ABSTRACT
Detrital zircon U–Pb and muscovite 39Ar/40Ar ages from sandstones in the fluviatile to shallow marine mid-Jurassic Clent Hills Group and Wakaepa Formation of mid-Canterbury, South Island, New Zealand, show characteristic patterns that demonstrate a derivation from local Permian (and possibly Triassic) basement rocks of the Rakaia Terrane (Torlesse Composite Terrane). In the Clent Hills and Wakaepa age data sets, Permian to Triassic zircons form the major age groups, 34% and 58% respectively, but early Paleozoic (20% and 10%) and Precambrian (32% and 28%) zircons also form minor groups. The Precambrian zircons are mainly late Mesoproterozoic and late Neoproterozoic. The muscovite 39Ar/40Ar age patterns reveal no Precambrian ages and the majority (48%) again fall in the Permian–Triassic age range. We postulate a local sediment source in the predominantly Permian Rakaia Terrane basement during Jurassic time, when the terrane was a deformed and stabilised upland block extending principally to the east. This was thus a convergent plate margin against which contemporary marine sedimentary basins developed and consolidated as the Kaweka Terrane (Torlesse Composite Terrane). The provenance of the Canterbury Jurassic cover rocks contrasts markedly with Jurassic-age cover rocks in the Brook Street Terrane. The latter show an almost unimodal pattern reflecting a volcaniclastic environment well-removed from continental sediment sources.
Introduction
The Torlesse Composite Terrane of New Zealand is the most extensive of the terranes that, with the Waipapa and Caples terranes, formed a Late Carboniferous to Early Cretaceous accretionary wedge at the eastern margin of Gondwana (Bishop et al. Citation1985). With companion volcanic arc terranes (Brook Street, Dun Mountain–Maitai and Murihiku), the accretionary wedge makes up an Eastern Province. Petrographic, geochemical and provenance studies of Torlesse sedimentary rocks indicate continental sources of granitoid compositions from beyond the immediate Zealandia Gondwana margin, more probably within the Australian or Antarctic sectors to the west (Bradshaw and Andrews Citation1973; MacKinnon Citation1983; Adams and Kelley Citation1998; Roser and Korsch Citation1999; Cawood et al. Citation1999; Pickard et al. Citation2000; Adams et al. Citation2007). However, there was also early advocacy for at least a local source to the east (Bradshaw and Andrews Citation1973; Andrews et al. Citation1976) and we report here detrital zircon and muscovite age patterns from Jurassic sandstones in the Clent Hills Group and Wakaepa Formation in the South Island that address this idea and its relevance to the Jurassic continental margin in southern Zealandia.
Geological outline
The Torlesse Composite Terrane comprises a Permian–Triassic Rakaia Terrane, a Jurassic Kaweka Terrane and an Early Cretaceous Pahau Terrane distributed across eastern and southern North Island, and the Marlborough and Canterbury regions of the South Island (Bishop et al. Citation1985; Adams et al. Citation2009, Citation2011) (). The southernmost part of the Rakaia Terrane extends into the Otago Schist (Mortimer and Roser Citation1992). In addition, within the Rakaia Terrane there are small enclaves (microterranes) of volcanic rocks, cherts, limestones and conglomerates of Late Carboniferous (Kakahu Terrane, Canterbury and Blue Mountain, Otago) and Permian (Akatarawa Terrane, Canterbury) age.
Figure 1. Basement terranes within the Eastern Province of southern Zealandia, showing principally the Permian to Early Cretaceous accretionary wedge in Canterbury and Marlborough, South Island, New Zealand. B, Barretts Formation; MCD, MacDonald Downs locality, sample MCD1.
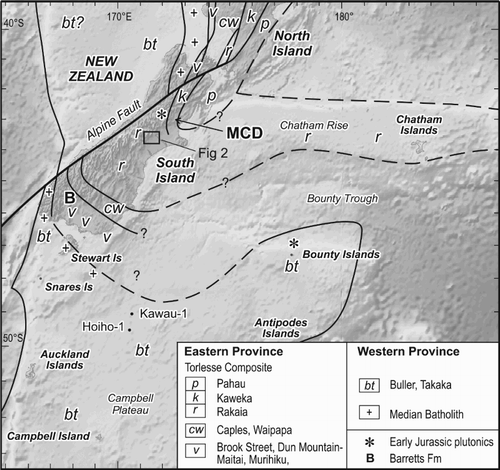
In the north and west of the Rakaia Terrane, in Wellington, Marlborough and Canterbury, marine mass-flow sedimentary rocks predominate (now at low metamorphic grade) – these are mainly mid-fan to distal turbidites in which conglomerates are very rare (Andrews et al. Citation1976; Begg and Johnston Citation2000; Wandres et al. Citation2004a, Citation2004b). To the east, in the Pahau Terrane in Canterbury and Marlborough, shallow-water facies are more common with fluviatile and marginal marine units. There are also relatively abundant conglomerates in which clast-size variation suggests derivation from land to the east (Andrews Citation1974; Andrews et al. Citation1976; Bassett and Orlowski Citation2004). Geochemical analyses of Torlesse Composite Terrane rocks indicate first-cycle, recycled-orogen sediment sources of granitoid compositions (Roser and Korsch Citation1999). Volcanic horizons are rare in the Rakaia Terrane but sandstone QFL diagrams indicate minor (< 30%) lithic components that are predominantly (> 50%) volcaniclastic (MacKinnon Citation1983).
