ABSTRACT
A revised stratigraphy is presented for the late Miocene–early Pliocene sedimentary rocks of the northern Aorangi Range, Wairarapa. Despite major differences in lithology, the Clay Creek Limestone and Bells Creek Mudstone are shown to be partially coeval, while the overlying Makara Greensand is shown to be a diachronous unit that ranges from late Miocene (Kapitean) to early Pliocene (Opoitian) age. This revised stratigraphy raises questions about the current classification of the Palliser and Onoke groups, and provides new insights into regional geological history. Previous seismic imaging studies have identified an episode of accelerated crustal shortening and deformation in the Wairarapa region near the Miocene–Pliocene boundary. The Clay Creek Limestone has proven to be a useful marker horizon for constraining the timing and style of this deformational episode, which is interpreted to have occurred prior to 7.2 Ma.
Introduction
Seismic reflection studies in the Wairarapa region (Cape et al. Citation1990; Nicol et al. Citation2002) show evidence for an episode of uplift and accelerated tectonic shortening during the latest Miocene or early Pliocene. Sedimentary rocks of this age are exposed in the northern Aorangi Range, where their stratigraphy varies greatly over distances of only a few kilometres (Vella and Briggs Citation1971). The late Miocene–early Pliocene succession can provide improved constraints on the paleogeography and tectonic history of the Wairarapa region during this time interval.
The Neogene stratigraphy of the northern Aorangi Range has been extensively studied since the early 20th century. However, the most detailed studies (Devereux et al. Citation1970; Kennett et al. Citation1971; Vella and Collen Citation1984) have focused on the Pliocene–Pleistocene succession, with relatively little attention paid to the less widespread late Miocene–early Pliocene units. The definitions and interpretations of some late Miocene–early Pliocene units, especially the Clay Creek Limestone and Makara Greensand, have proven to be problematical, with several published and unpublished studies (Couper Citation1948; McLean Citation1953; Bates Citation1967; Abbas Citation1971; Vella and Briggs Citation1971; Green Citation1981; Hatfield Citation1981; Vella and Collen Citation1984; Crundwell Citation1997) giving very different accounts of unit ages, distribution, thicknesses and depositional environments. Previous authors’ stratigraphic interpretations have been summarised by Bertaud-Gandar (Citation2015).
In this paper, we present a revised stratigraphy and chronology that provide new constraints on the late Miocene–early Pliocene tectonic history of the area. The Clay Creek Limestone, which partly overlies Cretaceous basement and partly overlies late Miocene sedimentary rocks, is identified as a key marker horizon for late Miocene deformation in the southern Wairarapa region.
Regional geological setting
The study area is located within the southern East Coast Basin, an actively deforming forearc basin associated with the Hikurangi subduction margin (e.g. Field et al. Citation1997; Begg and Johnston Citation2000). The southern part of the East Coast Basin is divided into five structural basement blocks: the Aorangi, Pongaroa, Woodville, Coastal and Tora blocks (Moore Citation1988; see ). Deformation associated with the Hikurangi Margin is partitioned into a zone of strike-slip faulting along the frontal ridge, and a fold-and-thrust belt extending from the inner forearc to the subduction trench (Cape et al. Citation1990; Nicol et al. Citation2002, Citation2007).
Figure 1. Location of the current study in the southern East Coast Basin, showing simplified geology and structural setting. Modified from Hines et al. (Citation2013).
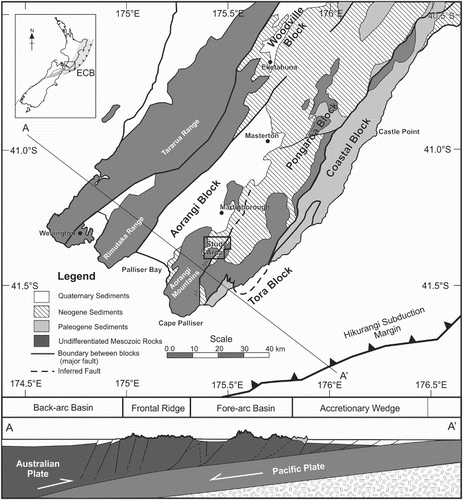
The Aorangi Range is the southernmost of a series of coastal ranges extending along the eastern side of the Wairarapa region, and represents the highest part of the uplifted and deformed inner forearc. The coastal ranges are separated from the North Island axial ranges by the Wairarapa–southern Hawkes’s Bay depression, part of the forearc basin. The area of this study (shown in ) crosses the boundary between the Aorangi and Pongaroa blocks, which is marked by the Wairangi–Mangaopari fault system (Field et al. Citation1997).
Basement rocks of Late Permian to Early Cretaceous age are exposed in the axial and coastal ranges. In the Wairarapa depression, these basement rocks are overlain by late Miocene to Quaternary sedimentary rocks (Begg and Johnston Citation2000; Lee and Begg Citation2002). This sedimentary record has allowed the paleogeography of the eastern North Island to be reconstructed in some detail through the Pliocene and Pleistocene (Kamp et al. Citation1988; Beu Citation1995; Trewick and Bland Citation2011). However, rocks of Miocene age in the southern East Coast Basin are less widespread than younger sedimentary rocks, and few paleogeographic details are known for this time interval (Beu Citation1995).
One of the defining features of the East Coast Basin Neogene sedimentary record is the presence of unusual coarse-grained, barnacle-dominated limestones, which are mostly of Pliocene age, but range from late Miocene to early Pleistocene (Beu et al. Citation1980; Kamp et al. Citation1988; Beu Citation1995; Nelson et al. Citation2003). By volume, these limestones account for < 10% of Neogene sediments in the basin (Nelson et al. Citation2003), but they are a distinctive, widespread and recurring facies throughout the Wairarapa and Hawke’s Bay regions. This barnacle-dominated limestone facies is known as the Te Aute lithofacies (Beu et al. Citation1980; Beu Citation1995). The Pliocene Te Aute limestones have been interpreted as having been deposited on the margins of a narrow, tide-swept forearc seaway called the Ruataniwha Strait (Beu et al. Citation1980; Pettinga Citation1980; Kamp et al. Citation1988; Beu Citation1995; Caron et al Citation2004; Trewick and Bland Citation2011).
Five limestones belonging to the Te Aute lithofacies are present in the study area (Beu Citation1995). The late Miocene Clay Creek Limestone is the oldest of these, and is sometimes described as a forerunner to the ‘true’ Te Aute lithofacies of the Pliocene (Nelson et al. Citation2003). It has previously been interpreted as representing a shallow offshore reef environment (Vella and Briggs Citation1971), or a shallow nearshore environment adjacent to an emergent island of uplifted basement rocks (Begg and Johnston Citation2000). The Clay Creek Limestone’s stratigraphic position, overlying both Cretaceous basement and late Miocene sedimentary strata, make this formation a particularly useful marker horizon for interpreting the late Miocene–early Pliocene tectonic history of the area.
Methods
Mapping
In preparation for field study, maps produced in previous studies of the northern Aorangi Range (see Figure S1) were scanned and the images were imported into ArcMap. Images were manually georeferenced to the NZMS260 rivers vector dataset. A draft synthesis map was digitised from these data, and was used to correlate units and to identify key locations for field study.
Detailed field mapping was carried out along Te Ahitaitai Ridge and in the adjacent Makara River valley. Station locations, outcrop lithology, and strike and dip data were plotted on a 20 m topographic contour map in the field. This Te Ahitaitai map, along with observations from measured section localities across the study area, were incorporated into the synthesis map presented in .
Figure 2. Geological map of the Makara and Ruakokoputuna valleys, northern Aorangi Range, Wairarapa, New Zealand. Compiled from field observations with additional data from Couper (Citation1948), Bates (Citation1967), Vella and Briggs (Citation1971), Crundwell (Citation1979), Green (Citation1981), Hatfield (Citation1981) and Vella and Collen (Citation1998). Topographic data sourced from LINZ. Background hillshade derived from the NZSoSDEM v1.0 dataset.
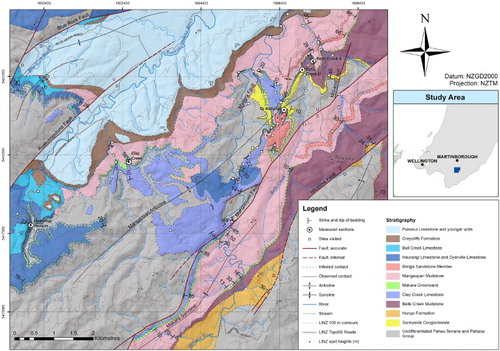
Sections were measured at five key localities: Clay Creek, Te Ahitaitai Ridge, two localities in Bells Creek, and a road cutting in the Haurangi Road, Ruakokoputuna Valley informally referred to as the Haurangi Hairpin (see ). These sections provide key reference sites for correlating stratigraphy from southwest to northeast across the study area. Sections were measured using a 30 m tape measure, geological compass, Jacob’s staff and Abney level. Sections were described from outcrop and shallow excavation where required, using standard terminology and symbols after Andrews (Citation1982). Fossil and bulk rock samples were collected, and their stratigraphic heights recorded in the section descriptions. Several additional samples were collected during field mapping around Te Ahitaitai Ridge to compliment the measured sections.
