ABSTRACT
The Reefton Goldfield is well known for its orogenic style of Au mineralisation although the timing remains largely unconstrained. Here, a first unequivocal occurrence of igneous-related mineralisation is documented and it is suggested to place a lower age constraint on when orogenic Au mineralisation occurred. Emplacement of the 118.5 ± 1.3 Ma (U-Pb zircon) Blackwater granitoid dike into Early Paleozoic Greenland Group caused contact metamorphism up to hornblende hornfels facies temperatures (>500°C) at low pressures (<3.75 kbar). A hydrothermal convective system that developed during cooling led to propylitic and phyllic alteration associated with the precipitation in order of decreasing abundance of: pyrite, chalcopyrite, molybdenite, arsenopyrite-galena-sphalerite-millerite. Quartz veins and stockworks containing combinations of pyrite, chalcopyrite and molybdenite are common within several hundred metres of the dike. This igneous-related mineralisation overprints Greenland Group metasedimentary rocks displaying evidence for a phase of ductile deformation and associated with the occurrence of pyrite and ankerite-cored siderite porphyroclasts, chalcopyrite and cobaltite. Au-in-pyrite trace element data indicate that these pyrite porphyroclasts are more enriched in Au than the pyrite associated with dike emplacement. Quartz lodes containing Au occur within zones of ductile deformation elsewhere in the goldfield, and therefore Early Cretaceous emplacement of the Blackwater dike provides a lower age constraint for the ductile mineralisation event.
Introduction
The Reefton area was one of the largest historic hard-rock goldfields in New Zealand with gold extracted from mines along narrow north-striking belts within the Early Paleozoic metasedimentary Greenland Group. Between 1870 and 1951, approximately 67 tonnes of gold was extracted from a number of mines. The main Au-producing locations were the Globe-Progress deposit, which follows a large discordant shear zone in an otherwise northward-striking regional fabric, and the Blackwater deposit, which followed the sub-vertical >1 km deep quartz Birthday Reef (Gage Citation1948; Christie and Brathwaite Citation2003; Hamisi et al. Citation2017) (). In all the documented cases across the goldfield, hard-rock Au mineralisation is intimately associated with arsenopyrite-bearing quartz veins or breccias, and the general model assumed is that elements were scavenged during dehydration at depth during orogenesis, transported in solution, and then precipitated along fractures and faults at shallow crustal levels (e.g. Christie and Brathwaite Citation2003; Bierlein et al. Citation2004; Milham and Craw Citation2009; Hamisi et al. Citation2017). No igneous-related mineralisation has so far been confirmed but many granitoid plutons occur in close proximity to the goldfield (Gage, Citation1948; Tulloch et al. Citation2009; Turnbull et al. Citation2016), which itself is known to contain scattered granitic, lamprophyric and trachyte dikes (Gage Citation1948; Suggate Citation1957; Muir et al. Citation1996; Nathan et al. Citation2002; Ritchie et al. Citation2015; van der Meer et al. Citation2016, Citation2017).
Figure 1. Location map of the area of study showing generalized geology. Modified from Nathan et al. (Citation2002).
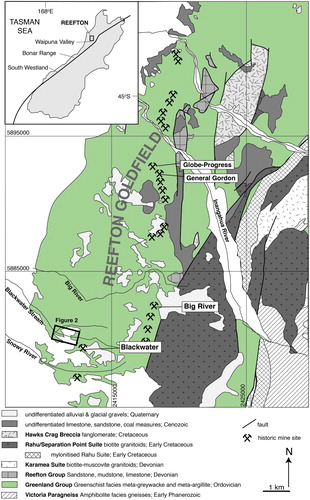
This short article reports the first occurrence of demonstrably igneous-related mineralisation within the Reefton Goldfield. We document the rocks associated with a porphyritic granitoid dike that has intruded Greenland Group approximately 1 km west of historic Blackwater Mine (). A U-Pb zircon age establishes the timing of its emplacement. Field relationships and microscopic observations indicate that the dike, which has caused molybendite mineralisation, intrudes earlier formed ductile fabrics that contain Au-bearing pyrite porphyroclasts, and hence the intrusion age may also provide a lower age constraint to the ductile stage of orogenic mineralisation that is widespread in the goldfield.
Geological setting
The Reefton Goldfield is located within the Buller Terrane on the western side of the South Island (). The host formation is the Greenland Group, which comprises meta-greywackes and meta-argillites that were deposited at the paleo-Pacific Gondwana margin (Laird Citation1972; Cooper Citation1974; Laird and Shelley Citation1974; Adams et al. Citation2015). The presence of graptolites (Cooper Citation1974) and youngest detrital zircon age populations in Greenland Group in the Reefton area indicate deposition in the Ordovician (Ireland Citation1992; Adams et al. Citation2015). The Greenland Group has been macroscopically folded and partially recrystallised at greenschist facies conditions (Gage Citation1948; Rattenbury and Stewart Citation2000; Ritchie et al. Citation2015) prior to emplacement of Late Devonian granitoids (Muir et al. Citation1996; Waight et al. Citation1997, Citation1998; Tulloch et al. Citation2009; Turnbull et al. Citation2016). Although the greenschist facies recrystallisation associated with folding must be pre-Late Devonian, higher metamorphic grade assemblages in exhumed deeper levels of the Greenland Group indicate that there have been subsequent phases of regional metamorphism. For example, monazite reveals c. 108 Ma assemblages in the Waipuna Valley just south of the goldfield (Ritchie et al. Citation2015), 371–343 Ma amphibolite facies Greenland Group-equivalents occurs in the Bonar Range in central Westland (Scott et al. Citation2011) and 355–340 Ma assemblages occur in the Paringa-Jackson River area of south Westland (Mortimer et al. Citation2013; Belton and Scott Citation2014; Palmer et al. Citation2015) (). The low metamorphic grade rocks of the goldfield may be the hanging wall of a low-angle Cretaceous ductile shear zone (the Waipuna Shear Zone) that has been correlated with detachment faults in the Paparoa Metamorphic Core Complex (Ritchie et al. Citation2015).
