ABSTRACT
Blue Spur Conglomerate is a Cretaceous paleoplacer gold deposit that yielded abundant gold in the 19th century Otago gold rush. A modern gold mine near Waitahuna in southeast Otago has provided new insights into the nature of the conglomerate and a window into some technical, logistical and environmental challenges that faced the historical miners. Most of the clasts in the conglomerate were eroded from fresh basement along the Tuapeka Fault Zone, with derivation from the north or northeast. There are three principal gold morphological groups in the conglomerate: angular nuggety gold (up to 15 mm), commonly intergrown with quartz; rounded ellipsoidal particles (10–500 µm); and extensively flattened and folded flakes (10–500 µm). Most of that gold was derived from nearby basement sources in the Otago Schist. Diagenetic alteration of the conglomerate and subsequent weathering has resulted in enhanced clay contents and friability of the conglomerate clasts, which contributed to elevated suspended sediment loads in mine-processing waters.
Introduction
The Otago placer goldfield of southern New Zealand (A,B) has been a significant gold producer (> 8 million ounces) since the first major gold discovery in 1861 (Williams Citation1974). That first discovery sparked one of the major 19th century gold rushes, initiated by Gabriel Read who found placer gold in the active bed of the Tuapeka River near what is now called Gabriels Gully (A). The immediate source for that gold was traced back to a nearby Cretaceous paleoplacer deposit, the Blue Spur Conglomerate (C,D; McKay Citation1897; Williams Citation1974; Els et al. Citation2003). The Blue Spur Conglomerate in Gabriels Gully became the focus of sporadic mining activity over the following century, eventually resulting in removal of much of the deposit (D). Other remnants of Blue Spur Conglomerate occur in the general vicinity, and those deposits were all mined to some extent as well (Williams Citation1974; Els et al. Citation2003; Barnett Citation2016).
Figure 1. Geological setting for Blue Spur Conglomerate. A, Location map for the Otago placer goldfield on Otago Schist basement, and the Tuapeka Fault Zone (from Bishop & Turnbull Citation1996). B, Location of Otago Schist belt in southern New Zealand. C, Stratigraphic setting for the Blue Spur Conglomerate. D, Geology of the Gabriels Gully historical gold mine in the conglomerate (bedding indicated with dashed lines). E, Location of Waitahuna Gully ().
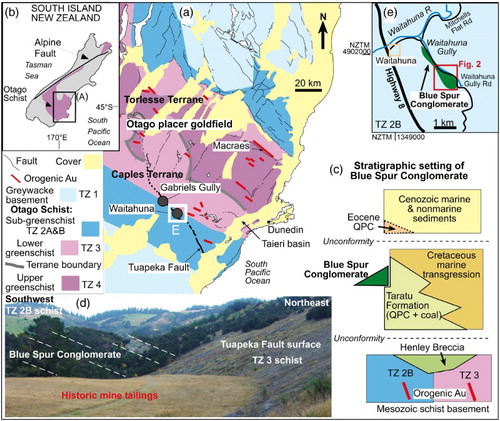
Despite the historical and economic significance of the Blue Spur Conglomerate paleoplacers, there is surprisingly little published information on their geological and mineralogical nature. In particular, there is almost no published information on the nature of the gold within the paleoplacers. Here, we present new information on the morphology of the paleoplacer gold in the Blue Spur Conglomerate. We describe the lithology and mineralogy of the hosting sediments and the heavy mineral suite that accompanies the detrital gold. This complements a previous sedimentological study (Els et al. Citation2003), and a study of diagenesis and cementation of the conglomerate with associated gold mobilisation (Kerr et al. Citation2017).
This study takes advantage of the development of a new mine in the Blue Spur Conglomerate at Waitahuna (A, ). Development of this mine has provided fresh outcrops of the conglomerate, and allowed examination of the nature of the sedimentary rock as it has been disaggregated during processing. The processing system has also provided access to gold and heavy mineral concentrates that have never been described before. In addition, the modern mine has provided a unique window through which to view the extensive past mining activities, and the various technical, logistical and environmental challenges that faced the historical miners during the early gold rush period in Otago.
General setting
The Mesozoic metasedimentary Otago Schist belt forms the basement for the Blue Spur Conglomerate (A,C; Bishop & Turnbull Citation1996; Turnbull Citation2000). The schist basement and the flanking lower grade metagreywackes were exhumed in the Cretaceous, exposing a range of metamorphic grades with varying textural reconstitution in two principal terranes, the Caples and Torlesse terranes (A; Turnbull Citation2000; Turnbull et al. Citation2001). Two generations of orogenic gold mineralisation formed a regionally extensive set of mineralised structures in the Cretaceous, including the Hyde–Macraes Shear Zone that hosts the active Macraes mine (A; Mortensen et al. Citation2010).
Regional extension in the middle and Late Cretaceous formed a set of northeast- and northwest-striking normal faults across the schist belt (Bishop & Turnbull Citation1996; Deckert et al. Citation2002; Mortensen et al. Citation2010). Erosion along the active normal fault zones formed nonmarine deposits of lithic basement-derived debris in the immediate vicinity of the fault scarps (Bishop et al. Citation1976; Els et al. Citation2003; Craw Citation2010). The oldest of these units in southeast Otago is early Late Cretaceous Henley Breccia (C; Bishop & Turnbull Citation1996). This unit is unconformably overlain by the Late Cretaceous nonmarine Taratu Formation, which is dominated by mature rounded quartz pebble conglomerates and associated finer-grained sediments including lignite (Harrington Citation1958; Bishop & Turnbull Citation1996). However, the Taratu Formation also includes some localised lithic deposits formed adjacent to active fault scarps (Harrington Citation1958).
The Tuapeka Fault Zone (A) in southeast Otago is a prominent member of the set of normal faults formed during regional Cretaceous extensional tectonics. The Blue Spur Conglomerate, which is the topic of this study, is a lithic deposit that formed along the Tuapeka Fault (A,C; Harrington Citation1958; Bishop & Turnbull Citation1996; Els et al. Citation2003). The conglomerate was included in the Cretaceous Taratu Formation by Harrington (Citation1958), and mapped as a separate late Cretaceous unit by Bishop and Turnbull (Citation1996). The remaining deposits of the Blue Spur Conglomerate rest unconformably on Caples Terrane schist basement near to the boundary between textural zone (TZ) 2B pumpellyite–actinolite facies and TZ 3 lower greenschist facies (A,C,E). The conglomerate now occurs as small (< 1 km2) erosional remnants along the fault zone and the associated stratigraphic succession has been largely eroded. Regional reconstruction of the structure and stratigraphy suggests that the conglomerate was unconformably overlain by Eocene quartz pebble conglomerates, remnants of which occur nearby (C; Bishop & Turnbull Citation1996). The Late Cretaceous and early Cenozoic nonmarine sedimentary succession was overlain by marine transgressive sediments that continued through to the Oligocene (C; Bishop & Turnbull Citation1996; Landis et al. Citation2008). Most of these sediments have been removed by erosion during late Cenozoic uplift associated with regional deformation related to the Alpine Fault plate boundary (A,B; Bishop & Turnbull Citation1996; Youngson et al. Citation2006; Landis et al. Citation2008).