Sample preparation and analysis
For foraminiferal analysis, crushed and disaggregated sediment samples were sieved at 64 µm, and a census of 300 foraminifera was picked from each sample where possible. A total of 15 censuses were carried out. Paleodepth and oceanicity assessments for foraminiferal assemblages were carried out based on Hayward et al. (Citation2010), with oceanicity based on planktic percentages and paleodepth based on benthic index species present within the samples. Macrofossils were identified both in outcrop and from bulk samples disaggregated in the laboratory.
Three bulk rock samples, which contained significant amounts of organic matter visible in hand sample, were processed using standard palynological processing techniques. Dissolution using 40% HF was followed by a heavy liquid separation using sodium polytungstate with a specific gravity of 1.85. Samples were mounted on glass microscope slides.
Further details of methodology, sample preparation and analysis are given in Bertaud-Gandar (Citation2015). Sample numbers and full faunal lists are recorded in the Fossil Record File Database administered by GNS Science and the Geoscience Society of New Zealand (www.fred.org.nz). A table of all samples collected for this study, with sample numbers, is included in the supplementary material for this article. Samples are held in the collection at Victoria University of Wellington.
Structural geology
The synthesis map displayed in shows several key structural features of the study area. The area is dominated by large, northeast–southwest striking reverse faults and folds, most notably the Mangaopari, Ruakokoputuna, Blue Rock, and Nikorima faults. The Nikorima Fault is a new name, after a nearby hill. The northern and southern segments of this fault have been mapped previously by several geologists (e.g. Crundwell Citation1979; Eggo Citation1979; Green Citation1981), whereas the central segment connecting the two is inferred here based on the mapped geometry of the contact between the Cretaceous basement and overlying Neogene units. Other smaller-scale structural features include the Birch Hill Syncline (new name), and the Makara Fault, which has been previously mapped by Couper (Citation1948) and Abbas (Citation1971).
The Mangaopari Fault, which separates the Aorangi Block from the Pongaroa Block (Moore Citation1988) is the most important structural feature in the study area. It is here shown to be a more complex feature than has previously been recognised. In addition to the previously recognised McLeod Fault (Vella and Collen Citation1998), one new splay fault, the Te Ahitaitai Fault, has been confirmed by field observations. The Mangaopari Fault is additionally inferred to split into two sub-parallel strands near the Makara River’s upper limestone gorge at Paruwai. The extent of the second, western fault strand to the northeast is not known; it is inferred to diminish rapidly to the southwest of its mapped location, where the main expression of the Mangaopari Fault is a large monoclonal flexure in the Clay Creek Limestone, which extends for a distance of 1 km along the strike of the fault. Further to the southwest, vertical offset across the Mangaopari Fault increases again (Green Citation1981; Hatfield Citation1981).
Stratigraphy
The basement rocks in the study area are indurated quartzose sandstone, argillite and minor spilite belonging to the Pahau Terrane and overlying Pahaoa Group of the Torlesse Composite Terrane (Moore and Speden Citation1984; Begg and Johnston Citation2000), which are not differentiated for the purposes of this study. The Neogene stratigraphy of the study area was originally described by Vella and Briggs (Citation1971), and revised by Vella and Collen (Citation1984), Beu (Citation1995) and Crundwell (Citation1997). The Neogene rocks described in this paper belong to the Palliser and Onoke groups (Vella and Briggs Citation1971; Begg and Johnston Citation2000) QMAP units Msp, Msb and Pea (Begg and Johnston Citation2000), and the Māui and Pākihi supergroups of Mortimer et al. (Citation2014).
The measured sections presented in show detailed late Miocene–early Pliocene stratigraphy at five key reference sites across the study area. From these we present description, distribution, paleontology, age () and depositional environment results for the Sunnyside Conglomerate, Bells Creek Mudstone, Clay Creek Limestone, Makara Greensand and the lower Mangaopari Mudstone. Other formations in the Neogene succession, such as the Hurupi Formation and Haurangi and Dyerville limestones, have only a limited distribution within the study area and are not considered in detail here.
Figure 3. Sections measured during field study, showing sample heights and correlations across the study area.
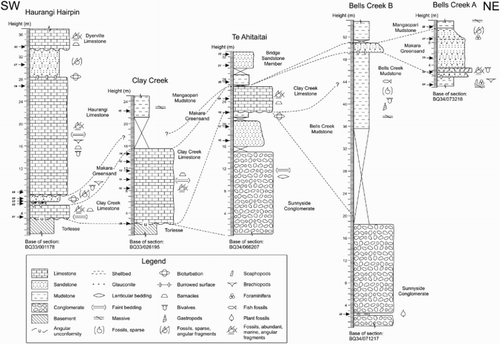
Table 1. Summary of results from foraminiferal censuses carried out for this study.
Sunnyside Conglomerate
Description
The Sunnyside Conglomerate is the basal unit of the Neogene succession in the northeast of the study area, and overlies the Torlesse basement rocks with an angular unconformity. Vella and Briggs (Citation1971) described the Sunnyside Conglomerate as an unsorted and poorly imbricated conglomerate, composed of indurated sandstone and argillite clasts that resemble the underlying Torlesse rocks. We observed the conglomerate as a mixture of matrix- and clast-supported; the matrix consists of medium to coarse-grained quartzose sand. Clasts range from sub-centimetre pebbles to boulders c. 60 cm in diameter, and from sub-angular to well-rounded. Smooth, rounded, oval or spheroidal cobbles are especially common. Interbedded within the conglomerate at some locations are discontinuous decimetre to metre-scale beds of friable, light brown sandstone and decimetre-scale beds of laminated, organic-rich siltstone.
Distribution and lithological variation
The Sunnyside Conglomerate is thickest on Te Ahitaitai Ridge. It was measured to be at least 22 m thick in the Te Ahitaitai section, and at least 18 m thick on the western side of Bells Creek (see ). Its basal contact was not exposed in either location, and the maximum thickness of the unit is unknown. In several locations along Te Ahitaitai Ridge, the upper part of the conglomerate fines to gritty sandstone and sandy mudstone. This uppermost sandy facies is often several metres thick, as in the Te Ahitaitai section shown in .
Figure 4. A, Basal contact of the Clay Creek Limestone overlying weathered Torlesse basement at Homestead Creek. B, Basal contact of the Clay Creek Limestone overlying Bells Creek Mudstone at Homestead Creek. C, Clay Creek Limestone folded and truncated against the Te Ahitaitai Fault (dotted line) at the southern end of Saw Cut Gorge, Makara River. D, Abundant small basement-derived pebbles within the Clay Creek Limestone at the southern end of Saw Cut Gorge. E, Well-bedded Clay Creek Limestone showing high-angle planar cross-stratification, Saw Cut Gorge. Image is c. 2 m wide. F, Giant-scale trough cross-stratification in the Clay Creek Limestone at the northern end of Saw Cut Gorge.
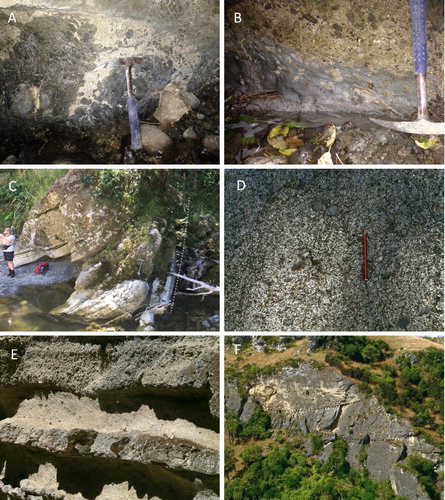
West of Te Ahitaitai Ridge, the conglomerate is truncated by the Makara Fault, and it thins to the south and eventually pinches out below the Clay Creek Limestone north of Saw Cut Gorge. The conglomerate also thins to the east; Vella and Briggs (Citation1971) reported its thickness as no more than 5 m at its type locality in a tributary of Mangaopari Stream known informally as McLeod’s Creek.
The Sunnyside Conglomerate is restricted to the northeastern part of the area mapped in ; however, Sunnyside Conglomerate is also present in the upper Makara River valley, south of the mapped area. In the upper Makara River and its tributary Haitai Stream, the Sunnyside Conglomerate overlies Torlesse basement and conformably underlies Hurupi Formation sandstones and mudstones (Hatfield Citation1981).