The goldfield is surrounded by several igneous plutonic suites. The oldest intrusive rocks are S-type granites of the Karamea Suite and were emplaced between c. 378 and 368 Ma (Muir et al. Citation1996; Tulloch et al. Citation2009; Turnbull et al. Citation2016). Although undated, rocks interpreted by Nathan et al. (Citation2002) to be part of the c. 110 Ma transitional I/S-type Rahu Suite (Waight et al. Citation1997, Citation1998) occur east of the goldfield (). Some of the Cretaceous Rahu Suite plutons outside of the Reefton goldfield have low levels of Mo-mineralisation and have been the focus of past exploration efforts (Eggers and Adams Citation1979; Tulloch and Rabone Citation1993; Shand and Scott Citation2016). Lamprophyric and trachyte dikes in the goldfield are associated with Late Cretaceous intraplate magmatism (Tulloch and Kimbrough Citation1989; van der Meer et al. Citation2016, Citation2018).
Hard-rock Au mineralisation within the Reefton Goldfield is confined to fault zones and their alteration haloes within the Greenland Group (Henderson Citation1917; Gage Citation1948; Christie and Brathwaite Citation2003; Hamisi et al. Citation2017). Mapping of the goldfield has revealed that while the mineralisation is parallel to macroscopic fold axial trend, it is not located in the hinges and it cuts across the axial planar cleavage associated with the folds (Hamisi et al. Citation2017). There are broadly two end-member types of Au mineralisation: an earlier ductile phase in which quartz, coarse rhombic arsenopyrite, pyrite and minor gold were deposited, followed by a brittle phase in which quartz, gold and needle-like arsenopyrite precipitated (Christie and Brathwaite Citation2003; Milham and Craw Citation2009). Stibnite, minor tetrahedrite, galena, bournonite, sphalerite and chalcopyrite may also be present (Christie and Brathwaite Citation2003; MacKenzie et al. Citation2014; Shand et al. Citation2015; Stick et al. Citation2015; Hamisi et al. Citation2017). The two phases of mineralisation have been best documented in the Globe-Progress deposit, with stibnite confined to the second event (Milham and Craw Citation2009). The timing of Au mineralisation is very poorly constrained to having occurred after regional folding, which predates 375 Ma plutonism, and prior to deposition of overlying Eocene coal measures. Our study offers new insight into the age constraints on Au mineralisation.
Methods
Mineral identification was confirmed using semi-quantitative analyses on a Zeiss Sigma scanning electron microscope (SEM) with an electron dispersal spectrometer (EDS) attachment at the University of Otago. The instrument was operated with beam current at 15 kV and an accelerating voltage of 2.7 nA at 60 µm aperture. Data were processed with the Aztec software from Oxford Instruments.
Trace element concentrations were measured in pyrite grains using the laser ablation inductively coupled plasma mass spectrometry (LA-ICP-MS) facility at the Center for Trace Element Analysis at the University of Otago. The analyses were collected on a Resonetics RESOlution M-50-LR laser ablation system with a Coherent CompexPro 102 193 nm ArF excimer laser and Laurin Technic sample cell. The laser was operated at 100 mJ and 12.5% transmission for an on-sample fluence of c. 2 J/cm2. Ablated material was carried by He gas from the sample cell, mixed with Ar and N2, and measured by an Agilent 7500cs ICP-MS. Data were collected in time-resolved mode with one point per peak. Integration times were 10 ms for 54Fe, 57Fe, 75As, 197Au, and 50 ms for 204Pb, 206Pb, 207Pb, and 208Pb. Background (laser off) data were acquired for 20 s followed by 40 s with the laser operated at a 10 Hz repetition rate using a spot diameter of 130 μm. Each set of six samples was bracketed by analyses of standard glass NIST SRM610. Trace-element concentrations were obtained by normalising count rates for each element to those for 57Fe in the sample and standard using concentrations in NIST 610 (Jochum et al. Citation2011) and Fe in the sample assumed from ideal stoichiometry for pyrite (45.6 wt%).
Zircon grains were extracted and U-Pb isotopes measured by LA-ICP-MS following the method of Scott and Palin (Citation2008). The same instrument and operating conditions were used as those described above for pyrite, except that ablation was at 5 Hz with a 33 μm spot and a different suite of masses were analysed with integration times of 40 ms for 206Pb, 207Pb, 208Pb, 232Th, 235U and 238U. Trace element data were normalised to Si in NIST SRM610 using the ideal content of SiO2 content in zircon (32.78 wt%), while U–Th–Pb isotopes were calibrated and corrected for down-hole fractionation against TEMORA 2 zircon. Both standards were analysed between every ten dike zircons. U–Th–Pb ratios and dates were calculated using the decay constants and 238U/235U ratio recommended by Steiger and Jäger (Citation1977), then corrected for common-Pb using 208Pb and the Cumming and Richards (Citation1975) model. Ages were calculated using Iolite (Paton et al. Citation2011)
Geology of Blackwater creek
Blackwater dike
The porphyritic granitoid dike is exposed in the bed of the lower Blackwater Creek (). It was first documented by Gage (Citation1948) who reported that ‘a granite sill is injected along the steep bedding planes of the quartzites and phyllites … contact effects are visible on both sides, and it is practically certain not to have been faulted into its present position.’ Aside from a sampling programme by OceanaGold Ltd in 2014, the dike has not been further investigated. The dike has a true width of about 10 m, sub-vertical contacts, and strikes northeastwards. The lateral extent is unknown because it is not exposed in streams to the north or south and it is not visible in aeromagnetic data.
