ABSTRACT
Paleogeographic and tectonic models for the southern Taranaki Basin are refined with sandstone and conglomerate provenances determined from the Late Cretaceous fluvial Rakopi, marginal marine North Cape and Paleocene fluvial Farewell formations. These syn-rift deposits were controlled by the Wakamarama Fault, which bounds the eastern side of the Pakawau Sub-basin graben. The conglomerates of the North Cape and Farewell formations are dominated by metasedimentary clasts with petrography and geochemistry consistent with the Takaka Terrane; minor Takaka Terrane volcanics; and minor Separation Point Suite granitoids and an unknown A-type granite. The sandstones have Takaka Terrane/Separation Point Suite provenance. This indicates that the deposits were sourced from the east with sediments fed into the basin from the Wakamarama Fault, in contrast to previous works indicating axial drainage and southern Buller Terrane provenance. The Farewell Formation has decreasing labile components up-section, indicating increased chemical weathering with the cessation of rifting.
Introduction
The Late Cretaceous Rakopi and North Cape formations (Pakawau Group) and Paleocene Farewell Formation (Kapuni Group) of the southern Taranaki Basin crop out in the northwest Nelson region of New Zealand (). These sedimentary units are marginal marine and terrestrial graben-fill, deposited within the Pakawau Sub-basin of the Taranaki Basin (King and Thrasher Citation1996). The extensional Pakawau Sub-basin developed in the Late Cretaceous (the ‘West Coast-Taranaki Rift’ of Strogen et al. (Citation2017)). The northeast-trending Pakawau Sub-basin is bound by the Wakamarama Fault in the east, and the present-day offshore Kahurangi Fault to the west (). Both of these faults had active normal offset in the Late Cretaceous, which slowed and stopped at the end of the Paleocene (Reilly et al. Citation2015). The faults were inverted in the Miocene, uplifting and folding the graben-fill ().
Figure 1. Geological map of the southern Taranaki Basin, showing key basement lithologies and structures. Coordinates are in degrees and decimal degrees, WGS84. Onshore geology modified from Rattenbury et al. (Citation1998), offshore geology modified from Tulloch and Mortimer (Citation2017), structure from King and Thrasher (Citation1996).
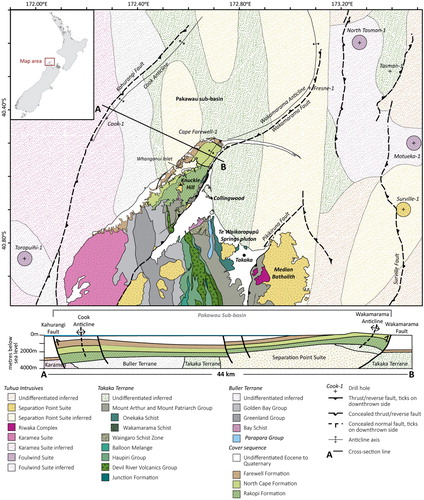
The purpose of this paper is to describe the composition of conglomerates and sandstones in the Late Cretaceous and Paleocene syn-rift deposits of the Pakawau Sub-basin and determine their provenance by petrographic and geochemical comparison to potential basement source material. Although previous studies have presented field descriptions of the conglomerates, this is the first study to use petrographic and geochemical techniques to identify the provenance of the clasts. The sandstone samples analysed here cover the stratigraphy and outcrop area in greater resolution than previous studies (Titheridge Citation1977; Wizevich Citation1992; Stark Citation1996; Browne et al. Citation2008; Higgs et al. Citation2010).
The provenance is useful for refining paleogeographic models for these units, and thereby understanding Zealandia’s changing tectonic regime as it shifted from rifting during the Late Cretaceous to a passive margin in the Paleocene. The provenance is also of interest for petroleum exploration, as these units are either producing intervals, or are potential source and reservoir units in the offshore Taranaki Basin (Killops et al. Citation1994; Higgs et al. Citation2010; Joyce Citation2018).
Late Cretaceous and Paleocene stratigraphy
The oldest syn-rift fill in the Pakawau Sub-basin is the Haumurian Rakopi Formation of the Pakawau Group (palynological PM2a-c zones, Raine et al. Citation2016). Outcrops of the Rakopi Formation are limited, the best outcrops occur along the Paturau River and in small cuttings along Pakawau Bush Road and the Kaituna Route track (). The Rakopi Formation comprises interbedded sandstone, carbonaceous mudstone, siltstone, conglomerate, and coal beds, interpreted as meandering stream, overbank, and swamp deposits (Thrasher Citation1992; Rattenbury et al. Citation1998; Browne et al. Citation2008). Locally at the base of the Rakopi Formation is the Otimataura Conglomerate Member, interbedded conglomerates, breccias, sandstones, minor siltstone and rare coal interpreted as alluvial fan deposits shed from the Wakamarama Fault scarp (Wizevich Citation1994).
The Late Haumurian North Cape Formation of the Pakawau Group (palynological PM2c-d zones, Raine et al. Citation2016) conformably overlies the Rakopi Formation. The North Cape Formation crops out primarily in coastal cliff and shore platform exposures around the Whanganui Inlet, including at Oyster Point, Maori Point, Moki Point, and Pecks Point (). The North Cape Formation is a succession of sandstones interbedded with siltstones, minor conglomerates, and rare coal seams (Titheridge Citation1977; Bussell Citation1985; Thrasher Citation1992; Rattenbury et al. Citation1998; Higgs et al. Citation2010). It is interpreted as paralic and marginal marine deposits, with coastal plain and fluvial environments, estuary deposits, and tidal channels on fans or fan deltas fed from the Wakamarama Fault scarp (Wizevich et al. Citation1992; Bal and Lewis Citation1994; Higgs et al. Citation2010; Joyce Citation2018). These environments are in the distal part of fan deltas, with north to northwest paleoflow directions from cross-bedding and plane-bed laminations suggesting the fans drained the uplifted footwall of the nearby Wakamarama Fault (Higgs et al. Citation2010; Joyce Citation2018; Smithies Citation2018). A fan delta is inferred by Thrasher (Citation1992) to have an apex near Puponga, resulting in conglomeratic facies in outcrop around the northern Whanganui Inlet.
The Teurian Farewell Formation (palynological PM3a Zone, Raine et al. Citation2016) of the Kapuni Group unconformably overlies the North Cape Formation in northwest Nelson (Bal Citation1994). It crops out in the Whanganui Inlet at Oyster Point and Moki Point, and to the north near Cape Farewell, such as Puponga Point, Pillar Point, Wharariki Beach, and Green Hills Beach (). The Farewell Formation contains sandstones, siltstones, pebbly sandstones, conglomerates, and minor coals (Titheridge Citation1977; King and Thrasher Citation1996; Stark Citation1996; Rattenbury et al. Citation1998). It is interpreted as a floodplain environment with both braided and meandering fluvial styles (Titheridge Citation1977; Stark Citation1996; Smithies Citation2018). The Farewell Formation is separated from overlying marine units by a significant regional unconformity spanning the majority of the Early and Middle Eocene (Waipawan into at least the Bortonian) (Rattenbury et al. Citation1998). The top 20 m of the Farewell Formation is pervasively iron-stained, increasing in intensity towards the top unconformity. This is dominated by sandstones, but also includes conglomerates in sections at Puponga Point. It is included in the Farewell Formation due to the gradual transition up the stratigraphy and inclusion of conglomeritic and sandstone lithofacies similar to the underlying Farewell Formation.
Basement geology
Basement rocks of northwest Nelson are the most likely source material for the Pakawau Group and Farewell Formation in the outcrop area. The basement includes the Buller and Takaka Terranes of the Western Province, and several intrusive suites of the Tuhua Intrusives ( and ) (Mortimer et al. Citation2014).
The Buller Terrane comprises Late Cambrian to Silurian sedimentary and metamorphic rocks, the greatest volume of which are the Greenland Group quartz-muscovite sandstone, siltstone, and mudstone units (Laird Citation1972; Cooper Citation1989; Roser et al. Citation1996; Rattenbury et al. Citation1998) (). In the northwest Nelson region the Greenland Group is conformably overlain by the Golden Bay Group, which includes quartzites, quartz sandstones, black siliceous shale, and minor chert (Harrison Citation1993; Roser et al. Citation1996; Rattenbury et al. Citation1998; Nebel-Jacobsen et al. Citation2011). In some regions the Golden Bay Group has been metamorphosed to the Bay Schist, a dark quartz-muscovite-chlorite and quartz-biotite pelitic schist (Stallard Citation1994; Rattenbury et al. Citation1998).
Figure 2. (A) chronostratigraphic diagram showing Late Cretaceous to Paleocene stratigraphy of the Pakawau Sub-basin, after King and Thrasher (Citation1996) and Higgs et al. (Citation2010). (B) summary of northwest Nelson basement ages, showing units discussed in text. Tuhua Intrusives shown as magmatic activity throughout Zealandia after Mortimer et al. (Citation2014), with units discussed in text labelled.