Paleontology and age
No macrofossils or foraminifera were found in the Sunnyside Conglomerate; however, the laminated siltstone facies (S27/f0693) was found to contain very abundant fossilised spores, pollen and plant debris. These include Nothofagidites, abundant swamp types, Myrtaceae type, Podocarpaceae type, Proteacidites, Haloragacidites and Asteraceae type.
The age of the Sunnyside Conglomerate in the study area is not well-constrained; however, based on its position conformably underlying the Hurupi Formation, which has a well-established Tongaporutuan age (King Citation1933; Vella Citation1954; Beu and Maxwell Citation1990) to the south of the present study area, the Sunnyside Conglomerate is considered to be lower Tongaporutuan or possibly Waiauan in age (c. 10–12 Ma).
Depositional environment
The Sunnyside Conglomerate has previously been interpreted as a beach deposit or shallow marine debris flow (Vella and Briggs Citation1971; Begg and Johnston Citation2000); however, it is here interpreted as a fluvial conglomerate. The pollen assemblage found in the interbedded organic siltstone indicates the presence of nearby shrubland and forest. The abundance of plant material in this sample, combined with the absence of marine fossils in the Sunnyside Conglomerate, strongly implies that this unit was deposited in a terrestrial rather than a marine environment. This interpretation is further supported by the generally spheroidal clast morphology and the absence of the disc-shaped clasts commonly seen in greywacke beach gravels; river pebbles are generally more spherical than comparable beach pebbles (Dobkins and Folk Citation1970).
Bells Creek Mudstone
Description
The Bells Creek Mudstone is a massive, blue–grey, clay-rich mudstone. It was initially defined by Vella and Briggs (Citation1971) as a calcareous mudstone containing a distinctive assemblage of sparse, scattered bivalves, gastropods and scaphopods, and the definition was broadened by Vella and Collen (Citation1984) to include laterally equivalent mudstones lacking this fossil assemblage.
Distribution and lithological variation
The Bells Creek Mudstone is present only in the eastern half of the study area, and thickens significantly from west to east. In Bells Creek Section B, the thickness of the Bells Creek Mudstone cannot exceed 31 m (see measured section, ), but in Mangaopari Stream its thickness has been measured as 290 m (Vella and Briggs Citation1971). It variably overlies Sunnyside Conglomerate, Hurupi Formation and Torlesse basement. Vella and Briggs (Citation1971) considered the relationship between the Bells Creek Mudstone and Sunnyside Conglomerate to be conformable. However, the units’ differing relationships with the Makara Fault indicate an angular unconformity: the Sunnyside Conglomerate is present on the downthrown side of the Makara Fault, but absent on the upthrown side, where lenses of Bells Creek Mudstone rest unconformably on Torlesse basement.
In the southern Makara River valley, at Paruwai, the Bells Creek Mudstone overlies the Hurupi Formation disconformably (Green Citation1981; Hatfield Citation1981). Northeast of Paruwai, the upper, muddy part of the Hurupi Formation is interpreted to grade laterally into the lower Bells Creek Mudstone; in the southern part of Mangaopari Stream, the Hurupi Formation is represented only by a thin sandstone unit which underlies Bells Creek Mudstone and overlies Torlesse basement (see ).
While the Bells Creek Mudstone is generally massive, centimetre-scale bedding was observed at one locality in the Paruwai area (BQ34/052178; FRF number S28/f0514), where the mudstone contained abundant shell fragments and foraminifera visible in hand sample. Millimetre-scale fragments of dark-coloured carbonaceous matter, clearly visible in a hand sample, were observed in the Bells Creek Mudstone at Bells Creek and the Makara River tributary informally known as Homestead Creek.
Paleontology and age
Foraminifera in the Bells Creek Mudstone are abundant, diverse, and generally well-preserved. Benthic assemblages are dominated by buliminids and rotaliids; Uvigerina spp. are especially abundant in some samples, where they account for up to 36% of benthic assemblages (see ). Planktic percentages range from 8% to 38%. The unit has an early Tongaporutuan age at its base, where sample S27/f0664 is assigned to the Tukemokihi Coiling Zone (Crundwell et al. in Cooper Citation2004; Raine et al. Citation2015) based on the presence of abundant dextrally coiling Globoconella miotumida in addition to both Globoquadrina dehiscens and Neogloboquadrina pachyderma. Sample S28/f0514, taken near the top of the unit, has a Kapitean age, as indicated by the presence of Globoconella conomiozea. Mudstone samples from Bells Creek (S27/f0679) and Homestead Creek (S27/f0664) were found to contain moderately abundant spores, plant debris, and pollen, including Nothofagidites, Lateropora, Cyathea and monolete fern spores.
Depositional environment
Although benthic foraminiferal assemblages from the Bells Creek Mudstone indicate deposition at upper bathyal depths, the low planktic percentages indicate a neritic water mass. The abundance of Uvigerina spp. suggests a depositional environment with high carbon flux and low oxygen levels (Hayward et al. Citation2010). The Bells Creek Mudstone is therefore interpreted as having been deposited in a deep coastal basin, sheltered from the open ocean. The lack of exposure to oceanic water and sheltering from currents would have created a stratified water column, with low oxygen levels at depth. Low-oxygen conditions also allowed for the preservation of carbonaceous matter.
Clay Creek Limestone
Description
The Clay Creek Limestone was defined by Vella and Briggs (Citation1971) as the basal unit of the Onoke Group. It is a coarse-grained, well-cemented coquina limestone that is white to pale yellow–grey on fresh surfaces and weathers to dark grey. One of its most distinguishing features is the presence of sparse to very abundant pebbles and cobbles of basement-derived sandstone and argillite, which range from sub-angular to rounded, and in some places cause the limestone to resemble a calcareous conglomerate. Our analysis shows that in a typical thin section, the limestone is shown to have micritic cement, with only small amounts of sparry calcite present.
Distribution and lithological variation
In the western and central parts of the study area, the Clay Creek Limestone unconformably overlies Cretaceous metasedimentary basement rocks. The basal contact of the limestone is highly irregular, with 1–2 m of relief visible at several localities. The underlying Torlesse rocks are often highly sheared and brecciated. Limestone infills fissures in the Torlesse surface to depths of c. 50 cm, and horizontal limestone stringers can also be observed extending into brecciated Torlesse at some locations (A).
In the east and south of the study area, the Clay Creek Limestone variably overlies the Sunnyside Conglomerate, Hurupi Formation and Bells Creek Mudstone. The contact between the Clay Creek Limestone and Bells Creek Mudstone is unconformable, and appears heavily bioturbated and possibly sheared at exposures in Homestead Creek (B). In the Paruwai area, however, the Clay Creek Limestone overlies Bells Creek Mudstone conformably, and Clay Creek Limestone on the western side of the Makara Syncline passes laterally into Bells Creek Mudstone on the eastern side of the syncline. A lateral gradation between the Clay Creek Limestone and Bells Creek Mudstone is also inferred in Bells Creek, with limestone passing laterally into mudstone on the eastern side of Te Ahitaitai Ridge.
Vella and Briggs (Citation1971) reported the thickness of the Clay Creek Limestone as varying between 1 and 10 m, but it is now apparent that the Clay Creek Limestone is much thicker in the central part of the study area. It reaches an estimated maximum thickness of 100 m at the Makara River’s upper limestone gorge (BQ34/501179). Between the Makara River and Clay Creek is a broad limestone plateau with numerous large sinkholes and other karst features. Observations from caves in the plateau (G. Holden, pers. comm.) indicate that the Clay Creek limestone is at least 30 m thick in this area.
Saw Cut Gorge
The type locality of the Clay Creek Limestone, designated by Vella and Briggs (Citation1971), is now overgrown by vegetation. However, a section with excellent exposure was identified at a location in the Makara River informally known as Saw Cut Gorge. In this area, the Makara River has cut a narrow, 350 m long gorge through the Clay Creek Limestone. The Clay Creek Limestone has an estimated thickness of 30–35 m in this section. At the north end of the gorge, the limestone overlies sheared and brecciated Torlesse basement with an angular unconformity, and dips to the southeast. It forms a syncline through the gorge and dips west–northwest at the southern end, where it is truncated by the Te Ahitaitai Fault (C). The Clay Creek Limestone at this location is unconformably overlain by Dyerville Limestone of late Opoitian to Waipipian age.
