ABSTRACT
The Birthday Reef was the most productive gold producer during historic mining of the Reefton goldfield on the west coast of the South Island of New Zealand. Deep exploration drill holes (up to 1.6 km long) intersected the mineralised quartz vein zone and adjacent hydrothermal alteration halo beneath the historic mine workings. The Paleozoic metasedimentary host rocks contain between 0.5 and 8 ppb Au and between 4 and 30 ppm As, and metamorphic pyrite typically contains 0.1 to 1 ppm Au in solid solution. The Au and As halo, above these background values, extends <20 m from the Birthday Reef, and other petrographic indicators of alteration are also confined to this narrow envelope. Porphyroblasts of pyrite and arsenopyrite, with minor Au enrichment, grew across the metamorphic cleavage in the alteration halo before emplacement of the Birthday Reef and associated shearing, and reflect an earlier, late metamorphic precursor to the main gold mineralisation phase.
Introduction
Most orogenic gold deposits are dominated by metre-scale quartz veins that constitute a small target for explorationists (Groves et al. Citation1998; Ramsay et al. Citation1998; Goldfarb et al. Citation2005). There is typically some alteration of the immediate wall rock to the veins, which can increase the width of the exploration target (Gao & Kwak Citation1997; Bierlein et al. Citation1998, Citation2000; Eilu & Groves Citation2001; Eilu et al. Citation2001; Goldfarb et al. Citation2005). However, the widths of these alteration zones vary as a result of the structural, lithological and geochemical processes involved in mineralisation (Bierlein et al. Citation1998, Citation2000; Eilu & Groves Citation2001; Eilu et al. Citation2001; Goldfarb et al. Citation2005; Craw et al. Citation2009). Hence, each individual deposit has its own characteristics, which hinders accurate prediction of the scale of exploration targets at the surface and in drill holes.
The Reefton goldfield of New Zealand () is a dismembered portion of the Lachlan Orogen of southeastern Australia, a well-endowed gold-bearing terrane with abundant orogenic vein deposits (Bierlein et al. Citation2004, Citation2006). Several attempts have been made to define the scale of wall rock alteration in Lachlan Orogen gold deposits (Gao & Kwak Citation1997; Bierlein et al. Citation1998, Citation2000; Dugdale et al. Citation2009; Thomas et al. Citation2011), but because each deposit is unique, the characteristic footprint features defined in one deposit are rarely extendable to other deposits. Similarly, within the Reefton goldfield itself, there is a wide range of styles of mineralisation and resultant alteration footprints, which range from only centimetres to several tens of metres from veins (Christie & Brathwaite Citation2003; Milham & Craw Citation2009; MacKenzie et al. Citation2016).
Figure 1. Cross section through the Birthday Reef (modified from MacKenzie et al. Citation2016), with an example of a deep drillhole trajectory to intersect the vein at depth. Vertical scale = horizontal scale. (Inset) Location of the Reefton goldfield that hosts the Birthday Reef.
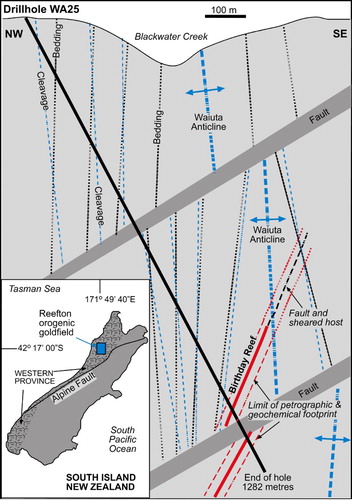
The Birthday Reef (; Cox Citation2000; Rattenbury & Stewart Citation2000; Christie et al. Citation2003), historically one of the richest gold-producers in the Reefton goldfield, apparently has an extremely narrow zone of adjacent alteration (metre scale), and this alteration zone is the focus of this study. The Birthday Reef is essentially a single quartz vein, typically c. 0.6 m wide, with a steep dip that was the target for deep historic underground mining. Modern exploration activity has required deep drilling beneath the historic workings, and drill holes up to 1600 m long have been emplaced as part of the deepest gold-related drilling programme in New Zealand (; MacKenzie et al. Citation2016). In this study, we used material from some of these deep drill holes to define the width of the alteration zone adjacent to the Birthday Reef and determine the hydrothermal features that constitute the footprint of the Birthday Reef mineralisation. We use low detection limit gold analyses to determine the extent of the gold enrichment halo in relation to background gold concentrations. We then integrate the gold halo with other geochemical and petrographic features of the alteration zone.
Table 1. Drill hole surface locations and averaged downhole directions, length of hole, and downhole depth of intercept of the Birthday Reef.
General geology
The Reefton goldfield lies on the western side of the South Island of New Zealand, and is hosted by Paleozoic metasediments of the Greenland Group in the Western Province (; Adams et al. Citation1975; Nathan et al. Citation2002). These host rocks in the Reefton goldfield consist of turbiditic greywackes and argillites that have been metamorphosed to lower greenschist facies, preserving bedding and some sedimentological features (Adams et al. Citation1975; Rattenbury and Stewart Citation2000; Nathan et al. Citation2002). A pervasive metamorphic cleavage cuts bedding at a range of angles associated with tight upright folding on the kilometre scale, with a north to northeast trend (; Gage Citation1948; Cox Citation2000; Rattenbury & Stewart Citation2000; Nathan et al. Citation2002). Granitoid intrusives were emplaced into the Greenland Group in the late Paleozoic and Cretaceous (Nathan et al. Citation2002). The Greenland Group in the Reefton goldfield was exhumed in the late Paleozoic, and is locally unconformably overlain by Devonian and early Cenozoic sediments (Nathan et al. Citation2002). Gold mineralisation in the Reefton goldfield was controlled by faults that locally cut across, but are partially related to, the upright to steeply inclined fold hinges (; Gage Citation1948; Cox Citation2000; Rattenbury & Stewart Citation2000).
The Birthday Reef strikes north–northeast, sub-parallel to the fold axial surfaces of the hosting folds and the bedding that defines those folds (Gage Citation1948; Cox Citation2000; Rattenbury and Stewart Citation2000). Known strike length is >1 km, and historic mining extended to >800 m below the surface. The historic mining extracted >700,000 ounces of gold (>22 tonnes), and typical gold grades were in excess of 15 g/tonne, before the mine closed in 1951 (Barry Citation1993). Recent exploration drilling has intersected the Birthday Reef a further 700 m below the last worked level of the mine, leading to an inferred resource estimate of c. 700,000 ounces of gold for this delineated portion of the reef.