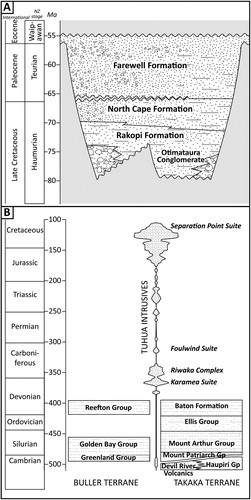
The Takaka Terrane is divided into fault slices containing Cambrian to Early Devonian siliciclastic, carbonate, and volcanic rocks (Rattenbury et al. Citation1998) (). The oldest units (Middle Cambrian to earliest Ordovician) are interpreted as a volcanic arc sequence (Cooper Citation1989; Rattenbury et al. Citation1998; Münker and Cooper Citation1999), including the feldspathic and lithic-rich Junction Formation, volcaniclastic Haupiri Group, and the dominantly basaltic to basaltic-andesitic Devil River Volcanics. The Haupiri Group and Devil River Volcanics are in places metamorphosed to the greenschist facies Waingaro Schist zone (Cooper and Tulloch Citation1992). The arc deposits are overlain by a passive margin succession, including the Late Cambrian to Early Ordovician volcaniclastic and calcareous Mount Patriarch Group, the Late Cambrian to Early Devonian calcareous Mount Arthur Group, and the Middle to Late Silurian highly quartzose Ellis Group and siliciclastic Baton Formation (Grindley Citation1980; Roser et al. Citation1996; Rattenbury et al. Citation1998). The Ellis Group is locally metamorphosed to the Onekaka Schist, which includes thick-bedded quartzite, metavolcanics, and siliceous quartz ± albite/oligoclase-biotite-muscovite schist (Bishop Citation1971; Rattenbury et al. Citation1998). The younger Takaka Terrane units have a relatively small outcrop area in northwest Nelson, but are inferred to extend offshore into Golden Bay in the footwall of the Wakamarama Fault () (Rattenbury et al. Citation1998; Tulloch and Mortimer Citation2017).
The Tuhua Intrusives in the northwest Nelson region include the Karamea and Separation Point suites. The Karamea Suite is a series of S-type, alkali granites intruded between 370 and 368 Ma into the Buller Terrane southwest of the Pakawau Sub-basin (Mortimer et al. Citation1997; Turnbull et al. Citation2013). The younger Separation Point Suite comprises I-type, subalkaline, gabbros to granites intruded into both the Buller and Takaka terranes between 125 and 105 Ma (Tulloch and Rabone Citation1993; Rattenbury et al. Citation1998; Mortimer et al. Citation1999). The Separation Point Suite occurs as part of the Median Batholith to the east of the Wakamarama Fault as well as in small plutons such as at Knuckle Hill outcropping within the Pakawau Sub-basin (). The Separation Point Suite is thought to underlie the Pakawau Group across much of the Whanganui Inlet area () (Tulloch and Mortimer Citation2017).
Previous provenance studies
The conglomerates have been previously described by visual estimation (Suggate Citation1956; Wizevich Citation1994) and clast counts (Titheridge Citation1977; Stark Citation1996), but the clast petrology and geochemistry has not been investigated. The Otimataura Conglomerate on the east side of the Wakamarama Ranges contains green micaceous schist and phyllite clasts with some green volcanic sandstone, vein quartz, and granite (Wizevich Citation1994). The conglomerate clasts of the North Cape and Farewell formations include metasedimentary clasts, schists, vein quartz, quartzite, granite, volcanics, gneiss, and syn-sedimentary mudstone and siltstone (Titheridge Citation1977; Wizevich Citation1994; Stark Citation1996). The granite and volcanic clasts become less abundant and more weathered towards the top of the Farewell Formation (Suggate Citation1956; Titheridge Citation1977). The metasedimentary and granite clasts are commonly assumed to have Buller Terrane and Karamea Suite provenance, indicating axial drainage from the southwest (Titheridge Citation1977; Stark Citation1996).
Previous studies of the onshore Pakawau Group and Farewell Formation sandstones used small sample sets (<30) (Titheridge Citation1977; Wizevich Citation1992; Stark Citation1996; Browne et al. Citation2008; Higgs et al. Citation2010). The Pakawau Group and Farewell Formation sandstones are generally classified as feldsarenites, lithic feldsarenites, and feldspathic litharenites, following the nomenclature of Folk et al. (Citation1970), with metamorphic, sedimentary, plutonic, and volcanic lithics (Wizevich Citation1992; Stark Citation1996; Browne et al. Citation2008; Higgs et al. Citation2010). Stark (Citation1996) and Browne et al. (Citation2008) report lithic content in the Rakopi and North Cape formations increasing from south to north. There is conflicting evidence for temporal changes: Wizevich (Citation1992) found that from the base of the Pakawau Group to the top, the feldspar content of the sandstones increases while lithics decreases. Other authors found no strong temporal trends (Browne et al. Citation2008). The dominant source is reported to be plutonic, either the Separation Point Suite or Karamea Suite, with input of metasedimentary lithics from the Takaka and/or Buller Terrane (Browne et al. Citation2008; Higgs et al. Citation2010). Wizevich (Citation1992) invoke a granitoid unroofing mechanism, to account for the observed increase in feldspar content through the Pakawau Group.
From detrital zircon analysis, Adams et al. (Citation2017) found that the Rakopi Formation in the Paturau River has dominantly Early Cretaceous Separation Point Suite/Median Batholith zircons, with minor Carboniferous Karamea Suite zircons, and recycled zircons from Buller and/or Takaka Terrane sedimentary rocks. There are also two very young 81 ± 2 and 84 ± 2 Ma zircons, possibly from rift-related magmatism.
Methodology
Clast count methods
Twenty-three conglomerate beds with medium pebble or larger clasts were selected for clast counts (). Clast counts were undertaken on exposed upper bedding surfaces of conglomerate units where possible: in the majority of locations however, clast counts were completed on <2 m thick cross-section exposures of a single bed. A ruler was placed against the outcrop surface and clast types noted every 5 cm for medium to very coarse pebble conglomerate sites, and every 10 cm for cobble conglomerate sites. A total of 100 or more clasts greater than 2 cm in diameter were counted at each location. Representative samples of each clast type were collected for later geochemical and petrographic analysis.
Table 1. Raw clast count data and conglomerate outcrop locations.
Clast petrography methods
Sixty-seven individual clast samples representing a range of clast types were prepared as thin sections (). The granitoids and metasedimentary clasts were stained for K-feldspar using Na-cobaltinitrate stain on half of the thin section after Lewis and McConchie (Citation1994). Each clast thin section was described, including whole rock and mineral textures, and the modal mineralogy was visually estimated. On a selection of the granitoid clasts, a point count of 100 grains at steps of 2 mm was conducted. The results of the point counts were compared to the estimated mineralogy of the same thin section to validate the modal estimation.
Table 2. sample: Sample details and location. Full geochemical and petrographic datasets are available online through the Petlab database (Strong et al. Citation2016), sample ID is provided.
Clast geochemistry methods
Seventy-eight representative conglomerate clast samples were selected for geochemical analysis (). The weathering rind and residual matrix was cut off with a rock saw if the clasts were large enough, smaller clasts were scrubbed to remove attached matrix and cement. The samples were crushed to a powder with a tungsten carbide ring mill.
Spectrachem Ltd. analysed the samples for major and trace elements by X-ray fluorescence spectrometry (XRF). Two granite basement samples from the Separation Point Suite at Knuckle Hill were also analysed (). Spectrachem Ltd. prepared the crushed samples by drying to 110°C, which was used as the reporting basis. Loss on ignition (LOI) was calculated by ignition at 1000°C. For major element analysis, a lithium metaborate/tetraborate fused glass bead was prepared. For trace element analysis, a pressed powder briquette was made using 10% analytical-grade wax binder. A SRS3000 Siemens/Bruker X-ray fluorescence spectrometer was used for both major and trace element analysis. The Spectrachem laboratory has ISO17025 Certification (CRL Energy Ltd Citation2017; International Organization for Standardization Citation2017). The geochemical data are available in supplementary data and online through the Petlab database () (Strong et al. Citation2016).
Geochemical and petrographic data for basement units were accessed through the Petlab database (Strong et al. Citation2016). Where there were sufficient data, they were filtered to include only results from published material. Data sources include unpublished data from N. I. Christensen, Dominion Labs Card Files, H. L. Fraser, N. Mortimer, D. T. Strong, A. J. Tulloch, I. M. Turnbull, and R. E. Turnbull; and data from Adams (Citation2004), Adams et al. (Citation2005), Allibone and Tulloch (Citation2004), Bradshaw (Citation1986), Brathwaite et al. (Citation2004), Burgess (Citation2004), Challis (Citation1971), Funk (Citation1996), Grapes and Palmer (Citation1996), Gutjahr (Citation2002), Ladley (Citation1999), Maclean (Citation1994), Mason (Citation1961), McCulloch et al. (Citation1987), Milton (Citation1983), Morgan (Citation1908), Mortimer et al. (Citation1997), Muir et al. (Citation1995), Muir et al. Citation1996, Muir et al. Citation1998), Münker (Citation1993, Citation1997, Citation2000), Nathan (Citation1976, Citation1999), Palmer (Citation1995), Pickett and Wasserburg (Citation1989), Price et al. (Citation2015), Rabone (Citation1977), Randall (Citation2004), Reed (Citation1958), Roser et al. (Citation1996), Ryland (Citation2011), Sagar (Citation2014), Sarll (Citation1996), Saunders (Citation1997), Scott (Citation2008), Scott and Palin (Citation2008), Smith (Citation1993), Stewart (Citation1988), Tulloch (Citation1979), Tulloch and Challis (Citation2000), Tulloch and Kimbrough (Citation2003), Tulloch et al. (Citation2009), and Waight et al. (Citation1998).
Sandstone petrography methods
One-hundred and seven sandstone samples were prepared as thin sections, medium sandstones were selected where possible (). Of these thin sections, 43 were stained with both Na-cobaltinitrate to identify alkali feldspar and K-rhodizonate to identify plagioclase following the method of Lewis and McConchie (Citation1994). The remaining 64 sandstones were stained with only Na-cobaltinitrate to limit the staining of K-bearing clay minerals.
Each sample was point counted using a PELCON Automatic Point Counter. Five-hundred point counts were made on the stained portion of the thin sections, following the method of Folk et al. (Citation1970). The spacing between each grid point was set to larger than the maximum grain size (van der Plas and Tobi Citation1965; Neilson and Brockman Citation1977). Where this was not practicable, for example in sandstones that had a few granules or pebbles, the grid spacing was set to be larger than the majority of the grains. The petrographic data are available in supplementary data and online through the Petlab database () (Strong et al. Citation2016).