The Clay Creek Limestone at Saw Cut Gorge varies from weakly bedded to well-bedded on a decimetre scale. In lithology it varies from sandy, pebbly shell hash to well-cemented calcareous conglomerate (D), sometimes changing laterally over distances of only a few metres. Finer-grained beds often display high-angle, planar, unidirectional cross-stratification (E). Disarticulated pectinid valves with varying orientations (concave up or concave down) are abundant at some horizons, but most shell material consists of sub-centimetre sized angular fragments.
At the northern end of Saw Cut Gorge, giant-scale cross-stratification (metres to tens of metres) can be seen in the limestone cliffs on the true right of the river, including bidirectional trough cross stratification resembling channel deposits (F) and some poorly-exposed possible high-angle planar cross-stratification. Giant cross-beds are a prominent feature of some Pliocene Te Aute limestones in Hawke’s Bay (Kamp et al. Citation1988), but these features have not, to our knowledge, been previously recognised in limestones from the Wairarapa region.
Paleontology and age
Macrofossils in the Clay Creek Limestone are generally poorly preserved; most shell material in the limestone consists of angular fragments. Rare identifiable molluscs include Mesopeplum burnetti, Mesopeplum (Borehamia) crawfordi, Phialopecten sp., Crassostrea ingens and Fissidentalium solidum. The most abundant molluscan fossils are disarticulated juvenile ostreid valves, which are generally < 2 cm across. Other macrofossils include abundant barnacle plates, spherical bryoliths generally < 1 cm in diameter, and the brachiopods Neothyris sp. and Notosaria nigricans.
Foraminifera are abundant in the Clay Creek Limestone, but their preservation is variable; many specimens are fractured and abraded and some show signs of partial dissolution. Assemblages consist mostly of benthic taxa, with planktic percentages ranging from 1% to 12% (see ). Agglutinated species, especially Gaudryina convexa, are abundant in some samples, while in other samples the benthic assemblage is dominated by buliminids and rotaliids. Age indicative species include Textularia kapitea and rare Globoconella conomiozea and Gc. miotumida. Micro-brachiopods and ostracods, including articulated specimens, were also found in some limestone samples. The unit has an early Kapitean age, based on the presence of Gc. conomiozea, and may extend back to the latest Tongaporutuan in some locations where Gc. miotumida is present.
Depositional environment
The low planktic percentages in Clay Creek Limestone foraminiferal samples indicate an inner to middle neritic water mass. Gaudryina convexa is most abundant in exposed, high-energy, inner to mid shelf environments (Hayward et al. Citation2010), and the presence of shallow-water taxa such as Elphidium novozealandicum and Pileolina sp. would also be consistent with an inner or mid shelf depositional environment. The presence of ostreids, pectinids, brachiopods and barnacles in the limestone also indicates a high-energy marine environment with a hard substrate and limited terrigenous input.
However, other foraminifera present in the limestone suggest a deeper-water depositional environment: Cibicides molestus and Trifarina bradyi both have upper depth limits of c. 150 m, and are considered depth index species for the outermost shelf (Hayward et al. Citation2010). This combination of species suggests that some mixing of sediments and benthic faunas has occurred, most likely by transportation of shallow water sediment and deposition on the outer shelf. The micritic cement and rarity of intact macrofossils also support this interpretation.
Makara Greensand
Description
The Makara Greensand disconformably overlies both the Clay Creek Limestone and Bells Creek Mudstone. It is a thin unit of friable glauconitic sandstone and mudstone, sometimes containing scattered pebbles of basement-derived sandstone and argillite. Sparse to very abundant shell fragments and rare intact shells are also present in the greensand at some locations.
Distribution and lithological variation
The thickness of the Makara Greensand in the study area is highly variable but does not exceed 10 m; at some locations, such as the Te Ahitaitai section (, column 3), the greensand is absent entirely. Where the greensand is present, its basal contact appears highly bioturbated, with burrows extending to depths of c. 30 cm into the underlying limestone or mudstone.
At some locations the Makara Greensand appears as a relatively homogeneous glauconitic sandstone or mudstone, whereas at other locations it can be divided into beds with differing lithologies. At the Haurangi Hairpin section, the Makara Greensand consists of two subunits: 60 cm of grey–green glauconitic mudstone overlain by 1.4 m of alternating glauconitic sandstone and shell hash (, column 1). Four distinct subunits are present in Bells Creek section A (, column 5). The glauconitic shell bed and underlying greensand in this section have previously been considered to be part of the Clay Creek Limestone (Vella and Briggs Citation1971; Dobbie Citation1976; Beu Citation1995), which was thought to grade laterally from cemented pebbly limestone on Te Ahitaitai Ridge to poorly cemented pebbly shell bed in Bells Creek. The absence of limestone or a shell bed in Bells Creek section B is here considered evidence against this interpretation.
Paleontology and age
Macrofossils are very rare at most Makara Greensand localities, but significant numbers were found at two localities in the study area. The upper, sandy subunit of the Makara Greensand at the Haurangi Hairpin section () contains centimetre-scale beds of Neothyris sp. and Phialopecten cf. tolagaensis, along with less common Mesopeplum waikohuense. The glauconitic shell bed in Bells Creek section A consists mostly of angular fragments, but contains rare intact specimens of Neothyris ovalis, Notosaria nigricans, Tucetona laticostata, Mesopeplum sp. and Purpurocardia sp.
Foraminiferal abundance and preservation in the Makara Greensand vary greatly across the study area. Foraminiferal censuses were successfully conducted on samples from the Haurangi Hairpin and Bells Creek section A, while samples collected from Homestead Creek and Te Ahitaitai Ridge contained few or no foraminifera.
Where foraminifera are present, assemblages show a pattern of higher planktic percentages with increasing stratigraphic height (see and ). Benthic assemblages vary significantly between samples but tend to be dominated by buliminids and rotaliids, with less abundant but still generally diverse lagenids and agglutinated specimens. See for key depth index species for each sample.
The presence of Globoconella puncticulata and Globoconella pliozea indicates an Opoitian age for both subunits of the greensand at the Haurangi Hairpin section. The upper two samples from Bells Creek section A also have an Opoitian age, but the lowest sample, taken from the shell bed, is Kapitean or possibly late Tongaporutuan, based on the presence of Globoconella conomiozea and Globoconella miotumida. The greensand mapped in the Paruwai area by Green (Citation1981) and Hatfield (Citation1981) is inferred to be entirely Kapitean in age, as the Kapitean index scallop Sectipecten wollastoni was recorded from shell beds in the overlying mudstone. The Makara Greensand is therefore interpreted as a diachronous unit which ranges in age from Kapitean to early Opoitian.
Depositional environment
Benthic foraminiferal assemblages from the Makara Greensand indicate an increase in water depth, with increasing stratigraphic height at Bells Creek, from upper bathyal for sample BC2 (S27/f0688) to mid- or lower bathyal for BC3 (S27/f0690) and BC4 (S27/f0691). The opposite trend is apparent at the Haurangi Hairpin, where sample HH2 (S28/f0515) has a mid-bathyal assemblage and sample HH3 (S28/f0511) has an outer shelf to upper bathyal assemblage. Both sections show an increase in oceanicity with stratigraphic height, as indicated by increasing planktic percentages in higher samples.
The greensand is considered indicative of a decrease in the rate of sedimentation, which allowed glauconite to form over an extended period. The Makara Greensand represents a condensed interval of geological time and is tentatively interpreted as being mostly a winnowed lag deposit, with the shell bed in Bells Creek section A representing a debris flow.
Mangaopari Mudstone
Description
The Mangaopari Mudstone is a thick unit of predominantly massive, grey mudstone. Vella and Briggs (Citation1971) informally divided the Mangaopari Mudstone into lower, middle and upper subunits, and also formally recognised one member: the Bridge Sandstone Member of the lower Mangaopari Mudstone. The Bridge Sandstone Member consists of several packages of well-sorted, friable sandstone beds with sharp basal contacts, which fine upwards to grey mudstone on a decimetre to metre scale.
Distribution and lithological variation
In the eastern, southern and central parts of the field area, the Mangaopari Mudstone conformably overlies the Makara Greensand. The lower Mangaopari Mudstone is considered a deep-water lateral equivalent of both the Haurangi and Dyerville limestones (Vella and Briggs Citation1971; Beu Citation1995). The Bridge Sandstone Member has been previously mapped on the northwestern side of the Mangaopari Fault, in Mangaopari Stream and Bells Creek (Vella and Briggs Citation1971). This study shows it to be more widespread than previously thought; it is also present in Mangaopari Stream south of the Mangaopari Fault, and around the Te Ahitaitai summit area. It has not been observed in the Makara River, and is assumed to pinch out on the western side of Te Ahitaitai Ridge; its western extent on the southeastern side of the Mangaopari Fault is unknown.