The Birthday Reef consists almost entirely of multiple generations of quartz vein material, including some silicified breccias, that occurs in well-defined dilational zones within the hosting fault (A–D; Cox Citation2000; MacKenzie et al. Citation2016). Early-formed hydrothermal quartz has been variably recrystallised to form anhedral interlocking grains that have been crosscut by later quartz generations. Recrystallisation occurred during syn-mineralisation deformation, especially along narrow seams that irregularly cross the vein material. The hydrothermal quartz is accompanied by pyrite and arsenopyrite, and a range of other minor sulphide minerals (Christie & Brathwaite Citation2003; MacKenzie et al. Citation2016). Gold is commonly coarse grained and structurally controlled within the quartz, but also occurs as microparticulate grains within sulphide minerals in the vein zone (C–D). Abundant disseminated arsenopyrite and pyrite, with some associated gold, impregnate the immediate host rocks on the centimetre scale (A).
Figure 2. Photographs of the Birthday Reef mineralogy and textures. A, Hanging wall margin with disseminated arsenopyrite (scattered bright specks) in sheared argillite. B, Internal texture of quartz vein. C, Similar material to B, broken on a chloritic seam to show visible gold. D, Silicified breccia impregnated with arsenopyrite and pyrite.
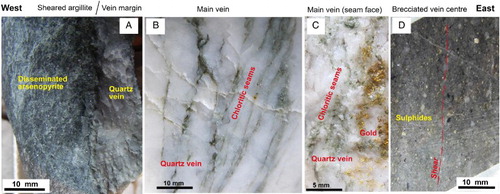
Methods
Samples (c. 100 g) were selected from fresh drill core in drillhole WA22C () at >600 m down the core, below the deepest level of oxidation (c. 200 m). Perpendicular distances from the Birthday Reef were calculated trigonometrically for these samples using drillhole trend and plunge (), and the inferred Birthday Reef strike and dip (). A suite of 25 samples of presumed unmineralised host argillites and greywackes was collected >200 m from the Birthday Reef to provide background data. Samples were taken systematically from host rocks adjacent to the Birthday Reef from several drill holes, and in a suite that extended from the vein itself to c. 200 m from the vein in drill holes WA22 and WA22C. In this latter suite, samples were taken every metre in the vicinity of the vein, and on both sides of the vein.
A representative set of samples from several of the drill holes in was prepared for light-optical and electron-optical petrography as polished thin sections. These were examined for textural and mineralogical features using standard incident and transmitted light microscopy. The selected samples were examined by scanning electron microscopy, augmented with energy-dispersive analytical systems, at University of Otago (Zeiss instrument) and Stockholm University (Quanta FEG650 instrument). Spot analyses were obtained at 15 or 20 kV with beam diameter between 3 and 10 µm.
Whole-rock analysis of samples from drillhole WA22C was carried out at Stockholm University using X-ray fluorescence spectrometry for major oxides and laser ablation inductively coupled plasma mass spectrometry (LA-ICPMS) for trace elements (Table S1). Both techniques were performed on fused glass discs produced with lithium metaborate flux in a mixture made of 66% lithium tetraborate and 34% lithium metaborate. The flux to sample proportion was 5 : 2 g. The beads were fused at 1100 °C for 10 min. The X-ray fluorescence spectrometry analyses were produced from a ZSX Rigaku Primus II X-ray fluorescence spectrometer that was regularly calibrated with international standard material AGV-2, BCR-2 and BGM-1 interspersed with analyses of unknowns (standard data are provided in Table S2). Major element results were obtained from the instrument as normalised anhydrous analyses. The LA-ICPMS analyses used a 193 nm Ar excimer laser coupled to a quadruple Thermo X-Series 2 mass spectrometer. The analytical settings were: beam size 150 μm, laser frequency and density respectively 10 Hz and 7.5 J/cm2, and a nitrogen flow rate of 3.0 ml/min. Accuracy and precision were assessed via external standard material NIST612a, and standard BCR-2 was analysed as known–unknown, with results matching published values within c. 10%. Estimates of volatile contents of rocks were determined as loss on ignition after heating at 105 and 550°C (Table S1).
Ultralow detection limit analyses were performed for Au via ICPMS using a Thermo X-Series 2 mass spectrometer at Stockholm University, following the method described in Pitcairn et al. (Citation2006a). Analytical precision and accuracy were controlled through analyses of CANMET reference materials TDB-1, WMS-1a and CH-4, with Au recovery ranging between 97 and 103% compared with certified values (Bédard and Barnes Citation2002; Constantin Citation2009). The 3σ method detection limit for Au analysis using acid-digested blanks is 0.02 ppb Au. The instrumental error is extremely small compared to the variability in the distribution of Au within each sample. For example, analyses of different aliquots of the same sample shows a variability of 20% (Pitcairn et al. Citation2006b), whereas repeat analysis of the sample digest on the ICP shows a variability of <1%. Analyses of As and Sb were performed using a PS Analytical Millennium Excalibur hydride generation atomic fluorescence spectrometer. CANMET reference materials TDB-1, WMS-1a and CH-4 were used for quality control with As and Sb recovery ranging between 88 and 111% compared to published values (Bédard and Barnes Citation2002; Dare et al. Citation2011). The 3σ method detection limits using acid digested blanks are 0.06 ppb As and 0.08 ppb Sb.
Trace element spot analyses for gold and arsenic contents of sulphide minerals in thin sections from drillhole WA22C were performed at the Department of Geology, University of Gothenburg. Analysis was performed using an Agilent 8800QQQ triple Quad ICPMS coupled with a New Wave NWR213 laser ablation system. The analytical conditions were: laser beam energy 5.5 J/cm2, spot size 30 and 10 μm for large and small crystals respectively, and a helium flow rate of 900 ml/min. Measured isotopes include 34S, 57Fe, 59Co, 63Cu, 66Zn 75As, 197Au and 208Pb. In the absence of a universal sulphide standard certified for all of the above elements, quantification was performed by using NBS SRM 610 glass (Jochum et al., Citation2011) as a primary standard for all elements except S, which was quantified using MASS-1 (Wilson et al., Citation2002). NBS SRM 610 is not certified for As, but performs well when analysing the certified glass BAM-S005 (measured 107 ppm; reference value 92 ppm; see Yang et al., Citation2012). For sulphides, concentrations for all elements were normalised to 100 wt%. Using silicate glass to analyse sulphides unavoidably compromises on accuracy, with different sulphide minerals displaying complex matrix effects due to fractionation during ablation-related melting effects. However, we monitored this matrix effect by analysing several sulphide standards for which some trace elements are well-determined: the pyrrhotite standard Po725 for Au (measured 45.2 ppm, certified value 44.5 ppm; Sylvester et al. Citation2005); the sphalerite standard MUL-ZnS-1 for As (measured 66 ppm, reported value 66 ppm; Onuk et al. Citation2016) and the Fe(Cu, Ni)S standard AI-3 for As (measured 370 ppm; reported 312 ppm) and Au (measured 11.4 ppm; reported 10.5 ppm; pers comm Dany Savard). Therefore, we can state a conservative accuracy of better than 20% for As and better than 10% for Au measurements in various sulphide minerals in this study. Some of the measurements returned mixed analyses as spots overlapped small inclusions, especially silicates.
