standard Temora 2 (Black et al. Citation2004) and NIST SRM 610 glass (Pearce et al. Citation1997). Data were reduced using Iolite v. 2.41 (Paton et al. Citation2011). Zircon grains that were more than 10% discordant were not considered for age calculations. Probability density plots and Concordia diagrams were generated using Isoplot software (Ludwig Citation2003).
Grid references quoted in this paper refer to the NZTM coordinate system (equivalent to WGS84), rather than the earlier NZMS260 coordinate system.
Primary characteristics of the mineralised plutonic rocks
Euchre Pluton
Much of the OSH is hosted by the c. 1.5 by 11 km east-trending Euchre Pluton, 90% of which comprises medium-grained granodiorite, monzogranite and minor tonalite in which magnetite comprises 90–95% of the opaque oxide (). Syenogranite, minor quartz syenite and minor alkali-feldspar granite, in which ilmenite is the dominant opaque oxide, comprise the westernmost 10% of the pluton.
Plagioclase throughout the Euchre Pluton has a characteristic euhedral to subhedral texture, regardless of the modal composition of the rock. Most K-feldspar is interstitial relative to plagioclase, commonly forming plates that partially enclose the plagioclase grains. Quartz is distinctly interstitial with respect to both plagioclase and K-feldspar, again regardless of the overall rock composition. These textures are one of the unifying features of the pluton which distinguish it from the adjacent Freds Camp and South West Arm plutons. Traces of relict coarse-grained brown biotite are probably representative of the primary magmatic ferromagnesian silicate in both magnetite and ilmenite-rich parts of the Euchre Pluton. Other accessory minerals include zircon, apatite, monazite, traces of euhedral epidote, sometimes with allanite cores, and traces of euhedral magmatic titanite, xenotime and thorite.
Aside from some variations in grain size in the syenogranite core there is little obvious internal textural variation within the Euchre Pluton (Figure S1A). Pegmatite dykes, miarolitic cavities, unidirectional solidification textures and composite pegmatite-hydrothermal veins present in many mineralised granites and intrusion-related hydrothermal systems are generally absent from the Euchre Pluton. Ilmenite granite from the pluton core locally contains quartz ± pyrite-filled blebs whose shape and association with potassic alteration imply they formed at relatively high temperatures during the transition from magmatic to hydrothermal conditions, perhaps in micro-scale miarolitic cavities (Figure S1B). Aplite dykes near the northern margin of the Euchre Pluton are the only obvious evidence that the Euchre Pluton may have produced a late highly fractionated melt similar to those described in many other intrusion-related hydrothermal systems (e.g. Mustard Citation2001).
Magmatic ilmenite grains in OU84923, OU84926 and OU84930 contain inclusions of scheelite up to 5 µm across, implying scheelite is a widespread accessory magmatic mineral throughout the ilmenite-rich part of the Euchre Pluton (Figure S1C). Scheelite grains have survived partial alteration of surrounding ilmenite grains to titanite, either during the waning stages of magmatism or subsequent potassic alteration.
Freds Camp Pluton
That part of the Freds Camp Pluton (Allibone & Tulloch Citation1997, Citation2004; Tulloch et al. Citation2009) within the OSH on the eastern side of South West Arm comprises massive, equigranular, fine- to medium-grained alkali feldspar granite. Discrete grains of sodic plagioclase are rare. Magnetite and biotite are the most abundant mafic minerals and are locally accompanied by riebeckite and accessory aegirine, ilmenite, zircon and apatite. Magnetite forms >95% of the Fe-Ti oxide in this part of the pluton. Widespread fine-grained green biotite and sericite and traces of titanite and epidote are inferred to be products of alteration rather than primary igneous minerals.
Silvertown Tonalite
The wedge-shaped body of coarse-grained, massive, equigranular tonalite and subordinate granodiorite c. 2 km long and up to 600 m wide between the southernmost strand of the FFS and Freds Camp Pluton is referred to as Silvertown Tonalite, after the Silvertown adit which is developed within it (). Small amounts of mineralised coarse-grained tonalite and granodiorite in the lower part of Ogles Creek, near the core of the Euchre Pluton (e.g. P58466, P58467 and P58468) may be xenoliths of Silvertown Tonalite in the younger (see geochronology below) Euchre Pluton syenogranite.
Euhedral to subhedral plagioclase, interstitial perthitic orthoclase and quartz dominate the magmatic mineral assemblage of Silvertown Tonalite. Minor coarse-grained brown biotite was the principal mafic mineral but is now largely replaced by intergrown clusters of fine-grained green-brown biotite and ilmenite whose shapes tend to mimic outlines of the original magmatic biotite grains. Accessory magmatic minerals include zircon, apatite, monazite and rare titano-magnetite, probable magmatic ilmenite (OU84916), xenotime and traces of uraniferous thorite (OU84914).
Hill 267 m leucogranitoid
Equigranular, medium- to coarse-grained, massive, leucogranite and leucogranodiorite form part of the ridge between the Scott Burn and Forked Creek in the vicinity of spot height 267 m (Figure S2A). Plagioclase in Hill 267 leucogranitoid is subhedral, whereas K-feldspar is generally interstitial. Mafic minerals are restricted to accessory amounts of biotite, magnetite and ilmenite. Magnetite is locally more common than biotite (e.g. OU84953) and ilmenite comprises <10% of the opaque oxide. Other accessory minerals include zircon, monazite, xenotime and apatite. Titanite and epidote are both present but generally fine-grained and commonly intergrown with phengite and chlorite inferred to have formed during alteration, which affected much of the leucogranitoid (Figure S2B).
Paleozoic dioritic rocks
Bodies of medium- to coarse-grained, equigranular, massive diorite are located along the contact between the Freds Camp and Euchre Plutons, within the southern part of the Freds Camp Pluton and within the northern tip of Ogles Point (). Primary diorite mineral assemblages that include hornblende, clinopyroxene, plagioclase and ilmenite have been largely replaced by epidote-rich alteration. Aplite dykes probably related to either the Euchre and/or the Freds Camp Plutons cut the diorite, indicating it is the older unit.
Dykes
Hypabyssal felsic and mafic dykes cut the Silvertown Tonalite along the eastern shore of South West Arm. The felsic dykes comprise numerous feldspar laths, very fine-grained interstitial quartz and feldspar, minor green-brown biotite and common accessory magnetite. Some (but not all) felsic dykes contain rare plagioclase phenocrysts, traces of actinolite and very fine ilmenite rimmed by titanite. The single mafic dyke examined has been overprinted by strong sericite-rutile alteration to the extent that no primary texture remains. Earlier, but possibly still hydrothermal, minerals preserved in the altered mafic dyke include green biotite, magnetite and ilmenite.
Basaltic andesite dykes cut the northern part of the Hill 267 leucogranitoid. The one dyke examined in detail is overprinted by chlorite, epidote and pyrite alteration, and cut by veinlets with a similar mineralogy (OU84950). However, neither this alteration nor the associated veinlets are associated with any elevated Au, Ag, Bi, Cu, Mo, Te or W values (Table S1).
Mafic dykes with alkaline and/or lamprophyric characteristics occur in many terranes prospective for precious metal deposits, and their presence is commonly interpreted as an indicator of higher prospectivity. Dykes seen to date in the OSH and Hill 267 HS lack phenocrysts of hydrous mafic minerals, indicating they are not lamprophyres. However, alteration has modified the original composition of some mafic dykes to the extent it is not known whether their original composition was alkaline or calc-alkaline.
Primary chemistry of the granitoid rocks
Unaltered parts of the Euchre Pluton are weakly peraluminous ferroan to magnesian, and calc-alkaline to alkali-calcic, whereas the adjacent Freds Camp Pluton is ferroan, alkali to alkali-calcic, and weakly peraluminous to weakly peralkaline (Figure S3). The ferroan, alkali-calcic composition of some Euchre Pluton samples suggests an affinity with the adjacent, more strongly ferroan and alkalic Freds Camp Pluton. Unaltered parts of the Hill 267 leucogranitoid and the Silvertown Tonalite both have magnesian, calc-alkalic and weakly peraluminous compositions that are slightly more magnesian and calc-alkalic than the Euchre Pluton. Rb vs Nb+Y and Ga vs high-field-strength element (HFSE) contents of the Euchre Pluton, Hill 267 leucogranitoid and Silvertown Tonalite are typical of arc-related I-type granites, whereas those of the adjacent Freds Camp Pluton scatter between volcanic arc and within-plate granite fields (Figure S3), characteristics typical of many A-type granites and consistent with the ferroan, alkalic composition of the pluton.