A new facies within the lower Mangaopari Mudstone is observed in the upper Makara River valley, to the south of the upper limestone gorge at Paruwai. In this area, the Clay Creek Limestone and Makara Greensand are overlain by a grey mudstone which is interbedded with cemented pebbly shell beds on a decimetre scale (). These shell beds have sharp basal contacts and grade upwards into mudstone in repeating packages; the shell beds resemble the Clay Creek Limestone in lithology. The mudstone-shell bed facies is overlain by massive mudstone.
Paleontology and age
The lower Mangaopari Mudstone in the Te Ahitaitai and Mangaopari Stream areas contains extremely rare macrofossils, including gastropods and scaphopods. Foraminifera are generally well-preserved and moderately abundant; large Lenticulina specimens are sometimes visible in hand sample. The Mangaopari Mudstone in Bells Creek section A (S17/f0692) contains the highest planktic percentage of any foraminiferal sample analysed in this study, at 64%.
The lower part of the Mangaopari Mudstone is Opoitian in age on the northwestern (upthrown) side of the Mangaopari Fault. However, on the southeastern (downthrown) side of the fault, the basal Mangaopari Mudstone is thought to be of Kapitean age. Both Green (Citation1981) and Hatfield (Citation1981) reported the presence of the Kapitean index scallop Sectipecten wollastoni in the pebbly shell beds which are interbedded in the Mangaopari Mudstone at Paruwai. The Opoitian foraminifer Globoconella puncticulata was reported as first occurring in the massive mudstone overlying the shell bed facies (Green Citation1981; Hatfield Citation1981).
Depositional environment
Foraminiferal samples from the lower Mangaopari Mudstone indicate a bathyal depositional environment, with oceanicity varying from outer neritic to sub-oceanic. The Bridge Sandstone Member and the interbedded mudstone–shell bed facies at Paruwai are interpreted as turbidite successions from different source areas, following the interpretations of Vella and Briggs (Citation1971), Green (Citation1981) and Hatfield (Citation1981).
Stratigraphic summary
combines data from measured sections with additional field observations and data from previous studies to show lateral relationships between units. The diagram shows marked changes in late Miocene–early Pliocene stratigraphy from the southwest to the northeast of the study area. It also shows a change in lithologies, from limestone-dominated stratigraphy at the Haurangi Hairpin, to limestone overlain by mudstone in central parts of the study area, and finally to mudstone-dominated stratigraphy overlying the Sunnyside Conglomerate in the Bells Creek sections.
Figure 6. Fence diagram showing correlations between units across the study area. Stratigraphic columns are compiled from measured sections and field observations, with additional unit thickness data from Vella and Briggs (Citation1971) for the Haurangi Hairpin column, Green (Citation1981) for the upper limestone gorge column, and Couper (Citation1948) for the Saw Cut Gorge column.
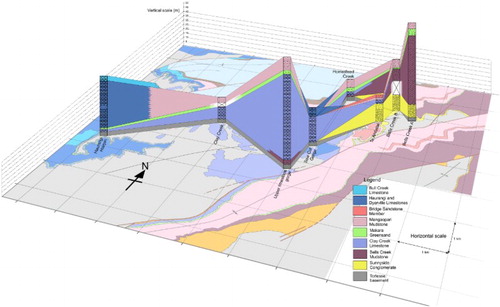
There are significant lateral variations in the thicknesses of both the Clay Creek Limestone and Bells Creek Mudstone. The Clay Creek Limestone is shown to be a wedge- or lens-shaped unit, varying in thickness between 1 m and as much as 100 m over distances of only a few kilometres. It reaches its maximum thickness at the upper limestone gorge at Paruwai, and thins rapidly to the northeast and to the west. The Bells Creek Mudstone shows a similarly rapid change in thickness across the Te Ahitaitai area.
Another key feature of the late Miocene–early Pliocene stratigraphy in the study area is the short distances (< 1 km) over which complete lateral transitions occur from coarse-grained limestone to fine-grained terrigenous mudstone. This can be seen in both the Clay Creek Limestone–Bells Creek Mudstone transition in the Te Ahitaitai area, and the Mangaopari Mudstone–Haurangi and Dyerville limestones transition in the Ruakokoputuna Valley.
Significant differences in stratigraphy are apparent on the upthrown and downthrown sides of the Mangaopari Fault. These differences are shown in , which summarises the inferred spatial and temporal relationships between lithostratigraphic units in the study area. These differences have proven useful for constraining the history of activity on the Mangaopari Fault, and its role in the geological history of the study area.
Figure 7. Late Miocene–early Pliocene lithostratigraphy in two parallel sections from southwest to northeast of the study area, separated by the Mangaopari Fault. Grey areas indicate intervals of erosion or non-deposition. Ages in Ma are based on Raine et al.’s (Citation2015) updated New Zealand Geological Timescale. The key horizon for constraining uplift in the latest Miocene is the base of the Clay Creek Limestone (c. 7.2 Ma). Also shown is the diachronous nature of the Makara Greensand and base of the Mangaopari Mudstone. Sw = Waiauan, Tt = Tongaporutuan, Tk = Kapitean, Wo = Opoition, Wp = Waipipian. Data from east of the Mangaopari Fault are from field observations with additional information from Green (Citation1981), Hatfield (Citation1981) and Crundwell (Citation1979).
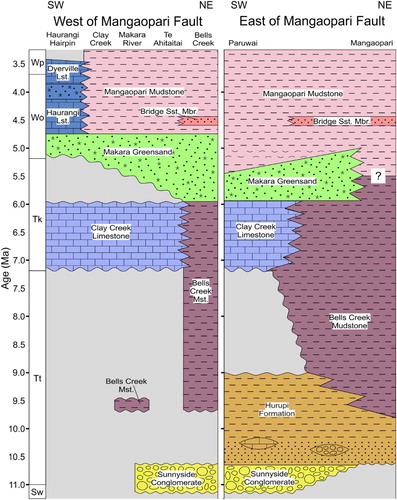
Geological history
The general tectonic and paleoenvironmental trend in the northern Aorangi Range throughout late Miocene and early Pliocene times was one of subsidence (Wells Citation1989a) and increasing oceanicity. However, episodes of accelerated shortening across the plate boundary were accommodated by localised reverse faulting and folding and the growth of contractional structures (Nicol et al. Citation2002), creating local uplift episodes which are superimposed on the regional subsidence trend. Although Antarctic ice sheets were present during the late Miocene and early Pliocene, glacio-eustatic fluctuations in sea level at this time were relatively small (< 50 m) in amplitude (Miller et al. Citation2005) and are not recognised in the rocks described in this paper.
At the end of the Waiauan and beginning of the Tongaporutuan Stage, the Aorangi Range was emergent as a hilly, forested island, ‘Aorangi Island’ (previously referred to as Haurangi Island by Beu Citation1995), separated from the westerly mainland by a proto-Ruataniwha Strait. The Sunnyside Conglomerate indicates the presence of a river system that eroded the uplifted basement rocks. Early in the Tongaporutuan, tectonic subsidence caused the sea to transgress across the entire study area. The Hurupi Formation was deposited in a shallow marine environment in the southern and central parts of the study area. Subsidence was more rapid in the east of the study area, causing the Hurupi Formation to grade vertically and laterally into upper bathyal Bells Creek Mudstone ().
An episode of uplift occurred in the early Tongaporutuan, and is superimposed on the trend of regional subsidence. This uplift is associated locally with movement on the Makara Fault, and is correlated with the regional uplift event identified by Wells (Citation1989a) as occurring at around 10.5–10 Ma.
Following this uplift event, the Sunnyside Conglomerate and Hurupi Formation were eroded from the upthrown side of the Makara Fault. As regional subsidence continued, Bells Creek Mudstone was deposited continuously across the Makara Fault in the later part of the early Tongaporutuan substage. Aorangi Island remained emergent during this time, possibly as a low-lying, swampy and forested island surrounded by relatively deep, sheltered coastal basins or embayments. Pollen and organic matter, washed out to sea from the island, were deposited into oxygen-poor coastal basins along with terrigenous mud, and are preserved in the Bells Creek Mudstone.
A second episode of tectonic shortening and uplift occurred in late Tongaporutuan time. The Mangaopari Fault became active at this time, along with the Huangarua and Martinborough faults (Nicol et al. Citation2002), and possibly also the Ruakokoputuna, Blue Rock and Nikorima faults. The western and central parts of the study area were raised above sea level by folding and reverse movement on the Mangaopari Fault. In the uplifted area, the Bells Creek Mudstone was eroded back to basement level, with the exception of a few localised patches from the base of the unit preserved in depressions in the Torlesse surface. Uplift was less pronounced in the northeast of the study area, where the Mangaopari Fault passed laterally into an anticline, and deposition of Bells Creek Mudstone continued on the anticline’s eastern limb. On the downthrown side of the Mangaopari Fault, a basin formed, which subsequently allowed for the accumulation of > 100 m of Kapitean age limestone and mudstone in the Paruwai area ( and ).
