ABSTRACT
At the southern Taupo Rift, tectonic faults and volcanic edifices have interacted during the last 350 kyr. The NNE-striking Tongariro Volcanic Complex is bounded and displaced by four parallel normal faults: National Park, Waihi, Poutu and Upper Waikato Stream, which collectively define the Tongariro Graben. Offsets of geomorphic surfaces of known age and fault trace geometry were used to characterise the seismic parameters of these faults. Along-strike distribution of fault displacement suggests that these faults could rupture as a whole. However, geomorphic data and geometrical characteristics of surface ruptures suggest that they could also rupture in shorter segments. This study calculates higher slip-rates (∼2.6 ± 0.8 and 2.2 ± 1.9 mm/yr for Waihi and Poutu fault zones, respectively) and greater earthquake magnitudes (up to MW 7.2 ± 0.1 and 6.9 ± 0.1, respectively) than previous studies, suggesting an important seismic hazard for the Tongariro area.
Introduction
The Tongariro Volcanic Complex in the central North Island of New Zealand is a very popular tourist attraction and site of one of the most popular alpine walkways in New Zealand, a UNESCO World Heritage Centre hosting up to 7000 people per day (Jolly et al. Citation2014). This area contains an active NNE-striking volcano vent corridor bounded by active normal faults (), and thus it is exposed to volcanic and tectonic hazards (Gregg Citation1964; Topping Citation1973; Gómez-Vasconcelos et al. Citation2017).
Figure 1. A, Location map of the Taupo Volcanic Zone in the North Island of New Zealand. B, Location of the Tongariro Graben and the Tongariro Volcanic Complex. Black and green dots represent 221 fault displacement measurements (117 Waihi Fault and 110 Poutu Fault) from geomorphic surfaces (see ) on individual traces. Individual displacements have been added across seventeen transects on each fault. Transects are marked with white lines. Field outcrops are marked with blue dots. UWS, Upper Waikato Stream. For more details about displacements, field outcrops, lithology and surface ages see Gómez-Vasconcelos et al., Citation2017.
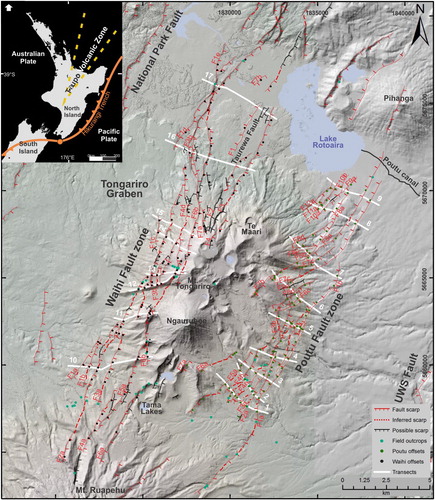
There have been many stratigraphic and volcanic studies in this region (Topping Citation1973; Donoghue et al. Citation1995; Cronin et al. Citation1997; Moebis et al. Citation2011; Pardo et al. Citation2012; Eaves Citation2015; Conway et al. Citation2016). Volcanic activity in the NNE-striking Tongariro Volcanic Complex has been more or less continuous since ∼350 ka (Gregg Citation1964; Tost et al. Citation2016), involving various eruption types and the emplacement of lava flows, debris avalanches, debris flows and pyroclastic deposits (Gregg Citation1964; Cronin et al. Citation1997; Procter et al. Citation2010; Pardo et al. Citation2012).
The Waihi and Poutu fault zones () are active normal faults which collectively form the Tongariro Graben (Gómez-Vasconcelos et al. Citation2017). A comprehensive seismic source charactersation for these major fault zones using that recent study (Gómez-Vasconcelos et al. Citation2017) has not yet been undertaken. Here we evaluate their geometry, slip-rates and segmentation, which could have important implications for seismic hazard assessment in the region.
Paleoseismic data provides input for fault source characterisation for seismic hazard assessment in the Tongariro Graben. We assign magnitudes and recurrence rates for large (MW > 6), potentially damaging earthquakes that could be generated by active faults in this area. Paleoseismic trenching is not permitted in the Tongariro National Park; therefore, fault source characterisation is based mainly on geomorphological analyses and scarce natural fault exposures.
Tectonic setting and previous active faulting studies
The Taupo Volcanic Zone (TVZ) is an NNE-striking Quaternary volcanic arc in the North Island of New Zealand that is associated with the Hikurangi subduction zone, where the Pacific Plate subducts below the Australian Plate (). Extension within the arc is caused by the current fore arc clockwise rotation (Wallace et al. Citation2004) as well as active slab rollback (Seebeck et al. Citation2014). Faults within the volcanic arc define the intra-arc Taupo Rift (Acocella et al. Citation2003).
The Tongariro Volcanic Centre (TgVC) represents the southernmost volcanic centre of the TVZ, with two volcanic complexes dominating the landscape: Mt. Ruapehu and the Tongariro Volcanic Complex. The Tongariro Volcanic Complex is an NNE-striking andesite volcano complex, with vents aligned along the same strike as the Tongariro Graben axis (Topping Citation1973; Rowland and Sibson Citation2001; Villamor and Berryman Citation2006). Four major NNE-striking parallel faults make up the Tongariro Graben: two rift-bounding faults, namely the National Park Fault and the Upper Waikato Stream Fault; and two intra-rift faults, namely the Waihi and the Poutu faults (Van Dissen et al. Citation2003; Lecointre et al. Citation2004; Villamor and Berryman Citation2006; Cassidy et al. Citation2009; Gómez-Vasconcelos et al. Citation2017).
The National Park Fault is an active NNE-striking normal fault that bounds the western Tongariro Graben and is downthrown to the SE. Ruptures along this fault have been characterised as producing MW 6.1 earthquakes every 4000 years (Stirling et al. Citation2012). The Upper Waikato Stream Fault is an active NE-striking normal fault that bounds the eastern Tongariro Graben and is downthrown to the NW, with a minimum slip-rate of 0.5 mm/yr over the last 45 kyr (from fault geometry, kinematics and faulting history; Gómez-Vasconcelos et al. Citation2016). This fault can produce MW 6.5 earthquakes every >1600 years if it ruptures by itself (15 km), or MW 7.1 if it ruptures together with the Rangipo and Kaimanawa faults (43 km; fault scaling relationships from Villamor et al. Citation2007 and Stirling et al. Citation2012; Gómez-Vasconcelos et al. Citation2016).