Rb/Sr ratios within the Euchre Pluton, Hill 267 leucogranitoid and Silvertown Tonalite are <1, whereas those within the Freds Camp Pluton have a range of 0.7–20 (Figure S3). None of the plutons described here have wider ranges of Rb/Sr ratios like those which commonly characterise highly fractionated mineralised granites (e.g. Mustard Citation2004). Higher Rb/Sr ratios in some samples of the Freds Camp Pluton do not correlate with higher SiO2 contents, implying that elevated Rb/Sr ratios in this pluton are not the result of feldspar fractionation.
Rare Earth element (REE) contents and profiles of all four locally mineralised plutons are generally comparable with those of average upper continental crust and shale (). One sample of Freds Camp Pluton has a higher REE content than average continental crust, whereas the REE contents of the Silvertown Tonalite are slightly lower than average continental crust. Small negative Eu anomalies are present in the profiles of all unaltered samples except one sample of the Euchre Pluton which has a small positive Eu anomaly, implying some local accumulation as well as fractionation of plagioclase within the pluton. The REE data again highlights the largely unremarkable geochemical character of the four mineralised plutons. However, comparison of the REE profiles of unaltered and mineralised samples highlights the marked effect hydrothermal activity had on the absolute and relative contents of REE in all four plutons (see below).
Figure 4. Rare Earth element (REE) profiles of unaltered, altered and mineralised rocks from each major pluton within the OSH and HHS. REE profiles of unaltered rocks are unremarkable and similar to those of arc-granitoids derived from rocks that lack residual garnet. However, REE profiles of altered and mineralised granites are more complex, implying mobility of REE during hydrothermal activity.
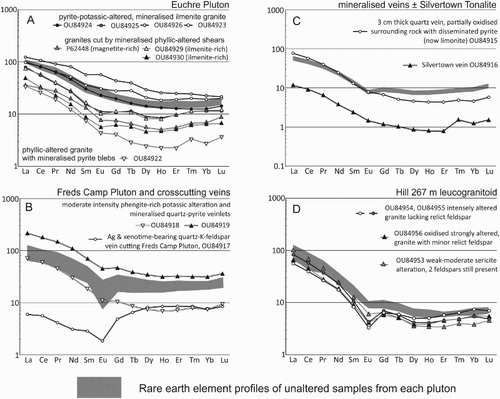
U–Pb zircon geochronology
Zircon crystallisation ages are calculated based on weighted mean 206Pb/238U dates from statistically coherent clusters of analyses. Relative probability density plots and weighted mean averages were plotted to visually assess the spread in data. Weighted mean averages were calculated after discarding clear outliers (i.e. Pb-loss and inheritance). Where mean square weighted deviations (MSWDs) were >2, signifying the existence of potentially more than one age population, the ‘Unmix’ algorithm of Isoplot was used to obtain an unbiased evaluation of the different ages.
OU84955–Hill 267: mineralised leucogranitoid
A total of 39 out of 40 zircon grains analysed were <10% discordant. After removal of two distinctly younger grains (Pb-loss affected), and one distinctly older (inherited) grain (A), a weighted mean average of 296.6 ± 4.5 Ma (2σ) and an MSWD of 14 was calculated for 36 grains. The high MSWD clearly indicates the probability of multiple age populations. The ‘Unmix’ function of Isoplot was therefore applied, resulting in a two-component split in the population: a younger age of 279.6 ± 2.1 Ma (28% of the population; 10 grains) and an older age of 304. 6 ± 1.5 Ma (72% of the population; 26 grains). Previous studies have demonstrated that zircons from nearby plutons (i.e. Freds Camp) are commonly affected by Pb-loss as a result of proximal Mesozoic intrusives (Tulloch et al. Citation2009), and we therefore consider the younger age to reflect Pb-loss. After excluding three distinctly older grains (A), a weighted mean average of 303.3 ± 1.6 Ma (MSWD = 1.5) was calculated from 23 grains that defined the older age population, which is considered the best estimate of the crystallisation age.
Figure 5. Zircon U–Pb LA-ICPMS ages for Hill 267, Euchre and Silvertown granitoid plutons. Probability density plots of 238U/206Pb ages and weighted means of selected spot ages are shown on the left- and right-hand sides, respectively. The broad probability density peak and lack of a plateau in the weighted mean plot for P62445 do not allow an age to be calculated. Gaussian deconvolution of this peak suggests the presence of three populations. The oldest peak (c. 295 Ma) is likely a minimum age for this sample.
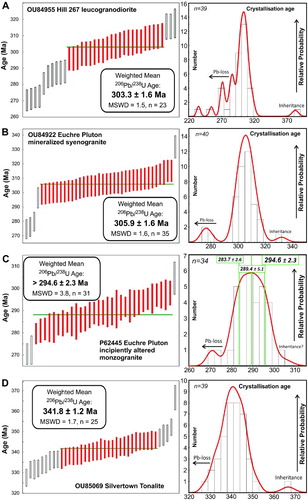
OU84922–Euchre Pluton: mineralised syenogranite
A total of 40 grains were analysed, all of which are <10% discordant. After excluding four younger grains interpreted to be affected by Pb-loss (<297 Ma) and three older outliers (>315 Ma), a weighted mean average of 305.9 ± 1.6 Ma (2σ) and an MSWD of 1.6 was calculated for 35 grains (B), which is considered the best estimate of the crystallisation age.
P62445–Euchre Pluton: unmineralised monzogranite
Thirty-four zircon grains were <10% discordant from a total of 48 grains analysed. Two young dates (<280 Ma) interpreted to be the result of Pb-loss, and one older date (>300 Ma) inferred to be inherited (C), were excluded from the weighted mean age calculations. A weighted mean average of 289.0 ± 2.0 Ma and an MSWD of 3.8 were calculated for 31 analyses. Application of the ‘Unmix’ function of Isoplot results in a three-component split in the population (relative misfit of 0.8): a younger age of 283.7 ± 2.6 Ma (37% of the population); a middle age of 289.4 ± 5.1 Ma (28% of the population); and an older age of 294.6 ± 2.3 Ma (36% of the population). The probability density plot in C does not display a clear Gaussian peak; determining an age of crystallisation for sample P62445 is therefore difficult. This granite displays alkaline chemistry and, as such, is more predisposed to suffer Pb-loss and less likely to contain inherited zircon as alkaline magmas commonly dissolve any xenocrystic zircon. Ti–Zr temperatures (Ferry & Watson Citation2007) for this granite are the highest for all analysed samples at 876 °C. The large spread in 206Pb/238U ages is interpreted to reflect widespread Pb-loss, and the 294.6 ± 2.3 Ma age is therefore likely to represent the minimum age of granite crystallisation.
OU85069–Silvertown Tonalite
Forty-eight zircon grains were analysed, of which 39 are <10% discordant and included in the age calculation. Probability density plots show some evidence for Pb-loss (negative skew) and inheritance (clear outlier) (D). A weighted mean of all 39 zircon analyses produced an age of 340.8 ± 2.0 with an MSWD of 8, reflecting the probability of multiple age populations. After removing the older outlier, application of the ‘Unmix’ function resulted in a four-component split (relative misfit of 0.6): 331.9 ± 2.3 Ma (16% of population, 6 grains); 339.0 ± 2.2 Ma (43% of population, 16 grains); 344.7 ± 2.5 Ma (36% of population, 14 grains); and 350.5 ± 3.9 Ma (5% of population, 2 grains) For the same reasons as outlined above for OU84955, the younger ages are interpreted to reflect Pb-loss and we consider the middle plateau of ages to best represent the crystallisation of this sample (D). A weighted mean average of 341.8 ± 1.2 Ma (MSWD = 1.7) for 24 grains (60% of the population) is calculated.
The Ogles Creek–Silvertown Hydrothermal System (OSH)
A complex variety of hydrothermal alteration assemblages, mineralisation styles and overprinting relationships are present within rocks affected by the OSH (). The various hydrothermal assemblages do however appear to have formed during three main stages, as described in the following.
Phase 0: Early unmineralised biotite-ilmenite potassic alteration confined to the Silvertown Tonalite. The restricted distribution and unmineralised character of this assemblage implies it formed prior to emplacement of the younger Freds Camp and Euchre Plutons, and is not part of the OSH. It may instead be related to cooling of the Silvertown Tonalite at c. 342 Ma.
Phase 1: Mineralogically complex, zoned, potassic and propylitic assemblages associated with several styles of sulphide-telluride mineralisation in the Euchre Pluton and southern part of the Freds Camp Pluton.
Phase 2: Mineralogically zoned phyllic and sericitic assemblages commonly developed along mineralised brittle-ductile shears, faults and fractures, and in alteration haloes around mineralised quartz veins.
Figure 6. Schematic illustration of paragenetic relationships and the relative distribution of mineral and metal assemblages within the OSH.