Clent Hills Group and Wakaepa Formation in Canterbury
Within the Rakaia Terrane in mid-Canterbury, the Clent Hills Group and Wakaepa Formation are rather unusual, occurring as local units (outcrop areas at c. 10 km2-scale and measurable sections of c. 100–500 m) of Middle–early Late Jurassic fluviatile, estuarine and marine sedimentary rocks (Cox and Barrell Citation2007; Forsyth et al. Citation2008; Raine Citation2016) (). They are cover sequences on the Permian–Triassic Rakaia Terrane basement, locally containing Permian Atomodesma, and Middle–Late Triassic Plicatula and Torlessia (Campbell and Pringle Citation1982). Contacts, where seen, are usually faulted occasionally thrusted, and the zeolite-facies metamorphic grade of the cover contrasts with the prehnite–pumpellyite facies of the basement (Oliver and Keene Citation1989; Cox and Barrell Citation2007; Forsyth et al. Citation2008). Coarse conglomerates have large clasts, up to 1 m in size, mostly of Rakaia-type sandstones but also rare granitoids. The abundance of plant fragments, e.g. leaves, branches, pollen, together with marine bivalves (Inoceramus, Buchia sp.) indicates a fluviatile to shallow marine environment with a sediment source nearby (Oliver et al. Citation1982; Oliver and Keene Citation1989, Citation1990), but current flow directions are variable and inconclusive (Oliver et al. Citation1982). A Middle to Late Jurassic (Temaikan–Puaroan) depositional age-range is indicated (Arber Citation1917; JI Raine personal communication). The Wakaepa Formation is a succession (probably < 100 m) of fossil plant-bearing, unmetamorphosed siltstone and fine sandstone occurring locally at Whitecliffs, near Glentunnel (). Outcrop is poor, without good measurable sections but it appears to be unconformable upon Triassic Rakaia Terrane basement (Arber Citation1917; Cox and Barrell Citation2007; Forsyth et al. Citation2008). Palynomorphs suggest a possible Middle Jurassic–early Late Jurassic depositional age in a lacustrine environment (Raine Citation2016). The Wakaepa Formation and Clent Hills Group are thus regarded as contemporary rock units (Cox and Barrell Citation2007; Forsyth et al. Citation2008).
Figure 2. Geology of mid-Canterbury, New Zealand, showing Rakaia Terrane, Permian–Triassic basement, its Jurassic cover formations (Clent Hills Group and Wakaepa Formation) and overlying Late Cretaceous sedimentary and volcanic units. Sample locations discussed in text are shown.
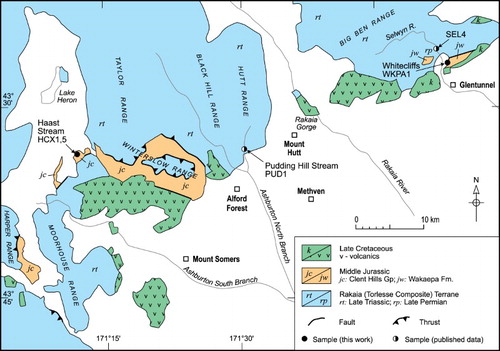
In northern Southland, sedimentary rocks of the Barretts Formation form a similar Jurassic cover sequence, unconformable upon Brook Street Terrane basement (Landis et al. Citation1999). Local outcrops (a few square km) are of fluviatile to shallow marine, quartzofeldspathic to volcaniclastic sandstones, coal and conglomerates (with metre-scale clasts) overlying Permian Takatimu Group and Productus Creek Group basement. Fossil pollen, molluscs and detrital zircons c. 200–170 Ma old, indicate an Early–Middle Jurassic age (Landis et al. Citation1999; Tulloch et al. Citation1999; Adams et al. Citation2002). Two granitoid boulders in conglomerate have Late Triassic U–Pb TIMS zircon ages, 237 ± 3 Ma, 224 ± 3 Ma but in three other cases are in a range to Early Jurassic, c. 180 Ma (Tulloch et al. Citation1999).