The Waihi Fault zone is an active NNE-striking normal fault zone on the western flanks of the Tongariro Volcanic Complex, downthrown to the SE (Grindley et al. Citation1961; Van Dissen et al. Citation2003). The Waihi Fault zone has been associated with historical earthquakes and landslides in the north-western TgVC (Hochstein Citation1995). The Hipaua Steaming Cliffs near Little Waihi village, at the south-western edge of Lake Taupo, have suffered devastating landslides in 1846 and 1910, each after MW 6.3 earthquakes on the Waihi Fault (Hegan et al. Citation2001; Cooper Citation2002). Stirling et al. (Citation2012) associate ruptures along this fault zone with MW ∼6.5 earthquakes and recurrence intervals between 490 and 1380 years.
The Poutu Fault zone is an active NNE-striking normal fault zone on the eastern flanks of the Tongariro Volcanic Complex (Villamor and Berryman Citation2006), downthrown both to the SE and to the NW, forming a small graben feature within the wider Tongariro Graben (). At the Poutu Canal, east of Lake Rotoaira, Van Dissen et al. (Citation2003) calculated a ≤2000 years recurrence interval for surface rupture of the Waihi and Poutu fault zones based on slip-rates and surface length estimations from faults in an area 85 km to the north, within the Taupo Rift. Stirling et al. (Citation2012) associate ruptures along the Poutu Fault zone with MW 6.6 earthquakes every 550 years. It is important to note that the Poutu Fault zone parameters in Stirling et al. (Citation2012) are very generalised due to lack of detailed information, and the general ‘one size fits all type’ approach in that study (Mark Stirling pers. comm, 2018).
The Waihi and Poutu fault zones are in close proximity (∼5 km) to the location of recently active (i.e. within the last 10000 years) vents in the Tongariro Volcanic Complex (; e.g. Ngauruhoe, Mt. Tongariro and Te Maari craters), suggesting a potential relationship between active faulting and magmatic processes (Gómez-Vasconcelos et al. Citation2017). In some volcanic environments, extensional fault surface displacement can be a response to arrested dike intrusion (e.g. 2005 Dabbahu rifting episode in Afar, and Krafla fissure swarm in Iceland; Wright et al. Citation2006; Paquet et al. Citation2007) unrelated to large/moderate seismogenic (crustal) fault ruptures. Those fault traces should not be used to assess seismic hazard. Gómez-Vasconcelos et al. (Citation2017) explored the origin of faulting in the Tongariro Volcanic Complex and concluded that the normal faults are essential of tectonic origin and thus their deformation histories should be considered for seismic hazard assessment. Those authors distinguished two types of seismogenic ruptures along these faults: pure tectonic faulting that is unrelated to magmatic processes; and hybrid faulting that is tectonic faulting triggered or promoted by deep-seated dike intrusion.
Fault slip-rates
The spacing between single fault traces within each fault zone is between ∼0.2 and 1 km, much shorter than the seismogenic width (12–15 km) and thus they are likely to merge into a single fault plane at depth, as suggested by Villamor and Berryman (Citation2001) and Nicol et al. (Citation2010) for structures with similar fault spacing in the central TVZ. If the surface fault traces represent the whole fault, the summation of displacements on all traces characterises the total deformation of the fault. Eight transects across the Waihi Fault zone and nine across the Poutu Fault zone (; Gómez-Vasconcelos et al. Citation2017) were used to estimate the cumulative throw accommodated since 100 ka. For the Waihi Fault zone, this amounted to an average of 28.0 ± 3.6 m since 20 ka and 173.0 ± 10.9 m since 60 ka. For the Poutu Fault zone, an average of 65.0 ± 9.3 m since 20 ka and 178 ± 14 m since 60 ka was measured (). This total displacement provided the basis for slip-rate calculations, leading to mean slip-rates of 2.6 ± 0.8 mm/yr and 2.2 ± 1.9 mm/yr, for the Waihi and Poutu fault zones, respectively ().
Table 1. Vertical displacements and slip-rates summary for the Tongariro Graben, from Waihi and Poutu fault zones transects and rift-bounding faults (from Gómez-Vasconcelos et al., Citation2017).
Methods
In order to evaluate the possible fault segmentation, the along-strike slip distribution for the Waihi and Poutu fault zones was analysed (). Transverse topographic profiles (n = 221) were traced to measure each fault trace offset from geomorphic surfaces of known age using a 2 m-resolution photogrammetry-based digital surface model (see Gómez-Vasconcelos et al. Citation2016). The mean slope angle of the displaced surface was obtained using trigonometric functions. The offsets at any one location were measured several times and averaged. To obtain the total displacement at several locations along the faults, measured displacements of each age along small sections of the fault zones were averaged and interpolated to 20–40 ka (for those values obtained in surfaces different to the 20–40 ka ones), and then summed across the fault zone (see Tables A1 and A2).
Figure 2. Along-strike fault slip distribution for the Waihi (A) and Poutu (B) fault zones. Bottom graph of each panel: The vertical axis displays individual fault strand vertical surface offsets of geomorphic surfaces for five different periods. Displacements for the same fault strand are connected with lines of different colours for different ages: blue for 0–20 ka, red for 20–40 ka, green for 40–60 ka, purple for 60–100 ka and black for >100 ka. Top graph of each panel: To obtain the total displacement for the 20–40 ka period at several locations along the faults, individual fault displacements were combined (summed) across transects perpendicular to the fault at those locations (see of transect locations). Within a transect, individual fault strands displace surface of different ages; therefore measured displacements of surfaces different to the 20–40 ka one, were interpolated to 20–40 ka. Summed values for the 20–40 ka period are used to evaluate the displacement variability along the faults and try to identify segmentation patterns (see Tables A1 and A2).