Methods
The modern mining operation at Waitahuna has provided access and exposures for field mapping for this study (), and this mapping was done in the context of recently published historical records of the original mining activity in the area (Barnett Citation2016). Fresh mine excavation outcrops have provided new and changing views of the internal structure and lithology of the coarse-grained fraction of the Blue Spur Conglomerate (). Paleocurrent directions were determined from imbricated elongate and tabular schist, and greywacke clasts in only three mine outcrops where suitable exposures had been created. These data were left uncorrected for the later rotation of the strata, which is only minor (< 5°; ), and are therefore still comparable with data in Els et al. (Citation2003).
Figure 2. Geological map of the Blue Spur Conglomerate (BSC) at Waitahuna Gully gold mining area near Waitahuna (A,E) with the location of the modern mine. Cross sections show the underlying structure and inferred topography before historic mining.
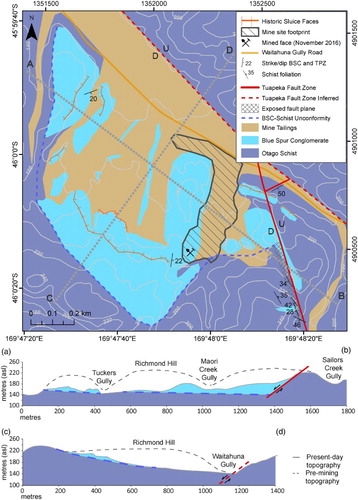
Table 1. Summary of the principal rock fragments, detrital minerals, and cementing minerals in the Blue Spur Conglomerate.
The mine sampling and processing systems have provided water-washed samples of medium-grained sediment fractions and heavy mineral concentrates, including gold (). The water management system at the mine has provided access to mine and background waters, and the suspended load from mine waters provided samples of the fine-grained fraction of the sediments ().
Samples from the Blue Spur Conglomerate were examined by standard light microscopic methods in polished thin sections, grain separates and grain mounts in epoxy resin. Representative hand-picked gold particles were mounted in epoxy resin blocks and polished for examination in incident light and scanning electron microscopy (SEM) with a Zeiss Sigma VP SEM and its energy dispersion analytical attachment (University of Otago Centre for Electron Microscopy). The operating voltage was 15 or 30 kV. Clay mineral textures and compositions were determined on carbon-coated polished thin sections with the same SEM, using element-mapping software. Detrital heavy minerals were identified with semiquantitative analysis of a polished grain mount via automated scanning of the whole section followed by off-line interpretation of element maps and spot compositions using AZTEC software (Oxford Instruments).
Water samples were obtained from six accessible points in the water drainage system through the mine and the Waitahuna Gully catchment. Suspended sediments were left to settle for 2 weeks before extraction of cloudy water subsamples by pipette from the base and the lower 4 cm in the waters with high sediment contents. Supernatant waters were then analysed for major ions by Hill-Laboratories (Hamilton, New Zealand) using standard methods. Dried suspended sediment samples were analysed for their mineral content by X-ray diffraction (CuKα radiation) in the Geology Department, University of Otago. A full diffractogram was obtained to identify the principal silicate minerals present, and an enhanced short diffractogram (3 to 20° 2θ) was obtained to identify the principal clay minerals. These latter diffractograms were repeated after overnight glycolation, although no significant clay layer expansion was detectable. The resultant clay mineral data was compared with more extensive previous work on clay minerals in similar sediments reported by Craw (Citation1984, Citation1994), Craw et al. (Citation1995) and Chamberlain et al. (Citation1999).
Blue Spur Conglomerate at Waitahuna
The modern mine was established in an erosional remnant of Blue Spur Conglomerate on schist basement in Waitahuna Gully, near the township of Waitahuna (A, ). The mine was developed in patches of the conglomerate that were left behind after extensive historical mining in the area (; Barnett Citation2016). The basement unconformity dips gently northeastwards (< 5°) from near a ridge crest into the base of Waitahuna Gully (). The unconformity is approximately planar on the kilometre-scale of the erosional remnants (), although some larger scale channelisation has been defined (Els et al. Citation2003). The northeastern and eastern sides of the conglomerate deposit are truncated by strands of the Tuapeka Fault Zone (, ). These fault strands have moderate dips (c. 35°) and normal senses of motion (, A). The original topographic surface, prior to historical mining, has been estimated from historical accounts and photographs of the area (Barnett Citation2016), and field-based projections from remnants of that original surface ().
The conglomerate is dominated by poorly sorted lithic boulders, cobbles, pebbles and granules (; ; ). Most of the lithic clasts are angular or subangular, and consist of lower greenschist facies and sub-greenschist facies (TZ 3 and 2B) Caples Terrane metasedimentary schist that have been derived from the nearby basement. Rare clasts of green pumpellyite-rich metavolcanic schist occur also (E). There is also a substantial component (locally > 30%) of rounded and subrounded Caples Terrane greywacke clasts (, ) in most outcrops, although some beds, especially near the base of the conglomerate, are almost entirely schist derived (A). Rounded chemically mature quartz pebbles are a common minor constituent of most outcrops, and these form > 20% in some rare thin beds.
Figure 3. Schist basement and Blue Spur Conglomerate at outcrops in the modern mining area at Waitahuna Gully. A, Outcrop of a strand of the Tuapeka Fault Zone in schist basement, with dark gouge zones. B–D, Conglomerate with crude bedding (partially indicated with dashed line) defined by clast size differences. E, Coarse clasts in conglomerate showing differential oxidation.
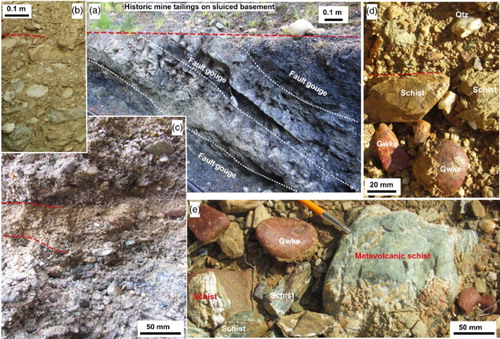
Figure 4. Blue Spur Conglomerate outcrop features in the modern Waitahuna Gully mine. A, Unoxidised conglomerate near the base of the unit. B, Partially oxidised conglomerate. C, Strongly weathered conglomerate near to the original topographic surface prior to historic mining. D–F, Rose diagrams showing inferred paleocurrent directions (mainly towards south and southwest) for the conglomerate, based on clast orientations and stacking, near the base of the unit.