Figure 2. Map and cross-section of lower Blackwater Creek illustrating the structural features and mineralisation of the valley.
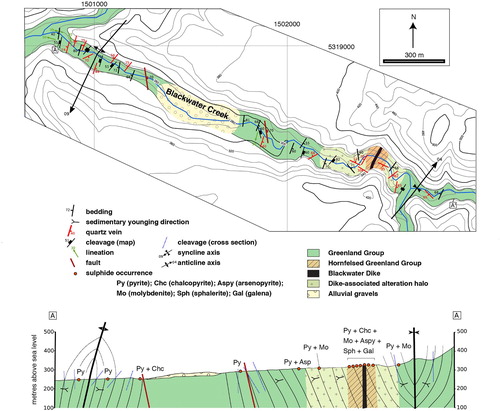
The dike is granitic and holocrystalline. On the northeast side of the exposure, it is distinctly green owing to the abundance of chlorite ((A)). This grades south-westwards over several metres into a pale-coloured chlorite-free portion ((B)). The dike is dominated, where fresh, by quartz, plagioclase and K-feldspar with lesser amounts of biotite, amphibole, titanite, magnetite and tourmaline. Plagioclase is the dominant feldspar and forms euhedral to subhedral phenocrysts that can reach up to about 5 mm ((C)). These grains are normally zoned, with semi-quantitative measurements indicating variations from labradorite to andesine (c. An58 to An45). Many of the feldspars have undergone sericitic and/or sausseritic alteration, and the biotite and amphibole phenocrysts have been partially to totally replaced by chlorite that is inter-grown with fine needles of rutile. Although the dike is dominated by igneous textures, a small band of rock containing phenocrysts of biotite and euhedral normally zoned feldspars (An55 to An45) set within a hornfelsic matrix of quartz, biotite, muscovite and rutile occurs 30 m west ((D)). Such coarse feldspars have not been seen in the Greenland Group, and this rock may therefore be an earlier porphyritic phase that has been contact metamorphosed and partially re-equilibrated. Alternatively, it may be an older tuff horizon within the Greenland Group that has been metamorphosed.
Figure 3. Blackwater dike textures. (A) and (B) display hand specimens of the dike. There is some feldspar colour change associated with sausseritic and/or sericitic alteration. (C) Cross-polarised light image of a zoned plagioclase phenocryst. (D) A back-scattered electron image of a coarse plagioclase within hornfelsic Greenland Group. Ser, sericite; Qtz, quartz; Bt, biotite; Musc, muscovite. (E) Hans specimen showing the dike to be cut by narrow quartz-pyrite veins. (F) A reflected light microscope image shows prominent pyrite, arsenopyrite (Aspy) and molybdenite (Mo).
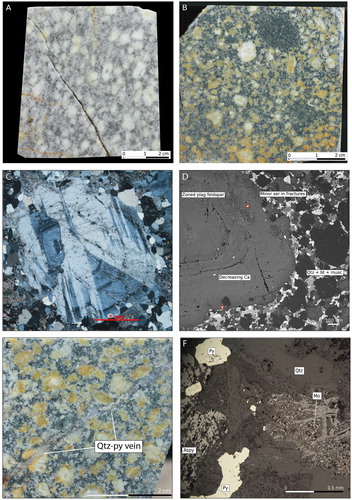
Alteration of the dike can be divided into phyllic (predominately sericite, muscovite and pyrite) and propylitic (chlorite, epidote and sericite) assemblages. These two assemblages are not mutually exclusive and a continuum occurs in outcrop. Towards the northeast of the exposure, the propylitic assemblages are dominant over several metres, whereas phyllic assemblages dominate the southwestern several metres of exposure. Within the altered rocks, pyrite is the dominant sulphide and is commonly found as anhedral grains associated with sericite and occasionally inter-grown with chlorite, or in quartz veins ((E)). Chalcopyrite occurs as very fine grains throughout sericitic patches and within adjacent coarser pyrite grains. Arsenopyrite is subhedral to anhedral although rare euhedral rhombs have been observed, and molybdenite forms fine acicular radiating masses ((F)). Arsenopyrite and molybdenite appear to be restricted to more phyllic-altered rocks. A third alteration style, argillic alteration, is indicated by the presence of illite identified by X-ray diffraction.
Greenland Group
North-northeast striking and steeply dipping inter-bedded metamorphosed greywackes and argillites of the Greenland Group form the dominant lithology in Blackwater Creek (). Bedding-cleavage relationships and sedimentary younging directions determined from delicately preserved cross-bedding (or grading where cross-bedding is absent) indicate the occurrence of a nor-northeast-trending anticline and syncline within the creek, although the axial planes are not exposed. Stereonet intersection of bedding surfaces indicates that the fold axes are close to horizontal.