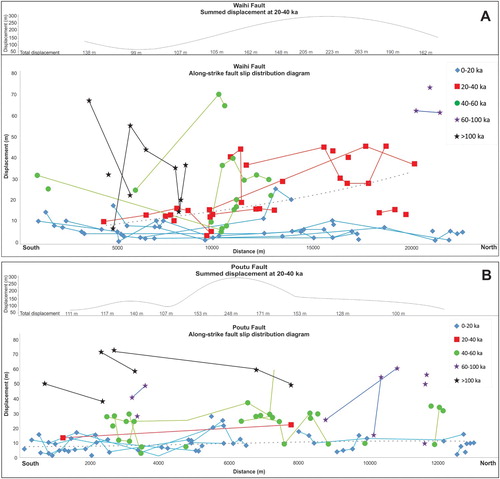
Earthquake moment magnitudes (MW) were calculated using the fault-scaling relationship developed for the Taupo Volcanic Zone (Villamor et al. Citation2007; Stirling et al. Citation2012), based on subsurface-rupture length (MW = 4.80 + 1.33 × log [subsurface rupture length]). For this work, fault lengths were measured based on fault displacements, geomorphology and field observations. To derive the Single Event Displacement (SED) from slip-rate and MW, we have calculated the fault plane area from downdip width and surface-rupture length. For the fault width, we have used published seismogenic thickness values. A 12 ± 2 km-thick seismogenic crust for the region was suggested by Stirling et al. (Citation2012). However, for the Rangipo Fault a 15 ± 2 km seismogenic crust was used by Villamor et al. (Citation2007; also used in Stirling et al. Citation2012). Reyners (Citation2010) showed a pronounced change in crustal thickness in the vicinity of Mt Ruapehu. That change may also represent an increase in seismogenic crustal thickness of the brittle crust, as earthquake activity also increases in depth to the south. We have thus estimated fault parameters using the two published values to address epistemic uncertainty, consistent with the predominant earthquake depths in this region (10–16 km; Hurst and McGinty Citation1999; Hayes et al. Citation2004; Stirling et al. Citation2012). Fault plane area also depends on the fault dip, where lower dip angles will result in larger fault areas and steeper dip angles would result in shorter areas; furthermore, lower fault dip angles will result in shorter SED, and vice versa. In the Tongariro Graben most of the faults mapped showed very steep dips (60°–90°), nevertheless we suspect that this geometry changes with depth, and faults tend to decrease their dip within hundreds of metres below the surface. For these reasons we applied a 60° average for fault dip, which was based on other studied faults within the Taupo Rift (e.g. Villamor and Berryman Citation2001; Nicol et al. Citation2006; Mouslopoulou et al. Citation2008).
Fault recurrence intervals were calculated (after Wallace Citation1970) as RI = AD/SR, where AD is the average displacement for each segment and SR is the slip-rate in mm/yr for each segment. For propagating the uncertainty in the slip-rate, the recurrence interval was averaged from the minimum and maximum recurrence intervals. SED could not be obtained from field data, so SED was derived from the fault area based on the seismic moment (MO) using the equation of Aki and Richards (Citation1980) (SED = [MO] / [fault area * 2.52E + 23]). Nevertheless, fault area could change if we use a different fault dip, where lower fault dip angles will result in shorter recurrence intervals, and vice versa. Mo is calculated from the MW by the equation of Hanks and Kanamori (Citation1979).
Results
Fault geometry
The Waihi Fault zone comprises 19 fault strands (three of which were previously defined as Taurewa Fault; Lecointre et al. Citation2002). They are all NNE-striking (average strike of 023°) normal faults and all dip to the southeast, except one antithetic fault. The fault zone is at least 2.5 km wide and 38 km in length, from the south-western shore of Lake Taupo to the north-western slopes of Mt. Ruapehu. The fault geometric pattern changes along the fault: the southern section has a ∼025° strike, while the northern section has a ∼000° strike. Some fault strands bound tilted blocks (e.g. Waihi F3b to the northwest; Miller and Williams-Jones Citation2016) and have very steep dips (>80°), while others show en-echelon geometry, fault splaying and non-parallel faulting.
The Poutu Fault zone consists of 23 parallel NNE-striking (average 040°) normal fault strands; 11 of them dip to the SE, while the others dip to the NW forming a graben-like structure. This fault zone is at least 3.5 km wide and 30 km long, extending from the northern part of Pihanga to the northeast slopes of Mt. Ruapehu. The fault geometric pattern changes along the fault: the southern section of the fault has a ∼060° strike, while the northern section has a ∼025° strike. Some fault strands dip at >70° in outcrop; some show en-échelon geometry, horsetail fault splaying, with soft-linked fault arrays and sometimes non-parallel faulting, especially to the north, near Lake Rotoaira ().
The Waihi and Poutu fault zones converge towards the south, narrowing the Tongariro Graben. To the north, adjacent to the Te Maari vents, the graben widens slightly: the Waihi Fault zone displays ∼N-striking fault strands and the Poutu Fault zone displays ∼060-striking fault strands, splaying slightly outwards of the graben ().
Fault slip distribution along the Waihi and Poutu fault zones
To assess possible segmentation along the Waihi and Poutu fault zones, displacements measured along the different traces of the faults were analysed for areas where there may be relevant changes in slip along-strike (, Tables A1 and A2). The slip along-strike diagram displays surface vertical offsets of individual fault strands for geomorphic surfaces of five different periods: 0–20 ka, 20–40 ka, 40–60 ka, 60–100 ka and >100 ka. To obtain the total displacement for the 20–40 ka period at several locations along the faults, individual fault displacements were summed along transects perpendicular to the fault at those locations. Summed values for the 20–40 ka period are used to evaluate the displacement variability along the faults to identify segmentation patterns. The maximum displacement for the Poutu Fault zone occurs near the middle of the mapped length and for the Waihi Fault zone it is slightly off-centre, towards the north ().
The data show that individual fault strands have variable displacements for different aged surfaces along the Waihi and Poutu fault zones. For example, displacements along the Waihi Fault increase for 20–40 ka displacements in the northern part of the fault zone. The Poutu Fault zone does not show a consistent pattern of displacement variation with age (increase or decrease) along individual fault strands ().