The Clent Hills Group and the Wakaepa Formation have more extensive but entirely marine time-correlatives in the Waipapa and Kaweka Terranes. These terranes are exposed principally within the North Island but the Kaweka Terrane does extend south, to the present study area in mid-Canterbury, South Island (Adams et al. Citation2009, Citation2011) ().
Technical details
Sandstone samples (1–2 kg) were collected from the Clent Hills Group (HCX1, HCX5) and Wakaepa Formation (WKPA1), and for comparison, from the Barretts Formation (BARX1). Locality details in the text and tables use grid references from the NZMS260 1:50,000 map series thus allowing simple cross-reference to those in texts accompanying the QMAP 1:250,000, Aoraki and Christchurch geological maps produced by GNS Science (Cox and Barrell Citation2007; Forsyth et al. Citation2008).
Technical details of rock preparation and mineral separation are given in Adams et al. (Citation2013, Citation2017). Recovered zircons (50–150 μm) were usually euhedral or moderately abraded but rarely rounded. Wherever possible, zircon analyses were made close to crystal terminations. ICPMS zircon analytical procedures are as described in Jackson et al. (Citation2004) and are summarised in Supplementary File 1. This also describes the methods of data presentation and recognition of significant age components. Full analytical results: U, Th, Pb isotopic ratios measured U–Pb zircon age data, are listed in Supplementary File 2. Muscovite grains, usually 0.5–1.0 mm maximum dimension, were handpicked from the light specific gravity fractions retained during heavy-liquid separation. Details of the routine preparation procedures for muscovite 40Ar/39Ar age determination used at Curtin University, Perth, are described in Supplementary File 3. The 40Ar/39Ar muscovite analytical and age data are listed in Supplementary File 2. This table lists the accepted data sets from which constituent significant age components (and errors) were then calculated. These data are shown as combined histogram and probability-density diagrams in and . The formats of vertical and horizontal (0–600 Ma) axes are chosen individually to best illustrate the significant zircon age components. Because the geological formations studied in this work vary from conglomerate to siltstone, the U–Pb detrital zircon data sets from the sandstone grades alone cannot, with certainty, provide provenance information from finer zircons that might be preferentially accumulated in the siltstone grades. Although the presence of major zircon age components in the sandstones still retains a provenance significance, the absence of particular (perhaps expected) components must therefore be treated with due caution.
Figure 3. Combined histogram/probability density diagrams of detrital 206Pb/238U zircon and 39Ar/40Ar muscovite ages from Jurassic sandstones in Canterbury: A, Wakaepa Formation, at Whitecliffs WKPA1 (zircon data); B, Clent Hills Group, Haast Creek HCX1 (zircon data); C, Clent Hills Group, Haast Creek HCX5 (muscovite data). Previously published detrital zircon age patterns for Rakaia Terrane sandstones in Canterbury: D, Lower Selwyn River SEL4 (Late Permian, Atomodesma locality); E, Pudding Hill Stream PUD1 (Late Triassic, Plicatula locality) (Pickard et al. Citation2000; Adams et al. Citation2007); F, Kaweka Terrane sandstone MCD1 (Jurassic) at MacDonald Downs (Adams et al. Citation2011). Ages > 600 Ma are stacked at the right-hand margin.
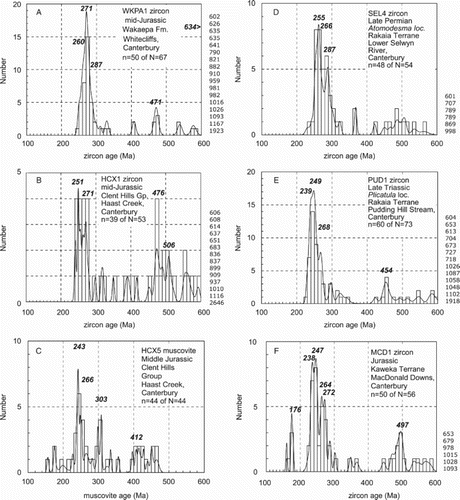
Results
Jurassic sandstones from the Clent Hills Group (HCX1, Haast Creek, grid ref. J36/637345) and Wakaepa Formation (WKPA1, Whitecliffs, grid ref. L35/211501) have broadly similar detrital zircon U–Pb age populations (). A major (30%–60%) well-defined Triassic–Permian age group, c. 240–280 Ma, dominates in both samples, with significant age components within this at c. 270, 260 and 250 Ma. However, a large and more scattered proportion (> 40%) is distributed in an early Paleozoic–Late Precambrian time-range, 440–1200 Ma, in which there are small but significant age components in the early Ordovician–Cambrian, c. 470–500 Ma, and late Neoproterozoic, c. 600–630 Ma. Although both sandstones are demonstrably of mid-Jurassic age (based on paleontology), they contain no Jurassic zircons.