Host rock petrography
Lithology and structure
General observations of the deep drill cores at hand specimen and thin section scales indicate that the hydrothermal effects of emplacement of the Birthday Reef on the host rocks are most apparent in the immediately adjacent metre of rock, and rapidly become less apparent farther from the vein. Some lithological and structural effects are visible out to c. 20 m from the vein, and >20 m the rocks show no sign of hydrothermal effects for the ensuing hundreds of metres. In this study, we have chosen a 20 m limit for the extent of this footprint based on petrographic observations (), and the following descriptions and geochemical characterisation are discussed in the context of this generalised cut-off.
Greywacke and argillite layers in unaltered rocks throughout the drill holes consist of combinations of the pervasive lower greenschist facies minerals quartz, albite, muscovite and chlorite. Quartz and subordinate albite dominate the greywacke layers, and mostly these minerals retain their primary clastic textures (A). These relict clasts occur in a matrix of recrystallised quartz, muscovite and chlorite. In contrast, argillites are largely recrystallised and are dominated by muscovite and chlorite, with few relict clasts (A–B). Relict detrital rutile, zircon, apatite, monazite and xenotime clasts are scattered through the metasediments, mainly in the greywacke layers. Some detrital rutile has been recrystallised to titanite. Epidote is rare and may be largely detrital. Minor calcite and/or ankerite form veinlets and localised cement in the metamorphic matrix. The recrystallised phyllosilicates are oriented to form a pervasive cleavage that is most apparent in the argillites (A–B). The cleavage is sub-parallel to bedding in all the drill holes, and commonly overprints and obscures the original lithological boundaries ( and A–B).
Figure 3. Thin-section views (plane polarised light) of unmineralised host rocks from drillhole WA22. A, Boundary between greywacke and argillite, with metamorphic cleavage (dashed) at a low angle to bedding (dotted), 1628 m depth. B, Box in A enlarged to show the cleavage wrapping around carbonate spots in argillite. C, Greywacke with cleavage overprinted by mineralisation-related shears, at 1634 m depth.
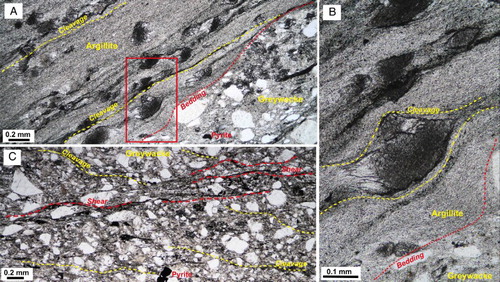
The bedding and cleavage are both overprinted locally by a spaced shear fabric that is subparallel to both these earlier surfaces (C). This shear fabric is best developed close to the Birthday Reef (A) and is clearly genetically related to the hosting fault structure. Early-formed hydrothermal quartz has been deformed by shears, whereas later vein quartz locally cuts across the shears. The shear fabric dominates the rock adjacent to the vein quartz (A), and both bedding and cleavage have been largely obliterated by this shear fabric within 10 cm of the vein. The shear fabric becomes less well developed, with spaced shear planes, over c. 20 m from the vein, and beyond c. 20 m the shear fabric is rare and widely spaced. The shear surfaces form dark seams that consist of finer grained rock-forming minerals that have been comminuted and locally recrystallised. This recrystallisation included carbonates and phyllosilicates, although many phyllosilicate grains have been broken, deformed and reoriented into parallism with the shears. Albite clasts have been partially or wholly replaced by fine-grained muscovite in sheared rock adjacent to the vein, giving the rock a paler bleached appearance, and this hydrothermal muscovite has been deformed by shearing into parallelism with the shear fabric. In addition, some detrital rutile clasts have been recrystallised to finer grained rutile in the shears (A).
Figure 4. Backscatter electron images (SEM) of textures in host rocks near to the Birthday Reef. A, Shear with variably oriented metamorphic phyllosilicates cuts obliquely across cleavage in greywacke. Detrital rutile (DR, light grey) has been recrystallized in the shear to finer grained hydrothermal rutile (HR). Drillhole WA22, 1641 m. B, Carbonate spot with relict ankerite in the core, now replaced by Mg-siderite (Mg-Sid), quartz (Q), and chlorite (Chl). Scattered white specks are sulphides: chalcopyrite, cobaltite and galena. Drillhole WA25, 1039 m. C, Carbonate spots in which ankerite has been fully replaced by Mg-siderite, quartz and chlorite. White dots are sulphides, as in B. Drillhole WA25, 1039 m. D, Carbonate spot in rock adjacent to the vein, almost entirely replaced by quartz, pyrite, with minor Mg-siderite, and chlorite. Drillhole WA22, 1625 m. E, Mineralisation related shear disrupts Mg-siderite spots, with hydrothermal chalcopyrite and cobaltite along the shear. Drillhole WA22, 1641 m.
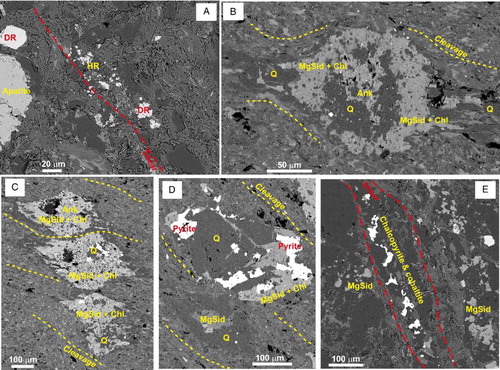
Carbonate spots
Most argillite layers contain carbonate spots (0.1–0.5 mm) throughout the drillhole rock sequence for the full length of all cores examined (A–B). The spots consist of coarsely crystalline ankerite, with some intergrown quartz, muscovite and chlorite. These spots are either diagenetic or metamorphic in origin, as they locally overgrew bedding boundaries, and therefore appear to be primary lithological features of the unmineralised host rocks distal from the Birthday Reef. These primary spots were subsequently deformed during metamorphism and cleavage development, and the cleavage anastomoses around the spots (A–B and 4B–C). Elongate quartz and chlorite intergrowths occur in pressure shadows formed as the cleavage deformed around the spots (A–B).
