ABSTRACT
The 2016 MW 7.8 Kaikōura earthquake involved complex rupture of multiple faults for > 170 km, generating strong ground shaking throughout the upper South Island leading to widespread landsliding. As a result of surface fault rupture and landslides, State Highway (SH) 1 and SH70 were blocked, isolating Kaikōura and the surrounding communities, and necessitating evacuations by air and sea. In all these respects, the Kaikōura earthquake can be considered an analogue for a future Alpine Fault earthquake, providing lessons for the necessary emergency response. Landslide blockages primarily occurred where surrounding slopes averaged > 17° and where peak ground acceleration was > 0.43 g, peak ground velocity was > 41 cm/s, or the modified Mercalli intensity was > 7.9. Using a potential future Alpine Fault scenario earthquake, this study identifies locations on other key state highway routes that have similar predictive variables that may, therefore, become blocked in a future earthquake. This suggests that SH6 between Hokitika and Haast, SH73 near Arthur’s Pass, and SH94 south of Milford Sound are all likely to be affected. This will necessitate the evacuation of large numbers of spatially distributed tourists as well as the resupply of isolated local populations. The possibility of bad weather along with a lack of sea ports south of Hokitika will likely make such activities challenging. Contingency planning based on experiences from the Kaikōura earthquake is therefore necessary and likely to prove invaluable following an Alpine Fault earthquake.
Introduction
The 14 November 2016 MW 7.8 Kaikōura earthquake was the largest to strike New Zealand since 2009. The earthquake involved the complex rupture of over 20 previously known and unknown faults in the upper South Island (Hamling et al. Citation2017) and propagated > 170 km north from the epicentre near Waiau (). With rupture initiating at a depth of just 15 km, the earthquake resulted in strong ground shaking (> 1 g) throughout the upper South Island and lower North Island (Kaiser et al. Citation2017). The affected region is steep and mountainous, rising from sea level to > 2500 m in c. 20 km. Consequently, > 10,000 landslides are thought to have occurred resulting in > 190 landslide dams (Massey et al. Citation2018). Despite its mountainous nature, the region is an important transport corridor linking Christchurch, Kaikōura, Blenheim, and Picton via State Highway (SH) 1. Consequently, the earthquake had substantial impact in the form of landslides and surface fault rupture blocking SH1, causing Kaikōura to be cut-off from the rest of the South Island. These blockages resulted in the road being closed for c. 1 year (Mason et al. Citation2017), although closures due to heavy rainfall and further landsliding continue to occur at the time of writing. A full description of the damage caused by the event has been summarised by numerous authors in a Special Issue of the Bulletin of the New Zealand Society for Earthquake Engineering (e.g. Cubrinovski et al. Citation2017; Liu et al. Citation2017; Orense et al. Citation2017; Palermo et al. Citation2017).
Figure 1. Road impacts and various measures of ground shaking from the 2016 MW 7.8 Kaikōura earthquake (Bradley, Razafindrakoto et al. Citation2017) compared with a proposed MW 7.9 Alpine Fault earthquake scenario rupturing from south to north (Bradley, Bae et al. Citation2017, Bradley, Lagrava et al. Citation2017) developed for project AF8 (www.projectaf8.co.nz). A, peak ground acceleration (PGA); B, peak ground velocity (PGV); and C, Modified Mercalli Intensity (MMI) from the Kaikōura earthquake. D, PGA; E, PGV; and F, MMI for the scenario Alpine Fault earthquake. Inset in A gives location of A–C within the upper South Island. Inset in D gives location of D–F within the South Island.