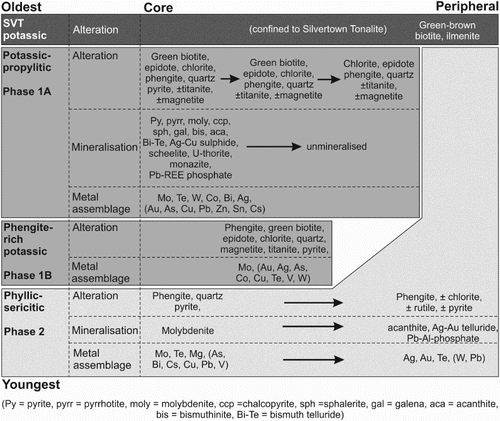
The change from potassic-propylitic to phyllic-sericitic assemblages apparent in the OSH has long been recognised in many porphyry-related hydrothermal systems (e.g. Lowell & Guilbert Citation1970), where it commonly reflects an initial episode of prograde magmatic-hydrothermal activity followed by a later phase of retrograde hydrothermal activity during incursion of meteoric fluids as the system cools. Phyllic and sericitic assemblages tend to initially develop on the margins of hydrothermal systems in rocks that may not have been affected by potassic-propylitic alteration; as the system cools, they migrate inwards replacing earlier propylitic and potassic assemblages. A similar general paragenesis is apparent in the OSH ().
Phase 1 alteration and mineralisation
Potassic-propylitic alteration
Four potassic-propylitic alteration assemblages are distinguished within rocks affected by phase 1 hydrothermal activity in the OSH (). Core biotite, pyrite-bearing potassic alteration in the ilmenite-rich part of the Euchre Pluton grades eastwards into a pyrite-absent potassic assemblage, and then a propylitic assemblage that lacks both biotite and pyrite (). This zoned sequence of potassic-propylitic alteration assemblages is inferred to reflect a decrease in sulphur fugacity and temperature with increasing distance from the ilmenite-rich core of the Euchre Pluton.
A fourth potassic assemblage which may mark a transition between potassic and phyllic alteration is present in the vicinity of the contact between the Euchre and Freds Camp Plutons (, 6). Here the amount of phengite relative to green biotite is greater than in the core of the Euchre Pluton implying a change in fluid chemistry, perhaps to a more acidic composition which favours phengite stability over biotite. Carbonate, a common mineral group in potassic and propylitic assemblages elsewhere, is conspicuous by its absence from the OSH.
Phase 1 mineralisation
Mineralised pyrite occurs as disseminated grains (Figure S4), millimetre–centimetre-sized clots (), and millimetre–centimetre-wide planar veins () within rocks overprinted by the core sulphide-bearing potassic alteration assemblage. This pyrite contains inclusions of a wide variety of sulphide, oxide, telluride and phosphate minerals typically <25 µm across (, 7, S4). Additional molybdenite is intergrown with silicate gangue minerals that form the core potassic assemblage (Figure S4C). The presence of biotite, epidote and less common magnetite in many pyrite-rich veinlets and biotite selvedges around some pyrite veinlets and clots indicate a close relationship between potassic alteration and mineralisation (A, C–D). Arsenopyrite and stibnite, common minerals in many intrusion-related hydrothermal systems (e.g. Thompson et al. Citation1999), are conspicuous by their absence from the OSH.
Figure 7. Mineralised pyrite-rich clots in granite affected by phase 1 potassic and phase 2 phyllic alteration (OU84922). A, Pyrite-rich blebs partly surrounded by the remains of biotite haloes which survived later phyllic alteration. B, Pyrite-rich clot in which pyrite grains are intergrown with adjacent sericite-altered feldspars and quartz, rather than forming a continuous vein that cuts the adjacent granite. C, Pyrite grains within the clots contain inclusions of pyrrhotite, chalcopyrite, sphalerite, galena, bismuthinite, Bi-telluride, molybdenite, acanthite and monazite.
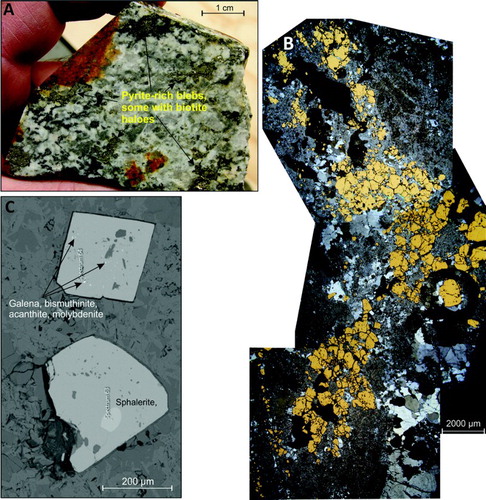
Figure 8. A, B, Mineralised pyrite, quartz, biotite, epidote, ± magnetite veins in the core of the Euchre Pluton (OU84924). C, Pyritic veins pass along-strike into biotite ± epidote-filled fractures a few hundred microns wide, indicating a direct link between the veins and potassic alteration (plane polarised light, OU84924). D, Some veins comprise coarse pyrite grains linked by segments that comprise finer quartz, biotite and epidote, a texture transitional between the planar veins illustrated in A–C and pyrite-rich clots in Figure S4 (OU84926). Magnetite is present in the vein despite the ilmenite-rich mineralogy of the host granite. E, F, Partially oxidised stockworks of mineralised quartz-pyrite veinlets and phengitic micro-shears in phengite-rich potassic-altered Freds Camp Pluton granite.
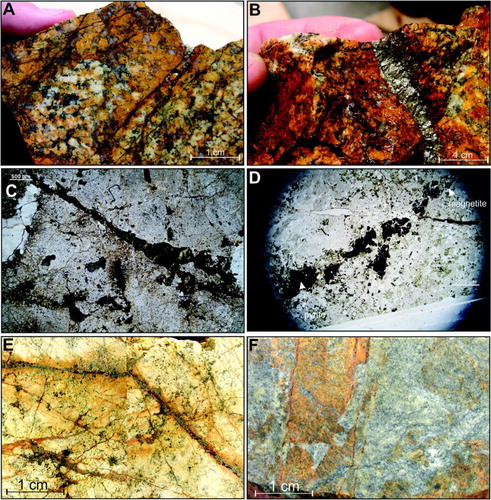
Isocon plots (Grant Citation1986) highlight the degree to which rocks affected by pyrite-bearing potassic alteration are enriched in particular metals, something which cannot be assessed from the mineralogical data (). Samples that contain pyrite veinlets and clots have 10–200 times the background amounts of S, Mo, Te, Co, W and Bi present in unmineralised Euchre Pluton granite (A, B). Mineralised rocks are also enriched in As, Ag, Au, Cu and Cs at 5–10 times the background contents of unmineralised granite. This particularly diverse metal assemblage is restricted to pyritic-potassic altered rocks in the core of the OSH, but absent from mineralised rocks associated with other alteration assemblages further to the north (, A). The isocon plots imply pyrite-bearing potassic alteration has not had a major effect on the bulk REE contents of the mineralised rocks (B). However, the lack of Eu anomalies and different slopes of the REE profiles of the altered rocks compared with REE profiles of the unmineralised granite implies the REE were selectively mobilised during potassic alteration (A).
Figure 9. Isocon plots (Grant Citation1986) comparing the composition of mineralised and unmineralised rocks from each pluton within the OSH and HHS. The isocon plots confirm the mineralised character of the pyrite ± quartz clots and veins associated with potassic, phyllic and sericitic alteration in OSH intensely altered rocks in the HHS.
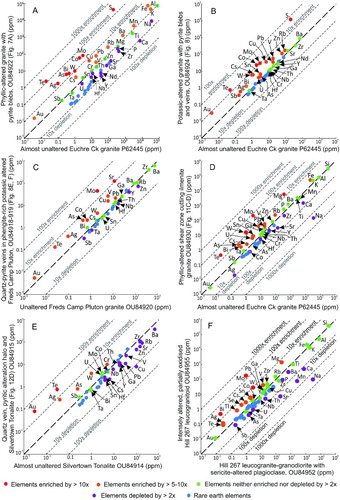
Figure 10. A, Geological map of the OSH showing the distribution of different metal assemblages and the orientation of mineralised structures. The listed metals are present at more than 5 times background values, whereas those metals denoted ± are present at 2–5 times background values. B, Contoured equal-area stereographic projections of mineralised vein and shear orientations. Mineralised veins and shears strike either northeast, parallel to the long axis of the OSH or northwest parallel to the FFS.