The textures and mineralogy of the carbonate spots change within c. 20 m of the Birthday Reef where there has been overprinting of the primary spot features by hydrothermal effects. Original ankerite is replaced by Mg-rich siderite and chlorite, with some relict ankerite patches locally preserved (B–C). Within 5 m of the Birthday Reef, the spots have been partially replaced by quartz as well as Mg-siderite and chlorite (D). The shear fabric has disrupted spots near the vein, and carbonate mineral recrystallisation associated with the shear fabric is more pronounced close to the vein. These transformations near the Birthday Reef have made the spots more readily visible in hand specimens in the immediate wall rocks.
Sulphides
Pyrite is ubiquitous throughout the drillhole rock sequence, as scattered irregular grains disseminated through the metamorphic rock. Pyrite occurs in both greywackes and argillites, although some argillite layers are particularly pyrite-rich (> 1%). Most of this pyrite has euhedral, subhedral or anhedral porphyroblastic textures that have overgrown bedding surfaces and clastic grains, and commonly have overgrown the pervasive cleavage (A–B,D). Pyrite is commonly accompanied by euhedral cobaltite porphyroblasts in the distal host rocks (B,D). Fine-grained (µm scale) chalcopyrite, sphalerite and galena commonly occur enclosed in pyrite or are scattered through the metamorphic matrix and within carbonate spots (B–C and A,C). Micron scale bismuth telluride is rarely associated with pyrite as well (B).
Figure 5. SEM backscatter images of sulphides in host rocks distant from the Birthday Reef in drillhole WA22, at indicated depths along core. A, Euhedral porphyroblastic metamorphic pyrite that has overgrown the cleavage; 1243 m. One porphyroblast has inclusions of galena (Gn, white specks). B, Anhedral pyrite with scattered euhedral cobaltite, and a single grain of Bi–Te mineral (white speck, top right); 996 m. C, Anhedral chalcopyrite aligned with the cleavage; 646 m. D, Euhedral pyrite and cobaltite porphyroblasts; 902 m.
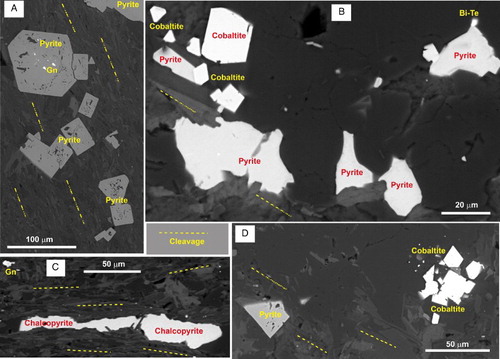
Porphyroblastic pyrite textures become more prominent near to (<20 m) the Birthday Reef where the cleavage anastomoses around some pyrite but the porphyroblasts clearly cut across that cleavage as well (A). Additional coarse-grained pyrite has become intergrown with carbonates, quartz and chlorite in carbonate spots that were recrystallised near the vein (D). Pyrite is joined by arsenopyrite porphyroblasts in this zone near to the Birthday Reef (B). Both pyrite and arsenopyrite porphyroblasts have undergone some deformation and rotation associated with development of the shear fabric, which developed after both pyrite and arsenopyrite porphyroblast growth. Pressure shadows filled with fibrous quartz are common where porphyroblasts have been rotated during this deformation (A–C). Growth of the fibrous quartz was accompanied by addition of narrow (1–10 µm) pyrite and arsenopyrite rims, forming visibly zoned sulphide grains (C–D). Cracks and cavities in the porphyroblasts are filled with other sulphide minerals, including chalcopyrite, sphalerite and galena (D–E). Minor cobaltite and/or gersdorffite have grown within the matrix and along shear surfaces within c. 20 m of the vein (E).
Figure 6. Sulphides in host rock near the Birthday Reef in drillhole WA22, at indicated depths along core. A, Polished thin-section view (crossed polars) of irregular pyrite porphyroblasts that have cleavage wrapping around them, but also locally truncate that cleavage. Pressure shadows are filled with fibrous quartz. Depth1634 m. B, Euhedral porphyroblastic arsenopyrite, with similar textures to pyrite in A. Depth 1641 m. C, Close view of the box in A, viewed with incident light as well, to show details of euhedral rims on the pyrite and the quartz fibres that grew with them. D, SEM backscatter image of an irregular pyrite porphyroblast with some euhedral rims. White specks are other sulphides, predominantly chalcopyrite (Cp), sphalerite (Sp) and galena (Gn), as in box enlarged in E. Depth 1641 m.
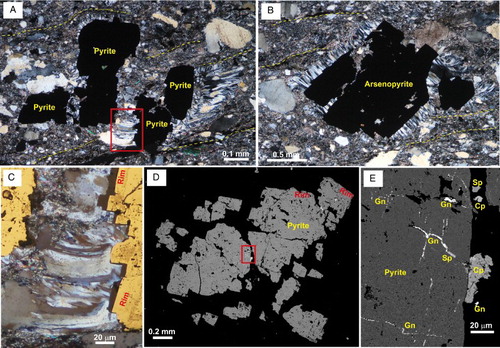
Gold, As and Sb analyses
Low detection limit Au analyses of unmineralised host rocks typically range from 1 to 3 ppb, with some argillites having Au up to 4 ppb (A–B). The sample set depicted in (A–B) includes some samples from >20 m from the vein, as well as the specifically selected unaltered samples from higher up the drillhole. There are negligible differences in Au content ranges of these distal samples, apart from one argillite at 175 m from the vein that has 8 ppb Au (A–B). The Au-enrichment signal of the Birthday Reef is well-defined immediately adjacent to the vein, but falls off rapidly over the first few metres from the vein on both sides (A). The Au contents of these proximal rocks are highly variable, but this halo of sporadic Au enrichment may extend between 10 and 20 m from the vein (A).
Figure 7. Arsenic and Sb contents of unmineralised and mineralised rocks in drillhole WA22C. A, B, Gold, As and Sb relationships in unmineralised host rocks distal from the Birthday Reef. C, D, Comparisons of bulk rock Al2O3, As and Sb relationships for rocks proximal and distal to the Birthday Reef.
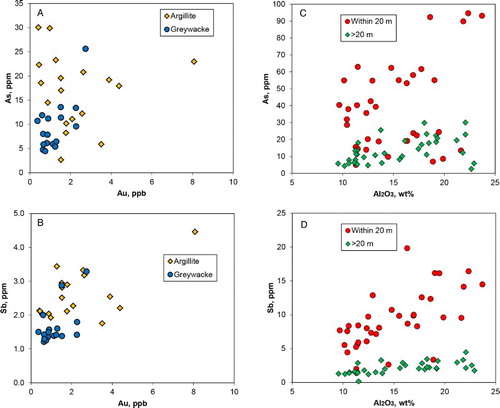
Figure 8. Low detection limit Au (A), and As and Sb (B) plotted with distance from the Birthday Reef (dashed line), to show the scale of the mineralisation-related haloes in drillhole WA22C.