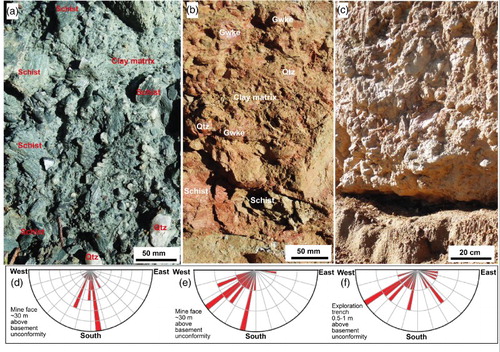
Bedding is poorly defined in most outcrops, and stratification is crude and diffuse at the 1–10 m scale through most of the unit. The bedding is mainly apparent from subtle changes in clast sizes and variations in proportions of greywacke and quartz pebbles (, ). However, finer scale bedding does occur locally (C). Schist-rich beds, especially near the base of the conglomerate, have some imbrication of clasts, and quantification of imbrication orientations in three outcrops shows that the depositional paleocurrents were directed towards the south and southwest (D–F). This confirms previous work by Els et al. (Citation2003).
The depositional unconformity between the Blue Spur Conglomerate and the underlying schist was an important feature for historical mining, and this surface was exhumed in several places and is now covered in historical tailings (). Exposures created during modern mining show varying degrees of tilting, shearing and post-depositional clay alteration. The schist basement is still hard and largely unaltered in many places (cf. A), whereas other exposures show almost complete transformation of basement and overlying lithic clasts to clays with the constituency of soft cheese (A–E). This soft unconformity has been sheared and weakly disrupted by post-depositional deformation. Only quartz clasts have survived the clay alteration (B–E), although many of these are readily fragmented on exposure.
Figure 5. Features of unconformity between Blue Spur Conglomerate and schist basement (white dashed lines in A–C). A, Mine face through the unconformity, with bedding in conglomerate indicated by red dashed lines. Vertical marks are from excavation machinery. B, Outcrop of unconformity, with a large hydrothermal quartz clasts (pyrite veins are arrowed). C, Contrasting oxidation (oxidised = brown; unoxidised = grey–green) at the unconformity. D, Thin section through unconformity (sample OU85595) showing shear disruption. E, Sediment immediately above the unconformity (sample OU85596), with green smectite–vermiculite in schist clasts. F, Gold from conglomerate immediately above the unconformity.
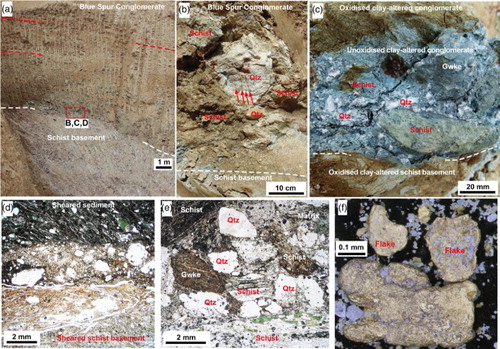
Oxidation of the conglomerate
Unoxidised conglomerate has a blue–green colour that reflects the abundant ferrous iron-bearing silicates in clasts and matrix (A, C–E). This blue–green colour is responsible for the name of the unit, which was coined by the historical miners at Gabriels Gully (D). Some of the ferrous iron-bearing silicates are original metamorphic minerals: chlorite, phengitic muscovite, pumpellyite and stilpnomelane. However, most of these phyllosilicates have been variably altered to blue–green ferrous iron-bearing clay minerals (Craw et al. Citation1995; Kerr et al. Citation2017). These minerals have been variably oxidised by interaction with shallow groundwater, and the oxidation has had strong effects on the appearance and physical properties of outcrops of the conglomerate. Oxidation has also further obscured the already subtle primary sedimentary features of the conglomerate (A–C).
Oxidation of the ferrous iron by groundwater has yielded abundant staining of the rocks by red and brown ferric iron oxyhydroxides (B–E, B,C). Pyrite in hydrothermal veins (B), schist clasts and matrix has been variably oxidised along with the phyllosilicates. In deeper parts of the conglomerate, the oxidation staining is superficial on the outsides of many cobbles, many of which have unoxidised interiors (E). At these deeper levels, green clasts without labile ferrous iron-bearing phyllosilicates, such as pumpellyite-rich metavolcanic rocks, remain unoxidised and unstained (E). The staining is progressively more pervasive through the clasts and matrix towards the pre-mining paleosurface (B,D,E). The amount of oxidation was also controlled by differential permeability of the bedding, because some relatively matrix-poor beds hosted enhanced groundwater flow and associated oxidation, leading to brown beds sandwiched between less-oxidised horizons and vice versa (C, C–E). Conglomerate close to that paleosurface is essentially completely oxidised, with extensive additional clay alteration that imparts paler colouring, and this material is highly friable in parts (C).
Conglomerate matrix and heavy minerals
Most of the conglomerate is clast-supported, but some matrix-supported beds occur as well. The distinctions between clasts and matrix have been obscured by post-depositional alteration, cementation and oxidation of the conglomerate in many places. Medium and fine-grained material washed and concentrated by mine activity give a cleaner, albeit biased, view of the mineralogy and textures of the matrix (A–D). Most matrix consists of sand, silt and mud clasts derived from the same schist, greywacke and mature quartz sources as the coarser clasts (; A,B). Matrix mineralogy is dominated by sand- and silt-sized clasts of angular or subangular quartz and albite, with a range of accessory silicate minerals, and apatite and pyrite, liberated from schist and greywacke sources ().
Detrital phyllosilicate minerals have retained their primary metamorphic shapes and textures, but have been largely replaced by authigenic clay minerals (; D,E). This replacement has occurred at the submicron scale, so that the delicate interlayering of different metamorphic phyllosilicates has survived, and original foliation orientation of the phyllosilicates is preserved (D,E). Despite the extensive chemical and internal structural changes that have occurred within individual phyllosilicate grains, the chemical distinctions between the different minerals has been retained. Hence, phengitic muscovite has transformed to illite–smectite, and chlorite and/or stilpnomelane have transformed to smectite–vermiculite (Kerr et al. Citation2017). These interlayered clay minerals have a broad compositional range with progressive alteration towards kaolinite (Craw et al. Citation1995; Kerr et al. Citation2017). Minor fine-grained detrital kaolinite in the matrix of the Blue Spur Conglomerate () occurs intermixed with these authigenic clay minerals derived from detrital phyllosilicates.
Detrital heavy minerals are scattered through the matrix of the conglomerate, with some concentrations at the bases of discrete beds, especially where these have scoured and channelised into underlying beds. The heavy mineral suite is dominated by metal oxides (), which form black sand accumulations locally, and are readily concentrated in the mine-processing system (A,C,D). The most prominent of these metal oxides is Fe oxyhydroxide that has relict cubic shapes pseudomorphous after pyrite (C). Some of these cubes have sharp edges and corners, but most have some rounding and many are almost spherical (C). Well-rounded hematite clasts dominate the coarser grained fraction of matrix concentrates (A). Ilmenite is also a common constituent of the black sand concentrates (D). Magnetite is relatively minor, and occurs as well-formed octahedral crystals, some of which have sharp corners and edges, and some of which are more rounded (D). Garnets are rare and well rounded (; D), as is chromite.
Figure 6. Lithologies and mineralogy of medium-grained components of the Blue Spur Conglomerate, as observed in water-washed material at the Waitahuna Gully Mine. A, Partial heavy mineral concentrate in medium-grained matrix material. Rounded dark clasts are predominantly hematite. B, Close view of similar material to a, showing the silicate matrix material. C, Heavy mineral concentrate with abundant variably rounded Fe oxyhydroxide clasts that are pseudomorphous after pyrite (Pp). D, Heavy mineral concentrate with abundant grey ilmenite (Ilm) and an almandine garnet (pink, centre), and variably rounded euhedral magnetite (Mt).