There are two textural types of Greenland Group present. The first type occurs to the east of the granitoid dike; here, distal (non-hornfelsed) meta-greywacke is dominated by coarse to fine-grained detrital sandstones of moderately to well-sorted sub-angular to well-rounded detrital quartz, plagioclase and K-feldspar. Biotite and epidote occur sporadically and are partially decomposed and may be detrital. Anhedral to subhedral grains of metamorphic muscovite and minor chlorite fill interstitial space in the matrix, and their orientation defines a weak cleavage ((A)). Accessory magnetite is also present. Argillites consist of muscovite, quartz and chlorite, with minor detrital feldspar and biotite. A spaced, disjointed foliation is defined by pressure solution seams and enhanced by preferred alignment of quartz and muscovite. This foliation anastomoses around detrital quartz grains. In textural regard, the Greenland Group east of the dike is identical to ‘Type I’ of Ritchie et al. (Citation2015). Type I Greenland Group begins to display spotted hornfels textures at about 50 m from the dike. The spots are typically composed of biotite ((B)) or pale patches of sericite. The associated plagioclase is andesine to labradorite. Pyrite is present and in one sample was found to be inter-grown with andalusite.
Figure 4. Greenland Group textures. (A) Plane-polarised light image of typical Greenland Group meta-greywacke to the east of the Blackwater porphyry dike. Black lines indicate pressure solution seams. Metamorphic minerals are muscovite (Musc) and chlorite (Chl). (B) Hand specimen of spotted hornfels from adjacent to the dike. The spots are biotite (Bt). (C) Hand specimen from west of the dike showing ductilely deformed Greenland Group. (D) Hand specimen of Greenland Group west of the dike showing pyrite (Py) porphyroclasts as well as carbonate (Cc) veins (typically running across the field of view) and abundant spherical to oblate pale-coloured carbonate spots. (E) Cross-polarised light image showing the pyrite grains and carbonate spots. The pyrite has quartz strain fringes.
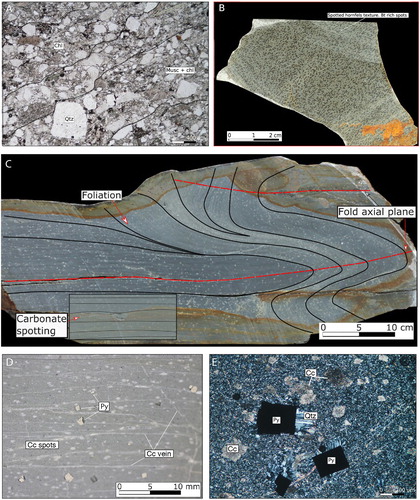
The second textural type of Greenland Group occurs to the west of the dike (), although the effects of subsequent contact metamorphism mean that it is unclear whether the dike is the boundary between the two types. In this area, argillitic lithologies are still dominated by albite and sericite but commonly contain a penetrative foliation and abundant pyrite grains and carbonate ‘spots’ and veins ((C, D)). The pyrite grains have strain fringes that are filled with quartz with or without minor siderite ((E)). Semi-quantitative analysis indicates that the carbonate spots have ankeritic cores and sideritic rims, and the carbonate veins have ankeritic interiors and sideritic margins. Chalcopyrite and cobaltite occur rarely. The argillites are also notable in this area for displaying isoclinal folds. This type of Greenland Group has been hornfelsed at up to 30 m from the granitoid dike, and biotite and oligoclase-andesine-bearing hornfels assemblages have formed.
Hydrothermal alteration surrounding the Blackwater dike extends up to c. 325 m to the west and ∼110 m to the east, and therefore extends beyond the contact aureole margins (). The extent of the halo is here defined by the distal-most observed occurrence of sulphide-bearing quartz vein stockworks. With proximity to the dike, contact metamorphic assemblages are crosscut by quartz-sulphide veinlets that have altered the biotite-bearing hornfels to pale green assemblages of chlorite and sericite ((A)). Illite was identified only by X-ray diffraction, but appears to be widespread. Quartz veins up to 20 cm in width, although more commonly about 2–5 cm, containing plentiful sulphides occur throughout the altered Greenland Group ((B)). A zone of concentrated sulphide-bearing quartz veins is visible over a c. 7 m wide zone within Greenland Group on the western side of the dike. Sulphide-bearing quartz veins decrease in frequency with distance away from this western contact. The veins, however, appear to absent from the immediate eastern side of the Greenland Group-dike contact. These veins commonly contain pyrite with or without chalcopyrite, molybdenite, sphalerite, arsenopyrite, galena and millerite ((C)); the latter six minerals also occur as inclusions in pyrite. Distinctive pyrite-, chalcopyrite- and molybdenite-bearing quartz stockworks and veins define the outermost part of the mineralised zone. The veins are typically 10 cm thick and contain coarse subhedral pyrite grains that have fine bundles of molybdenite on the margins ((D)).
Figure 5. Mineralisation textures. (A) Hand specimen showing bleached Greenland Group formed by biotite destruction adjacent to quartz (Qtz)-pyrite (Py) veins. (B) Hand specimen of a quartz vein cutting spotted hornfelsic Greenland Group. This sample contains abundant molybdenite (Mo) and pyrite at its margin. (C) Back-scattered electron image showing molybdenite growing in fine bundles around pyrite. (D) Back-scattered electron image of a chloritised patch of biotite containing small patches of chalcopyrite (Chc), sphalerite (Sph), galena (Gal) and millerite (Mil). Phases were confirmed by semi-quantitative EDS analysis.