Detrital muscovite 40Ar/39Ar ages in Clent Hills Group sandstone HCX5 (Haast Creek, grid ref. J36/641347, close to HCX1) have a pattern similar to the zircon data (). Within a major Triassic–Permian group (30%) at c. 230–270 Ma, the principal components are 266 and 243 Ma. However, a less prominent (< 10%) group is now confined to the Paleozoic with significant Silurian–Devonian and late Carboniferous components, 412 and 303 Ma respectively. Also, there are four Jurassic ages (156 ± 3, 175 ± 2, 176 ± 5 and 198 ± 7 Ma), two of which are within the depositional age range, Middle to early Late Jurassic, as determined from fossils (Cox and Barrell Citation2007; Forsyth et al. Citation2008; Raine Citation2016). These might be related to contemporary Jurassic magmatism at the Zealandia margin, for example the Pember Diorite, Canterbury (Jongens et al. Citation2009) or the Bounty Islands Granite (Adams et al. Citation2016) (). However, they also might be derived from local schist enclaves within the Rakaia Terrane in Canterbury that yield Jurassic K–Ar white mica ages (Adams Citation2003). The youngest age, 156 ± 3 Ma, clearly postdates the mid-Jurassic depositional age for the Clent Hills Group. This indicates that despite the very low (zeolite facies) metamorphism experienced, there has been unexplained loss of radiogenic argon from some mica grains.
Jurassic sedimentary rocks are recorded from elsewhere within the Eastern Province, including the Brook Street Terrane. In , new data are presented for a Barretts Formation volcaniclastic sandstone BARX1 at Productus Creek, Southland (D44/189789). This has a nearly unimodal Early Jurassic zircon population with age components at 179 ± 2 and 183 ± 2 Ma. There is also a small (25%) scattered group of Triassic–Permian zircons but this is better represented in a previously published data set from the Barretts Formation (BAR2A, Adams et al. Citation2002) that has age components at c. 230 and 202 Ma. (NB: These data are SHRIMP ages with some measurements from inner zircon areas which might thus be older). The Jurassic zircon age components are compatible with the Middle- to Early Jurassic (early Temaikan–late Ururoan, Toarcian–Pliensbachian) fossil-defined age range (Landis et al. Citation1999) and thus record contemporaneous felsic–intermediate volcanism.
Discussion
Provenance of the Clent Hills Group and Wakaepa Formation
Detrital zircon age patterns for the Clent Hills Group (HCX1) and Wakaepa Formation (WKPA1) sandstones resemble those of Rakaia Terrane basement nearby. This is exemplified () by typical data sets for the Rakaia Terrane sandstone from Pudding Hill Stream (PUD1, Triassic, Carnian–Ladinian, Plicatula locality, Pickard et al. Citation2000) and Lower Selwyn River (SEL4, Late Permian, Atomodesma locality, Adams et al. Citation2007). In general, the HCX1 and WKPA1 zircon age patterns, with only a few (< 5%) Late Triassic zircons and more prominent (> 25%) Permian zircon proportions are more typical of Permian rather than Triassic localities across Canterbury, for example at Selwyn River (Adams et al. Citation2007), Kakahu and Pareora (Pickard et al. Citation2000), Kurow (Wandres et al. Citation2004b), and in the Chatham Islands (Adams et al. Citation2008).