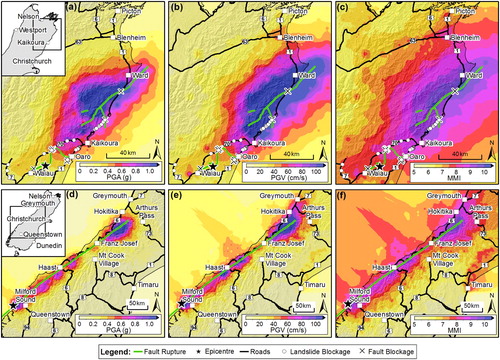
The impacts from the Kaikōura earthquake are, in many respects, a potential analogue for the impacts resulting from a future rupture of the Alpine Fault (). This oblique strike-slip fault runs along the western edge of the Southern Alps for c. 411 km (± 10%) and forms the onshore plate boundary between the Australian and Pacific plates, sustaining a slip rate of 27 ± 5 mm/yr. The Alpine Fault has generated large (MW 8.1 ± 0.2) earthquakes regularly over the past 8000 years, with a relatively invariable average recurrence of c. 341 years or less (Berryman et al. Citation2012; Stirling et al. Citation2012; Howarth et al. Citation2016; Cochran et al. Citation2017). The last known Alpine Fault earthquake occurred in AD 1717 (Yetton Citation1998; Wells et al. Citation1999; De Pascale and Langridge Citation2012; Howarth et al. Citation2012) giving an c. 26% conditional probability of rupture in the next 50 years (Biasi et al. Citation2015). Such an earthquake is expected to be MW c. 8.0, have a rupture length > 200 km, initiate at shallow (< 15 km) depth and, consequently, generate strong ground shaking throughout the affected area () resulting in widespread landsliding (Robinson and Davies Citation2013; Robinson et al. Citation2016). Like the Kaikōura area, the West Coast Region, where the Alpine Fault is located, forms a narrow coastal strip between the Tasman Sea and the Southern Alps. The region is rural, sparsely populated, and relatively inaccessible: only one road (SH6) traverses the region, and only three roads (SH6, SH7 and SH73) cross the Southern Alps connecting it with the rest of the South Island (). These routes navigate through steep and narrow alpine passes (Arthur’s Pass, Lewis Pass and Haast Pass) and cross the Alpine Fault at multiple locations. Consequently, there is potential for substantial disruption to these routes from landslides and other ground damage triggered by an Alpine Fault earthquake. Thus, despite significant differences in the seismological factors between the Kaikōura earthquake and a future Alpine Fault earthquake, the former presents an opportunity to learn from its impacts and the subsequent emergency response and formulate effective response and recovery plans for a future Alpine Fault earthquake.
Using observations from the 2016 Kaikōura earthquake, this study aims to envisage the potential road impacts resulting from a future rupture of the Alpine Fault. The conditions under which road blockages resulted in the Kaikōura event are investigated and subsequently an analysis of where similar conditions exist along road networks is undertaken. Using a scenario Alpine Fault earthquake, the locations where roads are liable to blockages are identified along with any resulting isolated areas. Reflecting on the experience of the Kaikōura earthquake, a discussion of the potential emergency response requirements is provided.
Methods
The primary form of road impacts in the Kaikōura earthquake resulted from fault rupture and landslides (Davies et al. Citation2017; Dellow et al. Citation2017; Mason et al. Citation2017; Stirling et al. Citation2017). By assessing the locations where road impacts occurred in this event, it may be possible to identify sections of road elsewhere in the South Island with similar conditions that may therefore suffer impacts in a future earthquake on other faults (e.g. the Alpine Fault). This study uses road blockage data collected by New Zealand Transport Agency (NZTA) in the immediate aftermath of the Kaikōura earthquake and therefore assumed to have resulted from the mainshock only. The data capture full and partial blockages of major public roads but does not include data on private roads or 4 × 4 tracks. The study area encompasses the region outlined in , which captures the region of South Island exposed to shaking greater than MMI 6.
Fault rupture impacts
Because of the number and complexity of surface fault ruptures in the Kaikōura earthquake (Stirling et al. Citation2017), multiple sections of both SH1 and SH70 were directly affected by surface fault rupture (). Where surface ruptures intersected these roads, the road surface was displaced, with vertical displacements in particular making the road impassable (see Davies et al. Citation2017 and Stirling et al. Citation2017 for examples). Identifying potential sites of future fault rupture impacts for an Alpine Fault earthquake requires identifying locations where other roads intersect the proposed surface rupture trace. Given uncertainties in accurately locating a fault surface trace, this study considers any road within 50 m of the known surface trace of the Alpine Fault to be potentially affected by surface fault rupture. It should be highlighted, however, that such an approach is applicable only for known faults and surface traces. During the Kaikōura earthquake, surface fault rupture occurred on several previously unknown faults and fault traces (Stirling et al. Citation2017). Fault rupture impacts for a future Alpine Fault earthquake scenario must therefore be considered as a minimum assessment, with further impacts from currently unknown faults possible.
Landslide impacts
Whereas assessing road impacts from surface fault rupture requires a simple analysis of the locations where roads and faults intersect, identifying potential locations for landslide impacts is more difficult. During the Kaikōura earthquake, landslide road impacts occurred as a result of slippage as well as falling debris (Davies et al. Citation2017; Dellow et al. Citation2017). Identifying road sections exposed to landslide impacts therefore requires identifying both where landslide source areas may occur and their corresponding runout paths, which is a difficult and time-consuming task. One way to simplify this is to assess the values of landslide predictor variables in proximity to roads and compare sections that were blocked because of earthquake-induced landslides in a previous earthquake with those that were unaffected. Such an approach can allow mean threshold values of various predictor variables above which all, or the majority, of blockages may occur.