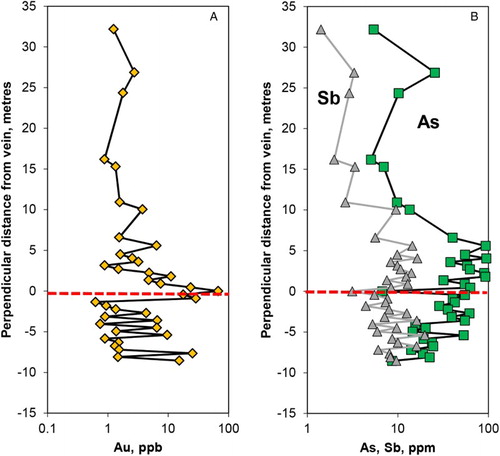
Background greywackes typically contain 5–15 ppm As and 1–2 ppm Sb, whereas some background argillites are distinctly enriched in As, up to 30 ppm (A–B). The two argillite samples with highest As contents are from 135 and 377 m from the vein, and hence do reflect primary background levels. These two samples are not relatively enriched in Sb or Au, however. There are clear distinctions between background As and Sb contents and those of rocks <20 m from the Birthday Reef, especially for Sb (C–D). In these latter plots, the Al2O3 contents are an imperfect proxy for mica content and therefore protolith lithology, with the more argillaceous rocks having higher alumina contents (A–D). In detail, definition of the extent of the hydrothermal As halo related to the Birthday Reef is difficult, but the most consistent zone of As enrichment falls off between 10 and 20 m from the vein, similar to that for Au (A–B). Likewise, the hydrothermal Sb enrichment halo also falls off between 10 and 20 m from the vein (B).
Solid solution gold in sulphides
Most metamorphic pyrites in host rocks distant from the Birthday Reef have Au contents of between 0.1 and 1 ppm (). There is a crude positive correlation between Au and As contents in these pyrites (). Pyrites in host rocks closer to the vein (<20 m) have similar Au and As contents to the more distal pyrites. Rims on these proximal pyrites (C–D) have similar Au and As contents to the interiors of the grains, although analysis of rims was hindered by their small sizes in relation to the analytical spot, and the presence of silicate inclusions. Hydrothermal pyrite grains in the Birthday Reef itself have similar Au contents to those of the host rocks, but their As contents are typically distinctly higher (). Pyrite Sb contents are highly variable, between 10 and 500 ppm, in all pyrites analysed.
Figure 9. Laser ablation inductively coupled plasma mass spectrometry analyses of spots on pyrite in situ in polished thin sections from the Birthday Reef, the immediate host rock, and distal unmineralised host rocks, in drillhole WA22C.
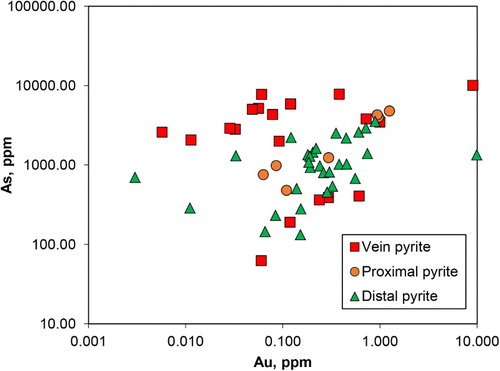
Sulphide minerals other than pyrite also contain variable amounts of Au (Tables S3a,b). Chalcopyrite in the host rocks has low Au contents, typically between 0.01 and 0.4 ppm, with most analysed grains near the lower end of this range. Sphalerite has <0.1 ppm Au. In contrast, cobaltite that commonly coexists with chalcopyrite (E) typically has c. 1 ppm Au and up to 4 ppm Au. Likewise, galena grains in both host rocks and vein typically contain c. 1 ppm Au and up to 5 ppm Au. Arsenopyrite in the Birthday Reef has up to 56 ppm Au, with most grains containing between 2 and 10 ppm Au. The anomalously high Au analyses in arsenopyrite may have arisen from overlap of the laser beam on an unseen gold microgranule.
Whole-rock compositions
Comparison of the major element compositions of host rocks near to the Birthday Reef with the unmineralised more distal rocks shows that, despite the various petrographic differences described above, the bulk compositions show little change (A–C; Table S1). There has been minor addition of quartz to carbonate spots and porphyroblasts near to the vein (D and 6A–C), but the bulk silica contents of these rocks are indistinguishable from background metasediments (A). The one high-silica sample analysed (A) is from the vein zone itself, where extensive silicification of breccias has occurred as well (D). Alteration of albite to muscovite near to the vein has caused distinct depletion of sodium from many of the proximal host rocks, but there has been only minor related change in bulk potassium contents, and no detectable change in aluminium contents (A–B). The most distinctive petrographic effects of mineralisation were the recrystallisation of ankeritic spots to Mg-siderite and chlorite, which involved redistribution of Fe and Mg, and Fe–Mg-bearing chlorite is a common hydrothermal mineral in the vein zone (B–C). However, the bulk iron and magnesium contents of the proximal host rocks do not show any changes associated with this redistribution (C). Localised CO2 variations near (c. 2 m) the Birthday Reef (Christie & Brathwaite Citation2003) may reflect hydrothermal alteration, but widespread and irregular carbonate veining throughout the drill holes limits the significance of this parameter.
Figure 10. Major element variations (anhydrous analyses) in host rocks near to the Birthday Reef compared to unmineralised distal rocks in drillhole WA22C.
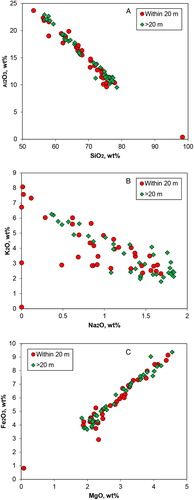
Trace element compositions (other than Au, As, Sb, above) also show little difference between proximal and distal rocks (A–D). There is some minor enrichment in Sr and Ba in some samples near to the vein (A), and some minor Pb enrichment reflecting the widespread galena in many proximal rocks (E and 11B). However, despite the widespread occurrences of sphalerite in these same rocks, there is no evidence for Zn enrichment of proximal rocks compared to more distal rocks (B). Similarly, there is no evidence for enrichment of Cu, despite the common occurrence of paragenetically late chalcopyrite in proximal rocks (E, 6E and 11C). There is minor enrichment of Mo in the main vein, but no Mo enrichment halo in proximal rocks (C). Likewise, there is minor Cr enrichment in the main vein sample, but no Cr enrichment halo in the host rocks (D). Nickel is not enriched in the host rocks, although there is rare millerite and pentlandite in the vein.