The pattern of detrital muscovite ages for Clent Hills Group sample HCX5 is similar to the age patterns seen in Rakaia Terrane sandstones (Adams and Kelley Citation1998), with a major Triassic to Permian group and a minor Early Devonian to Silurian group. In both zircon and muscovite data sets the relative proportions of Triassic–Permian and early Paleozoic groups are similar. Both U–Pb zircon and 40Ar/39Ar muscovite age populations have components in a Middle Permian to earliest Triassic interval, ca 245–270 Ma and to lesser extent in the Early Ordovician, 470–480 Ma. In other cases, however, there is no match, for example: Early Devonian ages, ca 400–440 Ma and Late Carboniferous ages, ca 290–310 Ma, occur in the muscovite populations but are far less common in the zircon data. Conversely, the substantial late-Neoproterozoic zircon populations, 550–700 Ma, have no counterpart in the muscovite data. Adams and Kelley (Citation1998) noted the same detrital zircon and muscovite age features in data sets from the Late Triassic Rakaia Terrane greywackes and suggested that where the two data sets matched, S-type granitoids would represent a realistic provenance. Where the muscovite data had no zircon counterpart then they suggested that an origin for the latter might be in medium-grade pelitic schists eroded from local basement that hosts younger granitoids. These same conclusions would apply to the detrital zircon and muscovite age data sets from the Jurassic Clent Hills Group and Wakaepa Formation, thus demonstrating a reasonably quantitative reworking of Rakaia Terrane basement rock material into the Jurassic cover sediments. This then strongly supports a local provenance for the Jurassic fluviatile and shallow marine sediments, in a stabilised part of the Rakaia Terrane. This was principally of Permian age and was uplifted during latest Triassic–Early Jurassic time (Adams and Graham Citation1996; Adams Citation2003; Adams and Maas Citation2004). The basement of the Permian greywackes is known only from the eastern and southern part of Rakaia Terrane, suggesting that an eroding upland was in this region. The absence of Jurassic zircons implies that there were no contemporary volcanic sources nearby. This is unusual, because in the other Eastern Province terranes (Waipapa, Kaweka), marine Jurassic basins did include sediment inputs from contemporary volcanism. (Adams et al. Citation2009, Citation2011) ().
Table 1. Detrital 206Pb/238U zircon and 40Ar/39Ar muscovite age components Jurassic cover sandstones, Canterbury and Southland, New Zealand.
Jurassic correlatives in the Brook Street, Waipapa and Kaweka terranes
An interior margin for the Wakaepa Formation and Clent Hills Group contrasts markedly with the active-margin Jurassic correlative rocks in the Kaweka, Waipapa and Brook Street terranes. These show petrographic, geochemical and geochronological evidence for first-cycle sedimentary environments into which volcanic arcs contributed considerable felsic–intermediate volcaniclastic detritus including zircons (Adams et al. Citation2009, Citation2011). Although the parent arcs are never seen, this volcanism is always reflected in minor proportions of Jurassic zircons that are coincident with depositional ages. These Jurassic age components accompany the more dominant reworked Triassic and Permian populations (Adams et al. Citation2009, Citation2011). In the Kaweka and Waipapa terranes, their probable sediment sources (including reworked zircons) during the Triassic were from granitoids in the southern section of the New England Orogen and in the Jurassic from a possible extension along the northern Lord Howe Rise (Adams et al. Citation2007, Citation2009, Citation2011).
The Kaweka Terrane in the South Island is largely unfossiliferous but invariably has a minor proportion (< 10%) of mid-Jurassic (c. 176–167 Ma) zircons (Adams et al. Citation2011). For example, in a sandstone near its southernmost end at MacDonald Downs (MCD1, ) c. 50 km northeast of the Clent Hills area, the youngest zircon component is 176 ± 2 Ma (), setting an Early Jurassic maximum depositional age. Although the dominant pre-Jurassic detrital zircon age pattern in MCD1 is similar to that of the Clent Hills examples, it is the Jurassic zircons that provide the significant difference, and these were carried only into the marine basins along the active continental margin.
The Barretts Formation in the Brook Street Terrane () represents a fluviatile to shallow-marine analogue of the Clent Hills Group and Wakaepa Formation. The felsic–intermediate volcaniclastic character of its sandstones is reflected in a dominantly Jurassic zircon age pattern (BARX1) that lacks early Paleozoic–Precambrian zircons (Adams et al. Citation2002). The tectonic environment of the Barretts Formation is thus unlike that of Clent Hills Group or Wakaepa Formation and indicates an isolated provenance within an active offshore volcanic island arc. This developed from Late Triassic to Early Jurassic time and was well-removed from continental sediment sources. Dominated by contemporary volcanic zircon inputs, the age pattern resembles those of Triassic–Jurassic volcaniclastic sandstones in the Murihiku Terrane and a possible connection has been argued (Landis et al. Citation1999; Adams et al. 2007, Citation2007).
A Jurassic upland at the Gondwana margin
The detrital zircon and mica ages from the Clent Hills Group and Wakaepa Formation sandstones confirm the petrographic and sedimentological evidence for a local upland source in the Jurassic. We regard this as being a broad exposed area of principally Permian Rakaia Terrane that extended from about the upper Selwyn River area and (1) eastwards through Banks Peninsula and along the Chatham Rise to the Chatham Islands, where the Chatham Schist has a Rakaia Terrane protolith, in part Late Permian (Adams Citation2003; Adams et al. Citation2008) and (2) to the south in South Canterbury and northeast Otago (Adams et al. Citation2007, Citation2017). This upland would have developed during the deposition of a marine Jurassic basin to the north, i.e. the Kaweka Terrane in North Canterbury, Marlborough and the southern North Island, against the Rakaia Terrane to the south, which was undergoing deformation and uplift. The sediments of the Clent Hills Group and Wakaepa Formation were locally derived and deposited in a fluviatile to shallow-marine environment on the southwestern flank of this upland, whereas the depositional basins of the Kaweka Terrane developed on its northeastern flank.