Discussion
Fault segmentation
Segment terminations along normal faults, such as the Waihi and Poutu fault zones, act as boundaries to rupture and their comprehension is important to fault source characterisation.
The lack of historic fault surface ruptures or paleoseismic trenches along the entire length of the Waihi and Poutu fault zones, together with the complexity of their surface expression in this region, make the fault segmentation uncertain. Different possible scenarios are suggested for segmentation (surface rupture length) for each of the fault zones, based mainly on the slip distribution along fault strike and the fault geometry (fault strike, morphometry and distribution; ).
Figure 3. Potential segment surface-length rupture models for the Waihi and Poutu fault zones, marked with purple and green lines, respectively. Ng, Ngāuruhoe; RC, Red Crater; NC, North Crater; Ph, Pihanga.
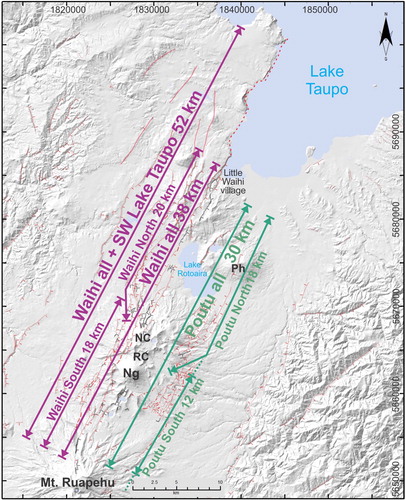
The higher slip-rate on the northern Waihi Fault zone could be a consequence of fault segmentation. The northern segment could rupture as a separate fault source with either larger single event displacement, or more frequent events. Alternatively, the larger slip-rate in the north could be an underestimation of the surface ages (see more on surface ages in Gómez-Vasconcelos (Citation2017) and Townsend et al. (Citation2017); ).
Total displacements along-strike of the Waihi and Poutu fault zones suggest that the faults could rupture their entire length (). In general, both faults have a central higher displacement that diminishes toward the tips, displaying a bell-shaped curve, similar to that defined by Morley and Wonganan (Citation2000) for ‘classical’ normal faults. For the 20–40 ka period, the Waihi Fault zone has slightly greater total displacement to the north, creating a central-north modal curve, while Poutu Fault zone has slightly greater displacements in the central part, creating a central-modal curve, although there is a possible sub-mode in the southern part of the fault zone. These results suggest that the increase in slip-rate for the 20–40 ka surface on the northern part of the Waihi Fault zone might be a consequence of the way the deformation (extension) is distributed across the Tongariro Graben.
This is a complex area were both normal faulting and dike intrusion are accommodating rift extension. Apart from the Waihi and Poutu fault zones, extension here is also accommodated by the rift bounding faults (National Park and Upper Waikato Stream faults) and the dikes in the middle of the graben comprising the Tongariro Volcanic Complex (Gómez-Vasconcelos et al. Citation2017). It is possible that in the rift sections where there is less deformation accommodated by the Waihi Fault zone, either the Poutu Fault zone or the rift-bounding faults, or the axial dikes may be accommodating more deformation than in other sectors. The partitioning of deformation across all these elements in this part of the Taupo Rift may not only vary along strike, but could also vary temporarily for each section depending on magma production changes (Gómez Vasconcelos Citation2017; Gómez-Vasconcelos et al. Citation2017).
The slight increase of the maximum displacement along the Waihi Fault zone to the north could also suggest that the fault extends further north than is currently mapped, along the linear western shore of Lake Taupo. If this is the case, the displacement could taper off beyond our northernmost transect ().
The along-strike changes in fault geometry used in this work to assess possible fault segmentation are the continuity of faults and changes in fault strike (e.g. DuRoss et al. Citation2016). Where fault zones change strike, new faults are created in the bends connecting fault strands in relay ramps. These bends could arrest a propagating rupture, creating a segment boundary (e.g. King and Yielding Citation1984).
The whole Waihi Fault zone is at least 38 ± 2 km in length and up to 52 ± 2 km if it extends along the south-western shore of Lake Taupo, including traces mapped north of the study area (; Langridge et al. Citation2016). Based on its geometry, the Waihi Fault zone can be divided into two or more segments. The Waihi South segment is 18 ± 2 km long and is based on relatively continuous ∼025-striking parallel fault strands (). The change in fault strike and displacement increment is used here to define the possible northern boundary of the segment. Therefore, the fault segment terminates against ∼N-striking fault strands, c. 1 km north of North Crater. The Waihi North segment is 20 ± 2 km long. It starts with the ∼N-striking fault strands, changing to ∼025-striking rift-parallel fault strands to the north and terminating close to Lake Taupo. The fault segment termination is based on the abrupt rift variation that occurs at the Taupo Domain (Rowland and Sibson Citation2001), involving greater slip-rates (Wallace et al. Citation2004) and higher heat flux (Rowland et al. Citation2010). If this segmentation of the Waihi Fault is correct, then there needs to be a third segment to the north, along the SW Lake Taupo shore, as far north as the caldera collapse margin associated with the 26.5 ka BP Ōruanui eruption (Wilson et al. Citation2005). In this study, we have not examined this possible third segment because, if present, it requires an understanding of the fault expression within the lake.
The whole Poutu Fault zone is at least 30 ± 2 km in length and it can be divided in two segments. The Poutu South segment is 12 ± 2 km long and is based on relatively continuous ∼025-striking parallel fault strands. If the seismogenic crustal thickness in this area is 12 km (Stirling et al. Citation2012), this fault length is the minimum expected for a surface rupturing fault, as the aspect ratios length/width for fault sources are ≥1 (Stirling et al. Citation2012). However, if the seismogenic crust is 15 km-thick (Hurst and McGinty Citation1999; Hayes et al. Citation2004; Reyners Citation2010), this segment is expected to be at least as long as the thickness of the seismogenic crust. Some fault traces in the southernmost area displace younger sediments (e.g. debris fans or alluvium), but do not currently have a geomorphic expression (Gómez-Vasconcelos et al. Citation2016), thus erosion likely masks fault geomorphic expression in the South segment of the Poutu Fault zone. Some of the mapped ‘possible faults’ in the south that head towards the Mount Ruapehu crater, could be part of the Poutu South segment. The Poutu North segment is 18 ± 2 km long. In the south, there are ∼060-striking fault strands, changing to ∼025-striking rift-parallel fault strands to the north and terminating 5 km south of Lake Taupo, to the north of Pihanga volcano (). Changes in fault strike coincide with a decrease in slip at the northern part of this fault ().