The most important predictor variables for landslide occurrence have previously been shown to be some measure of ground shaking (such as peak ground acceleration, PGA), local hillslope gradient and local geology (Parker et al. Citation2015). This study assesses the average slope angle and ground shaking surrounding roads affected by the 2016 Kaikōura earthquake in order to identify the threshold values above which landslide road impacts occurred. Geology is not considered because most slope failures occurred in greywacke which is the dominant rock type in the area (Massey et al. Citation2018). This assumes that slopes comprised of schist along the West Coast, where the Alpine Fault is located, will perform similarly to the greywacke slopes north and south of Kaikōura.
Ground shaking can be measured using a variety of different variables, including PGA, peak ground velocity (PGV) and Modified Mercalli Intensity (MMI). All three measures of ground shaking are compared for the Kaikōura earthquake () using data from Bradley, Razafindrakoto et al. (Citation2017) at 1 km grid resolution. It should be noted, however, that the shaking values for the Kaikōura earthquake from Bradley, Razafindrakoto et al. (Citation2017) differ from those derived by the United States Geological Survey (USGS) and ShakeMap NZ models. Nevertheless, scenario outputs for an Alpine Fault earthquake from the USGS and ShakeMap NZ are not available, whereas outputs using the same model as Bradley, Razafindrakoto et al. (Citation2017) are (Bradley, Bae et al. Citation2017; Bradley, Lagrava et al. Citation2017); this study therefore uses the Bradley, Razafindrakoto et al. (Citation2017) outputs to maintain consistency. Slope angle is calculated from the Land Information New Zealand Digital Elevation Model (DEM) using a grid size of 25 m. It should be noted, however, that such a resolution is likely too coarse to identify small artificial slopes such as road cuts. Although these slopes present a notable and local hazard, Mason et al. (Citation2017) highlight that in the Kaikōura earthquake, road cut failures were limited to minor rockfall which predominantly resulted in minor impingement of the road course or the blockage of a single lane. This may not, however, be the case for an Alpine Fault earthquake.
Affected roads are split into 500 m segments and buffers of 100, 250 and 500 m are created around each segment. A length of 500 m was chosen to provide a reasonable resolution while allowing consistent analysis when applied to the much larger study area for the Alpine Fault earthquake scenario. Buffer widths are selected primarily to account for short-, medium-, and long-landslide runout lengths, with few landslides having runouts > 500 m (Massey et al. Citation2018). Buffer widths < 100 m are assumed too fine to be meaningful due to the pixel resolution of 25 m. Mean values of ground shaking and slope angle are calculated within each buffer zone and values relating to blocked and unblocked road sections are compared.
An Alpine Fault earthquake scenario
In order to identify potential locations of road impacts from a future Alpine Fault earthquake, a possible scenario earthquake must first be derived. For this study, the scenario used in Project AF8 (www.projectaf8.co.nz) was selected. Project AF8 aims to inform emergency response planning using scenario-based analysis. The scenario developed involves an MW 7.9 earthquake with c. 350 km rupture between Charles Sound and Hokitika (). Ground shaking variables for this scenario have been taken from Bradley, Bae et al. (Citation2017) and Bradley, Lagrava et al. (Citation2017) at 1 km grid resolution. The effect of rupture directivity has been considered as part of Project AF8, with three different rupture directions considered: south to north, bilateral and north to south. In this study, only the south-to-north rupture direction has been considered () because, at the time of writing, it is the only scenario for which shaking data are openly available. Slope data are calculated using the same data set and resolution as the Kaikōura data set for consistency.
Results
Observed blockages from the Kaikōura earthquake
Surface fault rupture crossed SH1 and SH70 at eight locations (). Five of these are located on SH1 as a result of surface rupture on the Kekerengu, Papatea and Hundalee faults, and three on SH70 from the Humps, North and South Leader, and Conway–Charwell faults (Stirling et al. Citation2017). Placing a 50 m buffer around the fault ruptures yields 10 locations, with the two extra locations occurring west of Oaro on SH70 where fault rupture ran parallel and close to the road (). This suggests that a 50 m buffer for the Alpine Fault scenario is appropriate although conservative.
Results for the analysis of landslide blockages show there were 20 blocked locations (). Notably, regardless of buffer width, all road segments affected by landslides had surrounding slope angles that averaged > 17° and shaking that averaged > 0.43 g (PGA), > 41 cm/s (PGV) or MM > 7.9 (MMI) (). By comparison, road segments with mean slope angle and ground shaking values that exceed these thresholds in the scenario Alpine Fault earthquake can therefore be considered at risk of landslide blockage. However, a large number of unblocked road segments also had average slope angles and ground shaking in excess of these thresholds ().