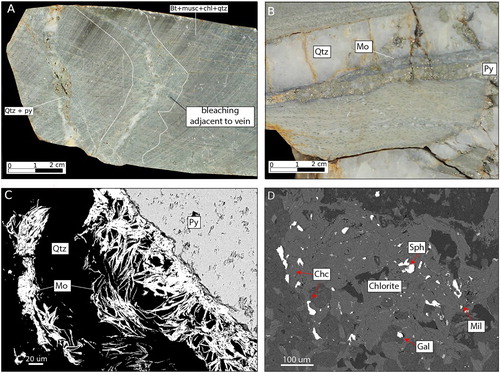
Trace elements in pyrite
The lack of appropriate natural mineral or synthetic metal standard means that the pyrite trace element values should be considered semi-quantitative (). However, the data are comparable to each other. Pyrite grains in the docilely deformed Greenland Group trend towards the highest Au, As and Pb levels compared to the pyrite grains associated with propylitic and phyllic alteration ((A, B)). The quartz-sulphide veins have intermediate values of these inspected elements.
Figure 6. LA-ICP-MS Au, As and Pb trace element measurements on pyrite grains in different settings shows that those from the ductiley deformed Greenland Group contain the highest concentrations of Au and, typically, Pb.
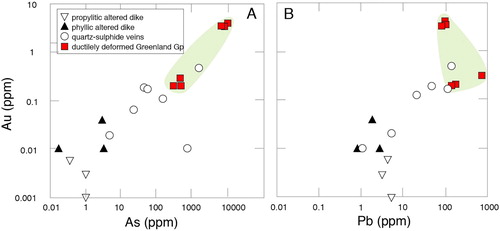
Table 1. Pyrite trace elements by LA-ICP-MS. bdl, below detection limit.
U-Pb Zircon
The zircon grains inspected are euhedral, elongate and terminate in prisms. Cathodoluminescence electron imaging (not shown) revealed them to display the common zircon pattern of oscillatory zoning with some grains containing U-rich (dark) cores. The thirty-four dates obtained range from 106–2306 Ma (). Twenty-five of these are Early Cretaceous, < 5% discordant and comprise a population with an error-weighted mean age of 118.5 ± 1.3 Ma (2σ; mean squared weighted deviation = 0.79) (). The older dates (≥237 Ma), some of which come from the dark cores, are likely inherited zircon. A single 106 Ma date has very high U and therefore may be the result of radiation damage-facilitated Pb loss.
Figure 7. Probability density and histogram diagram showing Early Cretaceous zircon U-Pb dates from the Blackwater dike.
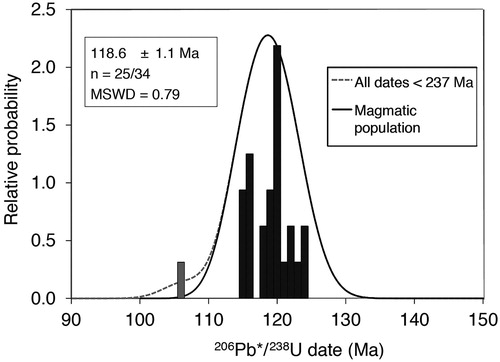
Table 2. Zircon U-Th-Pb data for Blackwater dike.
DISCUSSION
Ductile deformation-related mineralisation
There are broadly two styles of mineralisation in lower Blackwater Creek, the first of which appears related to ductile deformation in the Greenland Group and is overprinted by mineralisation associated with the Blackwater dike (). The ductile deformation-related mineralisation caused the precipitation of pyrite and carbonate, along with minor chalcopyrite and cobaltite, in Greenland Group west of the dike ( and ). The presence of the abundant Au-bearing pyrite porphyroclasts indicates that this phase of mineralisation occurred under ductile conditions and that deformation outlasted sulphide precipitation, although it is acknowledged that there is currently no unmineralised Greenland Group pyrite baseline with which to compare the results. The occurrence of ankeritic-sideritic spots and pyrite porphyroclasts in ductilely deformed Greenland Group is very similar to descriptions provided by Christie and Brathwaite (Citation2003) for Au mineralisation in the General Gordon and Globe-Progress deposits elsewhere in the goldfield. Furthermore, Hamisi et al. (Citation2017) also describe carbonate spotting in drillcore above the Blackwater Birthday Reef. These latter authors also note the occurrence of cobaltite and chalcopyrite but attribute it to metamorphism rather than mineralisation.
Figure 8. Summary of mineral textures, paragenesis and events recorded or inferred from the rocks examined in lower Blackwater Creek.
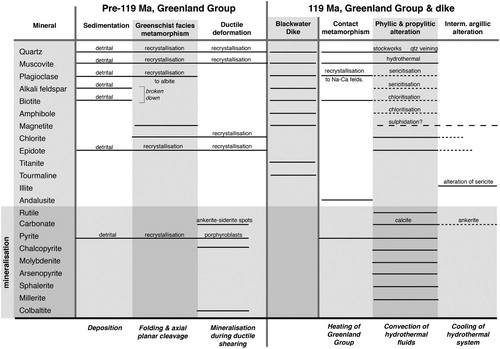
Carbonate porphyroblasts in the Bendigo Belt in the Victorian goldfields have been interpreted as having the ankeritic cores grow during diagenesis and the sideritic rims develop during subsequent CO2-fluxing associated with mineralisation (Dugdale et al. Citation2009). However, if the ankeritic cores represented carbonate growth during diagenesis, then ankerite should be abundant and widespread in all Greenland Group rocks; this is not the case (Laird and Shelly, Citation1974; Nathan, Citation1978; Mortimer et al. Citation2013; Belton and Scott Citation2014; Ritchie et al. Citation2015). The occurrence of the carbonate spots, pyrite porphyroclasts and occurrence of chalcopyrite + cobaltite in the Greenland Group in lower Blackwater Creek is here interpreted to be a result of mineralisation facilitated by ductile deformation. Other occurrences of carbonate spotting and pyrite porphyroclasts, such as documented in the Blackwater drillcore but distal to the Birthday Reef (Hamisi et al. Citation2017), may also represent zones of subtle mineralisation.
