ABSTRACT
Plio-Pleistocene sediments exposed in the dissected hill country of the Lower Pohangina Valley and Manawatu Saddle provide a unique record of sedimentation adjacent to the actively uplifting main axial range, North Island, New Zealand. Located in the eastern Whanganui Basin, which has formed behind the active Hikurangi subduction zone, renowned for one of the most comprehensive Quaternary stratigraphic records in the world. The oldest beds in the succession, unconformably overlying Torlesse Group, comprise Mangapanian (3–2.4 Ma) very fine conglomerate, sandstone and mudstone of the Komako Formation. Subsequent deposition of Konewa Formation continued through to late Nukumaruan time (2.4–1.63 Ma), including large tracts of conglomerate now preserved across the Manawatu Saddle, clearly visible from the Saddle Road. Takapari Formation consists of early Castlecliffian (1.63–1 Ma) sediments characterised by a sudden influx of primary volcanic and reworked volcaniclastic material. Distinctive rhyolitic marker beds and molluscan bio-events provide a means of correlating and dating the succession from the Lower Pohangina Valley across the Whanganui Basin. We present the geology of the Lower Pohangina Valley on a 1:30,000 geological map, accompanying cross section and 3D model. A new stratigraphic framework is presented, integrating work in the Komako District, Pohangina Valley with Whanganui Basin stratigraphy through the application of tephrochronology and biostratigraphy. The Lower Pohangina Valley succession provides evidence for marine conditions prevailing across what is now part of the main axial range of New Zealand from c. 3 to 1.6 Ma. An influx of conglomerate, eroded from Torlesse Group, into the sedimentary record during early Nukumaruan time (2.4 Ma) is attributed to an early phase of tectonic uplift along the North Island Dextral Fault Belt (NIDFB), before closure of the ‘Manawatu Strait’ commenced in early Castlecliffian time (1.63 Ma).
Introduction
Pohangina Valley contains a 500+ m thick Plio-Pleistocene succession located along the western foothills of the Ruahine Range () within the eastern sector of the Whanganui Basin. Mean annual uplift rates of 1.3 mm in the Ruahine Range (Beu et al. Citation1981; Marden Citation1984; Fuller et al. Citation2016) combined with high erosion rates (Mosley Citation1978; Purdie and Brook Citation2006) have led to streams and rivers incising over 150 m into the Plio-Pleistocene sediments and underlying Torlesse Group (Marden Citation1984). Well-preserved fossil fauna and rhyolitic tephra horizons provide a means for basin wide and inter-basin correlation across the southern North Island, New Zealand.
Figure 1. Location map showing the geological mapping boundaries of Carter (Citation1972) in the Komako District and Rees (Citation2015) in the Lower Pohangina Valley. Major regional faults and folds are displayed for reference. PFM = Pohangina Faulted Monocline after Marden (Citation1984). Main roads and urban areas are displayed. Geological data sourced from Rich (Citation1959), Carter (Citation1972), Marden (Citation1984), Beanland (Citation1995) and Rees (Citation2015). Topographic data sourced from LINZ Data Service, Crown Copyright Reserved.
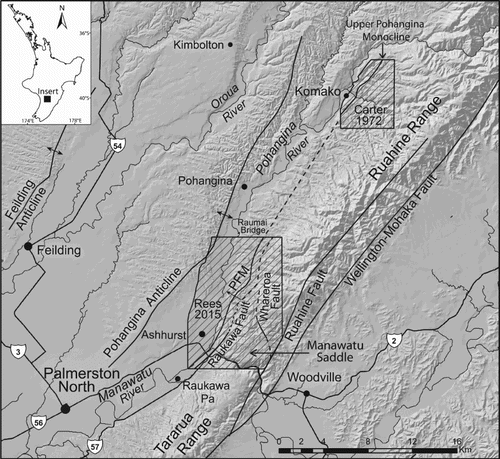
The study area, c. 50 km2, is located 20 km northeast of Palmerston North, near Ashhurst (). The Manawatu Gorge, which coincides with the southern boundary of the study area, is the geographical boundary between the Tararua Range to the south and Ruahine Range to the north. The Raumai Bridge, some 11 km northeast of the Manawatu Gorge, is the approximate northern boundary of the study area.
This paper presents the results of recent geological mapping in the Lower Pohangina Valley, a revision of stratigraphic nomenclature and correlation to the well documented cyclostratigraphy of the Whanganui Basin (Te Punga Citation1952; Fleming Citation1953; Abbott Citation1994; Abbott et al. Citation2005; Naish et al. Citation2005; Pillans et al. Citation2005). Results from the study are discussed revealing part of the Quaternary geological history of the study area.
Geological setting
The Lower Pohangina Valley and Manawatu Saddle contain a unique sedimentary succession straddling a structural low across the main axial range of the lower North Island, New Zealand. This succession represents the eastern most occurrence of Whanganui Basin strata recorded to date, part of a sedimentary basin containing one of the most comprehensive onland Quaternary stratigraphic records in the world. In the Lower Pohangina Valley sedimentary preservation occurred within a localised depocentre adjacent to the actively rising proto-axial range, hosting a wide range of fossil fauna and recording both the early eruptive history of the Taupo Volcanic Zone (TVZ) and interplay between sedimentation, accommodation space and tectonics.
Whanganui Basin is located in a back-arc position relative to the modern interface between the Australian and Pacific plates (Pillans Citation2017). Basin formation is the result of a combination of loading and compressional downwarping of the lithosphere caused by frictional shear between the westward dipping subducting Pacific Plate and the overriding Australian Plate (Stern et al. Citation1993). Progressive removal of mantle lithosphere is suggested to have been responsible for uplift north of and subsidence south of the Taranaki–Ruapehu line (Stern et al. Citation2013). Basin formation and geometry has been strongly influenced by faults operating within the upper plate (Nicol and Beavan Citation2003; Lamarche et al. Citation2005). The basin contains a 4000+ m thick Plio-Pleistocene sedimentary succession, including the type sections for the New Zealand Pleistocene and Upper Pliocene stages (Fleming Citation1953; Beu et al. Citation1987; Pillans Citation1991). Regional tilting of the strata has occurred in response to the southward migration of the basin depocentre and associated uplift along the northern basin margins (Naish and Kamp Citation1997; Trewick and Bland Citation2012).
Continental shortening of 1.35 ± 0.35 km has occurred in the Whanganui Basin over the last 5 Ma, driven by oblique convergence along the Hikurangi subduction margin (Lamarche et al. Citation2005). A number of northeast–southwest striking faults and folds run roughly parallel to the main axial range (Anderton Citation1981). These include a series anticlines trending northeast across the Manawatu plains to the west (Melhuish et al. Citation1996; Jackson et al. Citation1998). Pohangina Anticline (), the easternmost of these fold structures, forms the western ridgeline of the Pohangina Valley. Seismic reflection profiles across the Mount Stewart–Halcombe Anticline indicate this structure is underlain by two westward dipping reverse faults 1 km apart and a third reverse fault dipping to the east (Melhuish et al. Citation1996; Nodder et al. Citation2007). It is very likely the Pohangina Anticline, too, is underlain by a similar complex of reverse faults. Many of the fault traces throughout the Whanganui Basin are obscured by overlying late Quaternary and Holocene sediments. The major reverse faults in the area are believed to have been active since the early Pleistocene (Begg et al. Citation2005).
The structure of the Whanganui Basin was initially determined by the geological mapping of Feldmeyer et al. (Citation1943), Te Punga (Citation1952), Fleming (Citation1953) and Lensen (Citation1977). Later seismic reflection profiles showed that the sequence dips gently southward towards a depocentre located offshore (Anderton Citation1981). The emergence of the northern Whanganui Basin has been associated with uplift, offlap and southward migration of the basin depocentre through the Quaternary (Lewis et al. Citation1994; Nicol Citation2011).
During the Plio-Pleistocene the Whanganui and Wairarapa continental shelves were not the discrete basins recognised today but were connected via marine seaways which existed across areas now forming the North Island main axial range (Beu Citation1995; Browne Citation2004). This connectivity means equivalent successions are preserved on either side of the present-day range.
Pohangina Valley contains a Mangapanian to Castlecliffian (3–0.34 Ma) succession. Carter (Citation1972) mapped Plio-Pleistocene sediments in the Komako District, Upper Pohangina Valley (), recognising three formations: Komako, Konewa and Takapari formations. Carter’s formations have been recognised and correlated to the Lower Pohangina Valley in the present study. We further subdivide the formations into members specific to the Lower Pohangina Valley, on the basis of lithology, biostratigraphy and tephrochronology.
The geology of the Pohangina Valley and Manawatu Saddle is included in the New Zealand Geological Survey bulletin for the Dannevirke Subdivision (Lillie Citation1953), Sheet 11 of the 1 : 250,000 Geological Map of New Zealand (Kingma Citation1962) and is covered by the Wairarapa and Hawke’s Bay 1 : 250,000 QMAP sheets (Lee and Begg Citation2002; Lee et al. Citation2011). The Hawke’s Bay and Wairarapa QMAP sheets use Rangitikei, Mangaheia and Onoke groups respectively to refer to the Pliocene–earliest Pleistocene sediments, whilst Maxwell Group and Mangatarata Formation are used for the Pleistocene sediments of the study area.
Several unpublished petroleum reports cover aspects of the study areas geology including Feldmeyer et al. (Citation1943), Laing (Citation1961), Ower (Citation1943) and Turner (Citation1944). Studies specific to East Coast stratigraphy that also cover part of the study area include Beu (Citation1995) and Piyasin (Citation1966). The inclusion and relevance of East Coast studies to this study of Pohangina Valley and Manawatu Saddle means stratigraphic nomenclature from both Whanganui Basin and East Coast Basin are applicable, e.g. Maxwell Group in Hawke’s Bay QMAP and Mangatarata Formation in Wairarapa QMAP.