Figure 2. Average slope angle compared to average peak ground acceleration (PGA), peak ground velocity (PGV) and Modified Mercalli Intensity (MMI) values within 500, 250 and 100 m buffers for each 500 m section of road network between Christchurch and Picton. Sections blocked by landslides are shown in red with the total range of variable shown by error bars. Sections unaffected by landslides are shown in grey. Black dashed lines represent lower threshold values above which 100% of landslide blockages occur. Red dashed lines represent upper threshold values maximising the number of landslide blockages above the thresholds (true positives) whilst minimising the number of unblocked sections above the thresholds (false positives).
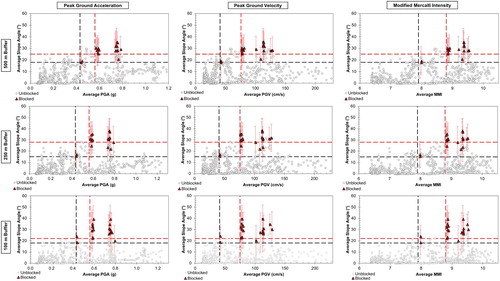
To optimise the predictive power of the model, higher thresholds are assigned that simultaneously maximise the number of blocked sections and minimise the number of unblocked sections above both thresholds. The upper threshold slope angle varies with buffer width, with 22° for 100 m buffers, 28° for 250 m buffers and 25° for 500 m buffers. For the ground shaking variables, buffer width has no effect on upper threshold values, with 0.56 g for PGA, 76 cm/s for PGV and 8.8 for MMI respectively. If such thresholds were applied to other roads and earthquake scenarios (e.g. an Alpine Fault scenario), it would be possible, with uncertainty, to forecast locations where landslide blockages could be expected.
Before doing so, it is important to consider the predictive ability of each threshold combination, particularly in terms of the number of blockages (true positives) as well as the number of unblocked sections (false positives). For the Kaikōura earthquake, a total of 20 road sections were blocked, leaving 634 sections unblocked. A perfect prediction should therefore have all 20 blocked sections above the threshold combination and all 634 unblocked sections below. Data for the thresholds in are summarised in . The lower threshold combinations for all buffer widths are deliberately set to successfully account for all 20 landslide-blocked sections; however, these thresholds also result in a large number of false positives, meaning the total number of blocked road sections are in the minority. For instance, using a 250 m buffer and PGA as the shaking predictor, 56 road segments are above the lower thresholds, meaning that just 36% were actually blocked (). Using the higher threshold combinations greatly reduces the number of false positives so that the majority of sections above the thresholds are true positives. However, this has the negative effect of reducing the total number of true positives. For instance, using the same example as before, 23 road segments are above the higher thresholds, of which 65% were actually blocked; however, five blocked segments are below the threshold combination.
Table 1. Upper and lower thresholds and corresponding prediction scores derived from for different buffer widths and ground shaking variables for landslide road blockages during the 2016 Kaikōura earthquake.
Scenario Alpine Fault earthquake
For the scenario Alpine Fault earthquake (), seven sections of state highway are located within 50 m of a known surface trace of the Alpine Fault () and thus at risk of blockage in this scenario. The majority of these occur between the townships of Franz Josef and Haast, with a further location between Franz Josef and Hokitika. Surface fault ruptures from previous Alpine Fault earthquakes are thought to have averaged c. 8 m horizontally and 1–2 m vertically (Berryman et al. Citation2012). Such displacements are similar to or larger than those experienced in the Kaikōura earthquake (Stirling et al. Citation2017) and suggest that these sections of road will be completely impassable to all vehicles.
Figure 3. Locations of road impacts related to surface fault rupture and landslides from an Alpine Fault earthquake for different buffer widths and shaking variables. Threshold data for landslide blockages are calculated from observations of the Kaikōura earthquake from and .
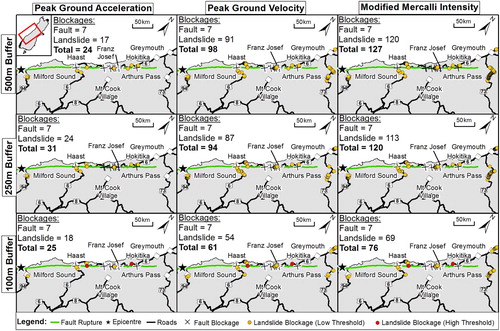
The number and location of road segments exceeding the corresponding upper and lower landslide blockage thresholds from the Kaikōura earthquake (; ) varies between 17 and 120 depending on the ground shaking variable and buffer width (). Using PGA consistently yields fewer segments at risk (between 17 and 24) of landslide blockages than PGV (between 54 and 91) or MMI (between 69 and 120). This appears to result from the lack of high PGA values in the Arthur’s Pass region compared to high PGV and MMI values ( and ). Without independent testing of the performance of each shaking variable it is impossible to say which performs best, however, the relative agreement between the PGV and MMI variables suggests the results associated with these measures may be more accurate.