Table 2. Moment magnitude and single-event displacement for different fault lengths.
Even though the fault history record is insufficient (no historic surface ruptures and or detailed paleoseismic trenches along the faults) to determine segment terminations, we think the segment boundaries defined here are non-persistent. Rupture termination at these locations may occur only in some ruptures because there is not a significant change in displacement or in slip-rate between the two segments proposed here. The along-strike slip distribution shows a small decrease in total fault displacement where this fault bend/segment boundary occurs (e.g. Poutu Fault zone).
Magnitude and recurrence interval of potential earthquakes
Because of the spatial coincidence between faults and volcanoes, the seismicity in this area is very complex, involving tectonic, volcano-tectonic, volcanic earthquakes and swarm activity (Hurst and McGinty Citation1999; Miller and Savage Citation2001; Reyners Citation2010). There have not been large main shocks with surface rupture in historic time in this area. An MW 6.3 earthquake occurred in 1846 at the south-western edge of Lake Taupo (Hegan et al. Citation2001; Cooper Citation2002), but there is no information about any surface rupture. Therefore, fault segment length is used here for an estimation of magnitudes of future potential earthquakes ().
If the entire length of the Waihi Fault zone (38 ± 2 km) were to rupture at once this would produce an MW 7.0 ± 0.1 earthquake, with an average single-event displacement of 1.8 ± 0.1 or 1.5 ± 0.1 m for 12 and 15 km-thick seismogenic crust, respectively (see Methods section). For a whole Poutu Fault zone rupture (30 ± 2 km-long), an MW 6.9 ± 0.1 earthquake could occur, with an average displacement of 1.5 ± 0.1 or 1.2 ± 0.1 m for 12 and 15 km-thick seismogenic crust, respectively. The worst-case scenario is a 52 ± 2 km-long rupture of the whole Waihi Fault zone (including the Waihi North segment along the SW boundary of Lake Taupo), generating an MW 7.2 ± 0.1 earthquake, with an average single-event displacement of 2.6 ± 0.1 or 2.1 ± 0.1 m for 12 and 15 km-thick seismogenic crust, respectively (; ). Short- or single-segment ruptures of the Waihi Fault zone could generate an MW 6.5 ± 0.1 earthquake (), in accordance with the 1846 MW 6.3 event, which was retrospectively assigned to the Waihi Fault zone (Scott and Potter Citation2014).
The information obtained from progressive displacements of geomorphic surfaces of different ages () is not precise enough to assess recurrence intervals (Gómez-Vasconcelos et al. Citation2017). This is because fault rupture ages are not well constrained by stratigraphic markers and obtained time intervals are larger than the likely recurrence interval for fault rupture. Gómez-Vasconcelos et al. (Citation2017) identified at least 19 events occurring over the last 200 kyr for the Waihi Fault zone and at least 15 events for the Poutu Fault zone over the same period. This estimate of surface-rupture events is assumed to be an underestimate because of the large difference in age of the chronological markers and the unreasonably large progressive displacements obtained (>5 m).
Fault rupture recurrence intervals were thus derived from slip-rates and average single-event displacements. When slip-rates are combined with the derived average displacements, recurrence intervals of 570 ± 100 and 540 ± 1100 years are obtained for the Waihi (MW 7.0) and Poutu (MW 6.9) fault zones, respectively, for a 15 km-thick seismogenic crust and a whole fault rupture; (). For the possible largest rupture along the Waihi Fault zone (including the Lake Taupo section; MW 7.2), the recurrence interval is 790 ± 130 years. If the Waihi and Poutu fault zones were to rupture by segments (), their recurrence intervals would be shorter: 280–300 and 190–280 years, respectively. This suggests that MW 6.3–6.6 earthquakes could be twice as frequent as MW 6.9–7.0 earthquakes in the Tongariro Graben (). Note that the calculations above are for a 15 km-thick seismogenic crust and a 60° averaged fault dip (see methods). Values for a 12 km-thick seismogenic crust are in .
Updated fault source parameters for the Tongariro Graben
Earlier studies on the Waihi Fault zone estimated mean slip-rates of 1.1 mm/yr (Lecointre et al. Citation1998) and 2 ± 0.5 mm/yr (Litchfield et al. Citation2013), with a MW 6.4–6.6 ‘characteristic’ earthquake and a recurrence interval of 500–1400 (Stirling et al. Citation2012) or 2000 years (Van Dissen et al. Citation2003). Litchfield et al. (Citation2013) defined the Waihi East and Waihi East fault segments instead of our Waihi North segment, proposing a 2 ± 0.5 mm/yr slip-rate for both Waihi North and Waihi South segments. Earlier studies on the Poutu Fault zone estimated a mean slip-rate of 1.85 mm/yr (Litchfield et al. Citation2013), a ‘characteristic’ MW 6.6 earthquake and a recurrence interval of 550 (Stirling et al. Citation2012) or 2000 years (Van Dissen et al. Citation2003).
The new results outlined here yield higher slip-rates (∼2.6 ± 0.8 and 2.2 ± 1.9 mm/yr) for Waihi and Poutu fault zones, respectively, and consider possible multi-segment ruptures that yield larger magnitudes (up to MW 7.2 ± 0.1 and 6.9 ± 0.1 for Waihi and Poutu fault zones, respectively) than earlier studies. If single segment ruptures are considered, the magnitudes obtained in this study are similar to those previously published. The potential occurrence of large earthquakes (multi-segment) is the largest difference with previous studies and suggests more local damage and secondary effects (e.g. landslides) during this type of events than previously thought (large earthquake damaging scenario). However, large ruptures are less frequent (RIs of ∼540–710 years for the whole Waihi and Poutu fault zones; and 790–980 years for a possible maximum event of MW 7.2) than short ruptures (190–450 years; ). As we proposed above, it is likely that both types of ruptures may occur and thus the final impact on the hazard can only be assessed when these fault sources are built into hazard calculations. For probabilistic seismic hazard assessment and if the slip-rate is distributed among short and long ruptures, it is likely that the hazard (e.g. hazard curves) could be lower than the current NSHM for a short return period (e.g. 500 years), but perhaps higher for longer return periods (e.g. 2500 years).