The crossover in stratigraphic nomenclature is largely the result of the location of the Manawatu Saddle between the Whanganui Basin to the west and East Coast Basin to the east. The purpose of this paper is to document the stratigraphy of the Lower Pohangina Valley, using the stratigraphic framework of Carter (Citation1972) together with new field data to propose a revised stratigraphic nomenclature based on chronohorizons preserved within the Whanganui Basin. It is the authors’ belief that correlating Pohangina Valley to the Whanganui Basin will contribute to understanding New Zealand’s Plio-Pleistocene history. It is suggested here that the North Island Dextral Fault Belt (more specifically the Ruahine Fault) become the ‘line in the sand’ delineating the cross over from east coast to west coast stratigraphic nomenclature, with special provision for merging stratigraphic units between Whanganui and East Coast basins through the use of key marker horizons as stratigraphic unit boundaries.
We show, through the application of tephrochronology, that some of the geological units preserved on the western front of the Manawatu Saddle and Lower Pohangina Valley have a close affinity to the eastern Whanganui Basin succession providing an early record of TVZ eruptive products.
Methods
A 1 : 30,000 geological map of the Lower Pohangina Valley has been compiled in this study. This has involved the incorporation and reinterpretation of previous work by Ower (Citation1943), Rich (Citation1959), Piyasin (Citation1966), Marden (Citation1984), Beanland (Citation1995) and Beu (Citation1995), together with additional field work conducted in the present study between 2013 and 2014. During fieldwork we logged the stratigraphy at key outcrops and used marker horizons to track units laterally across the landscape. Dr Alan Beu (Geological and Nuclear Sciences, Lower Hutt, Wellington) in 2014 and he examined my fossil samples and provided species identification and discussion on paleo-environmental interpretation and age ranges.
Primary tephra and remobilised volcaniclastic material were collected from Pleistocene units and mounted for electron microprobe (EMP) analysis at Victoria University. We have correlated the glass chemistry to known tephras documented within the published geochemical datasets of Pillans et al. (Citation2005). These correlations are made possible through the work of many authors in the Whanganui Basin over the last several decades (Seward Citation1974b, Citation1974a, Citation1976, Citation1979; Shane Citation1991; Pillans et al. Citation1994, Citation2005; Naish et al. Citation1996; Shane et al. Citation1996; Alloway et al. Citation2005). Recognition of key glass chemistries and identification of important bio-events within the sedimentary record of the Lower Pohangina Valley form the basis of stratigraphic subdivision and correlation presented in this paper (, Supplementary Data File A).
We constructed the map within a Geographic Information System (GIS) utilising ArcGIS™ software. A single digital coverage of the study area was produced using database schema outlined by Rattenbury and Heron (Citation1997) and Bland (Citation2006). During map construction historic information was scanned and georeferenced into the geological database to enable a comprehensive interpretation of the local geology and immediate surrounding areas (Feldmeyer et al. Citation1943; Firth and Feldmeyer Citation1943; Ower Citation1943; Te Punga Citation1952; Lillie Citation1953; Rich Citation1959; Kingma Citation1962; Piyasin Citation1966; Marden Citation1984; Beu Citation1995; Brackley Citation1999). The basement geology of the southern part of the Ruahine Range was mapped and subdivided in detail by Marden (Citation1984) who details the basement lithologies of the southern Ruahine Range. In the present study basement is presented as a single unit (Torlesse Composite Terrane); focus is on subdivision of the overlying Plio-Pleistocene succession.
During our field work and map construction, geological cross sections were drawn and balanced to aid with interpretation of the local geology. This process was carried out on paper by hand with ruler and protractor using the principals outlined by Marshak and Woodward (Citation1988). Cross section balancing was undertaken using the equal line method in which the line lengths of all units in the cross section are conserved during deformation, to facilitate the interpretation of structural geometries which show equal line lengths of all units in the restored state. Cross section A–A′ trending east–west across the Manawatu Saddle is shown in to display the sedimentary succession where it attains its maximum thickness demonstrating the stratigraphic relationships outlined in this paper.
We employed Leapfrog Geo™ software (ARANZ Geo Ltd) to build a preliminary 3D geological model of the Lower Pohangina Valley, utilising the structural and lithological data obtained during fieldwork and the interpretations made during map and cross section compilation. Leapfrog uses borehole data imported as comma-separated values (CSV) files as the basis for building 3D meshes (surfaces) and volumes of lithological units. Because of the lack of available borehole information, we decided to use the geological map and cross sections of the Lower Pohangina Valley as the source of data to develop a preliminary 3D geological model.
The base of the model was a digital elevation model, which we imported as an ASCII grid into Leapfrog. Point, polygon and line data were then imported as ESRI shapefiles including the model boundaries, rivers, faults, folds and structural data. Aerial photos and cross sections were imported into Leapfrog as TIFFs before being georeferenced using the in-house importation process. We created CSV geological data sets from our field and cross section data, generating depth to surface profiles for a series of points spread through the study area. Measurements were taken along six cross section lines at even increments recording X, Y, Z coordinates for each point, together with depth to contact measurements. We imported the CSV files into the modelling software, applying lithological descriptions and symbologies to each unit. A geological model was built by setting the model extent to approximate the geological map and cross section boundaries. We then used the imported geological data to create lithological meshes and volumes by spatial interpolation of the point dataset in 3D space using radial basis functions (Cowan et al. Citation2002 Citation2004; Alcaraz et al. Citation2011). We imported faults into the modelling software as 3D lines that were used to create 3D planes with specific attitudes (dip, dip direction) that split the geological model into separate fault blocks. Model development included altering the attitude of and relationships between structures (faults and structural trends) and lithological meshes to obtain a geometry consistent with observations made in the field.
Stratigraphy
Komako Formation
The Komako Formation was defined by Carter (Citation1972) as the group of conglomerate, unfossiliferous brown sandstone and sparsely fossiliferous blue–grey siltstone unconformably overlying the Torlesse Group basement rocks of the Ruahine Range. The designated type section is located in Te Ekaou Stream, Komako District, Pohangina Valley (WGS84 40°06′23.71′′S, 175°53′46.77′′E, elevation 191 m). The Komako Formation can be correlated southward from Komako to the Lower Pohangina Valley, where it crops out extensively throughout the Manawatu Saddle area, reaching a maximum thickness of c. 150 m. The formation thins rapidly northward away from the Manawatu Saddle (Ower Citation1943). On the basis of lithology and faunal content, three members are recognised in the present study area including: the Wharite Member (Wh), the Turanga Mudstone Member (Tu), and the Ngarangi Member (Ng) ().
The well-preserved molluscan macro-fauna collected by Carter (Citation1972), Beanland (Citation1995), this study and micro-fauna collected by Finlayson (Citation1980) indicate that the Komako Formation is Mangapanian to earliest Nukumaruan (3–2.4 Ma) in age. The chronostratigraphic boundary between the Mangapanian (3–2.4 Ma) and Nukumaruan (2.4–1.63 Ma) stages within the Lower Pohangina Valley succession is placed here between the Wharite Member (Komako Formation) and Te Apiti Conglomerate Member (Konewa Formation). This chronostratigraphic break is based on the last occurrence of Crassostrea ingens, Phialopecten triphooki, Phialopecten thomsoni and Polinicies waipipiensis within the sediments mapped here as Komako Formation, together with distinctly Nukumaruan faunal assemblages collected from Konewa Formation in the present study (Supplementary Data File A), including the occurrence of Pelicaria convexa, Tanea zelandica and Alcithoe arabica.
The type locality of the Komako Formation is retained in the present study at Te Ekaou Stream in the Komako District. However, a second key locality is proposed in the upper reaches of an unnamed tributary of the Pohangina River referred to informally as Stallion Stream (WGS84 40°17′20.16′′S, 175°48′52.10′′E, elevation 317 m).
Konewa Formation
The Konewa Formation was defined by Carter (Citation1972) as the dominantly fine-grained marine sediments, including coquina, sandstone and mudstone, cropping out in the Komako District. Carter (Citation1972) defined the base of the Konewa Formation as the lowest coquina unit in the type section, Konewa Stream, Komako District (WGS84 40°03′51.19′′S, 175°56′13.03′′E, elevation 328 m).
Farther south in the Lower Pohangina Valley we interpret the base of the Konewa Formation to lie at the unconformable contact between the Wharite Member of the Komako Formation and the Te Apiti Conglomerate Member of the Konewa Formation. Correlation from Komako to the Lower Pohangina Valley is tentative due to the occurrence of up to 80 m of conglomerate (Te Apiti Conglomerate Member) cropping out on the Manawatu Saddle. This unit is localised and thins rapidly north and south of the Manawatu Saddle area.
The Te Apiti Conglomerate Member signals a substantial change in depositional style within the Lower Pohangina Valley succession, from the muddy sandstone of the Komako Formation to the shallow marine conglomerate, sandstone, mudstone and coquina of the Konewa Formation. The Konewa Formation is split into five members in the present study from oldest to youngest, Te Apiti Conglomerate Member, Makohine Mudstone Member, Tokewa Member, Maungatukurangi Member and Ratanui Limestone Member ().
Carter (Citation1972) correlated the Konewa Formation to Lower Nukumaruan (2.4–2 Ma), through to possible Upper Nukumaruan (2–1.63 Ma) within the upper part of the formation based on biostratigraphy. These ages are confirmed by the fauna we collected and identified from Konewa Formation in the Lower Pohangina Valley (Supplementary data A).
Carter (Citation1972) defined the top of the Konewa Formation as the top of the Mafic Sand Member, a readily recognisable sequence of brown sandstone with interbedded mafic rich sandstone bands and pumice. In the Lower Pohangina Valley, the top of the Konewa Formation is marked by the Ratanui Limestone Member, a 10 m thick coquina (B,C), which marks an unconformity between the dominantly shallow marine sandstone, mudstone, conglomerates and coquina of the Konewa Formation and the sandy mudstone interbedded with lignite, carbonaceous mudstone and tephra of the Waitokanui Member, Takapari Formation. The change from Konewa to Takapari Formation is distinguished by an influx of volcaniclastic pumice, signalling the onset of volcanic activity within the TVZ during early Castlecliffian time (c. 1.63 Ma).