In total, for the same shaking variable, the number of segments at risk of blockage generally decreases with decreasing buffer width. This likely relates the fact the majority of road segments for the Alpine Fault scenario are inland and therefore increases in buffer width produce larger changes in average slope angle than on the predominantly coastal roads affected by the Kaikōura event. Nevertheless, despite the variability, several sections of road are consistently at risk of landslide blockage, irrespective of the different buffer widths used. These are:
SH6 immediately south of Franz Josef;
SH6 c. 20 km northeast of Haast;
SH6 c. 10 km east of Haast;
SH94 immediately south of Milford Sound; and
SH73 immediately north of Arthurs Pass.
If these sections were blocked, access to the West Coast Region would only be possible via SH7, with access only possible to c. 50 km south of Hokitika. Consequently, according to the most recent New Zealand census (Statistics New Zealand Citation2017), > 10,000 local people would be cut-off by road. Depending on the time of year, several thousand domestic and international tourists could also be affected. This is a substantially larger number of people than affected by the Kaikōura earthquake, with the population also being more widely distributed. Further, unlike during the Kaikōura earthquake when alternative access to Blenheim remained possible via SH7, no alternative road routes to Milford Sound, Franz Josef, Haast or Arthur’s Pass exist. Re-establishing connections with these townships is likely to prove a substantial challenge in both the short and long term following an Alpine Fault earthquake.
Discussion
Gaining emergency access
Following the Kaikōura earthquake, the Ministry of Civil Defence & Emergency Management (CDEM) immediately prioritised gaining access to isolated communities to provide essential supplies and evacuate stranded tourists in order to reduce the load on what limited local supplies were available (Davies et al. Citation2017). Because of road blockages, this was only possible via air and sea; however, both options proved unreliable. Poor weather conditions resulted in several days when flying was not possible, while the small size of, and damage to the Kaikōura port restricted the number and size of ships that could dock (Davies et al. Citation2017). In total, 998 tourists were evacuated from Kaikōura either by air or by sea.
Similar issues are also expected to occur following an Alpine Fault earthquake. Gaining access to the West Coast Region and Milford Sound is likely to be CDEM’s main priority, and with the expected damage to roads (), air and sea routes are likely to provide the only options. However, weather conditions west of the Southern Alps can be difficult and changeable, with monthly average rainfall in Hokitika averaging 250 mm, while in Milford Sound this can exceed 500 mm, and with rainfall occurring > 200 days per year. Furthermore, few locations in West Coast Region have access to airstrips, meaning that they are likely to be reliant on helicopters which have smaller weight-carrying capacities than fixed-wing aircraft. As in Kaikōura, ports in West Coast Region and Milford Sound are small and likely to have sustained damage during the earthquake, limiting the size and number of ships that can dock. However, no viable berthing spots for ships exist between Hokitika and Milford Sound, meaning isolated communities in this region are likely to be entirely reliant on air access.
A further complication exists in the number and location of tourists affected by an Alpine Fault earthquake. Whereas c. 1000 tourists were evacuated from Kaikōura following the 2016 earthquake, an Alpine Fault earthquake could affect far larger numbers. More than 1.3 million travellers visit the West Coast Region each year, with enough capacity for up to 4000 visitors per night in the popular Franz Josef area (Tourism West Coast Citation2015). Milford Sound is one of New Zealand’s most popular tourist sites, attracting > 650,000 visitors per year, equating to c. 1700 per day (Venture Southland Citation2017). Although at night visitors cluster in major townships, during the day most will be spread out across the region, with many visiting remote areas on foot. Consequently, it is possible that following an Alpine Fault earthquake, nearly five times as many tourists will require evacuation than following the Kaikōura earthquake, with these people distributed across a region of > 10,000 km2.
Restoration and recovery
NZTA began clearing road blockages 2 days after the Kaikōura earthquake, focusing initially on SH70 and SH1 south of Kaikōura (Davies et al. Citation2017). The focus was primarily to return road access to all isolated communities and allow emergency vehicles access to affected regions. However, it took 11 days before non-emergency vehicles could leave Kaikōura via a supervised convoy on SH70 (Davies et al. Citation2017). It took 23 days to restore supervised access to all isolated communities, with SH70 becoming the first road fully reopened after 38 days. SH1 was finally reopened in December 2017, albeit only to single-file traffic during daylight hours and in dry weather conditions.