Our results are based on a detailed geomorphic analysis with high resolution maps, not previously available for this region, and a better understanding of the age of geomorphic surfaces (Leonard et al. Citation2014; Gómez-Vasconcelos et al. Citation2017; Townsend et al. Citation2017). These data and approach address uncertainties on slip-rates, magnitudes and fault segmentation, which can be used to update the National Seismic Hazard Model for New Zealand (Stirling et al. Citation2012; Litchfield et al. Citation2013) and can provide an updated assessment of hazard, relevant to the population and regional infrastructure.
Conclusions and recommendations
New characterisation of the faults in the Tongariro Graben, central North Island, indicates a 2.6 ± 0.8 mm/yr mean slip-rate for the Waihi Fault zone and 2.2 ± 1.9 mm/yr for the Poutu Fault zone; these values are 19% to 136% greater than previous estimates. In the case of multi-segment rupture, the Waihi and Poutu fault zones are capable of producing MW 7.0 (could be up to 7.2) and 6.9 earthquakes, with recurrence intervals of 570 ± 100 (790 ± 130 for MW 7.2) and 540 ± 1100 years, respectively. Along-strike distribution of fault displacement in the Tongariro Graben suggests that the Waihi and Poutu fault zones each could rupture as a whole structure. However, fault trace geometry suggests that these faults could also rupture in shorter segments. If these faults rupture in segments, recurrence intervals would be shorter and magnitudes would be lower, increasing the short-term seismic hazard in the region. We recommend that these updated values be integrated into the National Seismic Hazard Model for New Zealand. Although more detailed paleoseismic studies on the Waihi and Poutu fault zones are needed to better understand their segmentation; the geologic, geometric and behavioural characteristics identified here are key elements in understanding the dynamics of these fault zones and improving the current characterisation of the seismic hazard of the Tongariro Graben.
TABLE A2. FAULT STRANDS VERTICAL DISPLACEMENT AND ALONG-STRIKE SLIP DISTRIBUTION CALCULATIONS FOR THE POUTU FAULT
Download PDF (156.5 KB)TABLE A1. FAULT STRANDS VERTICAL DISPLACEMENT AND ALONG-STRIKE SLIP DISTRIBUTION CALCULATIONS FOR THE WAIHI FAULT
Download PDF (161.8 KB)Acknowledgements
We thank Anja Moebis, Diana Cabrera, Agnes Samper, Denis Avellán, Alderik, Kate Arentsen, Nicola Litchfield and Ivan Martin for their support during field work, laboratory analysis and stratigraphic identification. We also want to acknowledge the Volcanic Risk Solutions group in Massey University and the Department of Conservation staff of Tongariro National Park. We thank the reviewers (Mark Stirling) and the associate editor of the New Zealand Journal of Geology and Geophysics (Timothy Little) for their comments that greatly improved the presentation of our data.
Disclosure statement
No potential conflict of interest was reported by the authors.
ORCID
Martha Gabriela Gómez-Vasconcelos http://orcid.org/0000-0001-9722-4198
Shane Cronin http://orcid.org/0000-0001-7499-603X
Additional information
Funding
References
- Acocella V, Spinks K, Cole J, Nicol A. 2003. Oblique back arc rifting of Taupo Volcanic zone, New Zealand. Tectonics. 22(4):1045. doi: 10.1029/2002TC001447
- Aki K, Richards P. 1980. Quantitative seismology—theory and methods. Vol 1. New York: WH Freeman & Co.
- Cassidy J, Ingham M, Locke CA, Bibby H. 2009. Subsurface structure across the axis of the Tongariro Volcanic Centre, New Zealand. J Volcanol Geotherm Res. 179(3):233–240. doi: 10.1016/j.jvolgeores.2008.11.017
- Conway CE, Leonard GS, Townsend DB, Calvert AT, Wilson CJ, Gamble JA, Eaves SR. 2016. A high-resolution 40 Ar/39 Ar lava chronology and edifice construction history for Ruapehu volcano, New Zealand. J Volcanol Geotherm Res. 327:152–179. doi: 10.1016/j.jvolgeores.2016.07.006
- Cooper L. 2002. Hipaua steaming cliffs and Little Waihi landslide. In: Chandrika K, editor. Thepra: earth movements. Vol. 19. Wellington, New Zealand: Ministry of Civil Defense. p. 42–47.
- Cronin SJ, Hodgson K, Neall V, Palmer A, Lecointre J. 1997. 1995 Ruapehu lahars in relation to the late Holocene lahars of Whangaehu River, New Zealand. N Z J Geol Geophys. 40(4):507–520. doi: 10.1080/00288306.1997.9514780
- Donoghue S, Neall V, Palmer A. 1995. Stratigraphy and chronology of late quaternary andesitic tephra deposits, Tongariro Volcanic Centre, New Zealand. J R Soc N Z. 25(2):115–206. doi: 10.1080/03014223.1995.9517487
- DuRoss CB, Personius SF, Crone AJ, Olig SS, Hylland MD, Lund WR, Schwartz DP. 2016. Fault segmentation: New concepts from the Wasatch fault zone, Utah, USA. J Geophys Res Solid B Earth. 121(2):1131–1157. doi: 10.1002/2015JB012519
- Eaves S. 2015. The glacial history of Tongariro and Ruapehu volcanoes, New Zealand. A thesis presented in partial fulfilment of the requirements for the degree of Doctor of Philosophy in Geology at Victoria University of Wellington, New Zealand.
- Gómez Vasconcelos MG. 2017. Paleoseismology, seismic hazard and volcano-tectonic interactions in the Tongariro Volcanic Centre, New Zealand. A thesis presented in partial fulfilment of the requirements for the degree of Doctor of Philosophy in Earth Science at Massey University. Palmerston North: Massey University.