Conglomerate outcrop description and clast count results
The Otimataura Conglomerate (Rakopi Formation) was observed in the Paturau River, southwest of the Whanganui Inlet (). Clast counts were performed at three sites, but clasts were not sampled. The conglomerate beds are up to 4 m thick. The lowest conglomerate bed is a subangular, matrix-supported very coarse pebble conglomerate. Further up the stratigraphy the conglomerates are subangular to subrounded coarse to very coarse pebble conglomerates. The conglomerates have a high percentage of metasedimentary clasts (c. 85%–100%), with minor volcanic clasts (<10%), vein quartz (<5%), and rare granitoids (<1%) ().
Conglomerates in the onshore North Cape Formation occur in outcrops in the northern Whanganui Inlet area within lithofacies associations interpreted as tidal delta-front deposits (lithofacies association A1 of Bal and Lewis Citation1994 and DF of Joyce Citation2018) and coastal plain and delta plain deposits (lithofacies association A3 of Bal and Lewis Citation1994 and DP of Joyce Citation2018).
The North Cape Formation conglomerate beds are 0.1–1 m thick and often occur at the base of trough cross-bedded sandstone beds. The bases of the beds are often erosive, forming scours or channels eroding into the underlying units. The conglomerates are typically clast supported, with moderately to well-sorted, sub-rounded to rounded, rod-dominated, medium to coarse pebble clasts. From six clast counts at three localities, the conglomerates are dominated by metasedimentary clasts (c. 50%–65%), with minor granitoid clasts (c. 10%–25%), vein quartz (c. 10%–20%), volcanics (c. 2%–10%), grey sedimentary clasts (<5%), and rare schist (1%–3%) and weathered granitoids (<2%) (). Granitoid clasts were described in the field as ‘unweathered’ (hard when struck with a hammer) or ‘weathered’ (soft, often easily broken with the hand). The composition of the North Cape Formation conglomerates exhibits no strong spatial or stratigraphic trends ( and ).
Figure 3. Clast count results organised by outcrop location, with locations discussed in text labelled. Geological map modified from Rattenbury et al. (Citation1998).
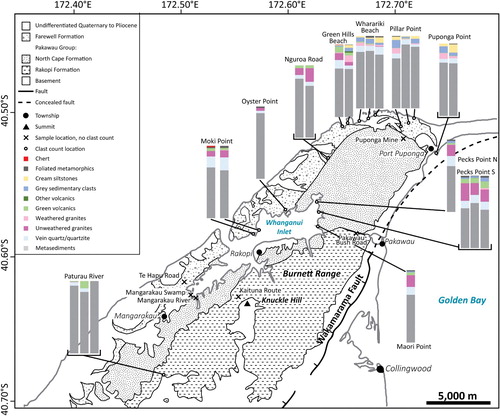
Figure 4. Clast count results organised into approximate stratigraphic position, with oldest conglomerates to the left.
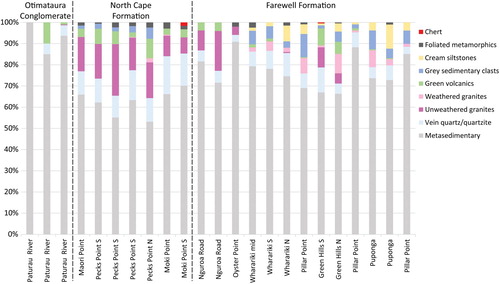
Conglomerates are abundant in the overlying Farewell Formation outcrops. They are more prevalent to the north around Cape Farewell and become more abundant up-section. The conglomerates occur in lithofacies associations interpreted as braided river deposits. A terrigenous setting is indicated by the abundance of coaly stringers (leaf mats or coalified branches) and absence of marine indicators such as marine fossils. A braided river system is suggested by the complex stacked channels, clast-supported sub-rounded conglomerates, and absence/rarity of overbank and floodplain deposits (Titheridge Citation1977; Smithies Citation2018).
The Farewell Formation conglomerate beds are typically 0.5–8 m thick, and are clast-supported, with clast size typically coarse pebble to cobble with rare boulders. Clasts are moderately to well-sorted and are sub-rounded rods. The beds may have bedding indicated by crude imbrication, or by lenses of sandstone. The bedding may show large-scale tabular cross-beds, with bedsets 1–4 m thick. From fourteen clast counts at seven localities, the conglomerates are dominated by metasedimentary clasts (c. 65%–90%) with minor fresh (<20%) and weathered (<10%) granitoid clasts, vein quartz (c. 5%–15%), volcanics (<10%), soft cream (<10%) and grey (<10%) fine sandstones to siltstones, and rare schist (<2%) and chert (<2%) (). The granitoids become more weathered towards the top of the formation and are almost entirely absent in some outcrops at the top of the stratigraphy (). Soft sedimentary clasts become more common towards the top of the Farewell Formation. The sedimentary clasts are either grey or cream in colour, moderately indurated, and typically siltstones. Cream-coloured sedimentary clasts are present only in the northern part of the field area ().
Metasedimentary conglomerate clasts
Metasedimentary clasts are the dominant clast type in all of the studied conglomerates. In hand sample, they are well-indurated very fine sandstone to siltstone, dominantly grey coloured with minor greenish-grey, red, and cream coloured clasts. Some clasts have quartz veins. Minor soft, cream-coloured siltstone clasts only occur within the Farewell Formation conglomerates in the northern field area ().
Petrography and geochemistry of metasedimentary clasts
The metasedimentary clasts of the North Cape and Farewell formations are highly quartzose, plotting as quartzarenites and subfeldsarenites () (Folk et al. Citation1970). They contain quartz with undulose extinction, minor alkali feldspar with microcline twinning, minor plagioclase, chert lithics, opaques, detrital muscovite, and rare high birefringence heavy minerals. The majority of the samples (c. 40%) are well-sorted very fine to fine sandstones with sutured quartz grain boundaries ((A)). Some (c. 30%) of the metasedimentary clasts are bimodal, with grain-supported very fine to fine sand in a silt matrix ((B)). Rare (c. 15%) sub-angular, very well-sorted fine sandstone with red-brown opaques concentrated along the grain boundaries also occur ((C and D)). The ‘dirty’ grain boundaries, retention of the original sedimentary textures, and absence of metamorphic minerals suggest a very low-grade metamorphism. Some strain is shown by the very strong undulose extinction of the quartz grains.
Figure 5. Quartz-feldspar-lithics (QFL) diagram after Folk et al. (Citation1970), showing composition of the metasedimentary clasts in the North Cape and Farewell formations compared to basement sources. Clasts are divided into their textural groups. Data source: Golden Bay Group Strong et al. (Citation2016), see methods for details; Greenland Group Laird (Citation1972); Baton Group Strong et al. (Citation2016); Ellis Group original work from thin sections UC16743-UC16746, Strong et al. (Citation2016); Mount Arthur Group Strong et al. (Citation2016); Haupiri Group Pound et al. (Citation1993).
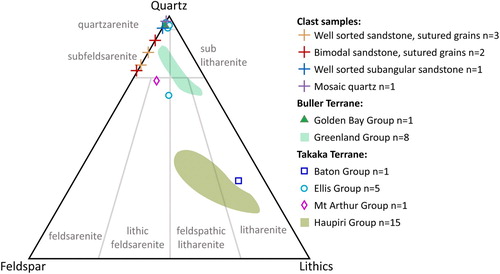
Figure 6. Selected photomicrographs showing metasedimentary clast mineralogy and textures in plane- (PPL) and cross-polarised light (CPL). Scale bar is 200 μm. (A) Wh285 (CPL), metasedimentary clast with sutured quartz (Q: quartz). (B) Pu226 (CPL), metasedimentary clast with sutured quartz (Q: quartz) in a silt matrix. (C/D) Wh277.1 (PPL/CPL), metasedimentary clast, subangular very fine sandstone with dirty grain boundaries. (E) Pu227.1 (CPL), cream coloured sedimentary clast with fine silt quartz and clay matrix. Vein in centre filled with quartz.
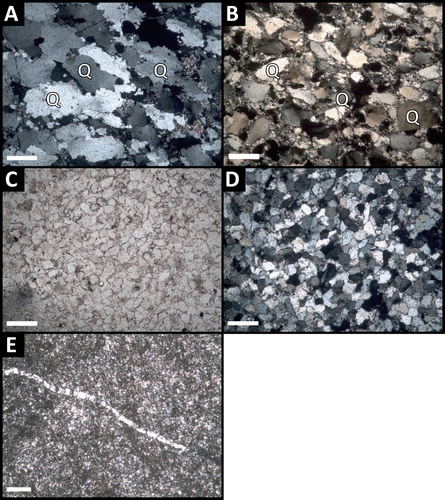
The metasedimentary clasts have high SiO2 (81–95 wt.%) and plot as having a ‘recycled’ provenance on the major element geochemical discrimination plot of Roser and Korsch (Citation1988) (). The lighter trace elements in all samples are slightly depleted compared to the average post-Archean shale (APAS) of Taylor and McLennan (Citation1985), particularly with respect to Ni, Zn, and Sr (). Sample Wh277.1 has lower Ba than the other metasedimentary clasts but is otherwise similar ().
Figure 7. (A) Plot of K2O/Na2O against SiO2, after Roser et al. (Citation1996), comparing the major element geochemistry of the Takaka and Buller Terrane sedimentary and metasedimentary units to metasedimentary clasts. Clasts are grouped by petrographic texture. (B) Major element provenance discrimination plot (Roser and Korsch Citation1988) of metasedimentary clast samples compared to basement sources. Mount Arthur and Mount Patriarch Group have anomalously high F1 and F2 due to calcareous biogenic components increasing CaO. F1 = −1.773 TiO2 + 0.607 Al2O3 + 0.76 Fe2O3T −1.5 MgO + 0.616 CaO + 0.509 Na2O −1.224 K2O − 9.09; F2 = 0.445 TiO2 + 0.07 Al2O3−0.25 Fe2O3T −1.142 MgO + 0.438 CaO + 1.475 Na2O + 1.426 K2O − 6.861.