Restoring road access following an Alpine Fault earthquake is likely to prove as difficult as the northern section of SH1. Although access to the West Coast Region is likely to remain possible via SH7 (), restoring access along SH6 and SH73 is expected to prove difficult due to the steep terrain, likelihood of poor weather and continuing aftershocks. Further considerations for restoration times include whether or not the road is confined, the volume of debris to remove and the ease of access. Reopening the alpine passes will prove most difficult, because the confined nature means rerouting the road is not an option and thus landslide debris will need to be removed. Furthermore, the narrow nature of the passes means that debris clearance will likely be restricted to the outer-most blocking landslides, with limited options for simultaneous clearance of multiple landslide blockages. Previous estimates by NZTA have therefore suggested that it would require > 6 months before restoration works could even begin on SH6 south of Franz Josef and east of Haast, and on SH73 (Robinson et al. Citation2015). Given the difficulties being experienced clearing SH1 north of Kaikōura these estimates appear to be realistic, if not underestimates. This means no road access is expected on SH6 between Franz Josef and Haast, and along SH73 for > 6 months after an Alpine Fault earthquake. In comparison, SH94 is expected to be cleared within 14 days because the route is regularly blocked by avalanches and rockfalls, and consequently NZTA are experienced at clearing this route and have sufficient equipment already in place (Robinson et al. Citation2015). It should be noted, however, that road bridge performance has not been accounted for here, and the collapse of a major road bridge is likely to present as large a challenge for restoration as landslide or surface fault rupture.
Implications for emergency response
The Kaikōura earthquake provides a useful analogue for a future Alpine Fault earthquake in terms of road impacts and loss of access. It therefore provides an opportunity to evaluate the emergency response undertaken and plan the necessary future response. First, it is clear that planning for the evacuation of large numbers of spatially distributed tourists is essential. In the 2016 earthquake, tourists were evacuated from Kaikōura township by a combination of air and sea, with 363 evacuated by helicopter and 635 by sea (Davies et al. Citation2017). This evacuation was partly aided by tourists being concentrated in a single township; however, in an Alpine Fault earthquake larger numbers of tourists are expected to be distributed across a much larger area, especially if it occurs during the daytime. This is in conjunction with an expectation that weather conditions in West Coast Region are likely to hinder air evacuations and the lack of sufficient port facilities will hinder evacuations by sea. It is therefore essential that contingency planning for the evacuation of tourists following an Alpine Fault earthquake is undertaken.
A further key issue is in the provision of emergency supplies to isolated local populations. While the current CDEM advice is to be self-sufficient for 3 days following a disaster, it is clear that many West Coast communities will be isolated for far longer following an Alpine Fault earthquake. As in the Kaikōura earthquake, ensuring emergency supplies can be delivered to these regions is essential. Although initially this may be able to be combined with evacuation of tourists, with some road routes expected to be impassable for > 6 months, continuous resupply by sea and air is unlikely to be sustainable. Considering alternative arrangements is therefore essential. One possibility is the temporary evacuation of isolated local populations until road access can be restored. However, this is likely to prove controversial and poses questions as to how to respond to anyone refusing to leave as well as the management of safe return. Planning how to maintain supplies to isolated locals for several months following an Alpine Fault earthquake is therefore urgently required.
A final implication to consider is the effect of such road impacts on the local economy. The West Coast Region has three major industries: mining, tourism and dairy. Mining is predominantly dependent on the rail network, which has not been investigated in this study. However, the main rail route that transports mining products to Christchurch for national and international distribution follows SH73 for much of its route and therefore is also expected to be severely impacted. The loss of reliable road access to much of the region will undoubtedly affect the dairy and tourism industries, with farms unable to distribute milk products and tourists unable to reach popular destinations such as Franz Josef. Although some work has been done to investigate the potential impact of an Alpine Fault earthquake on tourism (Orchiston Citation2012), to date little work has looked at the potential impacts to the dairy industry.
Conclusions
In terms of its impacts, particularly on roads, the 2016 Kaikōura earthquake provides a potential analogue for a future rupture of the Alpine Fault. The 2016 Kaikōura earthquake displaced major state highways in several locations making the roads completely impassable. Numerous landslides also blocked key access routes, causing many communities to be isolated and requiring the evacuation of tourists by air and sea. An analysis of the predictive variables for landslide occurrence along the SH1 and SH70 road corridors shows that the lower and upper thresholds respectively for road blockages were 17° and 28° for local slope, 0.43 and 0.56 g for PGA, 41 and 76 cm/s for PGV, and 7.9 and 8.8 for MMI. Using these observations, this study identifies up to 120 other locations where landslides may potentially block roads in a future Alpine Fault earthquake. This suggests that blockages can be expected along SH6 between Hokitika and Haast, on SH94 before Milford Sound and on SH73 around Arthur’s Pass. Previous studies have suggested several sections of these roads could take > 6 months to restore. However, it is notable that the number of potential blockage sites varies considerably with different shaking variables, and the length of blockage time is dependent on numerous local factors, which this study has not investigated. Nevertheless, contingency planning for the evacuation of large numbers of spatially distributed tourists and the provision of sufficient emergency supplies to local populations is urgently required. Examining the response to the Kaikōura earthquake may therefore highlight valuable lessons that can be learnt prior to an Alpine Fault earthquake to aid such planning.