- Gómez-Vasconcelos MG, Villamor P, Cronin S, Procter J, Kereszturi G, Palmer A, Townsend D, Leonard G, Berryman K, Ashraf S. 2016. Earthquake history at the eastern boundary of the South Taupo Volcanic Zone, New Zealand. N Z J Geol Geophys. 59(4):522–543. doi: 10.1080/00288306.2016.1195757
- Gómez-Vasconcelos MG, Villamor P, Cronin S, Procter J, Palmer A, Townsend D, Leonard G. 2017. Crustal extension in the Tongariro graben, New Zealand: insights into volcano-tectonic interactions and active deformation in a young continental rift. GSA Bull. 129(9-10):1085–1099. doi: 10.1130/B31657.1
- Gregg D. 1964. Letter to the term: the term “Taupo Volcanic Zone”. N Z J Geol Geophys. 7(2):397–397. doi: 10.1080/00288306.1964.10420186
- Grindley GW, Harrington HJ, Wood BL. 1961. The geological map of New Zealand 1: 2,000,000. New Zealand Department of Scientific and Industrial Research, (66).
- Hanks TC, Kanamori H. 1979. A moment magnitude scale. J Geophys Res Solid Earth. 84(B5):2348–2350. doi: 10.1029/JB084iB05p02348
- Hayes G, Reyners M, Stuart G. 2004. The Waiouru, New Zealand, earthquake swarm: persistent mid crustal activity near an active volcano. Geophys Res Lett. 31(19):L19613. doi: 10.1029/2004GL020709
- Hegan BD, Johnson JD, Severne C. 2001. Landslide risk from the Hipaua geothermal area near Waihi village at the southern end of Lake Taupo. Engineering and Development in Hazardous Terrain: New Zealand Geotechnical Society 2001 Symposium, Christchurch, August 2001; Institution of Professional Engineers New Zealand.
- Hochstein M. 1995. Crustal heat transfer in the Taupo Volcanic Zone (New Zealand): comparison with other volcanic arcs and explanatory heat source models. J Volcanol Geotherm Res. 68(1):117–151. doi: 10.1016/0377-0273(95)00010-R
- Hurst A, McGinty P. 1999. Earthquake swarms to the west of Mt Ruapehu preceding its 1995 eruption. J Volcanol Geotherm Res. 90(1):19–28. doi: 10.1016/S0377-0273(99)00019-0
- Jolly A, Jousset P, Lyons J, Carniel R, Fournier N, Fry B, Miller C. 2014. Seismo-acoustic evidence for an avalanche driven phreatic eruption through a beheaded hydrothermal system: An example from the 2012 Tongariro eruption. J Volcanol Geotherm Res. 286:331–347. doi: 10.1016/j.jvolgeores.2014.04.007
- King G, Yielding G. 1984. The evolution of a thrust fault system: processes of rupture initiation, propagation and termination in the 1980 El Asnam (Algeria) earthquake. Geophys J Int. 77(3):915–933. doi: 10.1111/j.1365-246X.1984.tb02229.x
- Langridge R, Ries W, Litchfield N, Villamor P, Van Dissen R, Barrell D, Rattenbury M, Heron D, Haubrock S, Townsend D. 2016. The New Zealand active faults database. N Z J Geol Geophys. 59(1):86–96. doi: 10.1080/00288306.2015.1112818
- Lecointre JA, Neall VE, Palmer AS. 1998. Quaternary lahar stratigraphy of the western Ruapehu ring plain, New Zealand. N Z J Geol Geophys. 41(3):225–245. doi: 10.1080/00288306.1998.9514807
- Lecointre JA, Neall VE, Wallace RC, Elliot MB, Sparks R. 2004. Late Quaternary evolution of the Rotoaira Basin, northern Tongariro ring plain, New Zealand. N Z J Geol Geophys. 47(3):549–565. doi: 10.1080/00288306.2004.9515075
- Lecointre JA, Neall VE, Wallace CR, Prebble WM. 2002. The 55-to 60-ka Te Whaiau formation: a catastrophic, avalanche-induced, cohesive debris-flow deposit from Proto-Tongariro Volcano, New Zealand. Bull Volcanol. 63(8):509–525. doi: 10.1007/s004450100167
- Leonard G, Townsend D, Wilson C, Gravley D, Calvert A, Begg J, Downs D, Deering C, Rosenberg M. 2014. Forensic analysis of caldera volcanism: integrating geomorphology, geochemistry, physical volcanology and geochronology to map and refine the history of Taupo Volcanic Zone. International Course & Workshop on Collapse Calderas.