Figure 3. Photographs of parts of the constituent members of the Konewa Formation and Kai Iwi group. A, High energy cross beds and rip up clasts of Potaka Pumice (0.99 Ma), Kaimatira Pumice Sand Formation, Kai Iwi group, unnamed tributary of the Pohangina River, Broadlands Station, Lower Pohangina Valley (WGS84 40°15′21.56′′S, 175°46′59.95′′E, elevation 134 m). B, Ratanui Limestone Member upper contact for Konewa Formation in the Lower Pohangina Valley, spade is approximately 1 m, Unnamed tributary of the Pohangina River informally referred to here as Scrimmys Stream, Broadlands Station, Lower Pohangina Valley (WGS84 40°16′44.78′′S, 175°46′44.78′′E, elevation 112 m). C, Close-up of B, Ratanui Limestone Member displaying common Talochamlys gemmulata, Ostrea chilensis, Calloria inconspicua, Purpurocardia purpurata and Talabrica senecta. Last appearance of Patro undatus in the Lower Pohangina Valley. D, High energy, erosional base of Whareroa Conglomerate Member, Kai Iwi group, note lenses of tephric rich silts (Kaukatea Tephra, 0.9 Ma) eroded from underlying beds incorporated into wavy beds of the pebbly conglomerate. Also, steep dip of the beds 40°@315, 200 m west of the apex of the Pohangina Faulted Monocline characterised by near vertical bedding, Whareroa Stream, Lower Pohangina Valley (WGS84 40°16′48.79′′S, 175°46′51.12′′E, elevation 115 m).
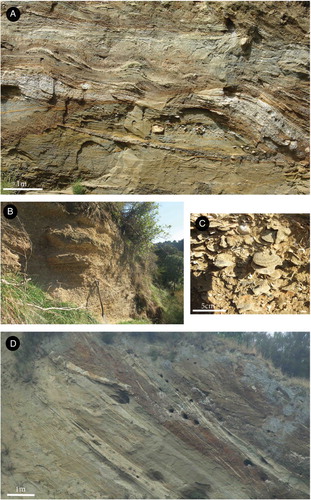
Takapari Formation
The Takapari Formation, which unconformably overlies the Konewa Formation, is defined as a succession of dominantly non-marine pumiceous sandstone and coarse conglomerate (Carter Citation1972). The Takapari Formation has been correlated in the present study to the early Castlecliffian (1.63–1 Ma) sediments mapped in the Lower Pohangina Valley. Here we split the formation into two members, the older Waitokanui Member and the younger Awahou Member based on changes in lithology, biostratigraphy and tephrochronology ().
The base of the Takapari Formation in the Lower Pohangina Valley is the contact between the Ratanui Limestone Member of the Konewa Formation and the base of the Waitokanui Member of the Takapari Formation. The placement of the contact is based on the Ratanui Limestone containing the Waipipian–Nukumaruan (3.7–1.63 Ma) fossil Patro undatus and the Mangapanian–Nukumaruan (3–1.63 Ma) fossil Talabrica senecta. The Waitokanui Member is pumiceous and contains Pakihikura (1.58 ± 0.08 Ma), Ridge (1.56 Ma) and Mangapipi (1.51 ± 0.16 Ma) tephras, indicative of early Castlecliffian (1.63–1 Ma) deposition (Pillans et al. Citation2005). The boundary between the Konewa and Takapari formations is set at the first evidence of terrestrial deposition within the Lower Pohangina Valley sedimentary succession. These terrestrial rocks include lignite interbedded with carbonaceous mudstone containing the intertidal mollusc Austrovenus stutchburyi.
Carter (Citation1972) defined the base of the Takapari Formation as the base of a thick bed of massive, medium-grained, light-brown sandstone in Makawakawa Stream (Komako District, Pohangina, WGS84 40°05′46.28′′S, 175°54′42.85′′E elevation 219 m) and left the top of the formation undefined. The base of the Takapari Formation in the present study is tentatively correlated to Carter’s (Citation1972) stratigraphy where a thick mudstone sequence with common lignite occurs in the lower part of the formation unconformably overlying the sandy Konewa Formation with common coquina, similar to the succession logged in the present study, Lower Pohangina Valley.
We propose a new definition for the top of the Takapari Formation at the Kaimatira Pumice Sand (Fleming Citation1953) indicated by the first occurrence of Potaka Pumice, 0.99 Ma (Pillans Citation2017) within the sedimentary succession. It is also suggested that at the Kaimatira Pumice Sand Formation, Kai Iwi group, Whanganui Basin stratigraphic nomenclature should be adopted (Te Punga Citation1952; Fleming Citation1953; Naish and Kamp Citation1995; Abbott et al. Citation2005). Preserving the local names and lithostratigraphic nomenclature of Carter (Citation1972) in the Pohangina Valley is suggested here to be sensible for all Plio-Pleistocene strata older than 1 Ma, as the sedimentary succession has been strongly influenced by the close proximity of an actively uplifting range. We note here that once sufficient high-level mapping has been completed across the eastern Whanganui Basin, extending from the western Ruahine Range across to the Rangitikei River, it would be useful to subsume Takapari Formation into a broader, regional stratigraphic unit based on early Pleistocene tephra horizons.
Kaimatira Pumice Sand Formation
The Kaimatira Pumice Sand Formation was first recognised in Whanganui District and defined by Fleming (Citation1953) as the basal formation of the Kai Iwi Group. This formation marks the first influx of Potaka Pumice into the Whanganui Basin. The Potaka Pumice is a widespread and voluminous tephra occurring across both the Whanganui and East Coast Basins. The Kaimatira Pumice Sand Formation is dominated by shallow marine deposition, including large thicknesses of laminated pumiceous sandstone and intercalated mudstone beds with less common cross bedded tephric sandstone units (A) (Seward Citation1976).
On the western side of the Whanganui Basin the Superior Oil Company (Feldmeyer et al. Citation1943) mapped the Coutts Creek Horizon, which was later correlated by Fleming (Citation1953) with the Kaimatira Pumice Sand Formation. Farther east the Superior Oil Company (Feldmeyer et al. Citation1943) mapped a horizon they called the Kimbolton Ash. This horizon was later shown to be the Potaka Pumice (Te Punga Citation1952). Te Punga (Citation1952) was the first to introduce the name ‘Potaka Pumice’, and traced the tephra’s extent in the eastern Whanganui Basin. Seward (Citation1976) later demonstrated the Potaka Pumice is part of the Kaimatira Pumice Sand and traced this important chronostratigraphic unit almost across the entire Whanganui Basin.
The basal contact of the Kaimatira Pumice Sand Formation in the Lower Pohangina Valley succession is erosional, characterised by very coarse to granule pumiceous sandstone with large scale cross bedding. The contact is defined by the first occurrence of Potaka tephra chemistry in the sedimentary record within basal transgressive deposits of cycle 34 of the Whanganui Basin cyclostratigraphy.
The upper contact is defined by the occurrence of Kaukatea tephra with an astronomically tuned age of 0.9 Ma (Pillans Citation2017). Kaukatea tephra is associated with condensed transgressive deposits at the base of cycle 35. In the Lower Pohangina Valley it is interpreted as occurring as a 30 cm fine-grained, primary airfall tephra within laminated alternating siltstone and sandstone at the base of the Whareroa Conglomerate Member, sometimes found reworked into basal portions of the conglomerate (D). We note a feature of the Pleistocene tephras in the eastern Whanganui Basin is that the same tephra chemistry can be preserved as both primary airfall tephra and reworked volcaniclastic material within different laterally adjacent depositional environments, considered approximate chronostratigraphic equivalents as a function of rapid lateral facies variation.
Stratigraphic framework
The stratigraphic framework proposed in this paper () has been based on the identification of characteristic tephra chemistry and bio events within the sedimentary record as listed on . Analysis of the Castlecliffian (1.63–0.34 Ma) sedimentology and tephrochronology including presentation of relevant geochemical data can be found in Rees (Citation2015).
Table 1. Stratigraphic nomenclature used in the Whanganui and East Coast Basins ordered from west to east. The stratigraphic nomenclature used in this paper is displayed in the centre column. Note there is a 450 ka unconformity at the base of the Butlers Shell Conglomerate in the coastal Whanganui section. This unconformity is not present to the same extent in the eastern Whanganui Basin where extensive early Pleistocene, volcaniclastic sediments are preserved.
Macrofossil assemblages were collected from fossiliferous units and identified by Dr Alan Beu (pers. comm., 2014) (Supplementary data file A). Biostratigraphy is particularly useful for the Mangapanian–Nukumaruan sediments (3–1.63 Ma) which contain abundant fossiliferous horizons and few tephra marker beds. We collated several macrofossil and microfossil lists from studies in the region to assist with interpretation of the succession of the Lower Pohangina (Carter Citation1972; Finlayson Citation1980; Beanland Citation1995; Beu Citation1995; Brackley Citation1999; McIntyre Citation2002).
The stratigraphic nomenclature of the eastern Whanganui Basin presented in is a compilation of work by Feldmeyer et al. (Citation1943), Te Punga (Citation1952) and Fleming (Citation1953) which was partially refined by Naish and Kamp (Citation1995) and this study. The underpinnings of these stratigraphic refinements are the result of detailed work by many authors in the Whanganui Basin over the last 50 years, including tephrochronology (Seward Citation1974a, Citation1979; Alloway et al. Citation1993, Citation2005; Shane Citation1994; Naish et al. Citation1996; Shane et al. Citation1996; Pillans et al. Citation2005; Pillans Citation2017); magnetostratigraphy (Seward and Lienert Citation1986; Turner and Kamp Citation1990; Naish et al. Citation1996; Turner et al. Citation2005); biostratigraphy (Collen Citation1972; Beu Citation1978, Citation2006, Citation2010, Citation2011, Citation2012; Naish and Kamp Citation1996; Abbott and Carter Citation1997; Beu et al. Citation2004; Carter Citation2005); and astronomical calibration and correlation to the marine oxygen isotope record (Pillans Citation1994; Carter and Naish Citation1998; Naish et al. Citation1998; Abbott et al. Citation2005; Pillans et al. Citation2005; Carter et al. Citation2014).