Acknowledgements
I would like to acknowledge Dr Sam McColl and another anonymous reviewer whose helpful feedback greatly improved the quality and scope of this manuscript.
Disclosure statement
No potential conflict of interest was reported by the author.
Additional information
Funding
References
- Berryman KR, Cochran UA, Clark KJ, Biasi GP, Langridge RM, Villamor P. 2012. Major earthquakes occur regularly on an isolated plate boundary fault. Science. 336(6089):1690–1693. doi: 10.1126/science.1218959
- Biasi GP, Langridge RM, Berryman KR, Clark KJ, Cochran UA. 2015. Maximum-likelihood recurrence parameters and conditional probability of a ground-rupturing earthquake on the southern Alpine Fault, South Island, New Zealand. Bulletin of the Seismological Society of America. 105(1):94–106. doi: 10.1785/0120130259
- Bradley B, Lagrava A, Savarimuthu D, Bae SE. 2017. Seisfinder: A web application for the extraction of high-fidelity outputs from computationally-intensive earthquake resilience calculations. Quakecore annual meeting, September 2017.
- Bradley BA, Bae SA, Polak V, Lee RL, Thomson EM, Tarbali K. 2017. Ground motion simulations of great earthquakes on the Alpine Fault: effect of hypocentre location and comparison with empirical modelling. New Zealand Journal of Geology and Geophysics. 60(3):188–198. doi: 10.1080/00288306.2017.1297313
- Bradley BA, Razafindrakoto HN, Polak V. 2017. Ground-motion observations from the 14 November 2016 Mw 7.8 Kaikoura, New Zealand, earthquake and insights from broadband simulations. Seismological Research Letters. 88:740–756. doi: 10.1785/0220160225
- Cochran UA, Clark KJ, Howarth JD, Biasi GP, Langridge RM, Villamor P, Berryman KR, Vandergoes MJ. 2017. A plate boundary earthquake record from a wetland adjacent to the Alpine fault in New Zealand refines hazard estimates. Earth and Planetary Science Letters. 464:175–188. doi: 10.1016/j.epsl.2017.02.026
- Cubrinovski M, Bray JD, de la Torre C, Olsen MJ, Bradley BA, Chiaro G, Stocks E, Wotherspoon L. 2017. Liquefaction effects and associated damages observed at the Wellington centreport from the 2016 Kaikoura earthquake. Bulletin of the New Zealand Society for Earthquake Engineering. 50(2):152–173.
- Davies AJ, Sadashiva V, Aghababaei M, Barnhill D, Costello SB, Fanslow B, et al. 2017. Transport infrastructure performance and management in the South Island of New Zealand, during the first 100 days following the 2016 Mw 7.8 Kaikōura earthquake. Bulletin of the New Zealand Society for Earthquake Engineering. 50(2):271–299.
- De Pascale GP, Langridge RM. 2012. New on-fault evidence for a great earthquake in AD 1717, central Alpine fault, New Zealand. Geology. 40(9):791–794. doi: 10.1130/G33363.1
- Dellow S, Massey C, Cox S, Archibald G, Begg J, Bruce Z, et al. 2017. Landslides caused by the 14 November 2016 Mw7.8 Kaikōura earthquake and the immediate response. Bulletin of the New Zealand Society for Earthquake Engineering. 50(2):106–116.
- Hamling IJ, Hreinsdóttir S, Clark K, Elliott J, Liang C, Fielding E, et al. 2017. Complex multifault rupture during the 2016 Mw 7.8 Kaikōura earthquake, New Zealand. Science. 356(6334). doi: 10.1126/science.aam7194
- Howarth JD, Fitzsimons SJ, Norris RJ, Jacobsen GE. 2012. Lake sediments record cycles of sediment flux driven by large earthquakes on the Alpine fault, New Zealand. Geology. 40(12):1091–1094. doi: 10.1130/G33486.1
- Howarth JD, Fitzsimons SJ, Norris RJ, Langridge R, Vandergoes MJ. 2016. A 2000 yr rupture history for the Alpine Fault derived from Lake Ellery, South Island, New Zealand. Geological Society of America Bulletin. 128:627–643. doi:10.1130/B31300.1.