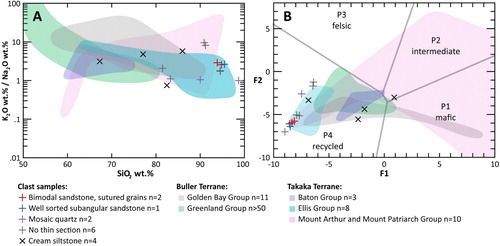
Figure 8. Spider diagrams for metasedimentary clasts compared to key basement sources. Normalised to average post-Archean shale (APAS) of Taylor and McLennan (Citation1985).
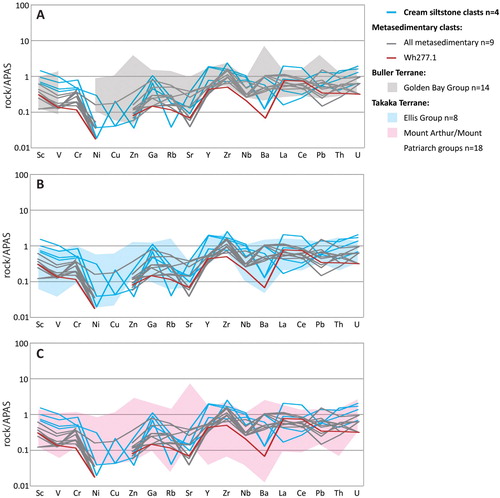
Thin sections reveal the cream coloured siltstone to be very fine silt sized quartz, brown opaques and undifferentiated clays ((E)). There are sparse, coarse silt sized, sub-rounded to sub-angular, quartz grains in the matrix. One sample is cut by very thin quartz veins ((E)), suggesting these are not intrabasinal clasts but instead have a longer burial and metamorphic history. The cream siltstone clasts have moderate SiO2 (67–86 wt.%). The light trace elements are depleted compared to the APAS, particularly with respect to Ni, Cu, and Zn ().
Provenance of metasedimentary clasts
In the northwest Nelson region, the metasedimentary basement can be characterised into five compositionally unique groups: 1. the quartz-muscovite Greenland Group (Buller Terrane); 2. the more quartzose Golden Bay Group (Buller Terrane); 3. the volcaniclastic Haupiri Group (Takaka Terrane); 4. the calcareous Mount Patriarch and Mount Arthur groups (Takaka Terrane); and 5. the highly quartzose Ellis Group and Baton Formation (Takaka Terrane).
The high quartz content of the metasedimentary clasts eliminates the volcaniclastic Haupiri Group of the Takaka Terrane as a source () (Grindley Citation1980; Pound et al. Citation1993; Roser et al. Citation1996; Jongens Citation1997). From available data the Devonian Baton Formation of the Takaka Terrane has a higher lithic content, making it an unlikely source () (N. Mortimer, unpublished from Petlab database, Strong et al. Citation2016). The metasedimentary clasts contain no carbonate minerals, making a Takaka Terrane Mount Arthur or Mount Patriarch Group source unlikely. The Buller Terrane Greenland Group is also unlikely as it has plagioclase feldspar not microcline, polycrystalline quartz, and a higher lithic content and therefore lower SiO2 concentration than the metasedimentary clasts ( and ) (Laird Citation1972).
The Buller Terrane Golden Bay Group has a similar mineralogy and texture to the metasedimentary clasts. The Aorangi Mine Formation of the Golden Bay Group is quartzose with chert lithics and dusty grain boundaries, very similar to the textures of the well sorted fine sandstone metasedimentary clast observed in this study ((C and D) (Burgess Citation1978; Harrison Citation1993). The Roaring Lion Formation and the Leslie Formation of the Golden Bay Group contain bimodal angular quartzarenites, similar in texture to the bimodal very fine sandstone metasedimentary clasts in this study ((B)) (Harrison Citation1993). Despite the mineralogical similarities, the geochemistry of the metasedimentary clasts is dissimilar to the Golden Bay Group. The clasts have lower F1 and F2 values on the discrimination plot of Roser and Korsch (Citation1988) ((B)), lower Ni concentrations than the Buller Terrane Golden Bay Group, and have detectable Cr concentrations ().
The remaining potential metasandstone source is the Ellis Group of the Takaka Terrane. The Hailes Quartzite of the Ellis Group is described by Grindley (Citation1980) as consisting of ‘subangular quartz, minor albite, detrital muscovite, biotite, microcline, epidote, titanite, and accessory tourmaline, zircon, and Fe oxide’ (p. 20). Thin sections of Ellis Group metasandstones from the University of Canterbury collection (C Anderson UC16744–UC16748) have strongly undulose quartz with sutured boundaries, minor plagioclase, microcline, and chert lithics similar to the metasedimentary clasts of this study. The clasts commonly have detrital muscovite and biotite, similar to the bimodal sandstone clasts. The overall texture and composition of these Ellis Group samples is similar to the well-sorted sandstone clasts with sutured quartz grains observed in this study. The geochemistry of the samples also fits well with the Ellis Group (). The clasts have high SiO2 concentrations, similar to those of the Ellis Group, low F1 and F2, low Ni, and low Sr ( and ). Sample Wh277.1 has lower Ba than the other samples, but still fits within the range of the Ellis Group ().
The cream siltstones clasts are too fine-grained to have a diagnostic petrography. However, a Takaka Terrane source is preferred for these samples, as they are more depleted in the light trace elements than the Buller Terrane Golden Bay Group, particularly Ni, Cu, Zn, and Rb ().
Granitoid conglomerate clasts
In hand sample the granitoid clasts exhibit a variety of textures. Light coloured, equigranular granitoids are most common. Also present are porphyritic granitoids with pink feldspar phenocrysts approximately 10 mm long.
Granitoid clast petrography
From their petrography, the granitoid clasts of the North Cape and Farewell formations can be divided into two textural groups: equigranular medium-grained granitoids (c. 60% of sampled clasts) ((A and B), and porphyritic, foliated granitoids ((C)).
Figure 9. Selected photomicrographs showing granitoid clast mineralogy and textures in cross-polarised light. Scale bar is 200 μm. (A) PP184.1, equigranular (syeno)-granite clast with perthitic alkali feldspar. (B) Wh273, weathered equigranular (monzo)-granite, with feldspar crystals partially replaced by kaolinite and indeterminate clays. Titanite crystal is replaced by opaques. Quartz crystals have undulose extinction and are unaltered. (C) MP038, foliated porphyritic (syeno)-granite clast with perthitic alkali feldspar. Medium-grained matrix with foliation (top-left to bottom-right) shown by biotite. Q: quartz; Kf: alkali feldspar; Pl: plagioclase; B: biotite; Ti: titanite replaced by opaques.
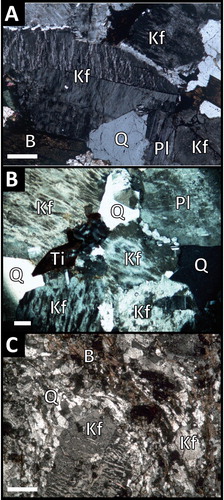
The equigranular granitoids are less quartz-rich than the other clasts, plotting mostly as granites in the classification scheme presented in . The alkali feldspars commonly have microcline twinning and perthitic texture ((A)). These granitoids also contain minor interstitial muscovite and biotite (<3%), and some contain trace titanite ((B)).
Figure 10. Quartz-alkali feldspar-plagioclase IUGS classification of granitoid clast petrography (Le Maitre Citation2004). Clasts are grouped based on the petrographic texture (indicated by colour, blue denotes equigranular, n = 16, red foliated, n = 1, and black foliated porphyritic, n = 10) and on the trace element geochemistry (symbol shape). Knuckle Hill data from this study, other basement data from Strong et al. (Citation2016).
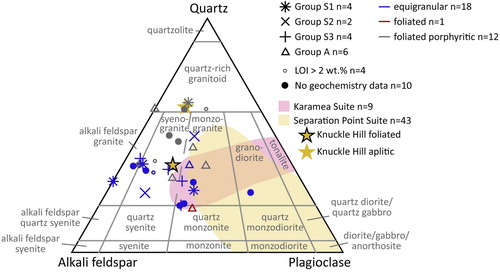
The porphyritic, foliated granitoids plot mostly as granites and minor quartz-rich granitoids (). They contain coarse-grained phenocrysts of alkali feldspar, which have perthitic texture and microcline twinning, set in a medium-grained foliated groundmass ((A)). The foliation is shown by quartz ribbons and strings of muscovite and biotite. The quartz has strong undulose extinction.
Both types of granitoid clast are weathered with feldspars altering to kaolinite and indeterminate clays, titanite to opaques, and biotite to indeterminate clays ((B)).
Granitoid clast geochemistry
Based on trace element geochemistry, granitoid clasts are divided into four geochemically distinct groups (). Geochemical data for those with loss on ignition (LOI) values greater than 2 wt.% are not included and not considered further, as these are likely to be affected by alteration (e.g. Windle and Craw Citation1991; Mercadier et al. Citation2010; Richard et al. Citation2011). As well as high LOI, alteration to clays was visible in thin sections of these samples and they could be easily broken in hand sample.
Figure 11. Chondrite normalised spider diagram of granitoid clasts with (A) trace element geochemistry similar to the Separation Point Suite, (B) trace element geochemistry dissimilar to either the Separation Point Suite or the Karamea Suite, and (C) likely A-type geochemistry compared to the Foulwind Suite including granitoids intersected by offshore wells. Samples with LOI >2 wt.% are excluded as these are likely to be affected by weathering. Chondrite data from Sun and McDonough (Citation1989).