Discussion
Temporal paragenesis
Mineralisation in the Reefton goldfield clearly post-dates the regional metamorphism and upright folding that gave rise to the pervasive cleavage, but there are close relationships between fold geometry and vein-hosting faults that imply some genetic relationships as well (Cox Citation2000; Rattenbury & Stewart Citation2000). In addition, widespread occurrences of porphyroblastic arsenopyrite in host rocks across the goldfield attest to hydrothermal activity in the goldfield in the latter stages of greenschist facies metamorphism (A; MacKenzie et al. Citation2016). In contrast, many of the deposits across the Reefton goldfield have evidence for late-stage brittle deformation and relatively low-temperature Sb-rich mineralisation that overprints earlier higher-temperature mineralisation (A; Christie & Brathwaite Citation2003; Milham & Craw Citation2009; MacKenzie et al. Citation2016). The Birthday Reef paragenesis stands out in the goldfield as one of the least complex of the polyphase deposits and has little or no evidence for the late-stage brittle, Sb-rich, phase of mineralisation (A; Christie and Brathwaite Citation2003; MacKenzie et al. Citation2016).
Figure 12. Summary diagrams showing A, the temporal paragenesis of mineralised rocks in the Reefton goldfield (modified from MacKenzie et al. Citation2016), with particular reference to the Birthday Reef, and B, the spatial footprint of alteration adjacent to the Birthday Reef as deduced in this study.
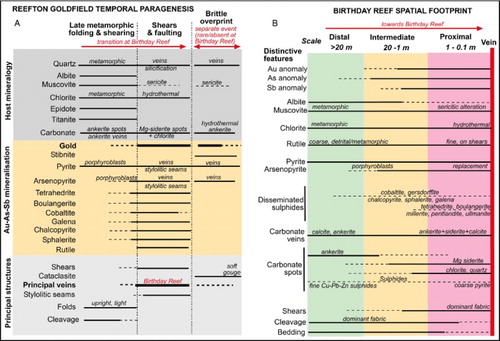
The shear fabric that has developed in the immediate host rocks adjacent to the Birthday Reef provides a useful structural and temporal marker for comparison of the relative timing of hydrothermal alteration features. This shear fabric developed parallel to, and in close relationship with, the fault zone that hosts the vein, and becomes progressively stronger towards the vein. The shear fabric is partly brittle in nature, but also involves some recrystallisation of hydrothermal quartz and host rock minerals ( and 6), and reflects some of the latest stages of metamorphic reconstitution of the rocks (A; MacKenzie et al. Citation2016).
Porphyroblasts of arsenopyrite and pyrite in the proximal host rocks to the Birthday Reef were initially formed before the development of the shear fabric, with some additional mineral growth during shear fabric evolution (). Hence, the hydrothermal processes that ultimately led to emplacement of the Birthday Reef were initiated under late metamorphic conditions before the formation of the main fault structure. These paragenetic relationships suggest that at least some of the As halo, and possibly the related Au and Sb haloes (), adjacent to the Birthday Reef are an earlier feature that predated emplacement of the vein-dominated Birthday Reef. If this speculation is correct, it is possible that the emplacement of the Birthday Reef vein system itself had little geochemical effect on the host rocks that were already weakly enriched in Au, As and Sb (A). The shear fabric, which is apparently closely associated with the hosting structure of the Birthday Reef, has also been superimposed on carbonate spots that were already recrystallised to Mg-siderite in the proximal host rocks (E). Hence, the mineralogical changes that occurred in carbonate spots in the proximal rocks may also reflect processes that occurred in those rocks before emplacement of the Birthday Reef vein system. These observations suggest that the structural zone that became the Birthday Reef had a history of weak and diffuse hydrothermal alteration accompanying late metamorphic deformation, before the fault developed as a dilational vein-hosting structure. Deformation along the fault was variably brittle and ductile during mineralisation, associated with development of the shear fabric. The rich gold-bearing quartz vein system was emplaced in that fault relatively late in the above paragenetic sequence (A). If the immediate wall rocks were already enriched in As, Sb and Au, there may have been little additional leakage of Au and As into these adjacent rocks during that late vein-forming mineralisation event. Conversely, in the same scenario, it is also possible that the hydrothermal fluids that emplaced the Birthday Reef leached some Au, As and Sb from the immediately adjacent and previously weakly mineralised wall rocks.
Spatial footprint
The combination of weak late metamorphic alteration (described above) and the well-defined Birthday Reef, has a spatial footprint of c. 20 m on either side of the vein (B). The footprint is defined primarily by variations in mineralogy and mineral textures in the proximal host rocks. The presence of the arsenopyrite porphyroblasts is one of the strongest indicators of the alteration halo, and the changes in mineralogy of the carbonate spots also occur in parallel with the emplacement of the porphyroblasts. The best geochemical signals for this footprint are moderate, as for Au, As and Sb (), or subtle, as for Na depletion and Pb enrichment (B and 11B). However, most geochemical components show little or no signal of the mineralisation that is distinguishable from the background host rocks.
The extent of the Au halo is difficult to resolve without low detection limit analyses, as presented herein ( and ). This Au halo is made more diffuse by the presence of Au in solid solution in distal metamorphic pyrite () and in cobaltite (B,D). Sulphide-rich distal host rocks, especially argillites, may have relatively elevated the background Au contents (>20 mg/kg) as a result, as well as localised As enrichment ( and A–B). This sulphide-hosted background Au may have been inherited from diagenetic enrichment in the primary sediments (Large et al. Citation2009, Citation2012). The combined Au and As compositions of the pyrites in Birthday Reef host rocks are broadly similar to those found in other metasedimentary belts that host orogenic gold deposits (Pitcairn et al. Citation2006b, Citation2010; Large et al. Citation2007, Citation2009, Citation2012; Thomas et al. Citation2011). Metamorphic mobilisation of Au and As from these background pyrite grains on a regional scale has been invoked as a source for the metals that were concentrated in orogenic vein systems (Pitcairn et al. Citation2006b, Citation2010; Large et al. Citation2007, Citation2009, Citation2012; Thomas et al. Citation2011). Hence, the rather nebulous distinction between the Au and As haloes adjacent to the Birthday Reef and the variably enriched As in background metasedimentary host rocks may reflect the close genetic links between these rocks and the metamorphic processes that drove the structurally controlled hydrothermal activity.