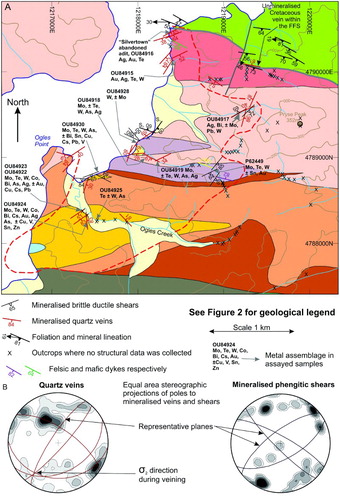
Southern parts of the Freds Camp Pluton affected by phengite-rich potassic alteration are locally cut by stockworks of quartz-pyrite veinlets (E, F) (OU84918, OU84919). Individual veinlets are generally <1–2 mm wide, but the related stockworks extend throughout outcrops c. 1 by 10 m in size. No sulphide or telluride minerals other than pyrite were observed in the stockwork veinlets, but assay data indicate they are enriched in Mo and, to a lesser degree, Au, Ag, As, Co, Cu, Te, Sr, V and W compared with unaltered Freds Camp Pluton (C). This metal assemblage is similar to that associated with pyritic-potassic alteration in the core of the Euchre Pluton (A). Negative Eu anomalies characteristic of unaltered Freds Camp Pluton are almost absent from rocks affected by phengite-rich potassic alteration (B, OU84918, OU84919), implying significant mobility of some REE during phengite-rich potassic alteration in the OSH.
Phase 2 alteration and mineralisation
Phyllic and sericitic alteration
Phyllic alteration is dominated by phengite, but also commonly includes minor quartz, pyrite and locally molybdenite (). Igneous K-feldspar that was generally stable during earlier potassic alteration, and biotite, epidote and magnetite derived from the earlier potassic assemblages, were partly to completely replaced by phengite where affected by phyllic alteration (A, B). Phyllic-altered rocks occur sporadically throughout the western part of the Euchre Pluton in both ilmenite and magnetite-rich granite previously affected by potassic alteration (Figure S4A). Phyllic assemblages are developed in patchy irregular-shaped zones with no obvious structural control and 1–3 dm wide brittle-ductile shears that contain entrained partial altered blocks of the adjacent granite, older quartz ± pyrite veins and isolated disseminated pyrite grains (C, D).
Figure 11. A, Patchy strong phyllic alteration partly replacing biotite and epidote formed during earlier potassic assemblage (OU84922, xpolars, scale bar 200 μm). B, Intense phyllic alteration enclosing remains of an igneous K-feldspar grain (OU84922, xpolars, scale bar 200 μm). C, Part of a phengite-altered shear that contains entrained fragments of ilmenite granite and disseminated hydrothermal pyrite (OU84930). D, Microscopic porphyroclasts of dismembered quartz +/− pyrite veins within the shear shown in C. Minor disseminated molybdenite (not shown) is intergrown with phengite.
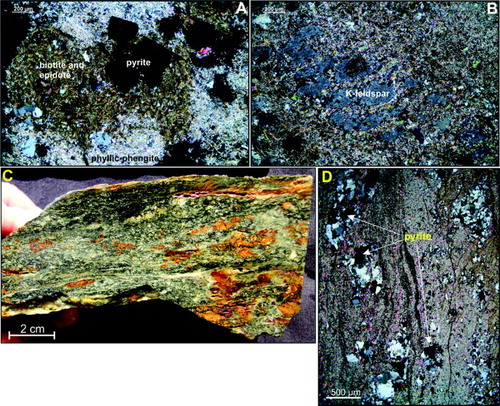
Sericitic alteration is also dominated by phengite but includes rutile, chlorite and pyrite, the latter generally restricted to narrow selvedges around mineralised quartz veins (). Molybdenite locally present in the phyllic assemblage has not been observed in the sericitic assemblage. In weakly sericite altered rocks phengite has replaced c. 10% of the plagioclase, but other minerals remain largely unaffected. Additional phengite is present in millimetre-wide fractures. In more strongly altered rock, >90% of the plagioclase is replaced by fine-grained phengite, and biotite and ilmenite are partly replaced by phengite, rutile and chlorite, but some K-feldspar remains unaltered. Where sericitic alteration is especially intense along cataclastic faults and in selvedges adjacent to mineralised quartz veins, phengite, rutile, minor chlorite and pyrite replace the entire rock aside from isolated relict quartz (B). Sericitic alteration is widely developed in the northern peripheral part of the OSH where it affects most of the Silvertown Tonalite, parts of the Freds Camp Pluton and cross-cutting felsic and mafic dykes to varying degrees.
Figure 12. A, Disseminated pyrite in sericite-altered tonalite adjacent to the Silvertown vein. B, Magnetite and biotite replaced by rutile and phengite, respectively, in altered tonalite adjacent to the Silvertown vein (backscatter SEM image). C, Acanthite inclusions in pyrite and along pyrite grain boundaries in the Silvertown vein (OU84916, backscattered SEM image). D, Minor mineralised quartz vein and halo of disseminated pyrite that contain anomalous Au, Ag and Te (E) in Silvertown Tonalite (OU84915).
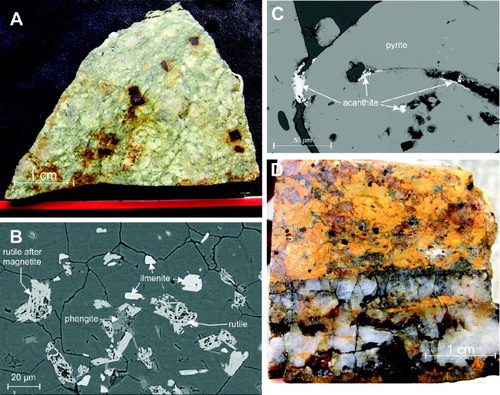
Mineralisation associated with phyllic alteration
Some phyllic-altered shears contain disseminated molybdenite intergrown with the phengite (C, D). No other ore sulphides or tellurides were found in the phyllic-altered shear investigated in detail (OU84930), but assay data indicate this shear contains elevated Mo, Te, As, Bi, Sn, Cu and V compared with unmineralised Euchre Pluton granite, assuming minimal change in rock volume during development of the shear (D). Scheelite forms part of the igneous assemblage in entrained fragments of ilmenite granite rather than the phyllic alteration assemblage. Euchre Pluton granite cut by phyllic-altered shears is commonly strongly depleted in REE compared with unmineralised Euchre Pluton granites (A), again highlighting the mobility of REE within the OSH.
The c. 20 cm thick quartz vein in the Silvertown adit is the thickest vein found to date in the OSH. It is flanked by a halo of sericite-altered granite that contains numerous cubes of disseminated pyrite (A), implying mineralised veins such as those at Silvertown formed from the same fluid as the sericitic alteration. Quartz comprises >95% of the vein with the remaining 5% largely made up of phengite and pyrite. Additional less common minerals within the vein include acanthite, Ag, ± Au, ± Pb telluride, apatite and an unidentified Pb–Al–Fe phosphate that locally rims apatite (C). Acanthite is the dominant ore mineral and occurs as grains up to 20 µm across along pyrite grain boundaries and less common inclusions within pyrite, whereas tellurides occur exclusively as inclusions in pyrite <3 µm across.
Narrower quartz ± K-feldspar veins likely related to the Silvertown vein occur widely along the coast and in hills to the east, and two were examined in detail. One 5–10 cm thick vein located along a 1–2 cm wide fault within the Freds Camp Pluton (OU84917) contains quartz, K-feldspar, magnetite, a Zn- and Mn-rich analogue of ilmenite, xenotime, an unidentified Pb-, Al-, Fe- and S-bearing phosphate mineral, traces of pyrite, barite, and ankerite, and 14 ppm Ag (). This is the only known occurrence of a carbonate mineral in the OSH. The presence of xenotime is reflected in the REE profile of the vein, which shows a marked enrichment in medium to heavy REE compared with the light REE (B), again indicating selective mobilisation of some REE during hydrothermal activity within the OSH.
The second vein is 3–4 cm wide, cuts the Silvertown Tonalite and is partially surrounded by an oxidised halo of pyrite-sericite alteration (OU84915) (D, A). No ore-minerals were seen in the quartz vein or its pyritic halo, but analyses of the vein and its alteration halo indicate they contain elevated Au, Ag, Te and, to a lesser extent, W compared with unaltered Silvertown Tonalite (E). The apparent depletion of this sample in many trace elements in the isogon plot likely reflects dilution of these elements concentrated in the country rock by the vein material.
Mineralised veins in the northern part of the OSH contain the highest Au and Ag values obtained to date from the OSH, as well as Pb- and Zn-bearing minerals not found elsewhere in the OSH. Metals such as Mo, W, Co and Bi which are all enriched in pyritic-potassic and phyllic-altered rocks further to the south are largely or completely absent from these northern veins associated with sericitic alteration ().