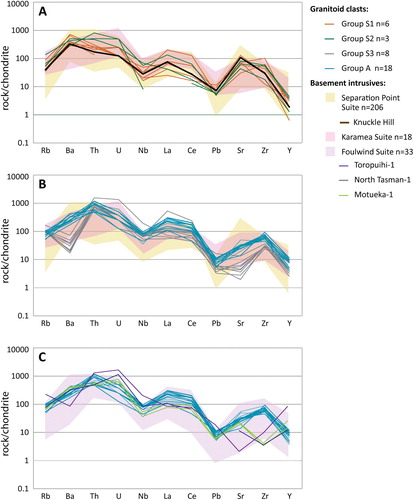
Group S1 granitoid clasts are characterised by high Ba and Sr, moderate Th, and low Nb and Y ((A)). Group S1 is relatively depleted in high field strength elements (HFSE) (Ce + Nb + Y + Zr in the range 95–415 ppm). The clasts have approximately equal proportions of Na2O (2.09–5.63 wt.%) and K2O (3.31–7.76 wt.%) and moderate CaO (0.72–1.30 wt.%) (), with an aluminium saturation index (ASI) of 1.04–1.11 (weakly peraluminous). Samples PP147.2 and PP147.3 are distinct from the rest of the clasts, with higher Na2O (5.38, 5.62 wt.%) relative to K2O (3.31, 3.37 wt.%) (). These two samples are equigranular aplitic granitoids in hand specimen. The thin-sectioned group S1 clasts are equigranular granites with accessory titanite and one foliated quartz-rich granitoid (). The presence of accessory titanite, their weakly peraluminous nature, elevated Sr, and low total HFSE, is most similar to I-type granites.
Figure 12. Ternary diagram of alkali major element geochemistry for the granitoid clast samples compared to potential basement sources.
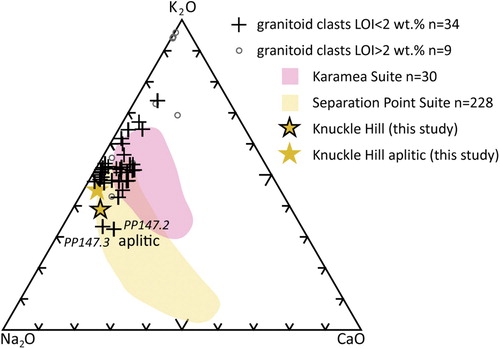
Group S2 granitoid clasts are characterised by high Ba, Sr, Th, U, and low Nb and Y ((A)). They are also depleted in HFSE (Ce + Nb + Y+ Zr between 81–284 ppm), and weakly peraluminous (ASI = 1.04–1.09). In thin section, group S2 clasts are equigranular granites (). The weakly peraluminous nature of the Group S2 clasts, elevated Sr, and low total HFSE is most similar to I-type granites.
Group S3 is characterised by very low Ba and Sr; high Th, La and Ce, and moderately high Nb ((B)). The HFSE concentrations are low, with Ce + Nb + Y + Zr between 170–284 ppm. The group S3 clasts have the highest SiO2 (75.08–77.56 wt.%) and lowest Al2O3 values (12.49–13.61 wt.%) with Fe2O3T (0.21–0.70 wt.%) of the clasts. As is expected from their high SiO2, S3 clasts are also the most plagioclase depleted, defining them as equigranular granites and an equigranular alkali feldspar granite (). Although the trace element trend of low Ba and Sr, and high Th and La is similar to A-type granites (Winter Citation2014), the total HFSE concentrations are lower than the typical 350 ppm minimum for A-type granites (Whalen et al. Citation1987). The trace element trends in group S3 may be a result of high degrees of fractional crystallisation of an I- or S-type magma. This interpretation is supported by the high SiO2, low plagioclase content, low Al2O3 and Fe2O3T, depletion in Ba, Sr, Zr, and the transition metals, and enrichment in Nb (Chappell and White Citation1992).
Group A clasts are characterised by high concentrations of Th, La, Ce, and Zr ((B)). Group A granitoids are less evolved, as indicated by typically lower SiO2 (62.38–82.77 wt.%) and higher Al2O3 (9.53–18.41 wt.%), Fe2O3T (0.25–4.36 wt.%), Sr, and Ba (). These clasts are defined as granites, a quartz-monzonite, and a quartz-rich granitoid (). They include both foliated and equigranular textures. The high Th, La, Ce, and Zr seen in group A are typical of A-type granites (Whalen et al. Citation1987; Winter Citation2014). The group A clasts have elevated HFSE, with total Ce + Nb + Y+Zr between 169 and 507 ppm, straddling the >350 ppm boundary used for defining A-type granites (Whalen et al. Citation1987). This suggests that the group A granitoids are weakly A-type.
Provenance of granitoid clasts
The Separation Point Suite is likely to be a major source for the granitoid clasts in both the North Cape and Farewell formations. Although the clasts do not match the modal mineralogy previously documented in either the Karamea Suite or the Separation Point Suite (), the Separation Point Suite is known to include a wider range of compositions (Hutton Citation1995). Accessory titanite, as seen in some clasts, is also present in the Separation Point Suite, but not the Karamea Suite (Tulloch Citation1983; Muir et al. Citation1995). The trace element geochemistry of clasts belonging to groups S1 and S2 best match the trace element geochemistry of the Separation Point Suite, which has peaks in Sr, Ba, and Th ((A)). Samples PP147.2 and PP147.3 from group S1 have lower K2O that clearly plot as Separation Point Suite on the Na2O, CaO, and K2O ternary diagram used by Tulloch (Citation1983) to distinguish Karamea Suite and Separation Point Suite granitoids (). These samples are aplitic and may be sourced from the aplitic quartz-rich granitoid veins such as those that cut the Knuckle Hill intrusion.
Group S3 is most likely to be a highly fractionated variant of the Separation Point Suite. The Separation Point Suite includes a wide range of compositions, including very silica-rich end-members. One pluton, just north of Te Waikoropupū Springs near Takaka (), has high SiO2 of 74.53–74.97 wt.% (Strong et al. Citation2016); is depleted in Ba and Sr; and enriched in Th, U, and Nb similar to the S3 group samples. S3 samples have relatively low aluminium saturation index (ASI) values of 1.02–1.08, which is consistent with the metaluminous to weakly peraluminous nature of the Separation Point Suite (Muir et al. Citation1995). One of the three thin sections from the S3 group clasts (Wh273) has large titanite crystals, a characteristic accessory mineral of the Separation Point Suite (Tulloch Citation1983; Muir et al. Citation1995).
The source of group A granitoid clasts is more enigmatic. The nearest documented outcrop of A-type granitoids to the study area is the A-type Foulwind Suite near Westport, 160 km to the SSW, which is likely to be too far away to be the source. However, A-type granites mapped as Foulwind Suite are present in offshore wells (Toropuihi-1 to the southwest, and North-Tasman-1 and Motueka-1 wells to the northeast and east) indicating a closer source is possible ( and ) (Cooper and Tulloch Citation1992; Mortimer et al. Citation1997; Tulloch and Mortimer Citation2017). The A-type granite source for the clasts may also be from a granite that was exposed in the Cretaceous to early Tertiary that has been completely unroofed and is no longer present. A-type granites are commonly associated with rifting (Winter Citation2014), it is possible that the granite was emplaced and eroded during the mid-Cretaceous Zealandia rift, similar to the Paparoa Metamorphic Core Complex on the West Coast (Tulloch and Kimbrough Citation1989; Laird and Bradshaw Citation2004; Sagar and Palin Citation2011).
Volcanic conglomerate clasts
Volcanic clasts are a minor constituent of the conglomerates in both the North Cape and Farewell formations. In hand sample these clasts are green in colour and may have white (feldspar), green or black (amphibole) tabular phenocrysts 0.5–5 mm long.
Petrography and geochemistry of volcanic clasts
All of the volcanic clasts collected are altered, although the primary porphyritic volcanic textures are preserved. From the estimated primary mineralogy, the majority of the clasts are andesites and basalts with minor rhyolite. The most abundant phenocryst is plagioclase, which ranges from almost unaltered with original polysynthetic twinning ((A)) to completely replaced by albite, zeolites, and sericite ((B)). Alkali feldspar is less common and is usually sericitised. Amphiboles are almost always replaced by chlorite and opaques and are distinguished by the alteration minerals taking up the original amphibole habit ((B)). Quartz is present in some samples, and usually shows resorption and embayments. The only sample to contain clinopyroxene was sample MP040.4, which contained clinopyroxene crystals with unaltered centres and rims replaced by chlorite ((B)).
Figure 13. Selected photomicrographs showing volcanic clast mineralogy and textures in cross-polarised light. Scale bar is 200 μm. (A) OP074, porphyritic volcanic clast with plagioclase and alkali feldspar phenocrysts in a fine-grained crystalline groundmass. (B) MP040.4, porphyritic volcanic clast with pseudomorphed amphibole phenocrysts replaced by chlorite, diagnosed by their characteristic six-sided habit. Darker, equant phenocrysts are interpreted as pseudomorphed pyroxene. (C) MP040.3, silicified tuff clast. Embayed quartz crystal (bottom middle). Matrix has silicified, cuspate glass shards. Q: quartz; Kf: alkali feldspar; Pl: plagioclase; A: (pseudomorphed) amphibole; Px: (pseudomorphed) pyroxene.