The complete extent of this upland is uncertain but similar terrestrial sedimentary rocks of Jurassic age probably do occur along the Chatham Rise because reworked Jurassic palynomorphs are found in the oldest cover rocks (Tupuangi Formation, Cenomanian–Turonian, 90–95 Ma) on Pitt Island, Chatham Islands (Campbell et al. Citation1993; Mildenhall Citation1994; JI Raine personal communication). However, no other Jurassic cover rocks are known south of mid-Canterbury because the Rakaia Terrane there passes into the Otago Schist (Adams et al. Citation2017). Extensions offshore to the Bounty Trough and northern Campbell Plateau remain speculative as the only basement outcrops there are Early Jurassic granite on the Bounty Islands, 191 ± 2 Ma (Adams et al. Citation2016).
Conclusions
Detrital zircon and muscovite age patterns in the Clent Hills Group and Wakaepa Formation sandstones in Canterbury indicate an interior continental-margin environment within the Rakaia Terrane in Middle–Late Jurassic time. A local sediment provenance is suggested, eroded from exhumed Rakaia Terrane basement, principally of Permian age, to the east and south. In detail, the zircon populations show significant age components in earliest Triassic–Late Permian (major), Carboniferous–Late Devonian (minor) and Ordovician–Cambrian–latest Neoproterozoic time (minor). A more scattered older Precambrian population is mainly late Mesoproterozoic.
The Barretts Formation sandstone in Southland has an almost unimodal zircon population, c. 180 Ma, recording contemporary mid-Jurassic volcanism within the Brook Street Terrane, probably in an offshore position isolated from continental sources of older zircons.
Supplementary File 1: U-Pb Analytical details, calibration and data treatment
Download MS Word (14.3 KB)Supplementary Data File 2: Clent Hills Group and Wakaepa Formation, Canterbury: U-Pb detrital zircon data
Download MS Excel (383.5 KB)Supplementary File 3: Western Australian Argon Isotope Facility data for Chris Adams
Download MS Word (47.5 KB)Acknowledgments
The authors thank GNS Science colleagues Simon Cox, Jane Forsyth, Nick Mortimer, Ian Raine, Dougal Townsend and the late Malcolm Laird for their advice on mid-Canterbury regional geology and their constructive criticism. The analytical data were obtained using instrumentation funded by DEST Systemic Infrastructure Grants, ARC LIEF, NCRIS/AuScope, industry partners and Macquarie University. Norman Pearson and Will Powell at GEMOC, Macquarie University, Sydney, provided valuable technical assistance throughout the programme. This is contribution 1126 from the ARC Centre of Excellence for Core to Crust Fluid Systems (http://www.ccfs.mq.edu.au) and 1217 in the GEMOC Key Centre (http://www.gemoc.mq.edu.au).
Disclosure statement
No potential conflict of interest was reported by the authors.
Additional information
Funding
References
- Adams CJ. 2003. K-Ar geochronology of Torlesse supergroup metasedimentary rocks in Canterbury, New Zealand. Journal of the Royal Society of New Zealand. 33:165–187. doi: 10.1080/03014223.2003.9517726
- Adams CJ, Barley ME, Maas R, Doyle MG. 2002. Provenance of Permian-Triassic volcaniclastic sedimentary terranes in New Zealand: evidence from their radiogenic isotope characteristics and detrital mineral age patterns. New Zealand Journal of Geology and Geophysics. 45:221–242. doi: 10.1080/00288306.2002.9514970
- Adams CJ, Campbell HJ, Griffin WL. 2007. Provenance comparisons of Permian to Jurassic tectonostratigraphic terranes in New Zealand: perspectives from detrital zircon age patterns. Geological Magazine. 144:701–729. doi: 10.1017/S0016756807003469
- Adams CJ, Campbell HJ, Griffin WL. 2008. Age and provenance of basement rocks of the Chatham Island: an outpost of Zealandia. New Zealand Journal of Geology and Geophysics. 51:245–259. doi: 10.1080/00288300809509863
- Adams CJ, Campbell HJ, Griffin WL. 2017. An Australian provenance for the eastern Otago Schist protolith, South Island, New Zealand: evidence from detrital zircon age patterns and implications for the origin of its gold. Australian Journal of Earth Sciences. 64:703–721. doi: 10.1080/08120099.2017.1362473
- Adams CJ, Campbell HJ, Mortimer N, Griffin WL. 2016. Perspectives on Cretaceous Gondwana break-up from detrital zircon provenance of southern Zealandia sandstones. Geological Magazine. doi:10.1017/S0016756816000285.