This uplift episode ended near the Tongaporutuan–Kapitean boundary, and regional subsidence continued. In the early Kapitean, Aorangi Island was fringed by shoals and reefs created by basement antiforms which had been uplifted in the late Tongaporutuan. These shoals, reefs and tide-swept, rocky coasts became carbonate factories for the Clay Creek Limestone. Tidal currents and storm events periodically transported shell material and basement-derived gravel from these coastal environments to the outer shelf, where the Clay Creek Limestone was deposited. Finer-grained terrigenous sediment bypassed the shelf, and was deposited at bathyal depths further offshore to the east, forming the uppermost Bells Creek Mudstone ().
Figure 8. Conceptual depositional model of the northern Aorangi Island during the early Kapitean, showing the depositional setting of the Clay Creek Limestone and the partly conformable relationship with the Bells Creek Mudstone. Developed after Kamp et al. (Citation1988) and Caron et al. (Citation2004). View is looking northwest along the range front.
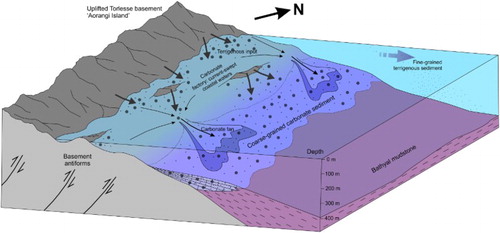
An acceleration of subsidence in the middle of the Kapitean reduced terrigenous sediment supply to much of the study area. The Makara Greensand began to form on the downthrown side of the Mangaopari Fault and eastern limb of the proto-Mangaopari Anticline at this time, as bottom currents winnowed away terrigenous mud and left behind a lag deposit of glauconite, pebbles and foraminifera. This interval of greensand formation was relatively brief at Paruwai, but extended into the early Opoitian in Bells Creek and Mangaopari Stream. In the western and central parts of the study area, greensand did not begin to form until early Opoitian times.
In the late Kapitean, deposition of mudstone resumed at Paruwai. The southwestern corner of the study area remained at shelf depths during this time, and carbonate sediments resembling the Clay Creek Limestone were deposited on this shelf. This was the source area for the shell beds which are interbedded in the lower Mangaopari Mudstone at Paruwai. Also during the latter part of the Kapitean, the shell bed in Bells Creek section A (see ) was deposited as a debris flow from the shelf, accounting for the abundant angular shell fragments and basement-derived pebbles in this subunit.
Throughout the late Kapitean and early Opoitian, the subsidence of Aorangi Island and the surrounding shelf and reefs reduced barriers to oceanic water. Increasing oceanicity in the study area at this time is recorded by the increased abundance of planktic foraminifera in successively younger Opoitian samples from both the Haurangi Hairpin and Bells Creek sections. In early Opoitian times, the study area was tilted, uplifting the far west of the area to shelf depths while the rest of the area continued to subside. The Haurangi Limestone was deposited in the far west of the study area, probably at shallower depths than the Clay Creek Limestone, as the better preservation of shell material in the Haurangi Limestone suggests a shorter transport distance. Across the central and eastern parts of the study area, the Mangaopari Mudstone was deposited as a deep-water lateral equivalent to the Haurangi Limestone. Regional subsidence continued, and by the beginning of the Late Pliocene, Aorangi Island was mostly or entirely submerged (Beu Citation1995; Trewick and Bland Citation2011).
Discussion
Regional correlations
The Sunnyside Conglomerate can be correlated with the Putangirua Conglomerate in Palliser Bay, which was located on the western margin of Aorangi Island in Tongaporutuan times. Another likely correlative for the Sunnyside Conglomerate is the basal conglomerate of the Mangaoranga Formation (sm1 conglomerate; Neef Citation1974) in the western Wairarapa and Tararua districts. Both of these suggested correlatives are terrestrial, fluvial conglomerates which rest unconformably on Torlesse basement, and are conformably overlain by shallow marine sandstone and mudstone of the Hurupi and Mangaoranga formations (e.g. Bates Citation1967; Neef Citation1974; Wells Citation1989b; Begg and Johnston Citation2000). The Kupe’s Sail Beds at Cape Palliser are also thought to correlate with the Sunnyside Conglomerate or Hurupi Formation, although their age is not well constrained (Begg and Johnston Citation2000).
In the Eketahuna district, the Mangaoranga Formation is overlain by the Kaiparoro Formation (Neef Citation1974) which includes the Kaiparoro Limestone Member, a Kapitean limestone of the Te Aute lithofacies which has been previously correlated with the Clay Creek Limestone (Beu Citation1995). The similarity of facies present Eketahuna and the northern Aorangi Range indicates that these areas have similar late Miocene–early Pliocene tectonic and paleoenvironmental histories.
The lateral extent of the Clay Creek Limestone beyond the study area is unknown. It is very likely present in the upper Tauanui River, west of the Haurangi Hairpin and adjacent to the Dry River Fault (approx. NZTopo50 coordinates BQ33/932161 to BQ33/947175). In this area, Bates (Citation1967) described a coarse-grained, cemented limestone unconformably overlying Torlesse basement and containing abundant basement-derived pebbles, which was overlain by greensand and by a finer-grained limestone believed to be Haurangi Limestone. Fittall (Citation1979) mapped isolated outcrops of loosely consolidated Kapitean calcarenite east of Mangaopari Stream, which he assigned to the Clay Creek Limestone. An isolated outcrop of Clay Creek Limestone unconformably overlying Torlesse basement rocks is present at the crest of the Harris Ridge anticline, 4 km southeast of Martinborough (Nicol et al. Citation2002), and the presence of Clay Creek Limestone has been interpreted in a seismic section between the Huangarua and Martinborough faults (Nicol et al. Citation2002). The Clay Creek Limestone is absent at Hinakura Road, northeast of the present study area (Vella and Collen Citation1984) and in Palliser Bay (Bates Citation1967; Vella and Briggs Citation1971), where the lower Kapitean is represented by Bells Creek Mudstone.
Despite being a thin unit, the Makara Greensand is present across much of the Wairarapa region, and is thought to represent a regional disconformity (Cape et al. Citation1990; Crundwell Citation1997). In Crundwell’s (Citation1997) stratigraphic interpretation, the base of the Makara Greensand is treated as the contact between the Palliser and Onoke groups; in sections where the greensand is absent, the contact is marked by the first appearance of Kapitean fossils. However, this study has shown the Makara Greensand to be diachronous and partly Opoitian in age; in the study area, the Makara Greensand is found stratigraphically above the Kapitean Clay Creek Limestone and laterally equivalent mudstone. As the Clay Creek Limestone is also part of the Onoke Group (Vella and Briggs Citation1971; Crundwell Citation1997) this creates a discrepancy.
A further discrepancy exists between the definitions of the Palliser and Onoke groups and the higher-level stratigraphy of Mortimer et al. (Citation2014), who placed the Palliser group within the Maui Supergroup and the Onoke Group within the Pākihi Supergroup. The base of the Pākihi Supergroup is defined as the base of second-order cycle 7 (Pliocene to recent) of King et al. (Citation1999), which likely occurs stratigraphically above both the Miocene Clay Creek Limestone and partly Miocene Makara Greensand.
In light of these findings, it may be necessary to re-evaluate the classification of the Palliser and Onoke groups. We suggest that the base of the Greycliffs Formation, or the base of the Pukenui Limestone, which lie stratigraphically above the Mangaopari Mudstone may be a more appropriate horizon for this boundary. Further lithostratigraphic investigation is needed to determine the appropriate position for this boundary.
Constraints on late Miocene–early Pliocene deformation
The findings from this study provide some constraints for the episode of accelerated shortening identified by Cape et al. (Citation1990) and Nicol et al. (Citation2002, Citation2007) as occurring in latest Miocene or earliest Pliocene time. The partly conformable and partly unconformable relationship between the Bells Creek Mudstone and Clay Creek Limestone, described in this study, was also noted in seismic data by Nicol et al. (Citation2002), who interpreted the Clay Creek Limestone as overlying Torlesse basement and Bells Creek Mudstone with an angular unconformity on the upthrown side of the Huangarua Fault, but showed the Clay Creek Limestone conformably overlying Bells Creek Mudstone further west, on the downthrown side of the Martinborough Fault. This relationship indicates that deformation immediately preceded the deposition of the Clay Creek Limestone, and that erosion in uplifted areas coincided with near-continuous sedimentation in other areas.