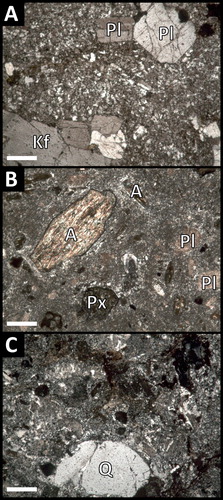
In the majority of the samples the groundmass is very fine-grained sericite, chlorite, albite, opaques, zoisite, and/or quartz likely replacing volcanic glass ((B)). Microlites are present in some samples, while some have a fine-grained groundmass of interlocking plagioclase, biotite, chlorite, and opaques ((A)).
Three volcanic samples are highly silicified. The porphyritic samples have a groundmass of fine-grained quartz, albite, sericite, and zoisite, with tabular shapes suggesting feldspar phenocrysts. Vesicles and fractures in these samples are filled with quartz. Sample MP040.3 is a highly altered tuff, with embayed quartz grains, silicified glass shards, and sericitised feldspars ((C)). The alteration is likely to be related to metasomatism or metamorphism of the volcanics, as the conglomerate matrix, sandstones, and other clasts do not exhibit this degree of alteration.
Due to the highly altered nature of the volcanic clasts, the major element geochemistry is unreliable. The trace element data show an island-arc signature rather than an OIB source, large iron lithophiles and high field strength elements do not increase smoothly with increasing incompatibility as would be observed in an OIB () (Winter Citation2014).
Figure 14. N-MORB normalised spider diagram for all available trace elements in the volcanic clasts compared to Takaka Terrane volcanics and to average OIB. Element order after Pearce et al. (Citation1984), N-MORB and OIB data from Sun and McDonough (Citation1989).
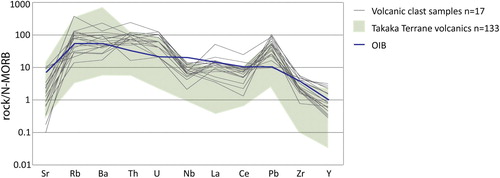
Provenance of volcanic clasts
There are two possible sources for the volcanic clasts: the Devil River Volcanics with associated volcaniclastic Haupiri Group conglomerates from the Cambrian Takaka Terrane volcanics; or syn-sedimentary volcanism. Late Cretaceous and Paleocene rift-related and intraplate volcanism is recorded across Zealandia, including the Greymouth Basin (Bishop Citation1992; Steadman Citation2017), Canterbury Basin (Wilding et al. Citation1971; Weaver and Pankhurst Citation1991), Great South Basin (Sahoo et al. Citation2014), and Marlborough (Grapes et al. Citation1992; McCoy-West et al. Citation2010). In the northern Taranaki Basin, Cretaceous volcanoes have been mapped in seismic data (Uruski Citation2008), and the Romney-1 well intersected volcanics intruding and interbedded with the Pakawau Group (Rad Citation2015). The rift-related and intraplate Cretaceous and Paleocene volcanics are generally basaltic with an ocean island basalt (OIB) geochemical signature (Baker et al. Citation1994; Jongens Citation1997; Nicholson and Black Citation2004), although the Cretaceous and Paleocene volcanics in the Taranaki Basin are poorly constrained. In contrast, the Takaka Terrane volcanics have arc-related geochemistry (Münker and Cooper Citation1999; Münker Citation2000). The trace element geochemistry of the volcanic clasts suggests that they formed in an arc-setting making the Takaka Terrane the likeliest source, although Taranaki Basin volcanics cannot be discounted based on the geochemistry alone.
The low-grade metamorphism of the clasts is similar to that seen in the Takaka Terrane volcanics. The Takaka Terrane Devil River Volcanics show extensive very low to low-grade metamorphism, with a mineral assemblage of albite + chlorite ± carbonate ± quartz ± epidote ± prehnite ± pumpellyite ± actinolite ± sericite ± (clino)zoisite ± stilpnomelane (Münker and Cooper Citation1999). Primary volcanic textures are usually preserved (Maclean Citation1994; Münker and Cooper Citation1999), with the volcanics generally andesitic to basaltic in composition and including tuffs and silicified volcanics creating cherts (Maclean Citation1994). The low-grade metamorphism of the volcanic clasts supports a Takaka Terrane source, in combination with the island arc geochemistry.
Other conglomerate clasts
Both the North Cape and Farewell formation conglomerates contain minor vein quartz clasts (3%–18%), typically less than 3 cm diameter. The metasedimentary clasts often have small quartz veins, probably similar to those from which the vein quartz clasts were eroded.
Rare (<2%), small (<3 cm) dark red chert clasts were observed in Farewell Formation conglomerates at Moki Point and Green Hills Beach. Chert is a component of both the Golden Bay Group and the Takaka Terrane, so it could be sourced from one or both of these units (Cooper Citation1979, Citation1989; Harrison Citation1993; Rattenbury et al. Citation1998). A Takaka Terrane source is the most likely as the majority of the other clasts are derived from the Takaka Terrane.
Minor schist clasts <5 cm in diameter were observed in all North Cape Formation conglomerates and half of the Farewell Formation locations. The schist clasts are small (<5 cm) and weathered, so were difficult to sample. The only thin section (OP073) is fine-grained and strongly foliated, with a mineral assemblage of quartz, biotite, opaques, minor alkali feldspar, and feldspars altered to clay (). Of the basement schists in the region, it is most similar to the Bay Schist (Buller Terrane) which contains quartz + albite + muscovite ± chlorite ± biotite ± graphite ± garnet ± calcite (Bishop Citation1971); or the Onekaka Schist (Takaka Terrane) which contains siliceous quartz + albite/oligoclase + biotite + muscovite + oxide + titanite ± calcite ± staurolite (Grindley Citation1980).
Figure 15. Photomicrograph of schist clast (OP073) with foliated quartz, biotite, clays. In cross-polarised light, scale bar is 200 μm.
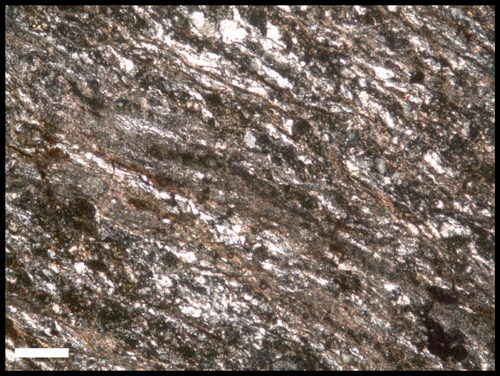
In both the North Cape and Farewell formations there are rare moderately indurated grey siltstones (<11%). No samples were collected, as the clasts were small (<5 cm) and eroded flat to the outcrop surface. The clasts were similar in grain size, colour, angularity, shape, and induration to siltstone rip-up clasts in the sandstone units. This, combined with the weaker induration compared to the metasedimentary basement, suggests that they are intrabasinal clasts.
Sandstone provenance
Sandstone composition
The Rakopi Formation sandstones (excluding the Otimataura Conglomerate) are feldsarenites and lithic feldsarenites following the nomenclature of Folk et al. (Citation1970) (). Metasedimentary and sedimentary lithics are dominant, with volcanic and plutonic lithics, and minor schist (). The sandstones from the Otimataura Conglomerate have high lithic contents, plotting as litharenites and a sub-litharenite (). The lithics are dominated by schist south of the Whanganui Inlet (sample PR200) ((A)). Further north, sandstones from the basal Rakopi Formation along the Pakawau Bush Road have entirely sedimentary and metasedimentary lithics ((B)), in contrast to the mid- and top-stratigraphy samples which have a typical mix of metasedimentary, schistose, volcanic, and plutonic lithics. Although there are no conglomerates in this outcrop area, the basal sandstones are grouped petrographically as a sandy equivalent to the Otimataura Conglomerate due to their different composition.
Figure 16. Quartz-feldspar-lithic (QFL) diagram after Folk et al. (Citation1970) showing overall composition and lithic composition of samples from the (A) Rakopi, (B) North Cape and (C) Farewell formations. Data from Browne et al. (Citation2008) and outcrop sample data from Higgs et al. (Citation2010) is shown as grey symbols. Metasedimentary lithics are grouped with sedimentary lithics on lithic ternary plot. Points are shaded according to the approximate stratigraphic position of the samples.
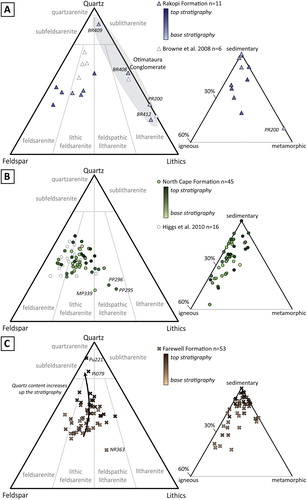
The majority of the North Cape Formation sandstones are feldsarenites and lithic feldsarenites, with minor feldspathic litharenites (). Metasedimentary and sedimentary lithics are dominant, with volcanic and plutonic lithics and minor schist. Plutonic lithics are more abundant than in either the Rakopi or Farewell formations.
The Farewell Formation is dominated by lithic feldsarenites with minor feldsarenites and feldspathic litharenites (). There is a strong stratigraphic trend, with the sandstones becoming more quartzose up the stratigraphy, with subfeldsarenites in the iron-stained unit at the top of the Farewell Formation (samples Pi079 and Pu221). The lithic composition at the base of the Farewell Formation is a mix of dominantly sedimentary and metasedimentary lithics, some volcanics, and minor schist (). Sedimentary and metasedimentary lithics become more abundant upward through the Farewell Formation, and volcanic and plutonic lithics become less common.
Description and provenance of framework grains
The quartz grains in all three formations display both undulose and straight extinction (). On the provenance discrimination plot of Basu et al. (Citation1975), this mixture of quartz types plots as ‘low rank metamorphic’ and ‘middle and upper rank metamorphic’ (). The metasedimentary and metamorphic sources in the Buller and Takaka terranes are mostly low-grade (chlorite and biotite zones). This distribution therefore likely represents a mixture of mostly low-grade metamorphic sources, with some plutonic sources contributing non-undulatory quartz.