- Adams CJ, Graham IJ. 1996. Metamorphic and tectonic geochronology of the Torlesse Terrane, Wellington, New Zealand. New Zealand Journal of Geology and Geophysics. 39:157–180. doi: 10.1080/00288306.1996.9514703
- Adams CJ, Kelley S. 1998. Provenance of Permo-Triassic and Ordovician metagreywacke terranes in New Zealand: evidence from 40Ar/39Ar dating of detrital micas. Geological Society of America Bulletin. 110:422–432. doi: 10.1130/0016-7606(1998)110<0422:POPTAO>2.3.CO;2
- Adams CJ, Maas R. 2004. Rb-Sr age and strontium isotopic characterisation of the Torlesse Supergroup in Canterbury, New Zealand, and implications for the status of the Rakaia Terrane. New Zealand Journal of Geology and Geophysics. 47:201–217. doi: 10.1080/00288306.2004.9515048
- Adams CJ, Mortimer N, Campbell HJ, Griffin WL. 2009. Age and isotopic characterisation of metasedimentary rocks from the Torlesse Supergroup and Waipapa Group in the central North Island, New Zealand. New Zealand Journal of Geology and Geophysics. 52:149–170. doi: 10.1080/00288300909509883
- Adams CJ, Mortimer N, Campbell HJ, Griffin WL. 2011. Recognition of the Kaweka Terrane in northern South Island, New Zealand: preliminary evidence from Rb-Sr metamorphic and U-Pb detrital zircon ages. New Zealand Journal of Geology and Geophysics. 54:291–309. doi: 10.1080/00288306.2011.569728
- Adams CJ, Mortimer N, Campbell HJ, Griffin WL. 2013. The mid-Cretaceous transition from basement to cover within sedimentary rocks in eastern New Zealand: evidence from detrital zircon age patterns. Geological Magazine. 150:455–478. doi: 10.1017/S0016756812000611
- Andrews PB. 1974. Deltaic sediments, Upper Triassic Torlesse Supergroup, Broken Hill, North Canterbury, New Zealand. New Zealand Journal of Geology and Geophysics. 17:271–299. doi: 10.1080/00288306.1974.10430395
- Andrews PB, Speden IG, Bradshaw JD. 1976. Lithological and paleontological content of the Carboniferous-Jurassic Canterbury suite, South Island, New Zealand. New Zealand Journal of Geology and Geophysics. 19:791–819. doi: 10.1080/00288306.1976.10420740
- Arber EAN. 1917. The earlier Mesozoic floras of New Zealand. New Zealand Geological Survey Paleontological Bulletin. 6, 80p.
- Bassett KN, Orlowski R. 2004. Pahau Terrane type locality: fan delta in an accretionary prism trench-slope basin. New Zealand Journal of Geology and Geophysics. 47:603–623. doi: 10.1080/00288306.2004.9515079
- Begg JG, Johnston MR. 2000. Geology of the Wellington area. Institute of geological & nuclear sciences 1: 250 000 geological map 10. Lower Hutt, New Zealand: Institute of Geological & Nuclear Sciences Ltd.
- Bishop DG, Bradshaw JD, Landis CA. 1985. Provisional terrane map of South Island, New Zealand. In Howell DG, editors. Tectonostratigraphic terranes of the Circum-Pacific region. Houston, TX: Earth science series /Circum-Pacific Council for Energy and Mineral Resources 1; p. 515–521.
- Bradshaw JD, Andrews PB. 1973. Geotectonics and the New Zealand geosyncline. Nature Physical Science. 241:14–16. doi: 10.1038/physci241014a0
- Campbell HJ, Andrews PB, Beu AG, Maxwell PA, Edwards AR, Laird MG, Hornibrook NdeB, Mildenhall DC, Watters WA, Buckeridge JS, et al. 1993. Cretaceous-Cenozoic geology and biostratigraphy of the Chatham Islands, New Zealand. Institute of Geological and Nuclear Sciences Monograph. 2:1–269.
- Campbell JD, Pringle IJ. 1982. An association of Torlessia and late Middle-early Upper Triassic fossils at Pudding Hill Stream, Central Canterbury. Journal of the Royal Society of New Zealand. 12:5–10. doi: 10.1080/03036758.1982.10427163
- Cawood PA, Nemchin AA, Leverenz A, Saeed A, Ballance PF. 1999. U/Pb dating of detrital zircons: implications for the provenance record of Gondwana margin terranes. Geological Society of America Bulletin. 111:1107–1119. doi: 10.1130/0016-7606(1999)111<1107:UPDODZ>2.3.CO;2
- Cox SC, Barrell DJA (compilers). 2007. Geology of the Aoraki area. Institute of Geological and Nuclear Sciences 1: 250 000 geological map 15.