Structure within the Ogles Creek–Silvertown Hydrothermal System
Mineralised veins and phyllic-altered shears commonly strike in a NNE–ENE direction, approximately parallel to the long axis of the OSH (). A smaller group of veins and shears strike in a northwest direction, approximately parallel to the FFS immediately to the north. Many veins and most shears dip steeply, but a smaller group of northeast-striking veins dip moderately to gently towards the southeast.
Little kinematic data is available for the mineralised shears and veins. A steeply plunging down-dip mineral elongation lineation was noted on two of the NE–ENE-striking shears, implying phyllic alteration occurred during dip-slip movement on these structures. Quartz vein orientations intersect along a gently southwest-plunging direction (B) that may represent the σ2 direction during veining, again consistent with dip rather than strike-slip motion on the northeast-striking shears during veining.
The axis of the hydrothermal system is discordant to the generally east-trending orientation of intrusive contacts between the various plutons and internal contacts within the Euchre Pluton (A). Hydrothermal activity may therefore have been focused in a cryptic transfer-like fracture system, which cut across the general extension direction that controlled the orientation and shape of late Carboniferous granitoid plutons.
The Hill 267 Hydrothermal System (HHS)
Altered and mineralised rocks within the HHS are generally orange, yellow and/or red coloured (A–C), reflecting their partially oxidised character. Fe-oxyhydroxides form disseminated specks, veins and centimetre-scale gossanous blebs within the altered granite. Relict pyrite and less common molybdenite are preserved sporadically (B, D) within the Fe-oxyhydroxides. Jarosite staining is present on some weathered surfaces, especially those with a more sheltered aspect, implying sulphides were an important component of the unoxidised protolith.
Figure 13. Altered, veined and mineralised rocks from the HHS. A, Strongly altered partially oxidised granite comprising relict igneous quartz, phengite and a limonite-filled cavity (2116800 E, 5357750 N). B, Miarolitic cavity filled with quartz, pyrite and molybdenite (OU84955). C, Strongly altered partially oxidised granite containing cavities filled with gossanous material and relict pyrite (OU84956). D, Silicified granite containing disseminated pyrite and traces of molybdenite (from the same outcrop as OU84955). E, F, Altered granite cut by irregular-shaped veins partly filled by vuggy white quartz developed in large miariolitic cavities. G, Disseminated molybdenite intergrown with phengitee, backscattered SEM image (OU84954). H, Disseminated rutile in quartz, the main source of W in the HHS, backscattered SEM image (OU84954).
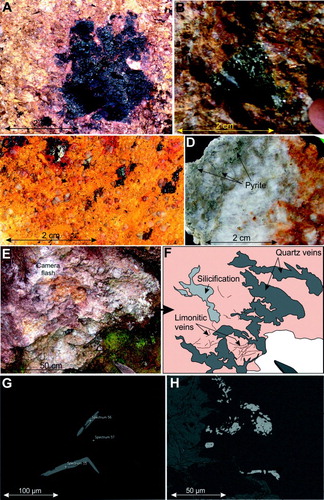
Irregular and ovoid-shaped cavities filled with quartz, pyrite and molybdenite or their oxidised remains occur widely in the altered granite (A, B). These cavities and their infill quartz crystals have textures typical of miarolitic cavities formed when exsolved gas bubbles become trapped in the granite magma during the final stage of crystallisation. Larger, irregular, sinuous and discontinuous veins up to a metre long and 10 cm thick filled with milky quartz prisms also cut the altered granite locally (E, F). Internal textures of the veins and smaller cavities are indistinguishable, suggesting the veins formed in larger miarolitic cavities in the host granite.
Strongly altered granite surrounding the cavities and quartz veins typically comprises relict igneous quartz and abundant phengitic muscovite with little or no relict feldspar. Common minor phases in the altered granite include rutile, monazite, xenotime and zircon. Rare tourmaline grains, generally only a few microns across, and an unidentified Al-rich phosphate also occur in the altered rocks. Laths of molybdenite a few tens of microns in size are sporadically intergrown with the phengitic muscovite (G). Pyrite was also probably widely disseminated throughout the altered rocks before being oxidised to Fe-oxyhydroxides (D). Some Fe-oxyhydroxides contain several percent Cu, suggesting chalcopyrite may also have been present in the unoxidised rocks. Rutile grains contain up to c. 1.2 wt% W, 0.6 wt% Nb and several percent V (when calculated as trivalent oxides) (H). No scheelite or wolframite were found in the altered rocks despite the presence of up to 25 ppm W in some samples (F; Table S1), suggesting all the W present in the altered rocks is contained within rutile.
More intensely altered rocks are ten–hundred times richer in Mo and W than unaltered granite (F), consistent with the mineralogy outlined above. The altered rocks also contain 5–20 times background Bi, Sn, Se, Cu, Ag and Te in unaltered Hill 267 leucogranitoid. These enrichment values are a minimum since some metal may have been removed from the rocks during oxidation. The more strongly altered and mineralised rocks are depleted in Ca, Na, Mn and Sr, reflecting destruction of feldspar and manganiferous Fe–Ti oxides during alteration. Depletion of the altered rocks in Sb and As may also be due to destruction of sulphides during oxidation rather than a primary feature of the hydrothermal system.
REE profiles of the more intensely altered and mineralised Hill 267 leucogranitoid consistently have larger negative Eu anomalies than the unaltered to weakly altered parts of the Hill 267 leucogranitoid (D). This implies the mineralised part of the pluton crystallised from a more fractionated magma than the unmineralised parts.
Discussion
Regional geological implications of the geochronology
Allibone (Citation1991) included the leucogranodiorite from Hill 267 in the 342 ± 4 Ma Ruggedy Granite based on the petrographic similarity and apparent continuity of leucogranite throughout the area in between. However, new geochronological data included here and in Allibone et al. (Citation2016) indicates that the Ruggedy Granite as mapped previously includes three plutons with ages of c. 140–342 Ma.
This new U–Pb zircon data indicates the Euchre Pluton and Silvertown Tonalite are Carboniferous in age rather than Jurassic (Turnbull & Allibone Citation2003; Allibone & Tulloch Citation2004). The Silvertown Tonalite is a correlative of c. 340 Ma Neck Granodiorite immediately south of the FFS 15 km to the east, whereas the Euchre Pluton is a correlative of the Hill 267 leucogranitoid c. 15 km to the west (). Both the I-type Euchre Pluton and Hill 267 leucogranitoid are the same age (within uncertainties) as the A-type Freds Camp Pluton, indicating coeval A- and I-type plutonism occurred in central Stewart Island c. 305–300 Ma ago.
Relationships between Carboniferous plutons and mineralisation
Mineralised rocks in central Stewart Island are restricted to the various plutons emplaced during the Carboniferous.
Epidote, chlorite, green biotite alteration similar to the sulphide-absent potassic-propylitic alteration in the Euchre Pluton does occur widely in the adjacent Jurassic plutons (Allibone & Tulloch Citation2004), indicating that hydrothermal activity also occurred during the Mesozoic. However, the altered Jurassic rocks lack sulphide mineralisation aside from barren pyrite-epidote-chlorite-filled fractures in OU84951 (, Table S1) and a decimetre-wide molybdenite-bearing shear c. 10 km south of the OSH at Hill 511 (, P60662A, B Table S1). The barren character of the Jurassic rocks implies that mineralisation within the OSH and HHS occurred during the Carboniferous.
The presence of scheelite inclusions in ilmenite from the Euchre Pluton (Figure S1C) indicates scheelite crystallised at the same time as ilmenite directly from the syenogranite melt in the core of the pluton. This is the most conclusive evidence found to date that mineral fractionation in the core of the Euchre Pluton produced a melt enriched in W. The coincidence of ilmenite-rich granite, metalliferous pyritic-potassic alteration and the most diverse metal assemblages in the OSH (A) all imply a direct relationship between hydrothermal activity and the core of the Euchre pluton. Sericitic alteration and Au-, Ag-, Te- and Pb-bearing metal assemblages more distant from the core of the Euchre Pluton (A) are similar to peripheral alteration and metal assemblages in many porphyry and intrusion-related gold deposits (e.g. Thompson et al. Citation1999; Morrison & Blevin Citation2001; Černý et al. Citation2005; Seedorff et al. Citation2005).
The widespread occurrence of quartz-sulphide-filled miarolitic cavities in the more fractionated upper part of the Hill 267 leucogranitoid implies a metalliferous magmatic-hydrothermal fluid exsolved from this part of the pluton. Intense phengite-rich alteration that includes W-bearing rutile and molybdenite is confined to the more strongly fractionated granite that contains miarolitic cavities. Intense quartz, muscovite alteration such as that at Hill 267 is similar to zones of greisen alteration associated with granite Sn, W, Mo and Bi deposits. Only the apparent lack of topaz, fluorite, beryl, andalusite and larger amounts of tourmaline in the HHS distinguishes it from typical greisens, implying the hydrothermal fluid at Hill 267 may have contained less F, Be and B than fluids associated with more typical greisens.