Igneous-related mineralisation
The second and most prominent phase of mineralisation in lower Blackwater Creek is the propylitic and phyllic alteration that overprints the biotite-bearing Greenland Group hornfels and Blackwater dike igneous textures. The association of biotite and oligoclase indicates temperatures of close to, or within the hornblende hornfels facies for contact metamorphism, and occurrence of andalusite constrains pressure of alteration to <3.75 kbar. This hydrothermal event caused precipitation of pyrite, chalcopyrite and molybdenite in quartz veins ( and ). Molybdenite is uncommon in the Au-mineralised assemblages in the Reefton Goldfield, but is widely associated with Early Cretaceous felsic plutons elsewhere in Buller and Nelson (Eggers and Adams Citation1979; Tulloch and Rabone Citation1993). The alteration of contact metamorphic assemblages by silicate-metal-bearing fluids is a common feature of porphyry mineralisation systems, and typically relates to the development of a geologically short-lived hydrothermal convection system as the aureole cools (e.g. Sillitoe Citation2010). Alternatively, the mineralisation may have a metamorphic origin and has localised on a pre-existing lithologic contrast, as was proposed for Au mineralisation of the Sams Creek dike in New Zealand (Windle and Craw Citation1991); however, this seems unlikely given the Cu, Mo, As, Zn, Pb and Ni metal assemblages in the Blackwater Creek occurrence.
Age of the Blackwater dike and the timing of mineralisation
The error-weighted mean U-Pb zircon age of 118.5 ± 1.3 Ma () is interpreted to record crystallisation of the Blackwater dike. The age of the dike overlaps the Separation Point and Rahu suites in Westland–Nelson (e.g. Muir et al. Citation1994, Citation1997; Waight et al. Citation1997; Tulloch and Kimbrough Citation2003; Sagar and Palin Citation2011; van der Meer et al. Citation2018). Since the ductile structures are overprinted by contact metamorphic assemblages associated with the dike, ductile deformation-related mineralisation must have occurred prior to dike emplacement at c. 119 Ma. Furthermore, if ductile deformation-related mineralisation in lower Blackwater Creek is related to the ductile phase of mineralisation associated with Au elsewhere noted in the goldfield, then the c. 119 Ma age provides a lower constraint on the timing of this event. Therefore, this mineralisation event cannot be associated with the c. 108 Ma amphibolite facies metamorphism in the Greenland Group recorded in the Waipuna Valley immediately south of the goldfield (Ritchie et al. Citation2015).
Conclusions
Intrusion of the 118.5 ± 1.3 Ma (U-Pb zircon) Blackwater dike in lower Blackwater Creek has contact metamorphosed and then mineralised rocks in the immediate area. Mineralisation is characterised by the formation of quartz-sulphide veins and the formation of pyrite ± chalcopyrite ± molybdenite with minor sphalerite, arsenopyrite, galena and millerite in propylitic- and phyllic-altered zones around this small porphyritic granitoid. An important discovery, is that this igneous phase of mineralisation overprints ductilely deformation-associated mineralisation within the host Greenland Group. The ductile deformation is characterised by Au-bearing pyrite porphyroclasts and carbonate spots, which is similar to ductile deformation-related orogenic Au mineralisation elsewhere in the goldfield. Therefore, the Blackwater dike ∼119 Ma age appears to place a lower constraint on when the ductile stage of orogenic Au mineralisation occurred.
Acknowledgements
Mike Palin helped with laser analyses and Marco Brenna commented on a draft. Tod Waight and Quinten van der Meer are thanked for reviews.
Disclosure statement
No potential conflict of interest was reported by the authors.
ORCID
James M. Scott http://orcid.org/0000-0001-5185-6261
Matt W. Sagar http://orcid.org/0000-0001-8178-1619
Additional information
Funding
References
- Adams CJ, Mortimer N, Campbell HJ, Griffin WL. 2015. Detrital zircon ages in buller and takaka terranes, New Zealand: constraints on early Zealandia history. N Z J Geol Geophys. 58(2):176–201. doi: 10.1080/00288306.2015.1025798
- Belton AD, Scott JM. 2014. P-T-t-D evolution across a Paleozoic greenschist to amphibolite facies transition in Greenland Group rock in south Westland. Annual Conference of the Geosciences Society of New Zealand, p. 8.