Geological structures
Pohangina Faulted Monocline
The Pohangina Faulted Monocline (PFM), located between the Ruahine Range and the Pohangina River (), becomes evident as the dip of strata increases from 5–15° to up to 80° west near the true left bank of the Pohangina River (Rich Citation1959; Carter Citation1972). This structure was first recognised as a monocline by Carter (Citation1972) in the true left bank of Makawakawa Stream.
Prior to 1972, the geological structure of the Pohangina Valley between the Ruahine Ranges and the Pohangina River had been interpreted in several different ways. A survey of the Pohangina area (Greenall et al. (Citation1951) and later mapping of the Manawatu Valley (Rich (Citation1959) both interpreted the structure as being a syncline. These two studies identified easterly dipping strata on the western side of the Pohangina River, forming the limb of a syncline. Rich (Citation1959) suggests the Pohangina River occupied the axis of the syncline. Ower (Citation1943), Kingma (Citation1962) and Te Punga (Citation1957) interpreted the structure as a fault. Kingma (Citation1962) mapped the fault as being active in recent times. Carter (Citation1972) reinterpreted the structure as a monocline that changes northward into a thrust fault in the Upper Pohangina Valley. Carter noted no offsets of late Pleistocene river terraces in the Komako District, even where the terraces are crossed by the structure. This led Carter to reject Kingma’s interpretation of the structure being an active fault.
Marden (Citation1984) introduced the term Pohangina Faulted Monocline (PFM) for the monocline which runs along the western margin of the southern Ruahine Range, a term which is adopted in the present study. Carter (Citation1972) and Marden (Citation1984) both interpret the PFM as being controlled by a blind fault in the Torlesse Group basement at very shallow depth.
In the present study, we interpret the structure as a monocline (PFM) laterally passing into fault zones which we map through the Lower Pohangina Valley. The PFM can be traced across the landscape using dip of bedding (A) and correlating between fault zones. The monocline is characterised by steadily increasing dip angles in the bedding of Plio-Pleistocene sediments, which reach maximum angles of up to 80° over an average distance of c. 500 m. West of the fold axis dips reduce again to between 5 and 15°. We interpret the PFM as being formed by thrust movement along the Raukawa Fault. No evidence for late Quaternary fault displacement along the PFM was found in the present study. However, given the close proximity and relationship of the fold with an actively rising axial range it may have been active during late Quaternary times.
Figure 4. Photographs of observed fault planes and dip slopes in the Lower Pohangina Valley. A, Whareroa Conglomerate Member, Kai Iwi group forming a dip slope 35°@280 on Broadlands Station, Lower Pohangina Valley (WGS84 40°15′37.78′′S, 175°47′07.41′′E, elevation 127 m). B, Exposure of a normal fault in an unnamed tributary of the Pohangina River, informally referred to here as Scrimmy’s Stream (). This fault is interpreted to be antithetic to the Raukawa Fault located at depth, Broadlands Station, Lower Pohangina Valley (WGS84 40°16′50.47′′S, 175°46′46.25′′E, elevation 98 m). C, Antithetic fault plane dipping 75° west, associated with the Raukawa Fault, south branch of Whareroa Stream, Broadlands Station, Lower Pohangina Valley, Manawatu (WGS84 40°14′53.57′′S 175°48′40.18′′E, elevation 221 m). Note the amount of offset in this area is such that Takapari Formation is offset beside Komako Formation. D, Te Apiti Conglomerate Member of Konewa Formation forms the ridge coming to the foreground. The high angle dip slope (52°@275°) is formed by movement on the Whareroa Fault located in the far right of this image, Maungatukurangi Stream, Broadlands Station, Lower Pohangina Valley.
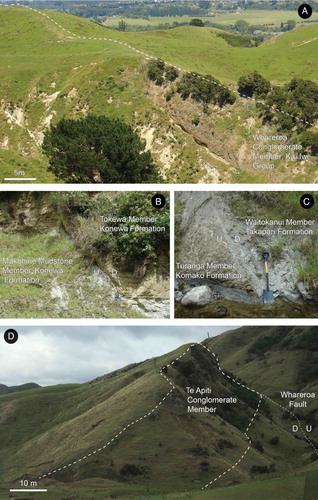
Raukawa Fault
The Raukawa Fault is a high-angle reverse fault trending northeast–southwest across the western flank of the main axial range ( –). Rich (Citation1959) traced the fault along the true left side of the Manawatu River valley, describing an outcrop of the fault on the bank of the Manawatu River near the site of the former Raukawa Pa (). Here the fault plane strikes 30° east and dips 80° southeast. Rich (Citation1959) mapped the Raukawa Fault from the confluence of the Pohangina and Manawatu Rivers 4.8 km southwest to Orrs Road, interpreting the fault as passing into a monocline to the southwest of the Manawatu Gorge. This interpretation was based on the measurement of unusually steep bedding angles of > 30° within the Plio-Pleistocene sediments that typically have dips of < 10° in this area. The steep bedding angles observed also occur in a linear manner across the landscape following the same trend as the Raukawa Fault.
Figure 5. Geological map of the Lower Pohangina Valley. Geological data sourced from Ower (Citation1943), Rich (Citation1959), Piyasin (Citation1966), Marden (Citation1984), Beanland (Citation1995) and Beu (Citation1995). Topographic data sourced from LINZ Data Service, Crown Copyright Reserved.
Figure 6. Geological cross section A–A′ trending west–east across the Lower Pohangina Valley. Geological data sourced from Rich (Citation1959), Marden (Citation1984) and Rees (Citation2015). Topographic data sourced from LINZ Data Service, Crown Copyright Reserved.
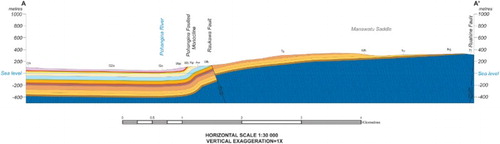
Figure 7. Preliminary 3D geological model of the Lower Pohangina Valley looking northeast. Deeply incised and eroded Plio-Pleistocene coverbeds unconformably overly Torlesse Group, exposed by uplift and erosion adjacent to the axial range. Note this is a schematic representation of the geology displayed on the 1:30,000 geological map and interpreted subsurface geology from cross section analysis. Geological data sourced from Ower (Citation1943), Rich (Citation1959), Piyasin (Citation1966), Marden (Citation1984), Beanland (Citation1995), Beu (Citation1995) and Rees (Citation2015). Topographic data sourced from LINZ Data Service, Crown Copyright Reserved. Model constructed using LeapfrogTM software developed by ARANZ Geo.
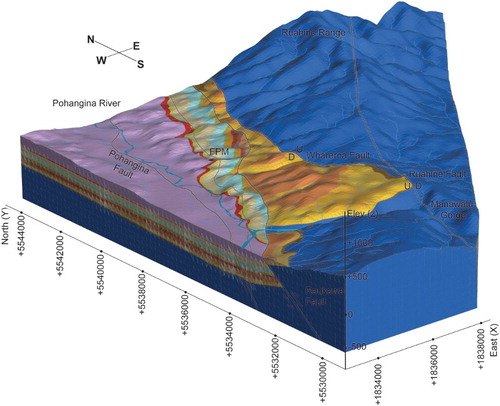
In the present study, the Raukawa Fault is traced a further 700 m from the confluence of the Manawatu and Pohangina rivers northeast toward Te Apiti wind farm (). Beyond here, the fault trace becomes obscured and the location of the fault is based on the correlation between isolated fault surface exposures and dip measurements taken from the Plio-Pleistocene beds. Two unnamed normal faults, with offsets of 2–5 m, are mapped on the Saddle Road and in an unnamed tributary of the Pohangina River referred to informally as Scrimmy’s Stream ( and ).
We see evidence of vertical offset along the Raukawa Fault including displaced strata in the Manawatu Saddle area, where Konewa Formation juxtapose Takapari Formation and Kai-Iwi group sediments. In cross section A–A′ (), a vertical displacement of 100 m is present on the Raukawa Fault representing the maximum displacement measured during this study.
We infer that the Raukawa Fault continues in a northeast direction from its exposure in vicinity of the Manawatu River to Whareroa Stream where it once again has surface expression. The fault exposure in Whareroa Stream is a zone of brittle deformation characterised by fractured strata with steep opposing dips. The deformation includes a normal fault dipping 75° west. Here, shallow thrust faulting in basement is inferred to have transferred stress into the overlying Plio-Pleistocene strata. The resulting strain includes fracture zones and normal antithetic faulting (C).
No river terraces were found to have been displaced by the Raukawa Fault in the present study. Therefore, no direct evidence exists of recent rupture along the Raukawa Fault. However, given active faulting occurring along the North Island Dextral Fault Belt (NIDFB) (Beanland Citation1995; Beanland and Haines Citation1998) and ongoing average uplift of 1.3 mm yr−1 within the Ruahine Range (Beu et al. Citation1981; Marden Citation1984; Fuller et al. Citation2016), the Raukawa Fault is classified as potentially active.
We suggest the surface exposure of the Raukawa Fault at the western end of the Manawatu Gorge is the result of local high erosion rates (up to 1215 m3 km−2 per year; Marden Citation1986) combined with down cutting of the Manawatu River, which have stripped away the majority of the Plio-Pleistocene sediments. Farther northeast, the fault is not exposed at the surface and we interpret this is to be due to a thick succession of Plio-Pleistocene sediment which has accommodated the strain by a combination of folding and associated antithetic normal faulting.