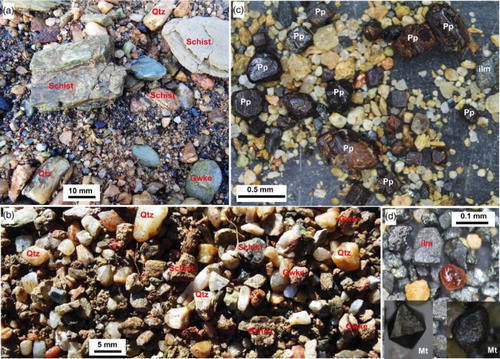
Detrital gold
The detrital gold extracted from the Blue Spur Conglomerate at the Waitahuna Gully mine has a wide range of particle sizes and textures, from centimetre-scale angular nuggets to micron-scale thin flakes (; F, A–D, A–J). The larger nuggets are rare, and most gold particles that are saved by the mine-processing system are 50–500 µm across. Nuggets and some finer particles have irregular angular shapes with some delicate protrusions and complex surface depressions (A, A). Many of these angular particles are intergrown with angular white quartz (B, B). Some surface protrusions reflect internal and external crystalline structures (C,D) similar to those found on coarse nuggets elsewhere in the Otago goldfield (Craw & Lilly Citation2016; Hesson et al. Citation2016).
Figure 7. Coarse nuggety gold from the Blue Spur Conglomerate at Waitahuna Gully mine. A, Large angular nugget. B, Closer view of opposite side of nugget in a, showing quartz intergrowth. C,D, Weakly rounded particles with relict coarse gold crystal shapes on surfaces.
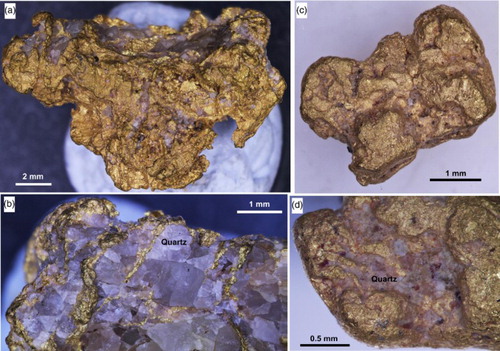
Figure 8. Range of typical gold particle sizes and morphologies in Blue Spur Conglomerate at Waitahuna Gully mine. A,B, Angular particles. C,D, Rounded ellipsoidal particles with authigenic clay in surface depressions, and a flake with Mn oxyhydroxide coating in C. E, Incident light image of a polished section through a flake with folded rim. Paler relict core zones (dotted lines) have higher Ag contents than rim zones. F–H, Folded flakes, with a quartz clast embedded in flake in G. I,J, Close views of rounded ellipsoidal particles with authigenic clay overgrowths. Particle in J has been incipiently amalgamated with mercury during the mine concentration process.
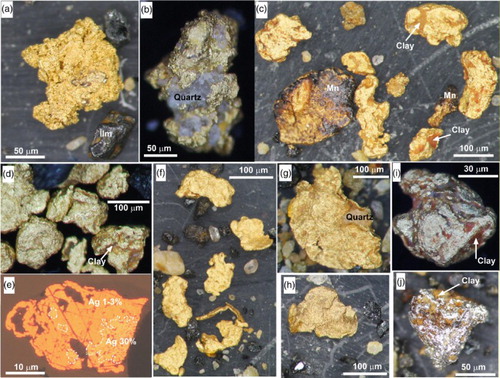
Most of the gold particles have been rounded to some extent and many of the particles are spheroidal or ellipsoidal with relict surficial depressions (F, A–J). A similar proportion of particles are flattened flakes with rounded edges (F, C,E–H). Some flakes have folded edges that have been reflattened (E–H). Particles with shapes intermediate between rounded spheroids and flattened flakes do occur, but the majority of the gold resembles one of these end members rather than intermediates. All gold particles have had some recrystallisation of gold grains on their rims, at the 1–50 µm scale, and this recrystallised gold has low Ag contents (1–3 wt%; Kerr et al. Citation2017). Cores of the gold particles typically have 3–10 wt% Ag (Kerr et al. Citation2017). However, one particle has a distinctively paler coloured core in reflected light, with c. 30% Ag (E).
Cementation
The Blue Spur Conglomerate has been extensively lithified by diagenetic processes, so that it forms erosion-resistant cliffs. The principal cementing minerals are calcite and authigenic clays (). Calcite is widespread through the matrix of unoxidised conglomerate, forming cementing coatings on the surfaces of clasts and some microveinlets that fill fractures within clasts. Calcite is locally intergrown with authigenic clay minerals that have formed from direct replacement of detrital phyllosilicates (D,E) with relatively minor dissolution and reprecipitation of these clays in interstices of the matrix. In addition, authigenic pyrite is widespread as a cementing mineral (A). The authigenic pyrite is generally fine grained and is commonly euhedral (A), but scattered fine anhedral pyrite occurs intergrown with calcite and authigenic clays as well. Marcasite and vivianite occur as additional cementing minerals, but these are minor and typically micron-scale components.
Figure 9. SEM backscatter images of authigenic minerals in the Blue Spur Conglomerate at Waitahuna Gully mine. A, Euhedral authigenic pyrite has overgrown silicate clasts and clay-altered phyllosilicates. B, Partially rounded ellipsoidal gold particle with authigenic clay coatings and intergrowth. C, Close view of crystalline gold on the surface of the particle in B, with authigenic clay overgrowths (electron-translucent, on right). D, Intergrown authigenic clay and authigenic gold on the surface of gold particle in B.
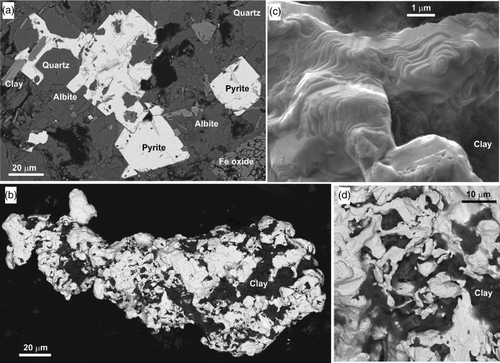
Black Mn oxide or oxyhydroxide is a widespread, but volumetrically minor cementing mineral that occurs with authigenic clays and commonly coats gold particles (C), lithic clast fractures and quartz clasts. Oxidation of the conglomerate has caused dissolution of calcite, and calcite is relatively rare in the most oxidised portions (B,C). Instead, Fe oxyhydroxide is an important component of the cement in these rocks, and this forms discrete coatings on clasts (D,E, B,C) or is intimately intergrown with variably oxidised clay minerals. Oxidation of the authigenic ferrous iron-bearing clay minerals has yielded kaolinite and a variety of smectites that further contribute to the cementation (C).