Foraminiferal biostratigraphy from this study shows that the base of the Clay Creek Limestone is of latest Tongaporutuan to early Kapitean age. The base of the Kapitean stage has a calibrated age of 7.2 Ma (Raine et al. Citation2015), so the deformational event is inferred to have occurred prior to 7.2 Ma, and deformation is constrained to the older end of the 8 Ma–6 Ma possible age range identified by Nicol et al. (Citation2002).
Furthermore, our interpretation of the Clay Creek Limestone as being deposited in an outer shelf environment, with a water depth in the order of 150 m, provides a constraint on the amount of local uplift during this deformational episode. Previous authors (Abbas Citation1971; Vella and Briggs Citation1971; Beu Citation1995; Begg and Johnston Citation2000) interpreted the pebbly, coarse-grained Clay Creek Limestone as representing a shallow water (< 50 m) depositional environment. Although we recognise that much of the material within the limestone originates in a shallow-water environment, we here interpret it to have been transported and redeposited at much greater depths.
In addition to movement on the Martinborough, Huangarua and Mangaopari faults, this deformational episode caused the growth of folds throughout the Wairarapa region, such as the Chester and Gladstone anticlines (Wells Citation1989a; Cape et al. Citation1990; Nicol et al. Citation2002). Deformation was associated with an erosional event which affected most of Wairarapa, and removed up to 1 km of sediment from the crests of anticlines (Wells Citation1989a). It should be noted that Nicol et al. (Citation2002) also correlated this shortening event with movement on the Makara Fault; however, we associate the Makara Fault with a separate, earlier deformational event, due to its truncation of the Sunnyside Conglomerate but not the overlying Tongaporutuan Bells Creek Mudstone.
A comparison between the stratigraphy described in this study and the stratigraphy described by Wells (Citation1989b) in the Carrington area, 45 km north of the present study area, suggests that the latest Miocene deformation event, although regionally widespread, was not isochronous. At Carrington, the Mangaoranga Formation records a history of subsidence throughout the late Miocene, from Tongaporutuan fluvial conglomerate and shallow marine sandstone to bathyal mudstone of Kapitean age. The Mangaoranga Formation is unconformably overlain by Hururua Limestone, a poorly sorted sandy limestone containing pebbles and cobbles of basement-derived, indurated sandstone and argillite, which is lower Opoitian in age (Wells Citation1989b; Beu Citation1995). This indicates that uplift in the Carrington area took place during Kapitean rather than latest Tongaporutuan times. We suggest that crustal shortening in latest Miocene time progressed from south to north across the Wairarapa, although more detailed study in other areas would be needed to confirm this.
Conclusions
The stratigraphy of the late Miocene–early Pliocene succession in the northern Aorangi Range has been revised, and detailed reinterpretations presented for key units. The Sunnyside Conglomerate is reinterpreted as a terrestrial deposit and shown to contain abundant plant debris, pollen and spores. The stratigraphic relationship between the Bells Creek Mudstone and Clay Creek Limestone is shown to vary from unconformable to conformable and laterally equivalent, and the Clay Creek Limestone is reinterpreted as a unit deposited by mass transport processes into an outer shelf environment. The Makara Greensand is shown to be diachronous, and a new facies has been identified within the Mangaopari Mudstone.
The geological history of the area shows a transition from terrestrial to successively deeper marine environments, punctuated by an episode of deformation and local uplift in the late Miocene. This deformational episode led to localised uplift and erosion of the Bells Creek Mudstone, but mudstone deposition continued in areas not affected by the uplift. The variable relationship between the Bells Creek Mudstone and Clay Creek Limestone indicates that the limestone was deposited after the deformational episode. The base of the Clay Creek Limestone is correlated with the base of the Kapitean stage, at 7.2 Ma (Raine et al. Citation2015), so deformation is inferred to have taken place prior to 7.2 Ma. Differences in stratigraphy and unit ages across the Mangaopari Fault indicate that this fault was active during the late Miocene deformational episode. Overall, the stratigraphic findings presented here provide new constraints on the history of regional tectonic development of the southern Hikurangi Margin during the latest Miocene and early Pliocene.
Supplementary table: All samples collected for this study, with short descriptions, FRF numbers, VUW locality numbers, and location coordinates
Download MS Word (15.1 KB)Supplementary figure: Location of the current study in relation to previous mapping studies in the northern Aorangi Range from 1948 to 1998
Download JPEG Image (639.1 KB)Acknowledgements
We would like to thank Katie Collins (Victoria University of Wellington) and Alan Beu (GNS Science) for their assistance with macrofossil identification; John Collen (Victoria University of Wellington) and Martin Crundwell (GNS Science) for assistance with foraminiferal identification; Matt Ryan and Bill McLea (both Victoria University of Wellington) for pollen identification; and Ben Hines (Victoria University of Wellington) for assistance with measuring sections and in particular for calculating unit thicknesses for Bells Creek Section B. Thanks to Kyle Bland and Colin Mazengarb for their reviews, which have resulted in an improved manuscript.
Thanks especially to Stuart and Caroline Ross (managers) and Harry Baigent at Birch Hill Station, and to Leigh and Wayne Finlayson at Greycliffs Station, for permitting us to work on their land, and to Simon and Liz Beveridge at Mangapari Station for providing accommodation during fieldwork.
Disclosure statement
No potential conflict of interest was reported by the authors.
References
- Abbas SH. 1971. Some aspects of the sedimentology of Pliocene and Miocene rocks north of Aorangi Range, Wairarapa [Unpublished MSc thesis]. Wellington, New Zealand: Victoria University of Wellington; p. 129.
- Andrews PB. 1982. Revised guide to recording field observations in sedimentary sequences. New Zealand Geological Survey Report 102. Lower Hutt: Department of Scientific and Industrial Research; p. 74.
- Bates TE. 1967. The geology of the northern Aorangi Range and part of Palliser Bay, sheet N165 [Unpublished MSc thesis]. Wellington, New Zealand: Victoria University of Wellington; p. 216.
- Begg JG, Johnston MR. (compilers) 2000. Geology of the Wellington area. Institute of Geological & Nuclear Sciences 1: 250 000 geological map 10. Lower Hutt: Institute of Geological & Nuclear Sciences Limited. 1 sheet + 64 p.
- Bertaud-Gandar TLB. 2015. Stratigraphic investigations into the late Miocene-early Pliocene of the northern Aorangi Range, Wairarapa [Unpublished MSc thesis]. Wellington, New Zealand: Victoria University of Wellington; p. 102.
- Beu AG. 1995. Pliocene limestones and their scallops: lithostratigraphy, pectinid biostratigraphy and paleogeography of Eastern North Island Late Neogene Limestone. Institute of Geological & Nuclear Sciences monograph 10. Lower Hutt: Institute of Geological & Nuclear Sciences Limited; p. 243.
- Beu AG, Grant-Taylor TL, de Hornibrook NB. 1980. Te Aute limestone facies. Scale 1:250,000. Wellington: Department of Scientific and Industrial Research. Miscellaneous series map / New Zealand Geological Survey 13 2 maps + 1 booklet.
- Beu AG, Maxwell PA. 1990. Cenoxoic Molusca of New Zealand. New Zealand Geological Survey Paleontological Bulletin 58. Lower Hutt: Department of Scientific and Industrial Research; p. 518.
- Cape CD, Lamb SH, Vella P, Wells PE, Woodward DJ. 1990. Geological structure of Wairarapa Valley, New Zealand, from seismic reflection profiling. Journal of the Royal Society of New Zealand. 20:85–105. doi: 10.1080/03036758.1990.10426734
- Caron V, Nelson CS, Kamp PJJ. 2004. Contrasting carbonate depositional systems for Pliocene cool-water limestones cropping out in central Hawke’s Bay, New Zealand. New Zealand Journal of Geology and Geophysics. 47:697–717. doi: 10.1080/00288306.2004.9515084
- Couper RA. 1948. The geology of the Makara and Ruakokopatuna Valleys, East Wairarapa [Unpublished MSc thesis]. Wellington, New Zealand: Victoria University of Wellington; p. 75.
- Crundwell MP. 1979. The occurrence of glauconite in late Miocene to early Pliocene sediments of the Northern Aorangi Range, Wairarapa [Unpublished BSc (Honours) thesis]. Wellington, New Zealand: Victoria University of Wellington; p. 35.
- Crundwell MP. 1997. Neogene lithostratigraphy of southern Wairarapa. Institute of Geological & Nuclear Sciences Science Report 97/36. Lower Hutt: Institute of Geological & Nuclear Sciences Limited; p. 57.
- Crundwell MP, Beu AG, Cooper RA, Morgans HEG, Mildenhall DC, Wilson GJ. 2004. Chapter 12, Miocene. In: Cooper RA, editor. The New Zealand Geological Timescale. Institute of Geological and Nuclear Sciences Monograph 22. Lower Hutt: Institute of Geological and Nuclear Sciences Limited; p. 165–190.