Whareroa Fault
The Whareroa Fault is a steep reverse fault which extends from the northern end of the Manawatu Saddle to near the southern branch of Whareroa Stream ( and ). This fault was mapped by Marden (Citation1984) as a 1.5 km long, steep, northwest striking scarp separating Torlesse Group bedrock to the east from gently dipping Plio-Pleistocene marine deposits to the west.
In the present study, the Whareroa Fault is interpreted as an c. 6 km long fault separating Torlesse Group from Plio-Pleistocene sediments. We infer the Whareroa Fault is a splay off the Ruahine Fault near Saddle Road (). Dips measured in this area show opposing directions, suggesting scissor-type displacement with the up-thrown eastern side dipping west to northwest and the downthrown western side dipping south. The fault trace trends northwest–southeast, separating pockets of highly shattered and sheared Torlesse Group. The fault veers sharply to the northeast at Whariti Road, from where it trends northeast–southwest, parallel with the Ruahine Range. The fault trace has no surface expression within the Manawatu Saddle. We interpret this as being due to thick overlying Plio-Pleistocene deposits and common recent landslides. Fault gouge and breccia composed of angular, Torlesse Group clasts are present along the Torlesse Group contact near Maungatukurangi Stream. Torlesse Group in this area is highly shattered and folded and Plio-Pleistocene sediments display dip planes of > 50° formed by offset along the Whareroa Fault (D).
The bedding of the Plio-Pleistocene sediments mapped during the present study to the west and south of the Whareroa Fault have been dragged into moderate to steep attitudes as a result of uplift along the fault. Dips rapidly decrease over short distances west of the Whareroa Fault. No geomorphic features suggest recent fault displacement and no river terraces were found to be displaced where crossed by the fault. Marden (Citation1984) suggests there has been no movement along the Whareroa Fault over the past 50,000 years. This was confirmed during field mapping in the present study, which included mapping of late Quaternary river terraces preserved in the study area.
Ruahine Fault
The Ruahine Fault is part of the NIDFB, running along the eastern side of the North Island’s main axial ranges (Beanland Citation1995). The fault splays off the Wellington–Mohaka Fault near the Manawatu Gorge. The Ruahine Fault trace is poorly preserved due to severe erosion which has worn away evidence of fault displacement in the landscape. Marden (Citation1984) mapped the fault trace mainly on the basis of fault gouge in the Torlesse Group, a marked topographical disruption in the landscape and the presence of fault guided streams.
Hanson (Citation1998) trenched the fault trace off Whitle Road, Puketitiri, Hawke’s Bay, c. 125 km northeast of the Manawatu Saddle, classifying the Ruahine Fault as active. She recognised at least nine fault movements within the last 12,000 years, both from observations of horizontal stream offsets across the trench site and vertical offsets within the strata exposed in the trench. Individual events were interpreted from stratigraphic analysis and dating of both organic material and tephra beds exposed in the trench sites. Approximately three of these faulting events have occurred since the deposition of the Taupo Tephra (1.8 ka) (Hanson Citation1998; Hogg et al. Citation2011). At the Whitle Road site, the Ruahine Fault scarp is evident in the landscape, displacing streamlets. We suggest the lack of surface expression of the Ruahine Fault across the Manawatu Saddle is most likely the result of both extensive erosion and the thick covering of weakly consolidated Plio-Pleistocene rocks prone to landslips. Another contributing factor could be that offset on the fault has not been even; either along the length of the fault trace or through time, with the least amount of movement observed during the neotectonic studies of Beanland (Citation1995) and Hanson (Citation1998) within the Manawatu Saddle area.
Discussion
3D model
A preliminary 3D geological model of the Lower Pohangina Valley () was developed during the present study from data collected during field mapping. The model shows a 3D representation of the presented 1:30,000 geological map and interpreted sub-surface geology of the Lower Pohangina Valley. Because of a lack of borehole data, cross sections were used to take depth to contact measurements and create a point dataset able to be imported into Leapfrog Geo modelling software. Topographic data from LINZ was also imported including an 8 m New Zealand digital elevation model we used as the topographic surface of the 3D geological model. Model construction took place within the implicit modelling environment of Leapfrog Geo software, which generates meshes representative of lithological boundaries based on interpolation between imported data points.
We found during model development that exclusion of minor faults with small surface extents was sometimes necessary to produce an accurate model of the geology observed in the field. The reason for this exclusion was due to an inability of the software to deal with a fault, in the middle of the model, that does not either terminate against another fault (fault interaction) or continue to the model boundary. The continuation of a fault beyond its mapped extent is not always advantageous, however, despite the difficulties encountered it was found that modelling the geology as a series of fault blocks within Leapfrog Geo led to an accurate representation of the data collected in the field.
We modelled the PFM as a structural trend in Leapfrog Geo, creating a flat ellipsoid anisotropy that varies in direction with the mesh. We defined the anisotropy of the structural trend manually and then applied the trend to the stratigraphic units within the model, represented by a series of interpolated meshes. To restrict the quantity of individual fault blocks formed during the modelling process we simplified the complexities observed in the field between the Raukawa Fault and PFM. This allowed us to develop a simple yet suitable model, which accurately displayed the outcrop distribution of units observed in the field.
Outcrop distribution and attitude of the Plio-Pleistocene beds mapped during the present study necessitates an approximately east–west orientated cross fault across the structural low of the axial ranges. This fault would appear to be blind as it is inferred from field relationships rather than surface rupture.
The model is a useful tool alongside traditional cross section techniques; it helps visualise lateral variations across the landscape and the broader architectural and structural relationships. In particular, we employed the ability to rapidly trial inclusion or exclusion of different data sets, test relationships between units and structures and change the attitude or attributes of various elements within the model to consolidate and explore the conceptual geological model of the study area originally developed during preliminary fieldwork.
Paleogeographic interpretation
Piyasin (Citation1966) and Beanland (Citation1995) documented a Waipipian (3.7–3 Ma) to possible Opoitian (5.33–3.7 Ma) aged concretionary mudstone unit overlying Torlesse Group to the east of the present study area, located on the downthrown block of the Ruahine Fault. This unit was mapped as a pocket of Mangatoro Formation by Piyasin (Citation1966) within an unnamed tributary of the Manawatu River containing Mactra (Maorimactra) chrydaea and Austrofusus pagoda, characteristic of a marine shelf environment. We interpret this older localised succession to the east of the present study area as potentially representing the earliest phase of deposition within the Manawatu Strait.
The oldest sediments mapped during the present study are of Mangapanian age (3–2.4 Ma) unconformably overlying the Torlesse Group of the axial range. Evidence from the present study indicates the Manawatu Strait was fully open by at least Mangapanian time (3–2.4 Ma) characterised by near shore to inner most shelf environments as indicated by common occurrence of Tawera subsulcata, Purpurocardia purpurata, Glycyerita tohunga, Aulacomya maoriana and Polinices waipipiensis.
The shallow marine environments in which Komako Formation accumulated were most likely partially protected from the open ocean by small shoals and islands of Torlesse Group basement given the occurrence of Ruditapes largillierti (Supplementary Data File A) together with an apparent source of Torlesse Group providing clasts to the very fine conglomerates of Ngarangi Member. Marine sediments recorded from Kuripapango 100 km northeast of the present study area by Browne (Citation1978, Citation2004) demonstrate that both the Manawatu and Kuripapango straits were open contemporaneously for a time (3–2.4 Ma) connecting Whanganui and East Coast Basins across what is today the main axial range of New Zealand ().
Figure 8. Schematic depiction of the paleogeography for the lower North Island during a sea level highstand in Mangapanian time (2.7 Ma) showing location of Kuripapango, Manawatu and Ruataniwha straits. Data derived from Beu (Citation1995), McIntyre (Citation2002), Bland (Citation2006), Nicol (Citation2011), Trewick and Bland (Citation2012) and Rees (Citation2015). Contains information licensed under the NIWA Open Data License v1.0. Faults derived from the GNS faults database.
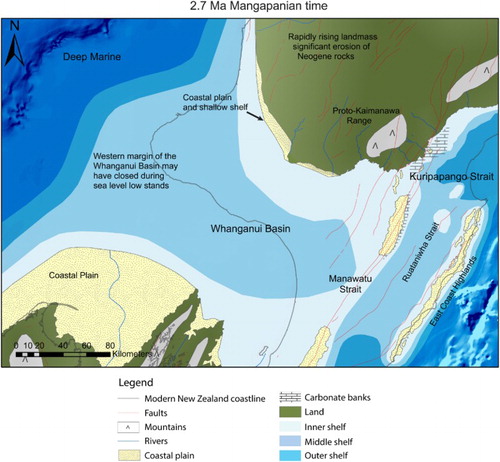
A drastic change in sedimentation occurred during early Nukumaruan time (2.4 Ma) signalled by an influx of conglomerate composed of eroded Torlesse Group (Te Apiti Conglomerate Member, Konewa Formation). We identify delta foresets within Te Apiti Conglomerate Member and interpret a fan delta building out into the western Manawatu Strait during early Nukumaruan time (A). Shallow marine conditions are still evident in the strait as shown by the occurrence of Tanea zelandica, Calloria inconspicua, Ostrea ingens, Paphies australis, Penion sulcata and Purpurocardia purpurata. We interpret the most likely source of eroded Torlesse Group as uplifted shoals or hinterland gradually emerging in the location of the present day Northern Ruahine Range, potentially related to a phase of uplift along the NIDFB. Based on the stratigraphic analysis of marine sediments from Kuripapango and the Hawke’s Bay region (Browne Citation1978, Citation1986, Citation2004; Beu Citation1995; Lee et al. Citation2011) it is unlikely the Torlesse Group clasts were sourced from outcrops either to the east or farther north than the present day northern Ruahine Range due to the presence of intervening seas.