Authigenic clay minerals of the conglomerate cement fill cavities in the exterior surfaces of detrital gold particles (C,D,I,J, B,C). These clays are locally intergrown with micron-scale authigenic gold overgrowths on detrital gold particle surfaces (D). Iron oxyhydroxide is intergrown with some of this surficial authigenic clay in oxidised parts of the conglomerate (C,I,J).
Mine waters and suspended sediments
Heavy mineral concentrates, including gold, have been produced at the Waitahuna Gully mine using traditional water washing over metal riffles (A,B). The high proportion of fine-grained matrix and cement in the Blue Spur Conglomerate results in high suspended sediment loads in the processing waters. In addition, cementation has limited the disaggregation of excavated conglomerate despite abundant tumbling in the processing plant (C). The suspended sediment from the processing system settled from waters in a series of ponds downstream of the processing plant (A,B,D). Individual ponds rapidly filled with sediment, on a time scale of days to weeks, and new ponds were utilised in the pond series while filled ponds were emptied and the extracted sediment added to tailings (D). Nevertheless, the suspended sediment load had essentially all been settled by the end of the pond series and water was generally suitable for re-use or environmental discharge (A).
Figure 10. Sediments in mine-processing waters. A, Map of the Waitahuna Gully mine site in early 2016, showing water pathways around the site and the series of suspended sediment settling ponds. Numbered sites refer to water analyses in . B, High suspended sediment load in water departing the initial concentration system in the mine. C, Coarse tailings cobble of Au-bearing conglomerate that did not disaggregate during processing. D, Sediment, and water draining from that sediment, after excavation from a filled settling pond.
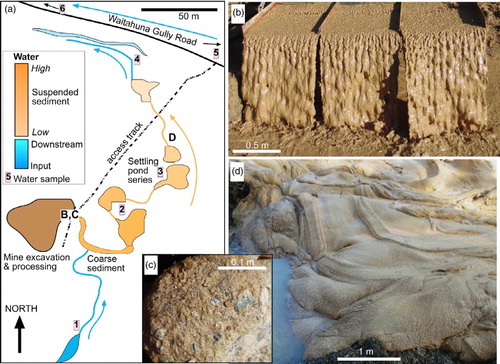
The suspended sediments were made up of the fine-grained fraction of the conglomerate (silt and mud) that consisted of quartz, albite and clay minerals: kaolinite, smectite–vermiculite and illite–smectite (). The clay content of the suspended sediments was enhanced by partial disaggregation during mine processing of lithic clasts that had been clay-altered in situ during conglomerate diagenesis (A–C, A–E). This clast disaggregation also released fine-grained quartz and albite grains that were originally intergrown with the metamorphic phyllosilicates, in addition to the fine-grained clasts in the matrix.
Six water samples were collected on a single day, to compare dissolved loads in the active mine waters to background waters and downstream discharge water (A, A–D, ). Background (upstream) waters have low total dissolved solid (TDS) contents (< 80 mg/L; ). These TDS contents are typical of surface waters on schist basement, and lower than shallow groundwater in schist-derived fluvial gravels (Litchfield et al. Citation2002). Analyses of waters from the mine settling pond series show that the dissolved load was similar to background levels in two samples, and highest in water with the least suspended sediments at the end of the pond series (sample 4; A, A–D; ).
Table 2. Major ion analyses of waters from the modern Waitahuna Gully mine area (, ) collected on 26 July 2016. Background waters were unaffected by mining, discharge waters were on the mine site, and the downstream sample was taken from Waitahuna Gully stream near the confluence with Waitahuna River (E).
The dissolved loads in the background and mine waters have Ca2+ and with molar ration of 1 : 2 (A), which results from dissolution of calcite as is typical for waters that have interacted with basement schist containing metamorphic carbonate (Litchfield et al. Citation2002; Jacobson et al. Citation2003). The presence of additional cementing calcite in the less-oxidised parts of the Blue Spur Conglomerate provides additional access for dissolution. This calcite dissolution maintains the mine water pH near to neutral, or slightly alkaline conditions (B; ). Slightly elevated dissolved sulphate in some mine waters results from dissolution of metamorphic and authigenic pyrite in the Blue Spur Conglomerate (A, B; , ). In addition, some of the sulphate, and most of the dissolved chloride contents, arise from input from marine aerosols in rain (C; Litchfield et al. Citation2002; Jacobson et al. Citation2003). There has been negligible dissolution by mine waters of the abundant albite in the suspended sediments, so the waters are not enriched in dissolved sodium in the same way as schist-hosted groundwaters typically are (C; Litchfield et al. Citation2002). Likewise, there has been only minor dissolution of Mg and K from the abundant suspended clay minerals in the mine waters (D).
Figure 11. Geochemical plots of water analyses from the Waitahuna Gully mine area (), with the analysis having the highest dissolved load (4) highlighted. Data are compared to a field of 50 schist-hosted groundwaters from the Taieri basin (A; Litchfield et al. Citation2002). A, Ca2+ vs . B, Water pH vs
. C, Na+ vs Cl−. D, Mg2+ vs K+.
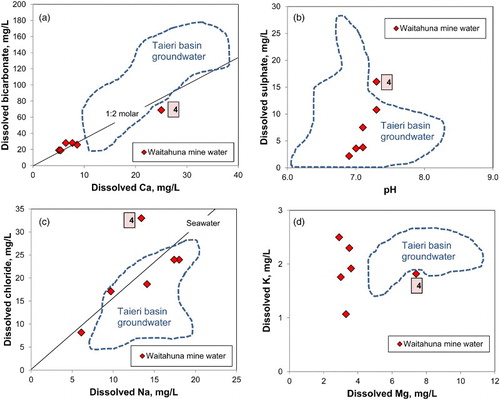
Discussion
Sediment provenances
Lithic clasts and liberated matrix minerals () show that most of the Blue Spur Conglomerate was derived from nearby schist basement near to the Tuapeka Fault Zone (A). Our observations support those of Els et al. (Citation2003), that the sediment was mainly derived by erosion of freshly exposed unweathered schist basement on the nearby active fault scarp. This debris was derived from the north or northeast (D–F) and was transported by steep streams that crossed the fault zone at a high angle to its strike (Els et al. Citation2003). These streams were incised with channel widths on a scale of kilometres, but the geometry of this channelisation is not resolvable at the scale of the small remnant of Blue Spur Conglomerate at the Waitahuna Gully mine (; Els et al. Citation2003).
Mature rounded quartz pebbles are a common, but subordinate component of the conglomerate (B,C, B). This quartz detritus forms much of the coeval Taratu Formation in southeast Otago (C; Harrington Citation1958; Craw Citation2010). The quartz pebbles have been largely derived from erosion and recycling of older sediments and clay-altered schist basement in the higher grade schists of inland Otago (Youngson et al. Citation2006; Craw Citation2010), and this is compatible with the observed fluvial current directions of Blue Spur Conglomerate (D–F; Els et al. Citation2003). By contrast, the abundant Caples Terrane greywacke clasts in the Blue Spur Conglomerate were ultimately derived from the south or southwest of the Tuapeka Fault Zone (A). This implies that the greywacke clasts were initially transported to the northeast and then recycled from an older sedimentary deposit on the Otago Schist basement that lay to the north or northeast of the Tuapeka Fault Zone (A; Els et al. Citation2003).