Conclusions
A mineralisation halo extends <20 m from the gold-bearing Birthday Reef quartz vein in the Reefton goldfield (B). Variable and minor gold enrichment has occurred in this zone (typically c. 10 ppb Au), along with anomalous As (up to 100 ppm) and Sb (up to 10 ppm). These Au, As and Sb values are only weakly enriched compared to distal host rock compositions. Most major element concentrations show negligible and inconsistent changes within the alteration halo, although some depletion of Na2O has occurred as a result of sericitisation of metamorphic albite. Minor enrichment of Sr, Ba and Pb has occurred in some rocks in the alteration halo, but most trace elements show no consistent changes during alteration.
The alteration halo is recognisable petrographically, but the changes are subtle (A–B). Diagenetic ankerite spots in some host rocks were altered to Mg-siderite in the halo, and further replacement of these spots by hydrothermal chlorite and quartz occurred close to the Birthday Reef. Pyrite porphyroblasts occur throughout the host rocks, and these become more common and have added pyrite rims within the alteration halo. Arsenic is hosted by fine-grained (micron scale) metamorphic cobaltite in the unaltered host rocks, and is hosted by coarser (up to mm scale) arsenopyrite porphyroblasts in the alteration halo. Pyrite porphyroblasts in unaltered and altered rocks contain c. 1 ppm Au and 1000 ppm As in solid solution, and pyrite in the Birthday Reef itself contains similar Au concentrations but up to 10,000 ppm As.
A distinctive ductile–brittle shear fabric has developed in the alteration zone, associated with the structural emplacement of the mineralised rocks that led to formation of the Birthday Reef. Deformation associated with this shear fabric has disrupted pyrite and arsenopyrite porphyroblasts in the alteration zone. Relative timing of mineral crystallisation and recrystallisation in relation to this structural marker (A) suggests that the alteration zone was emplaced before the main gold-bearing Birthday Reef quartz vein, in a complex late metamorphic paragenesis. It is possible that some of the rich Birthday Reef mineralisation resulted from localised remobilisation of Au, As and Sb from the previously weakly enriched alteration halo.
Table S1 XRF Analyses, XRF Standards, and Pyrite Analyses
Download MS Excel (52 KB)Acknowledgements
Funding for this study was provided by Stockholm University, NZ Ministry for Business Innovation and Employment, and University of Otago and JH gratefully acknowledges an MSc scholarship and support from The Swedish Institute. The project would not have been possible without extensive logistical support from Oceana Gold Ltd, in providing access to data and drill core. Discussions with Andrew Allibone, Tony Christie, Patti Durance, Phil Jones, Jonathan Moore, and James Scott were helpful in developing our ideas. TZ is grateful for sulphide standards provided by Peter Onuk (MUL-ZnS-1), Dany Savard (AI-3), Paul Sylvester (Po725) and Steve Wilson (MASS-1). Helpful reviews by Ross Large and Shaun Barker substantially improved the presentation of the manuscript and associated data. Associate editor: Dr Richard Wysoczanski.
Disclosure statement
No potential conflict of interest was reported by the authors.
Additional information
Funding
References
- Adams CJD, Harper CT, Laird MG. 1975. K-Ar ages of low grade metasediments of the Greenland and Waiuta Groups in Westland and Buller, New Zealand. New Zealand Journal of Geology and Geophysics. 18:39–48. doi: 10.1080/00288306.1975.10426345
- Barry JM. 1993. The history and mineral resources of the Reefton goldfield. Ministry of Commerce Resource Information Report 15. 59p.
- Bédard LP, Barnes S-J. 2002. A comparison of N-type semi-planar and coaxial INAA detectors for 33 geochemical reference samples. Journal of Radioanalytical and Nuclear Chemistry. 254:485–497. doi: 10.1023/A:1021685904319
- Bierlein FP, Arne DC, McKnight S, Lu J, Reeves S, Besanko J, Marek J, Cooke D. 2000. Wall-rock petrology and geochemistry in alteration halos associated with mesothermal gold mineralization, central Victoria, Australia. Economic Geology. 95:283–311. doi: 10.2113/gsecongeo.95.2.283
- Bierlein FP, Christie AB, Smith PK. 2004. A comparison of orogenic gold mineralisation in central Victoria (AUS), western South Island (NZ) and Nova Scotia (CAN): implications for variations in the endowment of Palaeozoic metamorphic terrains. Ore Geology Reviews. 25:125–168. doi: 10.1016/j.oregeorev.2003.09.002
- Bierlein FP, Fuller T, Stuwe K, Arne DC, Keays RR. 1998. Wallrock alteration associated with turbidite-hosted gold deposits. Examples from the Palaeozoic Lachlan fold belt in central Victoria, Australia. Ore Geology Reviews. 13:345–380. doi: 10.1016/S0169-1368(97)00026-7
- Bierlein FP, Groves DI, Goldfarb RJ, Dubé B. 2006. Lithospheric controls on the formation of provinces hosting giant orogenic gold deposits. Mineralium Deposita. 40:874–886. doi: 10.1007/s00126-005-0046-2
- Christie AB, Braithwaite RL. 2003. Hydrothermal alteration in metasedimentary rock-hosted orogenic gold deposits, Reefton goldfield, South Island, New Zealand. Mineralium Deposita. 38:87–107. doi: 10.1007/s00126-002-0280-9
- Constantin M. 2009. Trace element data for gold, iridium and silver in seventy geochemical reference materials. Geostandards and Geoanalytical Research. 33:115–132. doi: 10.1111/j.1751-908X.2008.00906.x
- Cox S. 2000. The geometry of mineralisation and exploration models for the Blackwater mine in the Reefton Goldfield. In: Proceedings of the 2000 New Zealand Minerals and Mining Conference, Wellington, Crown Minerals, Ministry of Economic Development, pp. 125–136.
- Craw D, Upton P, MacKenzie DJ. 2009. Hydrothermal alteration styles in ancient and modern orogenic gold deposits, New Zealand. New Zealand Journal of Geology and Geophysics. 52:11–26. doi: 10.1080/00288300909509874
- Dare SAS, Barnes SJ, Prichard HM, Fisher PC. 2011. Chalcophile and platinum-group element (PGE) concentrations in the sulfide minerals from the McCreedy East deposit, Sudbury, Canada, and the origin of PGE in pyrite. Mineralium Deposita. 46:381–407. doi: 10.1007/s00126-011-0336-9
- Dugdale AL, Wilson CJL, Leader LD, Robinson JA, Dugdale LJ. 2009. Carbonate spots: understanding the relationship to gold mineralization in central Victoria, southeastern Australia. Mineralium Deposita. 44:205–219. doi: 10.1007/s00126-008-0209-z
- Eilu P, Groves DI. 2001. Primary alteration and geochemical dispersion haloes of Archaean orogenic gold deposits in the Yilgarn Craton: the pre-weathering scenario. Geochemistry: Exploration, Environment, Analysis. 1:183–200.