- Bierlein FP, Christie AB, Smith PK. 2004. A comparison of orogenic gold mineralisation in central Victoria (AUS), western South Island (NZ) and Nova Scotia (CAN): implications for variations in the endowment of Palaeozoic metamorphic terrains. Ore Geol Rev. 25(1):125–168. doi: 10.1016/j.oregeorev.2003.09.002
- Christie AB, Brathwaite RL. 2003. Hydrothermal alteration in metasedimentary rock-hosted orogenic gold deposits, Reefton goldfield, South Island, New Zealand. Miner Depos. 38(1):87–107. doi: 10.1007/s00126-002-0280-9
- Cooper RA. 1974. Age of the Greenland and Waiuta Groups, South Island, New Zealand (note). N Z J Geol Geophys. 17:955–962. doi: 10.1080/00288306.1974.10418235
- Cumming GL, Richards JR. 1975. Ore lead ratios in a continuously changing Earth. Earth Planet Sci Lett. 28:155–171. doi: 10.1016/0012-821X(75)90223-X
- Dugdale AL, Wilson CJ, Leader LD, Robinson JA, Dugdale LJ. 2009. Carbonate spots: understanding the relationship to gold mineralisation in Central Victoria, southeastern Australia. Miner Depos. 44(2):205–219. doi: 10.1007/s00126-008-0209-z
- Eggers AJ, Adams CJ. 1979. Potassium-argon ages of molybdenum mineralisation and associated granites at Bald Hill and correlation with other molybdenum occurrences in the South Island, New Zealand. Econ Geol. 74(3):628–637. doi: 10.2113/gsecongeo.74.3.628
- Gage M. 1948. The geology of the Reefton quartz lodes. NZ Geological Survey Bulletin 45.
- Hamisi J, MacKenzie D, Pitcairn I, Blakemore H, Zack T, Craw D. 2017. Hydrothermal footprint of the Birthday Reef, Reefton goldfield, New Zealand. N Z J Geol Geophys. 60:59–72. doi: 10.1080/00288306.2016.1274332
- Henderson J. 1917. The geology and mineral resources of the Reefton Subdivision. NZ Geological Survey Bulletin 18.
- Ireland TR. 1992. Crustal evolution of New Zealand: evidence from age distributions of detrital zircons in Western Province paragneisses and Torlesse greywacke. Geochim Cosmochem Acta. 56:911–920. doi: 10.1016/0016-7037(92)90036-I
- Jochum KP, Weis U, Stoll B, Kuzmin D, Yang Q, Raczek I, Jacob DE, Stracke A, Birbaum K, Frick DA, et al. 2011. Determination of reference values for NIST SRM 610-617 glasses following ISO guidelines. Geostandard Newsletter. 35:397–429. doi: 10.1111/j.1751-908X.2011.00120.x
- Laird MG. 1972. Sedimentology of the Greenland Group in the Paparoa Range, West Coast, South Island. N Z J Geol Geophys. 15:372–393. doi: 10.1080/00288306.1972.10422338
- Laird MG, Shelley D. 1974. Sedimentation and early tectonic history of the Greenland Group, Reefton, New Zealand. N Z J Geol Geophys. 17(4):839–854. doi: 10.1080/00288306.1974.10418229
- MacKenzie D, Craw D, Blakemore H. 2014. Multi-stage ore formation at the Reefton goldfield, New Zealand. Aust Inst Min Metall Conf. 47:341–351.
- Milham L, Craw D. 2009. Two-stage structural development of a Paleozoic auriferous shear zone at the Globe-Progress deposit, Reefton, New Zealand. N Z J Geol Geophys. 52(3):247–259. doi: 10.1080/00288300909509889
- Mortimer N, Nathan S, Jongens R, Kawachi Y, Ryland C, Cooper AF, Stewart M, Randall S. 2013. Regional metamorphism of the Early Palaeozoic Greenland Group, South Westland, New Zealand. N Z J Geol Geophys. 56:1–15. doi: 10.1080/00288306.2012.734830
- Muir RJ, Ireland TR, Weaver SD, Bradshaw JD. 1994. Ion microprobe U–Pb zircon geochronology of granitic magmatism in the Western Province of the South Island, New Zealand. Chem Geol. 113:171–189. doi: 10.1016/0009-2541(94)90011-6
- Muir RJ, Ireland TR, Weaver SD, Bradshaw JD. 1996. Ion microprobe dating of Paleozoic granitoids: Devonian magmatism in New Zealand and correlations with Australia and Antarctica. Chem Geol. 127(1):191–210. doi: 10.1016/0009-2541(95)00092-5
- Muir RJ, Ireland TR, Weaver SD, Bradshaw JD, Waight TE, Jongens R, Eby GN. 1997. SHRIMP u–Pb geochronology of Cretaceous magmatism in northwest Nelson–Westland, South Island, New Zealand. N Z J Geol Geophys. 40:453–463. doi: 10.1080/00288306.1997.9514775
- Nathan S. 1978. Upper Cenozoic stratigraphy of South Westland, New Zealand. N Z J Geol Geophys. 21(3):329–361. doi: 10.1080/00288306.1978.10424061
- Nathan S, Rattenbury MS, Suggate RP. 2002. Geology of the Greymouth area: scale 1:250,000. Lower Hutt: Institute of Geological & Nuclear Sciences. Institute of Geological & Nuclear Sciences 1:250,000 geological map 12: 58p.