Most of the heavy mineral suite reflects the dominant derivation from Otago Schist basement sources (). In particular, hematite, epidote, pumpellyite, titanite, zircon and actinolite are characteristic accessory minerals of the immediately underlying schist basement in the Waitahuna area (Brown Citation1967; Kerr et al. Citation2017). Some euhedral and unrounded Fe oxyhydroxide pseudomorphs after pyrite (C) were probably oxidised in situ in the Blue Spur Conglomerate, either in basement clasts or in the diagenetic cement (cf. A). Euhedral magnetite crystals (D) are common constituents of metavolcanic rocks in the higher grade (upper greenschist facies) parts of the schist belt. Spessartine garnets occur sporadically throughout the schist belt from pumpellyite–actinolite (TZ 2b) to upper greenschist facies (TZ 4), and Mn-rich almandines occur in upper greenschist facies (Brown Citation1967).
Some constituents of the heavy mineral suite are exotic to the Otago Schist belt. The abundant ilmenite is the most prominent of these exotic minerals, and the rare chromite, almandine and grossular are also apparently exotic to the Tuapeka Fault area. It is possible that these exotic minerals were originally detrital mineral grains in the Caples Terrane greywacke. Detrital ilmenite, in particular, is present in many of the greywacke clasts albeit in small proportions, and is the dominant oxide mineral in the Caples Terrane outcrops to the southwest (Becker Citation1973; Pitcairn et al. Citation2010). These minerals have been recycled into the Blue Spur Conglomerate after liberation during erosion and transport, or perhaps were liberated from clay-altered greywacke clasts during mine processing. An alternative source for these exotic minerals may have been crystalline rocks to the south or west of the Otago Schist belt. Recycling of minerals such as these into gold placer deposits is common elsewhere in the southern South Island area, where longshore drift in marginal marine sediments has been followed by uplift and erosion into fluvial or beach sediments (Craw et al. Citation2015a, Citation2015b). There is currently no evidence for a Cretaceous marine sediment source older than the Blue Spur Conglomerate in the Waitahuna area, but it is possible that an unrecorded inland marine incursion occurred during the general marine transgression in the Late Cretaceous (C).
Sources of gold
The cores of gold particles probably represent the remnants of the original gold eroded from basement sources, and the rims reflect subsequent changes, including recrystallization in situ within the Blue Spur Conglomerate (Kerr et al. Citation2017). The cores of most of the gold particles have Ag contents between 3 and 10 wt%, which is typical of gold in the Otago placer goldfield and underlying basement sources (Youngson & Craw Citation1993; MacKenzie & Craw Citation2005; Craw et al. Citation2015c; Craw & Lilly Citation2016). Hence, Otago Schist orogenic deposits are the most probable sources for the gold in the Blue Spur Conglomerate. One gold particle, with 30% Ag (E), may be from an exotic source with the other exotic heavy minerals (e.g. ilmenite, chromite, garnets).
Despite the uniformity of compositions of the original gold, there is a wide range of particle shapes and degrees of physical modification, indicative of differing amounts of transport in the sedimentary environment (Knight et al. Citation1999; Youngson & Craw Citation1999; Craw et al. Citation2017). There are three principal morphological groups of gold particles: angular and subrounded nuggety gold; spheroidal and ellipsoidal particles; and thin folded flakes (, ). The nuggety gold commonly has attached and/or intergrown angular quartz (, ) and is almost certainly locally derived from orogenic veins, either known or unknown, in the immediate Waitahuna area, presumably to the north or northeast of the Waitahuna Gully mine (A; Williams Citation1974). The spheroidal and ellipsoidal gold particles have apparently travelled farther than the nuggety gold, but have not undergone the extremes of hammering and flattening that accompanies long-distance transport on a scale of tens or hundreds of kilometres (Youngson & Craw Citation1999).
Numerous euhedral and only weakly rounded detrital Fe oxyhydroxide pseudomorphs after pyrite in the heavy mineral suite (; C) may have come from gold-bearing vein systems located near to, or moderately distant from, Waitahuna Gully. Some of these pseudomorphs contain micron-scale gold inclusions (Kerr et al. Citation2017). The pseudomorphs were formed from pyrite prior to sedimentary transport, suggesting that the source orogenic vein systems had undergone oxidation in situ before erosion. This oxidised setting was different from, and probably more distant than, the unoxidised schist outcrops at the Tuapeka Fault scarp that shed most of the schist debris to form the conglomerate. The oxidised orogenic gold deposits may have been part of a supergene enrichment zone near the regional schist basement unconformity, as described by Craw et al. (Citation2015c) and Craw and Lilly (Citation2016).
The gold flakes have clearly undergone either long-distance (> tens of kilometres) travel, repeated recycling from older sediments, or both, and these strongly contrast with the more equant and less rounded locally derived gold (). The flaky gold is typical of that found in many of the younger quartz pebble conglomerate deposits of the Otago placer goldfield that have undergone repeated recycling (Youngson & Craw Citation1993; Youngson et al. Citation2006; Craw Citation2010). Hence, recycling of the flaky gold from pre-existing Cretaceous quartz pebble conglomerate deposits into the Blue Spur Conglomerate, along with the quartz pebbles, is a likely origin for that portion of the gold. The ultimate origin of that gold is unknown but presumably lay in the same general region as the quartz pebbles, in the core of the Otago Schist belt.
Implications for modern and historical mining
Historical mining at Waitahuna Gully in the late 19th and early 20th centuries yielded > 100,000 ounces of gold (Barnett Citation2016). Historical mining of the conglomerate was hindered by low gold contents, which can be as low as 100 mg/m3 in parts of the deposit (A. Roberts, Maruia Mining Ltd, pers. comm. 2016). Mining was mainly done by hydraulic sluicing, and this method encountered difficulties in disaggregating the cemented conglomerate (e.g. C; Barnett Citation2016). However, well-cemented parts of the conglomerate facilitated some underground tunnel development of richer portions of the conglomerate during the historical mining period (Barnett Citation2016), thereby avoiding handling large volumes of low grade material in surrounding beds. Substantial tailings accumulations developed at the historical mines in the low topographic gradient of the valley () and this was an additional hindrance to on-going operations (Barnett Citation2016). All these issues are inherent in the lithology, mineralogy and topographic setting of the Blue Spur Conglomerate along the Tuapeka Fault, including the original mine site at Gabriels Gully (D).