Figure 9. Schematic summary of the inferred environments of deposition in the Lower Pohangina Valley. A, Interpreted environments of deposition within the Manawatu Strait during early Nukumaruan time (2.4 Ma). B, Interpreted environments of deposition in the study area following closure of the Manawatu Strait in early Castlecliffian time (1.63 Ma).
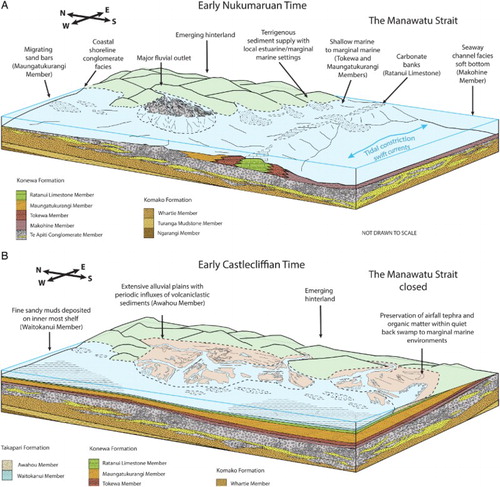
We suggest the general younging of strata and of the major unconformities between Torlesse Group and overlying Plio-Pleistocene sediments from east to west across the Manawatu Saddle recorded by Piyasin (Citation1966), Beanland (Citation1995), McIntyre (Citation2002) and in this study has been driven by movement along the NIDFB forcing a westward migration of the Manawatu Strait depocentre through Mangapanian to late Nukumaruan time (3–1.6 Ma).
The closure of the Manawatu Strait was in progress by late Nukumaruan time (around 1.6 Ma) as regional uplift of the Mount Bruce block to the south in the Wairarapa, in the Wairarapa, led to major changes in the configuration of the Lower North Island including closing off the southern end of the East Coast, Ruataniwha, strait (Beu Citation1995). Evidence in the present study for this closure is indicated by Takapari Formation, characterised by interbedded lignite, carbonaceous mudstone and sudden influxes of volcaniclastic sediments into the sedimentary record. The identification of Pakihikura (1.58 Ma), Ridge (1.56 Ma) and Mangapipi (1.54 Ma) tephras (Pillans Citation2017) within Waitokanui Member, basal Takapari Formation constrain the timing of a major change in depositional setting in the present study area.
Takapari Formation records a change from fully marine into marginal marine depositional environments with a strong terrestrial influence as the coastline gradually moved westward (B). Coinciding with this change in depositional setting, episodic influxes of early Pleistocene primary tephra and reworked volcaniclastic material from TVZ are preserved. The volcaniclastic influxes are interpreted as catastrophic flood deposits within overbank flow and alluvial plain settings as fluvial systems draining the central North Island were inundated with volcaniclastic material transported southwards into the Whanganui Basin. We observe that shallow marine conditions intermittently persisted in the area into Castlecliffian time, as evidenced by the occurrence of Talochalmys gemmulata, Stiracolpus symmetricus, Ostrea chilensis and Amalda murcronata in shellbeds 15 m below Rewa Pumice (1.19 Ma) at Broadlands Station, Lower Pohangina Valley. Marine beds alternate with marginal marine to terrestrial beds through the Castlecliffian succession of the Lower Pohangina Valley interpreted here as being driven by glacio-eustatic cycles within the Whanganui Basin through the correlation based on tephra horizons to the Whanganui Basin cyclostratigraphy (Carter and Naish Citation1998; Pillans et al. Citation2005).
Conclusions
The Plio-Pleistocene sedimentary fill of the Lower Pohangina Valley has been documented through a combination of traditional and digital geological mapping and modelling techniques. Stratigraphic formations described by Carter (Citation1972) in Komako District, Upper Pohangina Valley, have been retained and correlated to the geology of the Lower Pohangina Valley. Marker beds have been identified through their unique geochemistry (Rees Citation2015), facies and faunal content, allowing mapping of geological units across the landscape and correlation of Pohangina geology into the wider Whanganui Basin stratigraphic framework.
The earliest sedimentation within the Lower Pohangina Valley consists of Mangapanian (3–2.4 Ma) very fine conglomerates, sandstone and mudstone of the Komako Formation. Deposition of the overlying Konewa Formation continued through to late Nukumaruan time (2.4–1.63 Ma), including the deposition of up to 80 m of conglomerate now preserved in the Manawatu Saddle area. Takapari Formation consists of early Castlecliffian (1.63–1 Ma) sediments marked by sudden influxes of volcaniclastic material into the Whanganui Basin from the Central North Island. Volcanic material provides an important means of dating horizons within the Castlecliffian (1.63–0.34 Ma) strata of the Lower Pohangina Valley and wider Whanganui Basin. Well-preserved marine fauna throughout the successions are especially important for dating the older Pliocene to earliest Pleistocene sediments, which lack tephra marker beds.
The PFM is traced through the study area by correlating a linear trend of steeply dipping Plio-Pleistocene beds and fault zones. Whareroa and Raukawa reverse faults are mapped trending approximately parallel to the main axial range. Underlying thrust faulting at shallow depth is interpreted to have folded the overlying Plio-Pleistocene sediments and generated fracture zones characterised by antithetic normal faulting, fault gouge and tilted strata.
A 1:30,000 geological map is presented showing the updated stratigraphy and regional structure of the Lower Pohangina Valley. Structural interpretation has been supported through the collation of existing datasets, development of geological cross sections and a preliminary 3D geological model that provides a visual representation of the stratigraphic architecture. Sequential restoration and back-stripping of sedimentary units and structures using cross section balancing and 3D modelling has facilitated consolidation and development of ideas around the local geology and geological history of the study area.
The Pohangina Valley contains an important geological record within one of the easternmost documented areas of the Whanganui Basin. Preservation of tephra and fossil fauna allows the Pohangina Valley to be incorporated into the widely documented Whanganui Basin stratigraphic framework. Intermittent preservation of sediments over the past 3 Ma adjacent to an actively uplifting range has resulted in a unique record of the Lower North Island’s geological history. Including a Mangapanian to early Nukumaruan phase of tectonic uplift signalled by an influx of Torlesse Group clasts and eventual closure of the ‘Manawatu Strait’ by c. 1.6 Ma marked by terrestrial deposition associated with early Pleistocene volcaniclastics.
Supplementary data file A
Download MS Word (23.5 KB)Acknowledgements
We thank Alan Beu for help with macrofossil identification and interpretation. Thanks to Greg Browne and Bob Stewart for helpful comments on earlier versions of this work. Ian Schipper for his assistance with electron microprobe analysis at Victoria University, Anja Mobeis (Massey University) and Magret Damaschke with mount preparation. Special thanks to Matt Irwin (Massey University) and Andrew Cantwell (ARANZ Geo) for software support and especially to ARANZ Geo for making the Leapfrog Geo software available. This manuscript has been greatly improved by the helpful input of Kari Bassett, Andy Nicol and an anonymous reviewer. We thank the residents in the Lower Pohangina Valley for granting land access, in particular Hugh and Judy Akers of Broadlands Station. This paper follows the recommendation from the New Zealand Geographic Board (Ngā Pou Taunaha o Aotearoa) in using the spelling “Whanganui” rather than “Wanganui”.
Disclosure statement
No potential conflict of interest was reported by the authors.
Additional information
Funding
References
- Abbott ST. 1994. Sequence stratigraphy, sedimentology and paleoecology of Pleistocene cyclothems in the castlecliff section, Wanganui Basin, New Zealand [Unpublished PhD thesis]. Queensland, Australia: James Cook University of Northern Queensland. 297 p.
- Abbott ST, Carter RM. 1997. Macrofossil associations from mid-Pleistocene cyclothems, castlecliff section, New Zealand: implications for sequence stratigraphy. Palaios. 12(2):188–210. doi: 10.2307/3515306
- Abbott ST, Naish TR, Carter RM, Pillans BJ. 2005. Sequence stratigraphy of the nukumaruan stratotype (Pliocene-Pleistocene, c. 2.08–1.63 Ma), Wanganui Basin, New Zealand. Journal of the Royal Society of New Zealand. 35(1-2):123–150. doi: 10.1080/03014223.2005.9517779
- Alcaraz S, Lane R, Spragg K. 2011. 3d geological modelling using new Leapfrog Geothermal software. Thirty-sixth Workshop on Geothermal Reservoir Engineering: 1-6.
- Alloway BV, Pillans BJ, Carter L, Naish TR, Westgate JA. 2005. Onshore-offshore correlation of Pleistocene rhyolitic eruptions from New Zealand: implications for TVZ eruptive history and paleoenvironmental construction. Quaternary Science Reviews. 24(14-15):1601–1622. doi: 10.1016/j.quascirev.2004.07.026
- Alloway BV, Pillans BJ, Sandhu AS, Westgate JA. 1993. Revision of the marine chronology in the Wanganui Basin, New Zealand, based on the isothermal plateau fission-track dating of tephra horizons. Sedimentary Geology. 82(1-4):299–310 doi: 10.1016/0037-0738(93)90128-R
- Anderton PW. 1981. Structure and evolution of the South Wanganui Basin, New Zealand. New Zealand Journal of Geology and Geophysics. 24(1):39–63. doi: 10.1080/00288306.1981.10422697
- Beanland S. 1995. The North Island dextral fault belt, hikurangi subduction margin, New Zealand [Unpublished PhD thesis]. Wellington, New Zealand: Victoria University. 360 p.
- Beanland S, Haines J. 1998. The kinematics of active deformation in the North Island, New Zealand, determined from geological strain rates. New Zealand Journal of Geology and Geophysics. 41(4):311–323 doi: 10.1080/00288306.1998.9514813
- Begg JG, Palmer A, Gyopari M. 2005. Geological synopsis of the Manawatu-Horowhenua area for a review of the region’s hydrogeology. Institue of Geological and Nucelar Sciences Client Report 2005/172. 22 p.