Recognition of fertile granitoid plutons
Features such as miarolitic cavities, unidirectional solidification textures, widespread pegmatites and composite pegmatite-hydrothermal vein/dykes are commonly cited as indicators that a granitoid pluton may have generated a fertile magmatic-hydrothermal fluid (e.g. Thompson et al. Citation1999; Mustard Citation2001; Černý et al. Citation2005). These features are almost completely absent from the Euchre Pluton (Figure S1B), yet the pluton appears to have exsolved a metalliferous magmatic-hydrothermal fluid. Scattered aplite dykes north of the pluton core are similar to fine-grained leucogranite in the carapace of mineralised granite plutons in a variety of mineralised settings (e.g. White et al. Citation1981; Mustard Citation2004; Černý et al. Citation2005). However, apparently similar aplite dyke swarms occur around many barren plutons in other parts of southern New Zealand (e.g. Allibone & Tulloch Citation1997, Citation2004), indicating that the presence of aplite dykes is not generally an indicator of higher prospectivity. Although miarolitic cavities occur widely in mineralised parts of the Hill 267 leucogranitoid, pegmatites and composite vein-dykes were not observed in this pluton. Unaltered parts of the pluton are massive, homogenous and lacking any of the features listed above.
Neither the Euchre Pluton nor the Hill 267 leucogranitoid are highly fractionated, unlike mineralised granites in the core of many intrusion-related hydrothermal systems (Blevin & Chappell Citation1995; Mustard Citation2001, Citation2004; Blevin Citation2004; Černý et al. Citation2005). Most parts of both plutons are unremarkable I-type granitoids similar to numerous plutons in Phanerozoic continental-margin orogenic belts worldwide (Figures S2, S3). Although fractional crystallisation continued until the composition of the Hill 267 leucogranitoid approached the granite eutectic, it did not continue further and produce an incompatible trace-element-rich melt with Rb/Sr >10; the latter is more typical of intrusions associated with Mo- and Sn-rich mineralisation in particular.
Metal assemblages, original extent and prospectivity of the OSH and HHS
Intrusion-related metal deposits are generally parts of larger hydrothermal systems that include a variety of different metal assemblages zoned around the causative intrusion (e.g. Blevin & Chappell Citation1995; Thompson et al. Citation1999; Blevin Citation2004). Five main core metal assemblages are generally recognised in intrusion-related hydrothermal systems – Cu, Cu–Mo, W–Mo, Sn–W and Mo – reflecting variations in the oxidation state and degree of fractionation of the driving intrusion. Hydrothermal systems associated with each core metal assemblage may contain Au and Ag. Generally, the more fractionated and less oxidised the driving intrusion, the greater the distance between the high-temperature core assemblage and any related Au and Ag mineralisation (Blevin Citation2004).
Inner parts of the OSH and HHS are dominated by Mo, W, Bi, ± Co and Te (), implying similarities to hydrothermal systems that have a W–Mo core metal assemblage. Au and Ag are generally located in the peripheral parts of these systems, 1–3 km from the causative intrusion (Morrison et al. Citation1996; Thompson et al. Citation1999). Metal assemblages in the OSH show a similar zonation with Au- and Ag-rich veins located on the northern edge of the system c. 2 km from the Mo-, W-, Bi- and Co-rich core (, 12). General principles of convection imply hydrothermal activity would have been most intense above rather than to the side of the intrusion at the core of the OSH and HHS (e.g. Henley & McNab Citation1978). The most prospective parts of the OSH and HHS for precious metals were therefore likely to have been 1–3 km above the current land surface and have now been removed by erosion, leaving only the Mo-, W-, Bi- and ± Co-rich root of both systems and weakly mineralised peripheral Au-, Ag-, Te- and ± Pb-bearing veins such as those in the vicinity of Silvertown.
Although metal assemblages in the OSH and HHS are similar to those in many weakly oxidised intrusion-related Au deposits, the lack of arsenopyrite and Sb in both is unusual (Thompson et al. Citation1999). This may reflect metal zoning patterns in the wider now largely eroded hydrothermal systems, or unusual redox conditions such that As and Sb were not partitioned into the hydrothermal fluids. Carbonate is also an important gangue mineral in intrusion-related Au deposits, reflecting the CO2-rich composition of magmatic-hydrothermal fluids in many of these systems (Baker & Lang Citation2001; Baker Citation2002). However, only one grain of carbonate a few tens of microns across (OU84917) has been found in the OSH or HHS.
Both systems on Stewart Island may have contributed alluvial Au to local beach placers on Stewart Island and more extensive placers along the southern coast of the South Island (Craw et al. Citation2015). The source of Au in the placers along the south coast of the South Island has generally been assumed to be located within the South Island, but the discovery of kilometre-scale Au-bearing hydrothermal systems in Stewart Island provides an additional source of Au that may have contributed to South Island placers.
Regional metallogeny of southern and western New Zealand
Aside from the Sams Creek Au deposit in northwest Nelson, all major metalliferous hydrothermal systems discovered to date in southern and western New Zealand are located in the Buller rather than the Takaka terrane (e.g. Reefton Goldfield, Preservation Inlet Goldfield, Kirwans Au–W northwest Nelson porphyry Mo deposits). However, the OSH and HHS are both located on the northeastern limit of late Carboniferous plutonism on Stewart Island (), outboard of the margin of intact Takaka Terrane early–middle Paleozoic metasedimentary rocks. To date the only other metalliferous mineral occurrences documented in this tectonic setting in New Zealand are traces of molybdenite in the 157 ± 3 Ma Pomona Island Granite (Scott & Palin Citation2011) and minor Ag-base metal mineralisation along altered strands of the Grebe Shear Zone at Dana Peaks (Allibone et al. Citation2010), both in east-central Fiordland. The OSH and HHS therefore appear to be part of a previously unrecognised metallogenic event along the outboard margin of late Carboniferous New Zealand (Allibone & Craw Citationforthcoming). Mineralised intrusion-related hydrothermal systems developed in this setting during late Carboniferous and early Cretaceous extension and A-I-type granitoid plutonism. Similar hydrothermal systems may be present in this tectonic setting in east–central Fiordland and the Rotoroa Complex in the Buller district. Late Carboniferous–early Permian intrusion-related hydrothermal systems in northeast Queensland are more distant potential correlatives of the OSH and HHS (Morrison & Beams Citation1995; Lehrmann Citation2012).
Conclusions
Two major intrusion-related hydrothermal systems are present in central Stewart Island immediately south of the FFS. Both systems are directly related to late Carboniferous I-type granitoids emplaced close to the outboard margin of Gondwana at c. 305–300 Ma.
Unmineralised parts of the two causative intrusions are neither especially highly fractionated nor characterised by an unusual chemistry or mineralogy, which might indicate they are the source of metalliferous hydrothermal fluids. Features commonly associated with fertile metalliferous plutons such as miarolitic cavities, pegmatite dykes, unidirectional solidification textures and composite magmatic-hydrothermal vein-dykes are either absent from or confined to the most intensely altered and mineralised parts of the two causative intrusions.
Metal and gangue alteration assemblages within the two Stewart Island hydrothermal systems are similar to those present in intrusion-related metal deposits with Mo- and W-rich cores. Metal zoning patterns suggest more prospective upper parts of the two Stewart Island hydrothermal systems that may have been richer in Au and Ag have been removed by erosion, with only relatively weakly mineralised deeper and lateral-peripheral parts of both systems remaining. The weakly mineralised character of each system may also be due to the relatively unfractionated composition of the causative intrusions.
The two mineralised hydrothermal systems on Stewart Island are part of a previously unrecognised metallogenic event along the outboard margin of late Carboniferous Gondwana in southern New Zealand. Similar mineralised hydrothermal systems may be present in the same tectono-magmatic setting elsewhere in New Zealand.
Supplementary data
Table S1. Chemical analyses and summary descriptions of samples discussed here. Analytical methods noted in the table are the F650 and Au-AA21 fire assay methods for Au used by SGS and ALS laboratories, respectively, the M104 and ME-MS61/62 4 acid digest and ICP-MS finish methods for selected trace elements employed by SGS and ALS laboratories, respectively, and the ME-MS81 lithium metaborate fusion followed by 4 acid digest and ICP-MS finish method employed by ALS laboratories. Elements and methods denoted n.d. were not determined.
Table S2. LAICPMS zircon analytical data collected at the University of Otago.
Figure S1. Primary magmatic characteristics and possible miarolitic cavities within the mineralised Euchre Pluton.
Figure S2. Primary characteristics of unaltered to weakly altered parts of the mineralised Hill 267 leucogranitoid.