Figure 17. (A) Sandstone tectonic setting discrimination diagram after Dickinson et al. (Citation1983). Note that this diagram is intended for medium sandstones, coarse and very coarse sandstones are typically enriched in lithics and fine and very fine sandstones are depleted. (B) Quartz provenance discrimination plot after Basu et al. (Citation1975). Data points are shaded according to approximate stratigraphic position.
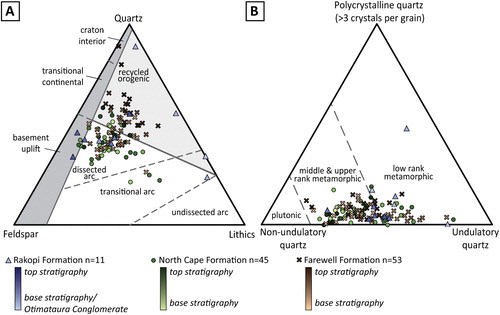
The feldspars in all samples from the Rakopi, North Cape and Farewell formations are dominated by alkali feldspars (c. 60%–90%) compared to plagioclase (c. 10%–40%). The alkali feldspars commonly exhibit microcline twinning and perthitic textures with rare myrmekite. Plagioclase feldspars commonly show strong polysynthetic twinning and occasional deformation twinning. They are commonly altered, more strongly than alkali feldspars in a given sample. The feldspars are most likely to be derived from the plutonic sources in the region, as the metasedimentary basement is highly quartzose and feldspars from the Takaka Terrane volcanics are more altered than those seen in the sandstones. Both the A-type granitoid clasts and clasts derived from the Separation Point Suite contain microcline, perthitic alkali feldspar, and plagioclase as seen in the sandstones and thus the feldspars in the sandstones could be derived from either.
Green-brown biotite is the most common mica type in all formations, followed by muscovite ((C)). Chlorite is rare in thin sections, and where present typically replaces biotite. The total mica content in the sandstone is variable, ranging from <1% of the total grains to 27%, decreasing slightly towards the top of the Farewell Formation. The variability within the formations is likely due to highly localised variations in mica concentration between beds. The schists and metasedimentary basement sources are all finer-grained than the micas observed in the sandstones (Harrison Citation1993; Rattenbury et al. Citation1998), suggesting that the micas are likely derived from a plutonic source. Clasts of the Separation Point Suite and the unknown A-type granitoid clasts in the conglomerates contain green-brown biotite, chlorite, and muscovite, suggesting that the micas in the sandstones are also from these sources.
Figure 18. Photomicrographs of sandstones showing key components. All photomicrographs in cross-polarised light. (A) PR200, schist lithics in Otimataura Conglomerate sandstone from the Paturau River. (B) BR408, Otimataura Conglomerate sandstone from the Pakawau Bush Road, with sedimentary and metasedimentary lithics in an organic rich silt matrix. (C) PP144, North Cape Formation sandstone with subrounded grains in porous matrix. Volcanic lithic (centre) has plagioclase laths. (D) Wh269, Farewell Formation sandstone with moderately sorted subangular texture, in centre of view is siltstone lithic with high birefringent clay minerals. (E) PP296, very fine sandstone lithic (outline) with quartz, feldspar, and lithic grains. (F) PP296, equigranular plutonic lithic (outlined) with microcline, plagioclase, quartz, and biotite. Qz: quartz; Poly: polycrystalline quartz; K: alkali feldspar; Plag: plagioclase feldspar; Bio: biotite; Musc: muscovite; Chl: chlorite; Opq: opaque; Org: organic matter; Sed: (meta)sedimentary lithic; Volc: volcanic lithic; Sch: schist lithic; Plut: plutonic lithic.
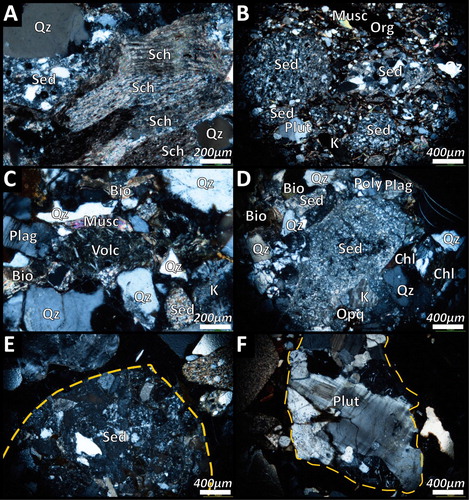
The metasedimentary lithics are highly quartzose very fine sandstones to siltstones with minor alkali feldspar and interstitial muscovite, and contain quartz with sutured boundaries, strained quartz, and quartz overgrowths ((A, B and D)). Some metasedimentary lithics are very fine siltstone to mudstone, which may be quartzose or dominated by illite and other clay minerals and may have small quartz veins. The metasedimentary lithics have a similar texture and composition to the metasedimentary conglomerate clasts. Without geochemistry, their source could be either the Golden Bay Group (Buller Terrane) or the Ellis Group (Takaka Terrane), however the provenance of the metasedimentary lithics is likely to be the same as the conglomerate clasts: the Takaka Terrane Ellis Group.
There are rare siltstone lithics, which have quartz, feldspar and sedimentary lithics without significant metamorphic textures ((E)). The composition and texture are similar to the Pakawau Group and Farewell Formation, they are therefore likely to be intrabasinal lithics derived from those units.
Volcanic lithics are common in the Rakopi and North Cape formations, becoming less abundant in the Farewell Formation. The volcanic lithics have devitrified and altered glass, which is green-brown in plane-polarized light, and may be black in cross-polarized light, or altered to clays. The volcanics have phenocrysts, usually plagioclase, which may also be altered and replaced ((C)). The volcanic lithics are likely to be from the Takaka Terrane Devil River Volcanics, due to their similar textures and composition. No evidence of syn-sedimentary volcanism, such as fresh glass shards, was observed.
Plutonic lithics with more than one interlocking crystal of quartz, feldspar, biotite, and/or muscovite were observed in the coarser-grained sandstones. Most are equigranular ((F)), while some show foliation, similar to the granitoid conglomerate clasts. They are likely to be derived from the same source as the granitoid clasts i.e. the Separation Point Suite and an A-type granitoid.
Foliated metamorphics are a moderately common lithic type. They are usually finely foliated, and often heavily altered to clays ((A)). They may have fine-grained muscovite, biotite, and quartz, which define the foliation. The texture and composition of these grains is similar to the schist clasts in the conglomerates, so they are also likely to be from either the Onekaka Schist (Takaka Terrane) or the Bay Schist (Buller Terrane).
Provenance of sandstones
The Rakopi, North Cape, and Farewell formation samples all plot in the ‘recycled orogen' and ‘dissected arc' fields in the tectonic setting discrimination plot from Dickinson et al. (Citation1983) (). The ‘dissected arc’ signature is anomalous, given that the Pakawau Group and Farewell Formation were deposited in a rift basin. However, the Takaka Terrane is a dissected arc sequence, with plutonic, volcanic, and sedimentary units. The tectonic discrimination plot therefore reflects provenance rather than tectonic setting.
The Takaka Terrane is the dominant source for all units apart from the Otimataura Conglomerate, as shown by the prevalence of metasedimentary lithics and minor Takaka Terrane volcanics. A secondary plutonic influence is indicated by the presence of plutonic lithics in the coarser sandstones, muscovite, biotite, and fresh feldspars. There are no strong stratigraphic trends through the upper Rakopi Formation and North Cape Formation, but the Farewell Formation gradually becomes more quartzose and metasedimentary lithics more abundant up-section (). The quartz type does not change through the stratigraphy, indicating that the variation is not due to a provenance change.
The sandstones from the Otimataura Conglomerate (Rakopi Formation) indicate a local provenance. The schist in sample PR200 is likely to be sourced from the Waingaro Schist of the Takaka Terrane, which crops out several kilometres to the east of the Paturau River outcrop (). The basement does not outcrop in the Pakawau Bush Road region (Rattenbury et al. Citation1998), although they do crop out a short distance to the south and probably the upper metasedimentary units of the Takaka Terrane provided the metasedimentary lithics in these samples ().
Discussion
The Takaka Terrane is the dominant source for the sediment of the Pakawau Group and Farewell Formation (). Conglomerates from both the North Cape and Farewell formations are dominated by Takaka Terrane metasedimentary clasts, an interpretation in contrast to previous work that interpreted the metasedimentary clasts as largely from the Buller Terrane. The upper (Ordovician) Takaka Terrane is differentiated from the Buller Terrane by its higher maturity and more felsic provenance (Roser et al. Citation1996). The metasedimentary clasts here are quartzose (SiO2 = 67.41–94.83 wt.%) and are depleted in the lighter trace elements similar to the upper Takaka Terrane. Volcanics derived from the Takaka Terrane are also present. There is a mix of plutonic sources identified from the petrography and geochemistry, including the Separation Point Suite, a high-silica end-member of the Separation Point Suite, and an unknown A-type granite. Granitoids from the Karamea Suite are absent. The Karamea Suite intrudes the Buller Terrane southwest of the field area () and is expected to be present if transport along the basin axis was dominant. Its absence supports a Takaka Terrane source.
Figure 19. Maps showing transport pathways of conglomerate clast types for (A) the North Cape Formation and (B) the Farewell Formation, flow directions interpreted from conglomerate clast provenance and outcrop of source material. See for map legend.