The abundant fine-grained constituents of the Blue Spur Conglomerate led to high suspended sediment contents in mine-processing waters. Elevated suspended sediments in water discharges have been a significant environmental issue in the Otago placer goldfield since the 1860s (Druzbicka & Craw Citation2013). However, most such suspended sediment was derived from the matrices of young, commonly Pleistocene, gravels with relatively low clay contents. In contrast, Blue Spur Conglomerate contains abundant clays that formed primarily during post-depositional alteration and diagenesis, followed by variable amounts of oxidation and further clay formation during weathering (A–C). Clay alteration of lithic clasts has also facilitated the liberation of fine-grained silicate minerals in addition to those already in the matrix. These fine-grained components () are readily mobilised during mine processing (A,B,D). Management of discharges of processing waters with such high suspended solid contents has been a major part of the modern mining operation (A,B,D). Historical miners in the Otago placer goldfield made few attempts at controlling the suspended sediments in their discharge waters, and those working in the Blue Spur Conglomerate must have been among the worst offenders because of the unique mineralogical nature of the conglomerate. This aspect of historical mining of the Blue Spur Conglomerate is no longer visible because the clay-laden mine waters were ephemeral, and the tailings have since become vegetated. However, high sediment loads in streams must have constituted a large component of the environmental footprint for the local and even distant inhabitants.
The high suspended sediment contents of processing waters increase the density of those waters. Fine gold particles, especially flaky gold, can float on the surface tension of any water, but this flotation effect can be enhanced by high-density sediment-rich slurries (Youngson & Adamson Citation2001). Hence, processing of Blue Spur Conglomerate inevitably led to some inefficiencies in gold extraction and loss of the finest gold particles, especially those < 50 µm diameter. These inefficiencies were enhanced by the common presence of attached silicate minerals, such as quartz and clay minerals, that decreased the net densities of the particles (B–D). The presence of authigenic mineral coatings, such as Fe and Mn oxyhydroxides and clay minerals (C,I,J) also inhibited mercury amalgamation of gold in final extraction from heavy mineral concentrates, a common process historically and in some modern mines. For example, the gold particle in J had sufficient gold exposed through a clay coating to receive some mercury during amalgamation, but insufficient surface area for full amalgamation to occur.
Conclusions
The Cretaceous Blue Spur Conglomerate near Waitahuna in southeast Otago was mainly derived from low-grade Otago Schist (pumpellyite–actinolite to lower greenschist facies) that forms the basement in the immediate area of the Tuapeka Fault Zone. The conglomerate was deposited by a steep stream flowing towards the south or southwest across the active fault scarp, and eroded basement sources were largely unweathered. The conglomerate has a high proportion of lithic clasts from the nearby basement, ranging in size from boulders to mud. The medium- and fine-grained clastic components of the conglomerate, including matrix, are dominated by quartz and albite with abundant accessory minerals from the schist basement as well.
There are three principal gold morphological groups in the conglomerate: angular nuggety gold (up to 15 mm), which is commonly intergrown with quartz; rounded ellipsoidal particles (10–500 µm); and extensively flattened and folded flakes (10–500 µm). The nuggety gold was probably derived from nearby orogenic sources in the schist basement to the north and/or northeast, whereas the ellipsoidal gold particles have probably been transported further from inland Otago. Abundant euhedral Fe oxyhydroxide pseudomorphs after pyrite occur in the conglomerate with the gold. These pseudomorphs have been variably rounded by sedimentary transport, and some contain minor gold inclusions. At least some of these pseudomorphs were probably derived from the same nearby hydrothermal vein sources as some of the proximal gold. The pseudomorphs were oxidised prior to sedimentary transport, possibly within a supergene zone developed on the orogenic deposit source(s).
The conglomerate also contains some exotic clasts, which are principally greywacke derived, ultimately from the southwest of the Tuapeka Fault Zone, and quartz pebbles ultimately derived from higher grade (upper greenschist facies) schist in inland Otago. Exotic heavy minerals that may be derived from these sources include ilmenite, garnets, magnetite and chromite. These minerals have almost certainly been recycled from older sediments. The flaky gold may have a similar history of recycling, and may have been transported from inland Otago with the quartz pebbles.
Diagenetic alteration and cementation of the conglomerate has resulted in strong clay-dominated alteration. The alteration, and the friability imposed on lithic clasts because of that alteration, results in formation of abundant fine-grained material in addition to the original clastic matrix when the conglomerate is mined and processed for the contained gold. Water-based processing of the conglomerate yields discharge waters with high suspended solid loads and elevated dissolved loads. Modern mining of this material requires a long series of settling ponds with regular maintenance as the ponds quickly fill. These issues undoubtedly had major environmental impacts during historical mining. Authigenic minerals, especially clays, that coat the parts of the gold particles in the conglomerate have caused inefficiencies during concentration processes in mines because of lowered composite particle density and a lack of availability of gold surface area for mercury amalgamation during the final concentration stages.
Acknowledgements
We appreciate the interest and enthusiasm from Alan Roberts who generously provided access to the Waitahuna Gully mine site and samples of mine material including gold. Barry MacDonell and John Youngson contributed useful discussions on geological aspects of the Blue Spur Conglomerate. Ross Barnett compiled helpful historical information on Waitahuna Gully. SEM data were obtained at the Otago Centre for Electron Microscopy, with skilful oversight from Kat Lilly. Brent Pooley and Luke Easterbrook-Clarke gave excellent technical support. Helpful comments from Senior Editor Nick Mortimer improved the presentation of the manuscript. Associate editor: Dr Nick Mortimer.
Additional information
Funding
References
- Barnett R. 2016. Gold entrepreneurs: the Norwegian Party at Waitahuna Gully, Otago. Lawrence, New Zealand: Lawrence Athenaeum and Mining Institute. 216 pp.
- Becker N. 1973. A preliminary study of the Tuapeka Group Rocks, lower Clutha valley, Otago, New Zealand [Unpublished BScHons dissertation]. University of Otago Library. 73 pp.
- Bishop DG, Laird MG, Mildenhall DC. 1976. Stratigraphy and depositional environment of the Kyeburn Formation (Cretaceous), a wedge of coarse terrestrial sediments in Central Otago. Journal of the Royal Society of New Zealand. 6:55–71. doi: 10.1080/03036758.1976.10421466
- Bishop DG, Turnbull IM. 1996. Geology of the Dunedin area. GNS Science 1:250 000 Geological Map, Lower Hutt, New Zealand. Sheet 21 + 52 pp.