- Kaiser A, Balfour N, Fry B, Holden C, Litchfield N, Gerstenberger M, et al. 2017. The 2016 Kaikōura, New Zealand, earthquake: preliminary seismological report. Seismological Research Letters. 88(3):727–739. doi: 10.1785/0220170018
- Liu Y, Nair N-K, Renton A, Wilson S. 2017. Impact of the Kaikōura earthquake on the electrical power system infrastructure. Bulletin of the New Zealand Society for Earthquake Engineering. 50(2):300–305.
- Mason D, Brabhaharan P, Saul G. 2017 Performance of road networks in the 2016 Kaikōura earthquake: observations on ground damage and outage effects. In: GJ Alexander, CY Chin, editors. Proceedings 20th NZGS geotechnical symposium. Napier.
- Massey C, Townsend D, Rathje E, Allstadt KE, Lukovic B, Kaneko Y, Bradley B, Wartman J, Jibson RW, Petley DN, et al. 2018. Landslides triggered by the 14 November 2016 MwMw 7.8 Kaikōura earthquake, New Zealand. Bulletin of the Seismological Society of America. doi:https://doi-org.ezphost.dur.ac.uk/10.1785/0120170305.
- Orchiston C. 2012. Seismic risk scenario planning and sustainable tourism management: Christchurch and the Alpine Fault zone, South Island, New Zealand. Journal of Sustainable Tourism. 20(1):59–79. doi: 10.1080/09669582.2011.617827
- Orense RP, Mirjafari Y, Asadi S, Naghibi M, Chen X, Altaf O, Asadi B. 2017. Ground performance in Wellington waterfront area following the 2016 Kaikōura earthquake. Bulletin of the New Zealand Society for Earthquake Engineering. 50(2):142–151.
- Palermo A, Liu R, Rais A, McHaffie B, Andisheh K, Pampanin S, Gentile R, Nuzzo I, Granerio M, Loporcaro G, et al. 2017. Performance of road bridges during the 14 November 2016 Kaikōura earthquake. Bulletin of the New Zealand Society for Earthquake Engineering. 50(2):253–270.
- Parker RN, Hancox GT, Petley DN, Massey CI, Densmore AL, Rosser NJ. 2015. Spatial distributions of earthquake-induced landslides and hillslope preconditioning in the northwest South Island, New Zealand. Earth Surface Dynamics. 3(4):501–525. doi: 10.5194/esurf-3-501-2015
- Robinson TR, Davies TRH. 2013. Potential geomorphic consequences of a future great (Mw = 8.0+) Alpine Fault earthquake, South Island, New Zealand. Natural Hazards and Earth System Sciences. 13(9):2279–2299. doi: 10.5194/nhess-13-2279-2013
- Robinson TR, Davies TRH, Wilson TM, Orchiston C. 2016. Coseismic landsliding estimates for an Alpine Fault earthquake and the consequences for erosion of the Southern Alps, New Zealand. Geomorphology. 263:71–86. doi: 10.1016/j.geomorph.2016.03.033
- Robinson TR, Wilson TM, Buxton R, Cousins WJ, Christophersen AM. 2015. An Alpine Fault earthquake scenario to aid in the development of the Economics of Resilient Infrastructure’s MERIT model. ERI Research Report 2016/04.
- Statistics New Zealand. 2017. Subnational population estimates: at 30 June 2017 (provisional). Retrieved from nzdotstat.stats.govt.nz/wbos/Index.aspx?DataSetCode=TABLECODE7541. Last Accessed 28 March 2018.
- Stirling M, McVerry G, Gerstenberger M, Litchfield N, Van Dissen R, Berryman K, Barnes P, Wallace L, Villamor P, Langridge R, et al. 2012. National seismic hazard model for New Zealand: 2010 update. Bulletin of the Seismological Society of America. 102(4):1514–1542. doi:https://doi.org/10.1785/0120110170.
- Stirling MW, Litchfield NJ, Villamor P, Van Dissen RJ, Nicol A, Pettinga J, et al. 2017. The Mw7. 8 2016 Kaikōura earthquake: surface fault rupture and seismic hazard context. Bulletin of the New Zealand Society for Earthquake Engineering. 50(2):73–84.
- Tourism West Coast. 2015. Minutes of the Annual General Meeting of Tourism West Coast Inc. 5th July 2015.
- Venture Southland. 2017. Southland tourism key indicators. MBIE Report.
- Wells A, Yetton MD, Duncan RP, Stewart GH. 1999. Prehistoric dates of the most recent Alpine fault earthquakes, New Zealand. Geology. 27(11):995–998. doi: 10.1130/0091-7613(1999)027<0995:PDOTMR>2.3.CO;2
- Yetton MD. 1998. Progress in understanding the paleoseismicity of the central and northern Alpine Fault, Westland, New Zealand. New Zealand Journal of Geology and Geophysics. 41(4):475–483. doi: 10.1080/00288306.1998.9514824