- Forsyth PJ, Barrell DJA, Jongens R (compilers). 2008. Geology of the Christchurch area. Institute of geological & nuclear sciences 1: 250 000 geological map 16. Lower Hutt, New Zealand: GNS Science.
- Jackson SE, Pearson NJ, Griffin WL, Belousova EA. 2004. The application of laser ablation-inductively coupled plasma-mass spectrometry (LA-ICP-MS) to in situ U-Pb zircon geochronology. Chemical Geology. 211:47–69. doi: 10.1016/j.chemgeo.2004.06.017
- Jongens R, Tulloch AJ, Spell TL, Rattenbury MS, Begg JG, Lyttle BS. 2009. Pember Diorite—an early Jurassic intrusion in the Rakaia Terrane, Puketeraki range, Canterbury, New Zealand. New Zealand Journal of Geology and Geophysics. 52:37–42. doi: 10.1080/00288300909509876
- Landis CA, Campbell HJ, Aslund T, Cawood PA, Douglas A, Kimbrough DL, Pillai DDL, Raine JI, Willsman A. 1999. Permian-Jurassic strata at Productus Creek, Southland, New Zealand: implications for terrane dynamics at the eastern Gondwanaland margin. New Zealand Journal of Geology and Geophysics. 42:255–278. doi: 10.1080/00288306.1999.9514844
- MacKinnon TC. 1983. Origin of the Torlesse terrane and coeval rocks, South Island, New Zealand. Geological Society of America Bulletin. 94:967–985. doi: 10.1130/0016-7606(1983)94<967:OOTTTA>2.0.CO;2
- Mildenhall DC. 1994. Palynological reconnaissance of early Cretaceous to Holocene sediments, Chatham Islands, New Zealand. Institute of Geological and Nuclear Sciences Monograph. 7:1–204.
- Mortimer N, Roser BP. 1992. Geochemical evidence for the position of the Caples-Torlesse boundary in the Otago Schist, New Zealand. Journal of the Geological Society of London. 149:967–977. doi: 10.1144/gsjgs.149.6.0967
- Oliver PJ, Campbell JD, Speden IG. 1982. The stratigraphy of the Torlesse rocks of the Mt Somers area (S81) mid-Canterbury. Journal of the Royal Society of New Zealand. 12:243–271. doi: 10.1080/03036758.1982.10415348
- Oliver PJ, Keene HW. 1989. Sheet K36AC and part sheet K35 – Mount Somers. Geological map of New Zealand 1:50 000 Map. Wellington: Dept of Scientific and Industrial Research.
- Oliver PJ, Keene HW. 1990. Sheet J36BD and part sheet J35 – Clearwater. Geological map of New Zealand 1:50 000 Map. Wellington: Dept of Scientific and Industrial Research.
- Pickard AL, Adams CJ, Barley ME. 2000. Australian provenances for upper Permian to Cretaceous rocks forming accretionary complexes on the New Zealand sector of the Gondwanaland margin. Australian Journal of Earth Sciences. 47:987–1007. doi: 10.1046/j.1440-0952.2000.00826.x
- Raine JI. 2016. Palynology of the Jurassic Wakaepa Formation, mid-Canterbury, New Zealand. GNS Science Consultancy Report 2016/115/.
- Roser BP, Korsch RJ. 1999. Geochemical characterization, evolution and source of a Mesozoic accretionary wedge: the Torlesse terrane, New Zealand. Geological Magazine. 136:493–512. doi: 10.1017/S0016756899003003
- Tulloch AJ, Kimbrough DL, Landis CA, Mortimer N, Johnston MR. 1999. Relationships between the Brook street Terrane and median tectonic zone (median batholith): evidence from Jurassic conglomerates. New Zealand Journal of Geology and Geophysics. 42:279–293. doi: 10.1080/00288306.1999.9514845
- Wandres AM, Bradshaw JD, Weaver S, Maas R, Ireland TR, Eby N. 2004a. Provenance analisis using conglomerate clast lithologies: a case study from the Pahau Terrane of New Zealand. Sedimentology. 167:57–89.
- Wandres AM, Bradshaw JD, Weaver S, Maas R, Ireland TR, Eby N. 2004b. Provenance of sedimentary Rakaia subterrane South Island, New Zealand: the use of igneous clasts compositions to define the source sedimentology. Sedimentary Geology. 168:193–226.