- Eilu P, Mikucki EJ, Dugdale AL. 2001. Alteration zoning and primary geochemical dispersion at the Bronzewing lode-gold deposit, Western Australia. Mineralium Deposita. 36:13–31. doi: 10.1007/s001260050283
- Gage M. 1948. The geology of the Reefton quartz lodes. New Zeal Geol Survey Bull. 42:68.
- Gao ZL, Kwak TAP. 1997. The geochemistry of wall rock alteration in turbidite-hosted gold vein deposits, central Victoria, Australia. Journal of Geochemical Exploration. 59:259–274. doi: 10.1016/S0375-6742(96)00079-9
- Goldfarb RJ, Baker T, Dube B, Groves DI, Hart CJ, Gosselin P. 2005. Distribution, character and genesis of gold deposits in metamorphic terranes. In: Hedenquist JW, Thompson JFH, Goldfarb RJ, Richards JP, Editor. Econ Geol 100th Anniv Volume, 407–450.
- Groves DI, Goldfarb RJ, Gebre-Mariam M, Hagemann SG, Robert F. 1998. Orogenic gold deposits: a proposed classification in the context of their crustal distribution and relationship to other gold deposit types. Ore Geology Reviews. 13:7–27. doi: 10.1016/S0169-1368(97)00012-7
- Jochum KP, Weis U, Stoll B, Kuzmin D, Yang Q, Raczek I, Jacob D, Stracke A, Birbaum K, Frick DA, Gunther D, Enzweiler J. 2011. Determination of reference values for NIST SRM 610-617 glasses following ISO guidelines. Geostandards and Geoanalytical Research. 35:397–429. doi: 10.1111/j.1751-908X.2011.00120.x
- Large RR, Maslennikov VV, Robert F, Danyushevshy LV, Chang Z. 2007. Multistage sedimentary and metamorphic origin of pyrite and gold in the giant Sukhoi Log deposit, Lena Gold Province, Russia. Economic Geology. 102:1233–1267. doi: 10.2113/gsecongeo.102.7.1233
- Large R, Thomas H, Craw D, Henne A, Henderson S. 2012. Diagenetic pyrite as a source for metals in orogenic gold deposits, Otago Schist, New Zealand. New Zealand Journal of Geology and Geophysics. 55:137–149. doi: 10.1080/00288306.2012.682282
- Large RR, Danyushevsky L, Hollit C, Maslennikov V, Meffre S, Gilbert S, Bull S, Scott R, Emsbo P, Thomas H, Singh R, Foster J. 2009. Gold and trace element zonation in pyrite using a laser imaging technique: Implications for the timing of gold in orogenic and Carlin-style sediment-hosted deposits. Economic Geology. 104:635–668. doi: 10.2113/gsecongeo.104.5.635
- MacKenzie D, Craw D, Blakemore H. 2016. Paragenesis of orogenic mineralisation in the Reefton goldfield, Westland. In: Christie AB, editor. Mineral deposits of New Zealand – Exploration and Research, Australasian Institute of Mining and Metallurgy: Melbourne, Monograph 31 (in press).
- Milham L, Craw D. 2009. Two-stage structural development of a Paleozoic auriferous shear zone at the Globe-Progress deposit, Reefton, New Zealand. New Zealand Journal of Geology and Geophysics. 52:247–259. doi: 10.1080/00288300909509889
- Nathan S, Rattenbury MS, Suggate RP. 2002. Geology of the Greymouth area. Institute of Geological and Nuclear Sciences 1:250 000 Geological Map 12.
- Onuk P, Melcher F, Mertz-Kraus R, Gäbler HE, Goldmann S. 2016. Development of a matrix-matched sphalerite reference material (MUL-ZnS-1) for calibration of in situ trace element measurements by Laser Ablation-Inductively Coupled Plasma-Mass Spectrometry. Geostandards and Geoanalytical Research. doi:10.1111/ggr.12154.
- Pitcairn IK, Olivo GR, Teagle DAH, Craw D. 2010. Sulfide evolution during prograde metamorphism of the Otago and Alpine Schists, New Zealand. The Canadian Mineralogist. 48:1267–1295. doi: 10.3749/canmin.48.5.1267
- Pitcairn IK, Teagle DAH, Craw D, Olivo GR, Kerrich R, Brewer TS. 2006b. Sources of metals and fluids in orogenic gold deposits: insights from the Otago and Alpine Schists, New Zealand. Economic Geology. 101:1525–1546. doi: 10.2113/gsecongeo.101.8.1525
- Pitcairn IK, Warwick PE, Milton JA, Teagle DAH. 2006a. Method for ultra-low-level analysis of gold in rocks. Analytical Chemistry. 78:1290–1295. doi: 10.1021/ac051861z
- Ramsay WRH, Bierlein FP, Arne DA, VandenBerg AHM. 1998. Turbidite-hosted gold deposits of central Victoria, Australia: their regional setting, mineralising styles, and some genetic constraints. Ore Geology Reviews. 13:131–151. doi: 10.1016/S0169-1368(97)00016-4
- Rattenbury MS, Stewart M. 2000. Structural setting of the Globe-Progress and Blackwater gold mines, Reefton goldfield, New Zealand. New Zealand Journal of Geology and Geophysics. 43:435–445. doi: 10.1080/00288306.2000.9514900
- Sylvester PJ, Cabri LJ, Tubrett MN, McMahon G, Laflamme JHG, Peregoedova A. 2005. Synthesis and evaluation of a fused pyrrhotite standard reference material for platinum group element and gold analysis by laser ablation-ICPMS. 10th International Platinum Symposium, Oulu, Geol Surv Finland, Extend Abstr, pp. 16–20.
- Thomas HV, Large RR, Bull SW, Maskennekov VV, Berry BF, Fraser R, Froud S, Moye R. 2011. Pyrite and pyrrhotite textures and composition in sediments, laminated Quartz veins, and reefs at Bendigo gold mine, Australia: insights for ore genesis. Economic Geology. 106:1–31. doi: 10.2113/econgeo.106.1.1
- Wilson SA, Ridley WI, Koenig AE. 2002. Development of sulfide calibration standards for the laser ablation inductively-coupled plasma mass spectrometry technique. J. Anal. At. Spectrom.. 17:406–409. doi: 10.1039/B108787H
- Yang QC, Jochum KP, Stoll B, Weis U, Kuzmin D, Wiedenbeck M, Traub H, Andreae MO. 2012. BAM-S005 Type A and B: new silicate reference glasses for microanalysis. Geostandards Geoanalytical Res. 36:3010313. doi: 10.1111/j.1751-908X.2012.00171.x