- Devereux I, Hendy CH, Vella P. 1970. Pliocene and early Pleistocene sea temperature fluctuations, Mangaopari Stream, New Zealand. Earth and Planetary Science Letters. 8:163–168. doi: 10.1016/0012-821X(70)90167-6
- Dobbie WA. 1976. A study of the Clay Creek Limestone, Makara Greensand, and lower Haurangi Limestone formations of the Northern Aorangi Range, Wairarapa [Unpublished BSc (Honours) thesis]. Wellington, New Zealand: Victoria University of Wellington; p. 51.
- Dobkins Jr JE, Folk RL. 1970. Shape development on Tahiti-Nui. Journal of Sedimentary Research. 40(4):1167–1203.
- Eggo AJ. 1979. Biostratigraphic zonation within late Cenozoic Mudstone, Mangaopari Stream, Wairarapa [Unpublished BSc (Honours) thesis]. Wellington, New Zealand: Victoria University of Wellington; p. 35.
- Field BD, Uruski CI, Beu AG, Browne GH, Crampton JS, Funnell RH, Killops SD, Laird M, Mazengarb C, Morhans HEG, et al. 1997. Cretaceous-Cenozoic geology and petroleum systems of the East Coast region, New Zealand. Institude of Geological & Nuclear Sciences monograph 19. Lower Hutt: Institute of Geological & Nuclear Sciences. 2 v.
- Fittall ME. 1979. The geology of an area North of Mangaopari Stream, south-east Wairarapa [Unpublished BSc (Honours) thesis]. Wellington, New Zealand: Victoria University of Wellington; p. 44.
- Green DR. 1981. The stratigraphy, structure, and geological history of late Tertiary strata, Makara River, S. E. Wairarapa [Unpublished BSc (Honours) thesis]. Wellington, New Zealand: Victoria University of Wellington; p. 40.
- Hatfield DI. 1981. The geology of upper Makara Valley, S. E. Wairarapa [Unpublished BSc (Honours) thesis]. Victoria University of Wellington, Wellington, New Zealand; p. 37.
- Hayward BW, Grenfell H, Sabaa A, Neil H, Busaz M. 2010. Recent New Zealand deep-water benthic foraminifera: taxonomy, ecologic distribution, biogeography, and use in paleoenvironmental assessment. GNS Science monograph 26. Lower Hutt: GNS Science; p. 363.
- Hines BR, Kulhanek DK, Hollis CJ, Atkins CB, Morgans HEG. 2013. Paleocene-Eocene stratigraphy and paleoenvironment at Tora, Southeast Wairarapa, New Zealand. New Zealand Journal of Geology and Geophysics. 56(4):243–262. doi: 10.1080/00288306.2013.836112
- Kamp PJJ, Harmsen FJ, Nelson CS, Boyle SF. 1988. Barnacle-dominated limestone with giant cross-beds in a non-tropical, tide-swept, Pliocene forearc seaway, Hawke's Bay, New Zealand. Sedimentary Geology. 60:173–195. doi: 10.1016/0037-0738(88)90118-2
- Kennett JP, Watkins ND, Vella P. 1971. Paleomagnetic chronology of Pliocene-Early Pleistocene climates and the Plio-Pleistocene boundary in New Zealand. Science. 171:276–279. doi: 10.1126/science.171.3968.276
- King LC. 1933. Tertiary molluscan faunas from the southern Wairarapa. Transactions of the New Zealand Institute. 63:334–354.
- King PR, Naish TR, Browne GH, Field BD, Edbrooke SW. (compilers) 1999. Cretaceous to recent sedimentary patterns in New Zealand. Institute of Geological and Nuclear Sciences folio series 1, version 1999.1. Lower Hutt: Institute of Geological and Nuclear Sciences Limited; p. 35. 1 enclosure.
- Lee JM, Begg JG. (compilers) 2002. Geology of the Wairarapa area. Institute of Geological & Nuclear Sciences 1:250,000 geological map 11. Lower Hutt: Institute of Geological and Nuclear Sciences Limited. 1 sheet + 66 p.
- McLean DBG. 1953. The geology of the Haurangi-Stoney Creek Area, South-East Wairarapa [Unpublished MSc thesis]. Wellington, New Zealand: Victoria University of Wellington; p. 135.
- Miller KG, Kominz MA, Browning JV, Wright JD, Mountain GS, Katz ME, Sugarman PJ, Cramer BS, Christie-Blick N, Pekar SF. 2005. The Phanerozoic record of global sea-level change. Science. 310:1293–1298. doi: 10.1126/science.1116412
- Moore PR. 1988. Structural divisions of eastern North Island. New Zealand Geological Survey Record 30. Lower Hutt, New Zealand: Department of Scientific and Industrial Research; p. 24.
- Moore PR, Speden IG. 1984. The Early Cretaceous (Albian) sequence of eastern Wairarapa, New Zealand. New Zealand Geological Survey Bulletin 97. Lower Hutt: Department of Scientific and Industrial Research; p. 98.
- Mortimer N, Rattenbury MS, King PR, Bland KJ, Barrell DJA, Bache F, Begg JG, Campbell HJ, Cox SC, Crampton JS, et al. 2014. High-level stratigraphic scheme for New Zealand rocks. New Zealand Journal of Geology and Geophysics. 57(4):402–419. doi: 10.1080/00288306.2014.946062
- Neef G. 1974. Sheet N153, Eketahuna. Geological map of New Zealand 1:63,360. Wellington, New Zealand, Department of Scientific and Industrial Research.
- Nelson CS, Winefield PR, Hood SD, Caron V, Pallentin A, Kamp PJJ. 2003. Pliocene Te Aute limestones, New Zealand: expanding concepts for cool-water shelf carbonates. New Zealand Journal of Geology and Geophysics. 46:407–424. doi: 10.1080/00288306.2003.9515017
- Nicol A, Mazengarb C, Chanier F, Rait G, Uruski C, Wallace L. 2007. Tectonic evolution of the active Hikurangi subduction margin, New Zealand, since the Oligocene. Tectonics. 26(4). doi:10.1029/2006TC002090.
- Nicol A, Van Dissen R, Vella P, Alloway B, Melhuish A. 2002. Growth of contractional structures during the last 10 m.y. at the southern end of the emergent Hikurangi forearc basin, New Zealand. New Zealand Journal of Geology and Geophysics. 45:365–385. doi: 10.1080/00288306.2002.9514979
- Pettinga JR. 1980. Geology and landslides of the eastern Te Aute district, southern Hawke’s Bay [Unpublished PhD thesis]. Auckland, New Zealand: The University of Auckland; 3 volumes.
- Raine JI, Beu AG, Boyes AF, Campbell HJ, Cooper RA, Crampton JS, Crundwell MP, Hollis CJ, Morgans HEG. 2015. New Zealand Geological Timescale NZGT 2015/1. New Zealand Journal of Geology and Geophysics. 58(4):398–403. doi: 10.1080/00288306.2015.1086391
- Trewick SA, Bland KJ. 2011. Fire and slice: palaeogeography for biogeography at New Zealand's North Island/South Island juncture. Journal of the Royal Society of New Zealand. 42:153–183. doi: 10.1080/03036758.2010.549493
- Vella P. 1954. Tertiary mollusca from south-east Wairarapa. Transcations of the Royal Society of New Zealand. 81(4):539–555.
- Vella P, Briggs W. 1971. Lithostratigraphic names, upper Miocene to lower Pleistocene, northern Aorangi range, Wairarapa. New Zealand Journal of Geology and Geophysics. 14:253–274. doi: 10.1080/00288306.1971.10421923
- Vella P, Collen JD. 1984. Four rhyolitic tuff marker beds, lower Pliocene, Wairarapa, New Zealand. Journal of the Royal Society of New Zealand. 14:133–138. doi: 10.1080/03036758.1984.10418186
- Vella P, Collen JD. 1998. Mcleod Fault: associated monocline and Pliocene angular unconformity, Point Range and Mangaopari Stream, Wairarapa, New Zealand. Journal of the Royal Society of New Zealand. 28:157–164. doi: 10.1080/03014223.1998.9517557
- Wells PE. 1989a. Burial history of late Neogene sedimentary basins on part of the New Zealand convergent plate margin. Basin Research. 2:145–160. doi: 10.1111/j.1365-2117.1989.tb00032.x
- Wells PE. 1989b. Late Neogene stratigraphy of the Carrington area, western Wairarapa, North Island, New Zealand. Journal of the Royal Society of New Zealand. 19:283–303. doi: 10.1080/03036758.1989.10427183