- Brown EH. 1967. The greenschist facies in part of eastern Otago, New Zealand. Contributions to Mineralogy and Petrology. 14:259–292. doi: 10.1007/BF00373808
- Chamberlain CP, Poage MA, Craw D, Reynolds RC. 1999. Topographic development of the Southern Alps recorded by the isotopic composition of authigenic clay minerals, South Island, New Zealand. Chemical Geology. 155:279–294. doi: 10.1016/S0009-2541(98)00165-X
- Craw D. 1984. Ferrous-iron-bearing vermiculite-smectite series formed during alteration of chlorite to kaolinite, Otago Schist, New Zealand. Clay Minerals. 19:509–520. doi: 10.1180/claymin.1984.019.4.01
- Craw D. 1994. Contrasting alteration mineralogy at an unconformity beneath auriferous terrestrial sediments, central Otago, New Zealand. Sedimentary Geology. 92:17–30. doi: 10.1016/0037-0738(94)90052-3
- Craw D. 2010. Delayed accumulation of placers during exhumation of orogenic gold in southern New Zealand. Ore Geology Reviews. 37:224–235. doi: 10.1016/j.oregeorev.2010.03.006
- Craw D, Hesson M, Kerr G. 2017. Morphological evolution of gold nuggets in proximal sedimentary environments, southern New Zealand. Ore Geology Reviews. 80:784–799. doi: 10.1016/j.oregeorev.2016.08.028
- Craw D, Kerr G, Falconer D. 2015a. Mineralogy and provenance of auriferous Waimumu Quartz Gravels, Southland, New Zealand. New Zealand Journal of Geology and Geophysics. 58:281–295. doi: 10.1080/00288306.2015.1070182
- Craw D, Kerr G, Reith F, Falconer D. 2015b. Pleistocene paleodrainage and placer gold redistribution, western Southland, New Zealand. New Zealand Journal of Geology and Geophysics. 58:137–153. doi: 10.1080/00288306.2015.1007462
- Craw D, Lilly K. 2016. Gold nugget morphology and geochemical environments of nugget formation, southern New Zealand. Ore Geology Reviews. 79:301–315. doi: 10.1016/j.oregeorev.2016.06.001
- Craw D, MacKenzie DJ, Grieve P. 2015c. Supergene gold mobility in orogenic gold deposits, Otago Schist, New Zealand. New Zealand Journal of Geology and Geophysics. 58:123–136. doi: 10.1080/00288306.2014.997746
- Craw D, Smith DW, Youngson JH. 1995. Formation of authigenic Fe2+-bearing smectite-vermiculite during terrestrial diagenesis, southern New Zealand. New Zealand Journal of Geology and Geophysics. 38:151–158. doi: 10.1080/00288306.1995.9514647
- Deckert H, Ring U, Mortimer N. 2002. Tectonic significance of Cretaceous bivergent extensional shear zones in the Torlesse accretionary wedge, central Otago Schist, New Zealand. New Zealand Journal of Geology and Geophysics. 45:537–547. doi: 10.1080/00288306.2002.9514990
- Druzbicka J, Craw D. 2013. Turbidity development and dissipation in paleoplacer gold deposits, southern New Zealand. Environmental Earth Science. 68:1575–1589. doi: 10.1007/s12665-012-1851-4
- Els BG, Youngson JH, Craw D. 2003. Blue Spur Conglomerate: auriferous Late Cretaceous fluvial channel deposits adjacent to normal fault scarps, southeast Otago, New Zealand. New Zealand Journal of Geology and Geophysics. 46:123–139. doi: 10.1080/00288306.2003.9515000
- Harrington HJ. 1958. Geology of the Kaitangata Coalfield. New Zealand Geological Survey Bulletin. 59: 131 pp.
- Hesson M, Stewart J, Stephens S, Kerr G, Craw D. 2016. Gold nuggets in proximal placers, Old Man Range, Central Otago. In: Christie AB, editor, Mineral deposits of New Zealand: exploration and research. Carlton, Australia: Australasian Institute of Mining and Metallurgy Monograph. 31: 359–366.
- Jacobson AD, Blum JD, Chamberlain CP, Craw D, Koons PO. 2003. Climatic and tectonic controls on chemical weathering in the New Zealand Southern Alps. Geochimica et Cosmochimica Acta. 67:29–46. doi: 10.1016/S0016-7037(02)01053-0
- Kerr G, Malloch K, Lilly K, Craw D. 2017. Diagenetic alteration of a Mesozoic fluvial gold placer deposit, southern New Zealand. Ore Geology Reviews. 83:14–29. doi: 10.1016/j.oregeorev.2016.12.018
- Knight JB, Morison SR, Mortensen JK. 1999. The relationship between placer gold particle shape, rimming, and distance of fluvial transport as exemplified by gold from the Klondike district, Yukon Territory, Canada. Economic Geology. 94:635–648. doi: 10.2113/gsecongeo.94.5.635
- Landis CA, Campbell HJ, Begg JG, Mildenhall DC, Paterson AM, Trewick SA. 2008. The Waipounamu erosion surface: Questioning the antiquity of the New Zealand land surface and terrestrial fauna and flora. Geological Magazine. 145:173–197. doi: 10.1017/S0016756807004268
- Litchfield NJ, Craw D, Koons PO, Edge B, Perraudin E, Peake BM. 2002. Geology and geochemistry of groundwater within the Taieri Basin, east Otago, New Zealand. New Zealand Journal of Geology and Geophysics. 45:481–497. doi: 10.1080/00288306.2002.9514987
- MacKenzie DJ, Craw D. 2005. The mercury and silver contents of gold in quartz vein deposits, Otago Schist, New Zealand. New Zealand Journal of Geology & Geophysics. 48:265–278. doi: 10.1080/00288306.2005.9515114
- McKay A. 1897. Report on the older auriferous drifts of Central Otago. 2nd ed. Wellington: Government Printer.
- Mortensen JK, Craw D, MacKenzie DJ, Gabites JE, Ullrich T. 2010. Age and origin of orogenic gold mineralisation in the Otago Schist belt, South Island, New Zealand: Constraints from lead isotope and 40Ar/39Ar dating studies. Economic Geology. 105:777–793. doi: 10.2113/gsecongeo.105.4.777
- Pitcairn IK, Olivo GR, Teagle DAH, Craw D. 2010. Sulfide evolution during prograde metamorphism of the Otago and Alpine Schists, New Zealand. The Canadian Mineralogist. 48:1267–1295. doi: 10.3749/canmin.48.5.1267
- Turnbull IM. 2000. Geology of the Wakatipu area. 1:250 000 Geological Map 18. Lower Hutt, New Zealand: Institute of Geological and Nuclear Sciences Limited.
- Turnbull IM, Mortimer N, Craw D. 2001. Textural zones in the Haast Schist—a reappraisal. New Zealand Journal of Geology and Geophysics. 44:171–183. doi: 10.1080/00288306.2001.9514933
- Williams GJ. 1974. Economic geology of New Zealand. Carlton, Australia: Australasian Institute of Mining and Metallurgy Monograph. 4: 490 pp.
- Youngson JH, Adamson S. 2001. The influence of alluvial gold shape and density on discrepancies between prospecting and mining gold grades: implications for plant efficiency, plant design, and mine profits. Proceedings, New Zealand Branch Conference, Parkville, Victoria, Australia: Australasian Institute of Mining and Metallurgy, 11 pp.
- Youngson JH, Craw D. 1993. Gold nugget growth during tectonically induced sedimentary recycling, Otago, New Zealand. Sedimentary Geology. 84:71–88. doi: 10.1016/0037-0738(93)90046-8
- Youngson JH, Craw D. 1999. Variation in placer style, gold morphology, and gold particle behavior down gravel bed-load rivers: an example from the Shotover/Arrow-Kawarau-Clutha river system, Otago, New Zealand. Economic Geology. 94:615–633. doi: 10.2113/gsecongeo.94.5.615
- Youngson JH, Craw D, Falconer DM. 2006. Evolution of Cretaceous-Cenozoic quartz pebble conglomerate gold placers during basin formation and inversion, southern New Zealand. Ore Geology Reviews. 28:451–474. doi: 10.1016/j.oregeorev.2005.02.004