- Litchfield N, Van Dissen R, Sutherland R, Barnes P, Cox S, Norris R, Beavan R, Langridge R, Villamor P, Berryman K. 2013. A model of active faulting in New Zealand. N Z J Geol Geophys. 57(1):32–56. doi: 10.1080/00288306.2013.854256
- Miller V, Savage M. 2001. Changes in seismic anisotropy after volcanic eruptions: evidence from Mount Ruapehu. Science. 293(5538):2231–2233. doi: 10.1126/science.1063463
- Miller CA, Williams-Jones G. 2016. Internal structure and volcanic hazard potential of Mt Tongariro, New Zealand, from 3D gravity and magnetic models. J Volcanol Geotherm Res. 319:12–28. doi: 10.1016/j.jvolgeores.2016.03.012
- Moebis A, Cronin SJ, Neall VE, Smith IE. 2011. Unravelling a complex volcanic history from fine-grained, intricate Holocene ash sequences at the Tongariro Volcanic Centre, New Zealand. Quat Int. 246(1):352–363. doi: 10.1016/j.quaint.2011.05.035
- Morley C, Wonganan N. 2000. Normal fault displacement characteristics, with particular reference to synthetic transfer zones, Mae Moh mine, northern Thailand. Basin Res. 12(3-4):307–327. doi: 10.1046/j.1365-2117.2000.00127.x
- Mouslopoulou V, Nicol A, Walsh J, Beetham D, Stagpoole V. 2008. Quaternary temporal stability of a regional strike-slip and rift fault intersection. J Struct Geol. 30(4):451–463. doi: 10.1016/j.jsg.2007.12.005
- Nicol A, Walsh J, Berryman K, Villamor P. 2006. Interdependence of fault displacement rates and paleoearthquakes in an active rift. Geology. 34(10):865–868. doi: 10.1130/G22335.1
- Nicol A, Walsh J, Villamor P, Seebeck H, Berryman K. 2010. Normal fault interactions, paleoearthquakes and growth in an active rift. J Struct Geol. 32(8):1101–1113. doi: 10.1016/j.jsg.2010.06.018
- Paquet F, Dauteuil O, Hallot E, Moreau F. 2007. Tectonics and magma dynamics coupling in a dyke swarm of Iceland. J Struct Geol. 29(9):1477–1493. doi: 10.1016/j.jsg.2007.06.001
- Pardo N, Cronin SJ, Palmer AS, Németh K. 2012. Reconstructing the largest explosive eruptions of Mt. Ruapehu, New Zealand: lithostratigraphic tools to understand subplinian–plinian eruptions at andesitic volcanoes. Bull Volcanol. 74(3):617–640. doi: 10.1007/s00445-011-0555-z
- Procter J, Cronin S, Fuller I, Sheridan M, Neall V, Keys H. 2010. Lahar hazard assessment using Titan2D for an alluvial fan with rapidly changing geomorphology: Whangaehu River, Mt. Ruapehu. Geomorphology. 116(1):162–174. doi: 10.1016/j.geomorph.2009.10.016
- Reyners M. 2010. Stress and strain from earthquakes at the southern termination of the Taupo Volcanic Zone, New Zealand. J Volcanol Geotherm Res. 190(1):82–88. doi: 10.1016/j.jvolgeores.2009.02.016
- Rowland J, Sibson R. 2001. Extensional fault kinematics within the Taupo Volcanic Zone, New Zealand: soft-linked segmentation of a continental rift system. N Z J Geol Geophys. 44(2):271–283. doi: 10.1080/00288306.2001.9514938
- Rowland JV, Wilson CJ, Gravley DM. 2010. Spatial and temporal variations in magma-assisted rifting, Taupo Volcanic Zone, New Zealand. J Volcanol Geotherm Res. 190(1):89–108. doi: 10.1016/j.jvolgeores.2009.05.004
- Scott BJ, Potter SH. 2014. Aspects of historical eruptive activity and volcanic unrest at Mt. Tongariro, New Zealand: 1846–2013. J Volcanol Geotherm Res. 286:263–276. doi: 10.1016/j.jvolgeores.2014.04.003
- Seebeck H, Nicol A, Villamor P, Ristau J, Pettinga J. 2014. Structure and kinematics of the Taupo Rift, New Zealand. Tectonics. 33(6):1178–1199. doi: 10.1002/2014TC003569
- Stirling M, McVerry G, Gerstenberger M, Litchfield N, Van Dissen R, Berryman K, Barnes P, Wallace L, Villamor P, Langridge R. 2012. National seismic hazard model for New Zealand: 2010 update. Bull Seismol Soc Am. 102(4):1514–1542. doi: 10.1785/0120110170
- Topping W. 1973. Tephrostratigraphy and chronology of late Quaternary eruptives from the Tongariro Volcanic Centre, New Zealand. N Z J Geol Geophys. 16(3):397–423. doi: 10.1080/00288306.1973.10431368
- Tost M, Price R, Cronin S, Smith I. 2016. New insights into the evolution of the magmatic system of a composite andesite volcano revealed by clasts from distal mass-flow deposits: Ruapehu volcano, New Zealand. Bull Volcanol. 78(5):1–22. doi: 10.1007/s00445-016-1030-7
- Townsend D, Leonard G, Conway C, Eaves S, Wilson C, (compilers), cartographers. 2017. Geology of the Tongariro National Park area. Lower Hutt, N.Z.: GNS Science. GNS Science geological map 4. 1. sheet + 109 p.
- Van Dissen RJ, Berryman K, Webb T, Stirling M, Villamor P, Wood PR, Nathan S, Nicol A, Begg J, Barrell D, et al. 2003. An interim classification of New Zealand’s active faults for the mitigation of surface rupture hazards. Pacific Conference on Earthquake Engineering.
- Villamor P, Berryman K. 2001. A late Quaternary extension rate in the Taupo Volcanic Zone, New Zealand, derived from fault slip data. N Z J Geol Geophys. 44(2):243–269. doi: 10.1080/00288306.2001.9514937
- Villamor P, Berryman K. 2006. Late Quaternary geometry and kinematics of faults at the southern termination of the Taupo Volcanic Zone, New Zealand. N Z J Geol Geophys. 49(1):1–21. doi: 10.1080/00288306.2006.9515144
- Villamor P, Van Dissen R, Alloway BV, Palmer AS, Litchfield N. 2007. The Rangipo fault, Taupo rift, New Zealand: an example of temporal slip-rate and single-event displacement variability in a volcanic environment. Geol Soc Am Bull. 119(5-6):529–547. doi: 10.1130/B26000.1
- Wallace RE. 1970. Earthquake recurrence intervals on the San Andreas fault. Geol Soc Am Bull. 81(10):2875–2890. doi: 10.1130/0016-7606(1970)81[2875:ERIOTS]2.0.CO;2
- Wallace LM, Beavan J, McCaffrey R, Darby D. 2004. Subduction zone coupling and tectonic block rotations in the North Island, New Zealand. J Geophys Res B Solid Earth. 109:B12406. doi: 10.1029/2004JB003241
- Wilson C, Blake S, Charlier B, Sutton A. 2005. The 26· 5 ka Oruanui eruption, Taupo volcano, New Zealand: development, characteristics and evacuation of a large rhyolitic magma body. J Petrol. 47(1):35–69. doi: 10.1093/petrology/egi066
- Wright TJ, Ebinger C, Biggs J, Ayele A, Yirgu G, Keir D, Stork A. 2006. Magma-maintained rift segmentation at continental rupture in the 2005 Afar dyking episode. Nature. 442(7100):291–294. doi: 10.1038/nature04978