Figure S3. Primary chemical characteristics of mineralised plutons within the OSH and HHS
Figure S4. Mineralogical and textural characteristics of pyritic potassic alteration in the Euchre Pluton.
Figure S4. Mineralogical and textural characteristics of pyritic potassic alteration in the Euchre Pluton.
Download JPEG Image (3.6 MB)Figure S3. Primary chemical characteristics of mineralised plutons within the OSH and HHS
Download TIFF Image (1.6 MB)Figure S2. Primary characteristics of unaltered to weakly altered parts of the mineralised Hill 267 leucogranitoid.
Download JPEG Image (2 MB)Figure S1. Primary magmatic characteristics and possible miarolitic cavities within the mineralised Euchre Pluton.
Download JPEG Image (4.2 MB)Table S2. LAICPMS zircon analytical data collected at the University of Otago.
Download MS Excel (686 KB)Table S1. Chemical analyses and summary descriptions of samples discussed herein.
Download MS Excel (36.7 KB)Acknowledgements
Damien Walls provided XRF analyses of samples collected in 2009. Further XRF analyses of samples collected in 2013 were supplied by CRL Energy (Spectrachem) Ltd. Initial reconnaissance gold fire assays and ICPMS metal analyses of samples collected in 1996 and 2002 were supplied by SGS laboratories in Townsville and Perth, respectively. Additional gold fire assays and ICPMS metal analyses of samples collected in 2009 and 2013 were supplied by ALS laboratories in Brisbane. Kat Lilly assisted with the acquisition of scanning electron microscope data at Otago University. Bob Braithwaite provided numerous valuable comments which improved an earlier version of the manuscript. Transport in the field was provided by Rakiura Water Taxis, Rakiura Helicopters, Stewart Island Flights and Real Journeys. Capable and enthusiastic field assistance was provided by Robert and Andrew Smillie, Yvonne Cook and Paul Stenhouse.
Associate Editor: Dr Nick Mortimer.
Disclosure statement
No potential conflict of interest was reported by the authors.
Additional information
Funding
References
- Allibone AH. 1991. Volcanogenic and granitoid rocks from northwest Stewart Island, New Zealand. New Zeal J Geol Geop. 34:35–50. doi: 10.1080/00288306.1991.9514437
- Allibone AH, Ashley PM, Mackenzie D, Craw D. 2010. Shear hosted base metal mineralisation at Dana Peaks, Murchison Mountains, Fiordland, New Zealand. New Zeal J Geol Geop. 53:271–294. doi: 10.1080/00288306.2010.498406
- Allibone AH, Craw D. Forthcoming. Lithospheric controls on the metallogeny of basement rocks in southern and western New Zealand. In: Christie AB, editor. Mineral deposits of New Zealand: exploration and research. Monograph 31. Melbourne: The Australasian Institute of Mining and Metallurgy.
- Allibone AH, MacKenzie D, Turnbull R, Tulloch AJ, Craw D, Palin JM. 2016. Polymetallic mineralised veins associated with ferroan/A–type leucogranite, Stewart Island, New Zealand. New Zeal J Geol Geop. doi:10.1080/00288306.2016.1174947.
- Allibone AH, Tulloch AJ. 1997. Metasedimentary, granitoid, and gabbroic rocks from central Stewart Island, New Zealand. New Zeal J Geol Geop. 40:53–68. doi: 10.1080/00288306.1997.9514740
- Allibone AH, Tulloch AJ. 2004. Geology of the plutonic basement rocks of Stewart Island, New Zealand. New Zeal J Geol Geop. 47:233–256. doi: 10.1080/00288306.2004.9515051
- Allibone AH, Tulloch AJ. 2008. Early cretaceous dextral transpressional deformation within the median batholith, Stewart Island, New Zealand. New Zeal J Geol Geop. 51:115–134. doi: 10.1080/00288300809509854
- Angus PVM. 2014. An update on the Sams Creek porphyry Au deposit, northwest Nelson, New Zealand. In 2014 AusIMM New Zealand Branch Conference Proceedings 7–20.
- Baker T. 2002. Emplacement depth and carbon dioxide–rich fluid inclusions in intrusion–related gold deposits. Econ Geol. 97:1111–1117.
- Baker T, Lang JR. 2001. Fluid inclusion characteristics of intrusion–related gold mineralisation, Tombstone–Tungsten magmatic belt, Yukon Territory, Canada. Mineral Deposita. 36:563–582. doi: 10.1007/s001260100189
- Bierlein FP, Christie AB, Smith PK. 2004. A comparison of orogenic gold mineralisation in central Victoria (AUS), western South Island (NZ), and Nova Scotia (CAN): implications for variations in the endowment of Paleozoic metamorphic terrains. Ore Geol Rev. 25:125–168. doi: 10.1016/j.oregeorev.2003.09.002
- Black LP, Kamo SL, Allen CM, Davis DW, Aleinikoff JN, Valley JW, Mundil R, Campbell IH, Korsch RJ, Williams IS, Foudoulis C. 2004. Improved 206Pb/238U microprobe geochronology by the monitoring of a trace-element-related matrix effect; SHRIMP, ID-TIMS, ELA-ICP-MS and oxygen isotope documentation for a series of zircon standards. Chem Geol. 205:115–140. doi: 10.1016/j.chemgeo.2004.01.003
- Blevin PL. 2004. Redox and compositional parameters for interpreting the granitoid metallogeny of eastern Australia: implications for gold-rich ore systems. Resour Geol. 54:241–252. doi: 10.1111/j.1751-3928.2004.tb00205.x
- Blevin PL, Chappell BW. 1995. Chemistry, origin, and evolution of mineralised granitoids in the Lachlan Fold Belt, Australia: the metallogeny of I- and S-type granitoids. Econ Geol. 90:1604–1619. doi: 10.2113/gsecongeo.90.6.1604
- Braithwaite RL, Rabone SDC. 1985. Heavy metal sulphides and geochemical surveys for heavy metal sulphides in New Zealand. J Roy Soc New Zeal. 15:363–370. doi: 10.1080/03036758.1985.10421713
- Černý P, Blevin PL, Cuney M, London D. 2005. Granite–related ore deposits. In: Hedenquist JW, Thompson JFH, Goldfarb RJ, Richards JP, editors. Economic geology one hundredth anniversary volume. Littleton (CO): Society of Economic Geologists Inc.; p. 337–370.
- Christie AB, Braithwaite RL. 2003. Hydrothermal alteration in metasedimentary hosted orogenic gold deposits, Reefton Goldfield, South Island, New Zealand. Miner Deposita. 38:87–107. doi: 10.1007/s00126-002-0280-9
- Craw D, Kerr G, Reith F, Falconer D. 2015. Pleistocene paleodrainage and placer gold redistribution, western Southland, New Zealand. New Zeal J Geol Geop. 58:137–153. doi: 10.1080/00288306.2015.1007462
- Eggers AJ, Adams CJ. 1979. Potassium-argon ages of molybdenum mineralisation and associated granites at Bald Hill and correlation with other molybdenum occurrences in the South Island, New Zealand. Econ Geol. 74:628–637. doi: 10.2113/gsecongeo.74.3.628
- Faure K, Braithwaite RL. 2006. Mineralogical and Stable isotope studies of Au-As mineralisation in the Sams Creek peralkaline porphyritic granite, South Island, New Zealand. Miner Deposita. 40:802–827. doi: 10.1007/s00126-005-0013-y
- Ferry JM, Watson EB. 2007. New Thermodynamic models and revised calibrations for the Ti-in-zircon and Zr-in-rutile thermometers. Contrib Mineral Petr. 154:429–437. doi: 10.1007/s00410-007-0201-0
- Gollan M. 2006. Plutonic petrogenesis and mineralisation in southwest Fiordland [Unpublished MSc thesis]. Dunedin: University of Otago.
- Grant JA. 1986. The isocon diagram—a simple solution to Gresens’ equation for metasomatic alteration. Econ Geol. 81:1976–1982. doi: 10.2113/gsecongeo.81.8.1976
- Grapes RH, Challis GA. 1999. Gersdorffite with pentlandite, violarite, pyrrhotite, and pyrite, northwest Nelson, New Zealand. New Zeal J Geol Geop. 42:189–204. doi: 10.1080/00288306.1999.9514839
- Henley RW, McNab A. 1978. Magmatic vapour plumes and ground–water interaction in porphyry copper emplacement. Econ Geol. 73:1–20. doi: 10.2113/gsecongeo.73.1.1
- Kimbrough DL, Tulloch AJ, Coombs DS, Landis CA, Johnston MR, Mattinson JM. 1994. Uranium-lead zircon ages from the Median Tectonic Zone, New Zealand. New Zeal J Geol Geop. 37:393–419. doi: 10.1080/00288306.1994.9514630
- Lehrmann B. 2012. Polymetallic mineralisation in the Chillagoe district of northeast Queensland: insights into base metal-rich intrusion-related gold systems [Unpublished PhD thesis]. Townsville: James Cook University. 233pp + 80pp appendices.