- Beu AG. 1978. Taxonomy and biostratigraphy of large New Zealand Pliocene pectinidae (phialopecten and mesopeplum). New Zealand Journal of Geology and Geophysics. 21(2):243–269. doi: 10.1080/00288306.1978.10424054
- Beu AG. 1995. Pliocene limestones and their scallops. lithostratigraphy, pectinid biostratigraphy and paleogeography of eastern North Island late Neogene limestone. Institute of Geological and Nuclear Sciences Monograph. 10(68):1–243.
- Beu AG. 2006. Marine Mollusca of oxygen isotope stages of the last 2 million years in New Zealand. part 2. biostratigraphically useful and new Pliocene to recent bivalves. Journal of the Royal Society of New Zealand. 36(4):151–338. doi: 10.1080/03014223.2006.9517808
- Beu AG. 2010. Marine Mollusca of isotope stages of the last 2 million years in New Zealand. part 3. gastropoda (vetigastropoda - littorinimorpha). Journal of the Royal Society of New Zealand. 40(3-4):59–180. doi: 10.1080/03036758.2010.500717
- Beu AG. 2011. Marine Mollusca of isotope stages of the last 2 million years in New Zealand. part 4. gastropoda (ptenoglossa, neogastropoda, heterobranchia). Journal of the Royal Society of New Zealand. 41(1):1–153. doi: 10.1080/03036758.2011.548763
- Beu AG. 2012. Marine Mollusca of the last 2 million years in New Zealand. part 5. summary. Journal of the Royal Society of New Zealand. 42(1):1–47. doi: 10.1080/03036758.2011.559727
- Beu AG, Alloway BV, Pillans BJ, Naish TR, Westgate JA. 2004. Marine Mollusca of oxygen isotope stages of the last 2 million years in New Zealand. part 1: revised generic positions and recognition of warm-water and cool-water migrants. Journal of the Royal Society of New Zealand. 34(2):111–265. doi: 10.1080/03014223.2004.9517766
- Beu AG, Browne GH, Grant-Taylor TL. 1981. New chlamys delicatula localities in the central North Island and uplift of the ruahine range. New Zealand Journal of Geology and Geophysics. 24(1):127–132. doi: 10.1080/00288306.1981.10422705
- Beu AG, Edwards AR, Pillans BJ. 1987. A review of New Zealand Pleistocene stratigraphy, with emphasis on the marine rocks. In Proceedings of the first international colloquium on Quaternary stratigraphy of Asia and the pacific areas: 250–269.
- Bland KJ. 2006. Analysis of the central hawke's Bay sector of the late Neogene forearc basin, hikurangi margin, New Zealand [Unpublished PhD thesis]. Hamilton, New Zealand: University of Waikato. 289 p.
- Brackley H. 1999. The stratigraphy and environments of deposition of early-mid Pleistocene sediments of the pohangina region, Eastern Wanganui Basin, New Zealand [Unpublished MSc thesis]. Palmerston North, New Zealand: Massey University. 113 p.
- Browne GH. 1978. Wanganui strata of the mangaohane plateau, northern ruahine range, taihape. Tane. 24:199–210.
- Browne GH. 1986. Basement-cover relationships and tectonic significance of Mt Miroroa, western Hawke's Bay. Journal of the Royal Society of New Zealand. 16(4):381–402. doi: 10.1080/03036758.1986.10416816
- Browne GH. 2004. Late Neogene sedimentation adjacent to the tectonically evolving North Island axial ranges: Insights from Kuripapango, western Hawke's Bay. New Zealand Journal of Geology and Geophysics. 47(4):663–674. doi: 10.1080/00288306.2004.9515082
- Carter RM. 1972. Wanganui Strata of komako district, pohangina valley, ruahine range, manawatu. Journal of the Royal Society of New Zealand. 2(3):293–324. doi: 10.1080/03036758.1972.10421819
- Carter RM. 2005. The status of local “stages” in the New Zealand Pliocene-Pleistocene. New Zealand Journal of Geology and Geophysics. 48:623-639.
- Carter RM, Abbott ST, Naish TR. 2014. Plio-Pleistocene cyclothems from Wanganui Basin, New Zealand: type locality for an astrochronologic time-scale, or template for recognizing ancient glacio-eustacy? Philosophical Transactions of the Royal Society A: Mathematical, Physical and Engineering Sciences. 357(1757):1861–1872. doi: 10.1098/rsta.1999.0404
- Carter RM, Naish TR. 1998. A review of Wanganui Basin, New Zealand: global reference section for shallow marine, plio-Pleistocene (2.5-0 Ma) cyclostratigraphy. Sedimentary Geology. 122(1-4):37–52. doi: 10.1016/S0037-0738(98)00097-9
- Collen JD. 1972. New Foraminifera from the Pliocene and Pleistocene of the Wanganui Basin, New Zealand. Journal of the Royal Society of New Zealand. 2(3):373–382. doi: 10.1080/03036758.1972.10421822
- Cowan EJ, Beatson RK, Fright WR, McLennan TJ, Mitchell TJ. 2002. Rapid geological modelling. Applied structural geology for mineral exploration and mining, international symposium(September): 23-25.
- Cowan EJ, Lane RG, Ross HJ. 2004. Leapfrog's implicit drawing tool: a new way of drawing geological objects of any shape in 3D. Innovations in Coal and Metallifeous Mining Geology Bulletin. 41:23–25.
- Feldmeyer AE, Jones BC, Firth CW, Knight J. 1943. The geology of the Palmerston- Wanganui Basin, “West side”, North Island, New Zealand. Unpublished Report of the Superior Oil Company of New Zealand, Petroleum Report Series 171, Ministry of Economic Development, Wellington, New Zealand. 46 p.
- Finlayson LJ. 1980. The geology of the northern Pohangina Valley [Unpublished thesis]. New Zealand: Victoria University of Wellington. 180 p.
- Firth GH, Feldmeyer AE. 1943. The geology of the Pahiatua-Dannevirke Basin “East Side” North Island. Unpublished Petroleum Report 166, The Superior Oil Company of New Zealand, Ministry of Economic Development, Wellington. 250 p.
- Fleming CA. 1953. The geology of Wanganui subdivision. New Zealand Geological Survey Bulletin. 52:1–362.
- Fuller IC, Riedler RA, Bell R, Marden M, Glade T. 2016. Landslide-driven erosion and slope–channel coupling in steep, forested terrain, ruahine ranges, New Zealand, 1946–2011. Catena. 142:252–268. doi: 10.1016/j.catena.2016.03.019
- Greenall AF, Hamilton D, Glass A, Sutherland N, Osborne B. 1951. Soil Conservation Survey of the Pohangina District, North-East Manawatu. Report to the Soil Conservation and Rivers Control Council, Wellington, New Zealand. 28 p.
- Hanson JA. 1998. The neotectonics of the Wellington and ruahine faults between the Manawatu Gorge and Puketitiri, North Island, New Zealand [Unpublished PhD thesis]. Palmerston North, New Zealand: Massey University. 156 p.
- Hogg A, Lowe DJ, Palmer J, Boswijk G, Ramsey CB. 2011. Revised calendar date for the taupo eruption derived by 14C wiggle-matching using a New Zealand kauri 14C calibration data set. The Holocene. 22(4):439–449. doi: 10.1177/0959683611425551
- Jackson J, van Dissen R, Berryman KR. 1998. Tilting of active folds and faults in the Manawatu region, New Zealand: evidence from surface drainage patterns. New Zealand Journal of Geology and Geophysics. 41(4):377–385. doi: 10.1080/00288306.1998.9514817
- Kingma JT. 1962. Geological map of New Zealand, 1:250,000, Sheet 11, Dannevirke. Department of Scientific and Industrial Research, Wellington, New Zealand: 1 sheet.
- Laing AC. 1961. The Geology and petroleum prospects of the Dannevirke area. Unpublished Open File Petroleum Report 318, Ministry of Economic Development, Wellington, New Zealand. 18 p.
- Lamarche G, Proust JN, Nodder SD. 2005. Long-term slip rates and fault interactions under low contractional strain, Wanganui Basin, New Zealand. Tectonics. 24(4):1–30. doi: 10.1029/2004TC001699
- Lee JM, Begg JG, (compilers). 2002. Geology of the Wairarapa area. Institute of Geological & Nucelar Sciences 1:250,000 geological map 11. Lower Hutt, New Zealand. GNS Science. 1 sheet + 66 p.
- Lee JM, Bland KJ, Townsend DB, Kamp PJJ, (compilers). 2011. Geology of the Hawke's Bay area. Institue of Geological & Nuclear Sciences 1:250,000 geological map 8. Lower Hutt, New Zealand. GNS Science. 1 sheet + 93 p.
- Lensen GJ. 1977. Late quaternary tectonic map of New Zealand 1 : 2 ,000,000. New Zealand Geological Survey Miscellaneous Series Map 12.
- Lewis KB, Carter L, Davey FJ. 1994. The opening of cook strait: interglacial tidal scour and aligning basins at a subduction to transform plate edge. Marine Geology. 116:293–312. doi: 10.1016/0025-3227(94)90047-7
- Lillie AR. 1953. Geology of the dannevirke subdivision. New Zealand Geological Survey Bulletin. 46:1–156.
- Marden M. 1984. Geology and its relationship to erosion in the southern ruahine range, North Island, New Zealand [Unpublished PhD thesis]. Palmerston North, New Zealand: Massey University. 386 p.
- Marden M. 1986. New Zealand theses in earth sciences. New Zealand Journal of Geology and Geophysics. 34(1):139. doi: 10.1080/00288306.1986.10427529
- Marshak S, Woodward N. 1988. Introduction to cross section balancing. In: Marshak S, Mitra G, editors. Basic methods of structural geology. Englewood Cliffs, New Jersey, USA: Prentice Hall; p. 303–332.