- Palmer MC, Scott JM, Muhling JR, Kennedy AK. 2015. Carboniferous metamorphism and partial melting of the Greenland Group in the Jackson River valley, south Westland. N Z J Geol Geophys. 58(1):22–32. doi: 10.1080/00288306.2014.982661
- Paton C, Hellestrom J, Paul B, Woodhead J, Hergt J. 2011. Iolite: freeware for the visualisation and processing of mass spectrometric data. J Anal At Spectr. 26:2508–2518. doi: 10.1039/c1ja10172b
- Rattenbury MS, Stewart M. 2000. Structural setting of the Globe-Progress and Blackwater gold mines, Reefton goldfield, New Zealand. N Z J Geol Geophys. 43(3):435–445. doi: 10.1080/00288306.2000.9514900
- Ritchie TW, Scott JM, Muhling JR, Kennedy AK. 2015. Cretaceous metamorphism, magmatism and shearing in the Waipuna Valley, directly south of the Reefton Goldfield. N Z J Geol Geophys. 58(2):89–103. doi: 10.1080/00288306.2014.992444
- Sagar MW, Palin JM. 2011. Emplacement, metamorphism, deformation and affiliation of mid-Cretaceous orthogneiss from the Paparoa Metamorphic Core Complex lower-plate, Charleston, New Zealand. N Z J Geol Geophys. 54(3):273–289. doi: 10.1080/00288306.2011.562904
- Scott J, Muhling J, Fletcher I, Billia M, Palin JM, Elliot T, Günter C. 2011. The relationship of Palaeozoic metamorphism and S-type magmatism on the paleo-Pacific Gondwana margin. Lithos. 127(3):522–534. doi: 10.1016/j.lithos.2011.09.008
- Scott JM, Palin J M. 2008. LA-ICP-MS U-Pb zircon ages from Mesozoic plutonic rocks in eastern Fiordland, New Zealand. N Z J Geol Geophys. 51(2):105–113. doi: 10.1080/00288300809509853
- Shand F, Scott JM. 2016. The Origin of Cu-Mo Porphyry Mineralisation at Cascade Creek, Westland. Proceedings of the 49th Australasian Institute of Mining and Metallurgy Conference 49. 9 p.
- Shand F, Scott JM, Blakemore H. 2015. Structural controls and mineralising fluids at the Big River South - St George Au deposits, Reefton. Aust Inst Min Metall Conf. 48:395–403.
- Sillitoe RH. 2010. Porphyry copper systems. Econ Geol. 105(1):3–41. doi: 10.2113/gsecongeo.105.1.3
- Steiger RH, Jäger E. 1977. Subcommission on geochronology: convention on the use of decay constants in geo- and cosmochronology. Earth Planet Sci Lett. 36(3):359–362. doi: 10.1016/0012-821X(77)90060-7
- Stick G, MacKenzie D, Scott JM. 2015. The energetic gold mine, Reefton goldfield, New Zealand. Aust Inst Min Metall Conf. 48:447–457.
- Suggate RP. 1957. The Geology of Reefton Subdivision:(Reefton Sheet, S 38) No. 56. NZ Department of Scientific and Industrial Research.
- Tulloch AJ, Kimbrough DL. 1989. The Paparoa metamorphic core complex, New Zealand: Cretaceous extension associated with fragmentation of the Pacific margin of Gondwana. Tectonics. 8:1217–1234. doi: 10.1029/TC008i006p01217
- Tulloch AJ, Kimbrough DL. 2003. Paired plutonic belts in convergent margins and the development of high Sr/Y magmatism: Peninsular Ranges batholith of Baja-California and Median batholith of New Zealand. In: Johnson S, Patterson S, Fletcher J, Girty G, Kimbrough D, Martín-Barajas A, editors. Tectonic evolution of northwestern Mexico and the southwestern USA. Boulder (CO): Geological Society of America Special Paper. p. 275–295.
- Tulloch AJ, Rabone SDC. 1993. Mo-bearing granodiorite porphyry plutons of the Early Cretaceous Separation Point Suite, west Nelson, New Zealand. N Z J Geol Geophys. 36(4):401–408. doi: 10.1080/00288306.1993.9514586
- Tulloch AJ, Ramezani J, Kimbrough DL, Faure K, Allibone AH. 2009. U-Pb geochronology of mid-Paleozoic plutonism in western New Zealand: implications for S-type granite generation and growth of the east Gondwana margin. Geol Soc Am Bull. 121:1236–1261. doi: 10.1130/B26272.1
- Turnbull R, Tulloch A, Ramezani J, Jongens R. 2016. Extension-facilitated pulsed SIA-type “flare-up” magmatism at 370 Ma along the southeast Gondwana margin in New Zealand: insights from U-Pb geochronology and geochemistry. Geol Soc Am Bull. 120:1500–1520. doi: 10.1130/B31426.1
- van der Meer QHA, Storey M, Scott JM, Waight TE. 2016. Abrupt spatial and geochemical changes in lamprophyre magmatism related to Gondwana fragmentation prior, during and after opening of the tasman Sea. Gondwana Res. 36:129–143. doi: 10.1016/j.gr.2016.04.004
- van der Meer QHA, Waight TE, Tulloch AJ, Whitehouse MJ, Andersen T. 2018. Magmatic evolution during the Cretaceous transition from subduction to continental break-up of the eastern Gondwana margin (New Zealand) documented by in-situ zircon O-Hf isotopes and bulk-rock Sr-Nd isotopes. J Petrol. 59:849–880. doi: 10.1093/petrology/egy047
- Waight TE, Weaver SD, Ireland TR, Maas R, Muir RJ, Shelley D. 1997. Field characteristics, petrography, and geochronology of the Hohonu Batholith and the adjacent Granite Hill complex, North Westland, New Zealand. N Z J Geol Geophys. 40(1):1–17. doi: 10.1080/00288306.1997.9514736
- Waight TE, Weaver SD, Muir RJ. 1998. Mid-Cretaceous granitic magmatism during the transition from subduction to extension in southern New Zealand: a chemical and tectonic synthesis. Lithos. 45:469–482. doi: 10.1016/S0024-4937(98)00045-0
- Windle SJ, Craw D. 1991. Gold mineralisation in a syntectonic granite dike, Sams Creek, northwest Nelson, New Zealand. N Z J Geol Geophys. 34:429–440. doi: 10.1080/00288306.1991.9514481