- Lowell DJ, Guilbert JM. 1970. Lateral and vertical alteration-mineralisation zoning in porphyry ore deposits. Econ Geol. 65:373–408. doi: 10.2113/gsecongeo.65.4.373
- Ludwig K. 2003. Isoplot 3.00: a geochronological toolkit for Microsoft Excel: Berkeley Geochronology Center Special Publication 4. Berkeley: Geological Society of America Bulletin.
- Meinert LD, Dipple GM, Nicolescu S. 2005. World skarn deposits. In: Hedenquist JW, Thompson, JFH, Goldfarb RJ, Richards JP, editors. Economic geology 100th anniversary volume. Littleton (CO): Society of Economic Geologists Inc; p. 299–336.
- Morrison GW, Beams SD. 1995. Geological setting and mineralisation style of ore deposits of NE Queensland. In: Beams SD, editor. Mineral deposits of northeast Queensland: geology and geochemistry, contributions of the mineral deposit research unit. Townsville: James Cook University; p. 1–32.
- Morrison GW, Blevin PL. 2001. A practical and predictive model for igneous metallogeny. In: Williams PJ, editor. A hydrothermal odyssey, economic geology research unit, 52. Townsville, Australia: James Cook University; p. 144–147.
- Morrison GW, Seed M, Bobis R, Tullemans F. 1996. The Kidston gold deposits: a piston–cylinder model of gold mineralisation in a porphyry system. Geol Soc Aust Abstr. 41:303.
- Mortimer N, Turnbull R, Palin M, Tulloch A, Rollet N, Hashimoto T. 2015. Triassic–Jurassic granites on the Lord Howe Rise, northern Zealandia. Aust J Earth Sci. 62:735–742.
- Mustard R. 2001. Granite–hosted gold mineralisation at Timbarra, northern New South Wales, Australia. Miner Deposita. 36:542–562. doi: 10.1007/s001260100188
- Mustard R. 2004. Textural, mineralogical and geochemical variation in the zoned Timbarra Tablelands pluton, New South Wales. Aust J Earth Sci. 51:385–405. doi: 10.1111/j.1400-0952.2004.01064.x
- Nathan S, Rattenbury MS, Suggate RP. 2002. Geology of the Greymouth Area. Institute of Geological & Nuclear Sciences 1:250 000 geological map 12. 1 sheet + 58 p. Lower Hutt: Institute of Geological & Nuclear Sciences Ltd.
- Paton C, Hellstrom J, Paul B, Woodhead J, Hergt J. 2011. Iolite: freeware for the visualisation and processing of mass spectrometric data. J Anal Atom Spectrom. 26:2508–2518. doi: 10.1039/c1ja10172b
- Pearce NJG, Perkins WT, Westgate JA, Gorton MP, Jackson SE, Neal CR, Chenery SP. 1997. A compilation of new and published major and trace element data for NIST SRM 610 and NIST SRM 612 glass reference materials. Geostand Geoanal Res. 21:115–144. doi: 10.1111/j.1751-908X.1997.tb00538.x
- Phillips M. 2014. Geochemistry and timing, Sams Creek gold deposit, northwest Nelson, New Zealand [Unpublished MSc thesis]. Dunedin, New Zealand: University of Otago.
- Pirajno F. 1981. Geochemistry and mineralisation of the southern part of the Darran Complex, Fiordland, New Zealand. New Zeal J Geol Geop. 24:491–513. doi: 10.1080/00288306.1981.10422741
- Pirajno F. 1982. Geology, geochemistry, mineralisation, and metal zoning of the McConnochie greisenised granite, Reefton district, Westland, New Zealand. New Zeal J Geol Geop. 25:405–425. doi: 10.1080/00288306.1982.10421507
- Pirajno F, Bentley PN. 1985. Greisen-related scheelite, gold, and sulphide mineralisation at Kirwans Hill and Batemans Creek, Reefton district, Westland, New Zealand. New Zeal J Geol Geop. 28:97–109. doi: 10.1080/00288306.1985.10422279
- Rattenbury MS, Cooper R, Johnston MR. 1998. Geology of the Nelson Area. Institute of Geological & Nuclear Sciences 1:250 000 geological map 9. 1 sheet + 67 p. Lower Hutt: Institute of Geological & Nuclear Sciences Ltd.
- Rattenbury MS, Townsend D, Johnston MR. 2006. Geology of the Kaikoura Area. Institute of Geological & Nuclear Sciences 1:250 000 geological map 13. 1 sheet + 70 p. Lower Hutt: Institute of Geological & Nuclear Sciences Ltd.
- Scott JM, Palin JM. 2011. Molybdenite in Pomona Island Granite at Lake Manapouri, Fiordland, New Zealand. New Zeal J Geol Geop. 54:347–352. doi: 10.1080/00288306.2011.580768
- Seedorff E, Dilles JH, Proffett JM, Einaudi MT, Zurcher L, Stavast WJA, Johnson DA, Barton MD. 2005. Porphyry deposits: characteristics and origin of hypogene features. In: Hedenquist JW, Thompson JFH, Goldfarb RJ, Richards JP, editors. Economic geology one hundredth anniversary volume. Littleton (CO): Society of Economic Geologists Inc; p. 251–298.
- Thompson JFH, Sillitoe RH, Baker T, Lang JR, Mortensen JK. 1999. Intrusion–related gold deposits associated with tungsten tin provinces. Miner Deposita. 34:323–334. doi: 10.1007/s001260050207
- Titley SR, Beane RE. 1981. Porphyry copper deposits, Part 1, Geologic settings, petrology, and tectogenesis. Economic Geology 75th Anniversary Volume: 214–235.
- Tulloch AJ, Dunlap WJ. 2006. A Carboniferous 40Ar/39Ar emplacement age for the gold–bearing alkali feldspar granite dike at Sams Creek, west Nelson, New Zealand. New Zeal J Geol Geop. 49:233–240. doi: 10.1080/00288306.2006.9515162
- Tulloch AJ, Rabone SDC. 1993. Mo-bearing granodiorite porphyry plutons of the Early Cretaceous Separation Point Suite, west Nelson, New Zealand. New Zeal J Geol Geop. 36:401–408. doi: 10.1080/00288306.1993.9514586
- Tulloch AJ, Ramezani J, Kimbrough DL, Faure K, Allibone AH. 2009. U-Pb geochronology of Paleozoic plutonism in western New Zealand: implications for S-type granite generation and growth of the east Gondwana margin. Geol Soc Am Bull. 121:1236–1261. doi: 10.1130/B26272.1
- Turnbull IM, Allibone AH (compilers). 2003. Geology of the Murihiku Area. Institute of Geological & Nuclear Sciences 1:250 000 geological map 20. 1 sheet + 74 p. Lower Hutt: Institute of Geological & Nuclear Sciences Ltd.
- Turnbull IM, Allibone AH, Jongens R (compilers). 2010a. Geology of the Fiordland area. Institute of Geological & Nuclear Sciences 1:250 000 geological map 17. 1 sheet and 97 p. Lower Hutt: GNS Science.
- Turnbull R, Weaver S, Tulloch A, Cole J, Handler M, Ireland T. 2010b. Field and geochemical constraints on mafic–felsic interactions and processes in high-level arc magma chambers: an example from the Halfmoon Pluton, New Zealand. J Petrol. 51:1477–1505. doi: 10.1093/petrology/egq026
- White WH, Bookstrom AA, Kamilli RJ, Ganster MW, Smith RP, Ranta DE, Steininger RC. 1981. Character and origin of Climax-type molybdenum deposits. Economic Geology 75th Anniversary Volume: 270–316.
- Williams GJ. 1934. The genetic significance of some tin-tungsten lodes in Stewart Island, New Zealand. Econ Geol. 29:411–434. doi: 10.2113/gsecongeo.29.5.411
- Williams GJ. 1974. Economic geology of New Zealand. 2nd ed. Monograph Series 4. Parkville (VIC), Australia: Australasian Institute of Mining and Metallurgy.
- Windle SJ, Craw D. 1991. Gold mineralisation in a syn-tectonic granite dike, Sams Creek, northwest Nelson, New Zealand. New Zeal J Geol Geop. 34:429–440. doi: 10.1080/00288306.1991.9514481
- Wodzicki A. 1972. Mineralogy, geochemistry, and origin of hydrothermal alteration and sulphide mineralisation in the disseminated molybdenite and skarn-type copper sulphide deposit at Copperstain Creek, Takaka, New Zealand. New Zeal J Geol Geop. 15:599–631. doi: 10.1080/00288306.1972.10423987