- McIntyre AP. 2002. Geology of mangapanian (late Pliocene) strata, Wanganui Basin: lithostratigraphy, paleontology and sequence stratigraphy [Unpublished PhD thesis]. Hamilton, New Zealand: Waikato University. 283 p.
- Melhuish A, van Dissen R, Berryman KR. 1996. Mount stewart-halcombe anticline: A look inside a growing fold in the Manawatu region, New Zealand. New Zealand Journal of Geology and Geophysics. 39:123–133. doi: 10.1080/00288306.1996.9514699
- Mosley MP. 1978. Erosion on the south-eastern ruahine range: its implication for downstream river control. New Zealand Journal of Forestry. 23(1):20–48.
- Naish TR, Abbott ST, Alloway BV, Beu AG, Carter RM, Edwards AR, Journeaux TD, Kamp PJJ, Pillans BJ, Saul G, et al. 1998. Astronomical calibration of a southern hemisphere plio-Pleistocene reference section, Wanganui Basin, New Zealand. Quaternary Science Reviews. 17(8):695–710. doi: 10.1016/S0277-3791(97)00075-9
- Naish TR, Field BD, Zhu H, Melhuish A, Carter RM, Abbott ST, Edwards S, Alloway BV, Wilson GS, Niessen F, et al. 2005. Integrated outcrop, drill core, borehole and seismic stratigraphic architecture of a cyclothemic, shallow-marine depositional system, Wanganui Basin, New Zealand. Journal of the Royal Society of New Zealand. 35(1-2):91–122. doi: 10.1080/03014223.2005.9517778
- Naish TR, Kamp PJJ. 1995. Pliocene-Pleistocene marine cyclothems, Wanganui Basin, New Zealand: A lithostratigraphic framework. New Zealand Journal of Geology and Geophysics. 38:223–243. doi: 10.1080/00288306.1995.9514651
- Naish TR, Kamp PJJ. 1996. Foraminiferal depth palaeoecology of late Pliocene shelf sequences and systems tracts, Wanganui Basin, new … sedlmentazy geology foraminiferal depth palaeoecology of late Pliocene shelf sequences and systems tracts, Wanganui Basin, New Zealand. Sedimentary Geology. 110:237–255. doi: 10.1016/S0037-0738(96)00085-1
- Naish TR, Kamp PJJ. 1997. Sequence stratigraphy of sixth-order (41 k.y.) Pliocene–Pleistocene cyclothems, Wanganui Basin, New Zealand: A case for the regressive systems tract. Geological Society of America Bulletin. 109(8):978–999. doi: 10.1130/0016-7606(1997)109<0978:SSOSOK>2.3.CO;2
- Naish TR, Kamp PJJ, Alloway BV, Pillans BJ, Wilson GS, Westgate JA. 1996. Integrated tephrochronology and magnetostratigraphy for cyclothemic marine strata, Wanganui Basin: implications for the Pliocene-Pleistocene boundary in New Zealand. Quaternary International. 34-36:29–48. doi: 10.1016/1040-6182(95)00067-4
- Nicol A. 2011. Landscape history of the marlborough sounds, New Zealand. New Zealand Journal of Geology & Geophysics. 54(2):195–208. doi: 10.1080/00288306.2010.523079
- Nicol A, Beavan J. 2003. Shortening of an overriding plate and its implications for slip on a subduction thrust, central hikurangi margin, New Zealand. Tectonics. 22(6):1070–1070. doi: 10.1029/2003TC001521
- Nodder SD, Lamarche G, Proust J, Stirling M. 2007. Characterizing earthquake recurrence parameters for offshore faults in the low-strain, compressional Kapiti-Manawatu fault system, New Zealand. Journal of Geophysical Research: Solid Earth. 112(12):1–25.
- Ower J. 1943. The Geology of the Manawatu Saddle and the Adjacent Fronts of the Ruahine Range, North Island, New Zealand. Unpublished Report of the Superior Oil Company of New Zealand Ltd. 169 p.
- Pillans BJ. 1991. New Zealand Quaternary stratigraphy: an overview. Quaternary Science Reviews. 10(1984):405–418. doi: 10.1016/0277-3791(91)90004-E
- Pillans BJ. 1994. Direct marine-terrestrial correlations, Wanganui Basin, New Zealand: The last 1 million years. Quaternary Science Reviews. 13(3):189–200. doi: 10.1016/0277-3791(94)90024-8
- Pillans BJ. 2017. Quaternary stratigraphy of Whanganui Basin-a globally significant archive. In: Schulmeister J, editor. Landscape and Quaternary environmental change in New Zealand. Brisbane: Atlantis Press; p. 141–170.
- Pillans BJ, Alloway B, Naish TR, Westgate J, Abbott S, Palmer AS. 2005. Silicic tephras in Pleistocene shallow-marine sediments of Wanganui Basin, New Zealand. Journal of the Royal Society of New Zealand. 35(1-2):43–90. doi: 10.1080/03014223.2005.9517777
- Pillans BJ, Roberts AP, Wilson GS, Abbott ST, Alloway BV. 1994. Magnetostratigraphic, lithostratigraphic and tephrostratigraphic constraints on lower and middle Pleistocene sea-level changes, Wanganui Basin, New Zealand. Earth and Planetary Science Letters. 121:81–98. doi: 10.1016/0012-821X(94)90033-7
- Piyasin S. 1966. Plio-Pleistocene geology of the Woodville area [Unpublished MSc thesis]. New Zealand: Victoria University of Wellington. 145 p.
- Purdie H, Brook MS. 2006. Drainage spacing regularity on a fault-block: A case study from the eastern ruahine range. New Zealand Geographer. 62: 97-104. doi: 10.1111/j.1745-7939.2006.00034.x
- Rattenbury MS, Heron DW. 1997. Revised procedures and specifications for the QMAP GIS, Institute of Geological and Nuclear Sciences science report 97/3, Lower Hutt, New Zealand. 52 p.
- Rees CJ. 2015. The geology of the Lower Pohangina Valley, Manawatu, New Zealand [Unpublished MSc thesis]. Palmerston North, New Zealand: Massey University. 298 p.
- Rich CC. 1959. Late Cenozoic geology of the lower Manawatu valley, New Zealand [Unpublished PhD thesis]. Cambridge (Massachusetts), USA: Harvard University. 188 p.
- Seward D. 1974a. Age of New Zealand Pleistocene substages by fission-track dating of glass shards from tephra horizons. Earth and Planetary Science Letters. 24(2):242–248. doi: 10.1016/0012-821X(74)90102-2
- Seward D. 1976. Tephrostratigraphy of the marine sediments in the Wanganui Basin, New Zealand. New Zealand Journal of Geology and Geophysics. 19(1):9–20. doi: 10.1080/00288306.1976.10423546
- Seward D. 1979. Comparison of zircon and glass fission-track ages from tephra horizons. Geology. 7(10):479–482. doi: 10.1130/0091-7613(1979)7<479:COZAGF>2.0.CO;2
- Seward D 1974b. Some aspects of sedimentology of the Wanganui Basin, North Island, New Zealand [Unpublished PhD thesis]. Victoria University of Wellington. 285 p.
- Seward D, Lienert B. 1986. Magnetic polarity stratigraphy of a plio- Pleistocene marine sequence of North Island, New Zealand. Earth and Planetary Science Letters. 80: 353-360. doi: 10.1016/0012-821X(86)90117-2
- Shane PAR. 1991. Remobilised silicic tuffs in middle Pleistocene fluvial sediments, southern North Island, New Zealand. New Zealand Journal of Geology and Geophysics. 34(4):489–499. doi: 10.1080/00288306.1991.9514485
- Shane PAR. 1994. A widespread, early Pleistocene tephra (Potaka tephra, 1 Ma) in New Zealand: character, distribution, and implications. New Zealand Journal of Geology and Geophysics. 37(1):25–35. doi: 10.1080/00288306.1994.9514598
- Shane PAR, Alloway B, Black T, Westgate J. 1996. Isothermal plateau fission-track ages of tephra beds in an early-middle Pleistocene marine and terrestrial sequence, cape kidnappers, New Zealand. Quaternary International. 34-36:49–53. doi: 10.1016/1040-6182(95)00068-2
- Stern TA, Houseman G, Salmon ML, Evans L. 2013. Instability of a lithospheric step beneath western North Island, New Zealand. Geology. 41(4):423–426. doi: 10.1130/G34028.1
- Stern TA, Quinlan GM, Holt WE. 1993. Crustal dynamics associated with the formation of Wanganui Basin, New Zealand. In: Ballance PF, editor. South Pacific sedimentary basins of the World. Amsterdam: Elsevier; p. 213–223.
- Te Punga MT. 1957. Live anticline in western Wellington. New Zealand Journal of Science and Technology B. 38:433–446.
- Te Punga MT. 1952. The geology of Rangitikei Valley. New Zealand Geological Survey Memoir 8, Wellington, New Zealand. 46 p.
- Trewick SA, Bland KJ. 2012. Fire and slice: palaeogeography for biogeography at New Zealand's North Island/South Island juncture. Journal of the Royal Society of New Zealand. 42:153–183. doi: 10.1080/03036758.2010.549493
- Turner RE. 1944. Geology of northern Wellington and southern Hawkes Bay Provinces. Unpublished Open File Petroleum Report 1225, Ministry of Economic Development, Wellington. 320 p.
- Turner GM, Kamp PJJ. 1990. Palaeomagnetic location of the jaramillo subchron and the matuyama-brunhes transition in the castlecliffian stratotype section, Wanganui Basin, New Zealand. Earth and Planetary Science Letters. 100(1-3):42–50. doi: 10.1016/0012-821X(90)90174-V
- Turner GM, Kamp PJJ, McIntyre AP, Hayton S, McGuire DM, Wilson GS. 2005. A coherent middle Pliocene magnetostratigraphy, Wanganui Basin, New Zealand. Journal of the Royal Society of New Zealand. 35(1-2):197–227. doi: 10.1080/03014223.2005.9517781