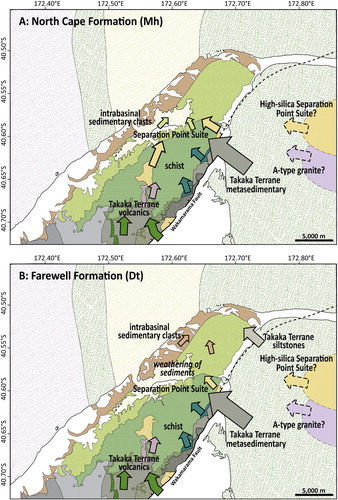
The Otimataura Conglomerate has monomictic conglomerates and lithic-rich sandstones, in strong contrast to the rest of the stratigraphy. This reflects a highly localised provenance, supporting the interpretation of alluvial fans with small catchments draining point sources along the Wakamarama Fault. Sandstones of the upper Rakopi Formation have a similar composition to the North Cape Formation and lower Farewell Formation suggesting they are from a similar source.
The provenance of the North Cape Formation supports the sedimentological interpretation of fan deltas sourced from material to the east uplifted by the Wakamarama Fault (Higgs et al. Citation2010). The Ellis Group of the Takaka Terrane is a dominant sediment source, and these units are likely to occur to the east in the present-day offshore Golden Bay area and are absent south of the field area along the basin axis (). Similarly, the Separation Suite which primarily outcrops east of the field area is a significant source. The Buller Terrane and Karamea Suite are absent, indicating axial drainage was minimal, or the basement units did not outcrop at the time of deposition. The presence of Takaka Terrane volcanic clasts suggests a minor component of axial drainage, as the modern outcrop of Takaka Terrane volcanics occurs to the south and southeast of the field area ( and (A)). Alternatively, the structurally complex Takaka Terrane may contain volcanics offshore, and thus volcanic clasts were supplied into the basin by fan deltas draining the Takaka Terrane in the uplifted fault block.
The Farewell Formation has previously been interpreted as having a Buller Terrane provenance, with a fluvial system draining from the southeast to southwest along the basin axis (Titheridge Citation1977; Stark Citation1996). However, it has been shown that drainage from the uplifted material southeast of the Wakamarama Fault was dominant ((B)), despite movement on the Wakamarama Fault inferred to have slowed in the Paleocene (Reilly et al. Citation2015).
The sediment composition in the upper two hundred metres of the Farewell Formation is affected by surface weathering. The granitoid clasts become more weathered towards the top of the Farewell Formation, and are almost absent at the top as they completely weathered to sand (). Similarly, volcanic clasts become less abundant towards the top of the Farewell Formation, likely because the labile volcanic glass, feldspars, amphiboles, and pyroxenes are readily weathered. Labile components in the sandstones also decrease in abundance up-section, with sandstones becoming more quartzose and rich in quartzose metasedimentary lithics, and plutonic, volcanic, and schist lithics becoming less abundant. We rule out modern weathering as a cause for the observed trends, as weathered grains are observed in fresh outcrops. The increased weathering is from the time of deposition, suggesting that the sediments had a longer residence time in the fluvial system and more exposure to weathering agents, or there was more in situ weathering of the source area, or some combination of both. This could be a result of changing climate. The lower Farewell Formation in northwest Nelson was deposited in cooler and slightly wetter conditions than the Pakawau Group (Kennedy Citation2003); a subtle change that does not account for the strong increase in weathered material in the Farewell Formation. There was abrupt, short-lived warming in the latest Paleocene at the Paleocene-Eocene thermal maximum (PETM) (Crouch and Brinkhuis Citation2005; Hollis et al. Citation2005; Handley et al. Citation2011; Pancost et al. Citation2013). This warming is unlikely to account for the increase in chemical weathering as warming was restricted to the end of the Paleocene and mainly occurred through the Eocene section absent in northwest Nelson (Pancost et al. Citation2013). The gradual increase in weathering through the upper third of the Paleocene Farewell Formation section is therefore unlikely to be due to dramatic climactic change. Alternatively, the increased maturity of the sediments was potentially a result of increased sediment residence time and basement weathering as movement on the Wakamarama Fault slowed decreasing the rate of erosion and transportation.
The stratigraphic trends observed in this study are apparent across the Taranaki Basin. The syn-rift Late Cretaceous sediments are typically the least mature, with the highest proportion of lithics, total feldspars, plagioclase, and mafic minerals (Higgs and King Citation2018). As in the Pakawau Sub-basin, the provenance of the Taranaki Basin syn-rift deposits is highly localised, indicating short transport distances and minimal chemical cleaning (Higgs and King Citation2018). This reflects localised deposition in rift sub-basins. Across the Taranaki Basin, Paleocene deposits become more quartzose, plagioclase-poor, and depleted in less stable heavy minerals (Higgs and King Citation2018). The same processes of tectonic slowing and increasing sediment transport distance and sediment recycling of older deposits occurred across the Taranaki Basin through the Paleocene.
From this study, we find no evidence of strike-slip faulting in the Pakawau Sub-basin or on the Wakamarama Fault. The provenance of the Pakawau Group and Farewell Formation is the uplifted Takaka Terrane basement material east of the Wakamarama Fault; it has not been offset as would be expected if there was lateral movement along the fault. Likewise, the outcrop pattern of the Takaka Terrane basement shows no appreciable lateral displacement across the Wakamarama Fault (). The results of this study agree with Reilly et al. (Citation2015), who found no evidence of strike-slip movement in seismic reflection studies in the offshore southern Taranaki Basin. This is in contrast to Thrasher (Citation1990) and King and Thrasher (Citation1996), who suggest strike-slip movement in the southern and central Taranaki Basin as a mechanism for Late Cretaceous extension. Similarly, transtension has been proposed as the mechanism for Late Cretaceous to Paleocene extension in the Greymouth Basin (Bishop Citation1992; Cody Citation2015), and Lamb et al. (Citation2016) suggested that there was significant sinistral offset on the Alpine Fault in the Late Cretaceous.
In this study there was no conclusive evidence of syn-sedimentary volcanism in the Late Cretaceous. This is in contrast to Adams et al. (Citation2017) who found Late Cretaceous (81 ± 2, 84 ± 2 Ma) detrital zircons in Rakopi Formation sandstones and suggested they were from rift-related magmatism. Many of the granitoid clasts in this study are from an A-type granitoid which does not presently crop out in the northwest Nelson region, potentially associated with rifting. During the first phase of Gondwana break-up in the mid-Cretaceous, the Paparoa Metamorphic Core Complex and associated rift-related intrusives developed in the Greymouth Basin, 160 km southwest of the Pakawau Sub-basin (Tulloch and Kimbrough Citation1989; Laird and Bradshaw Citation2004; Sagar and Palin Citation2011). Similarly, the Pakawau Sub-basin A-type granitoid may have been emplaced and unroofed during the extension of the continental crust in the early Late Cretaceous. The A-type granitoid may be Late Cretaceous in age, accounting for the young zircons, although this would require extreme rates of exhumation (<2 Ma). Alternatively, the A-type granitoid may be an unknown or completely removed outcrop of an older granitoid such as the Foulwind Suite (). Monzo-granite clasts with similar A-type chemistry are also found in conglomerates of the Late Cretaceous to Paleocene Paparoa Coal Measures in the Greymouth Basin (Steadman Citation2017). It is possible that this basin was also fed by a rift-related granitoid or by an A-type granite offshore, possibly of the same magmatic origin as the A-type clasts in the Pakawau Sub-basin.
Zircon ages from Rakopi Formation sandstones in the Pakawau Sub-basin indicate a mixed provenance consistent with the conglomerate composition. A sandstone from the middle of the Rakopi Formation stratigraphy in the Paturau River includes zircons aged 93 ± 1 Ma, 100 ± 1 Ma and 111 ± 1 Ma likely from the 125–105 Ma Separation Point Suite (Mortimer et al. Citation1999), and zircons 340 ± 4 Ma (Adams et al. Citation2017). Adams et al. (Citation2017) attribute the older 340 ± 4 Ma zircons to a Karamea Suite provenance, which is dated to 371–368, c. 349 Ma (Tulloch et al. Citation2009). Alternatively, the 340 ± 4 Ma zircons may be from the A-type 350–305 Ma Foulwind Suite (Tulloch et al. Citation2009), which has a better age correlation with the sandstone zircons and is a possible source for the A-type granitoid conglomerate clasts. We therefore prefer a Foulwind Suite-type source for the A-type granitoid clasts, given the age correlation of zircons in the sandstones and the similarity of the granitoid’s geochemistry to the Foulwind Suite.
Conclusions
This work concentrated on the provenance of conglomeritic and sandstone lithologies of the Rakopi, North Cape, and Farewell formations where they crop out in northwest Nelson. We generally agree with previous work but add considerable detail with regard to the provenance of these units.
The North Cape and Farewell formation conglomerates contain mixed metasedimentary, granitic, volcanic, and schist sources. A Takaka Terrane provenance is preferred for the metasedimentary clasts based on their geochemistry, in contrast to previous workers who inferred a Buller Terrane source. The granitic clasts are consistent with a Separation Point Suite origin, without clasts derived from the Karamea Suite. Previous work was not able to separate these plutonic source areas. An A-type granitoid source was also identified, likely associated with Foulwind Suite basement in the offshore southern Taranaki Basin. Volcanic clasts show petrographic and geochemical affinity to the Devil River Volcanics, but they might also potentially represent Cretaceous-Paleocene volcanics which are poorly represented in the modern outcrop or in wells, and consequently are poorly known in terms of composition. We believe the majority of the conglomerate clasts were derived from drainage feeding laterally into the Pakawau Basin from eastern and south-eastern sources. Previous workers had in contrast, argued for significant axial drainage bringing conglomerate clasts from the southwest.
Conglomerate clasts have a greater abundance of weathered lithologies toward the upper stratigraphic portions of the Farewell Formation. There is also an increase in sandstone maturity with greater quartz abundance, this has not previously been recognised from the formation. This may relate to derivation of weathered clasts eroded into the formation, or in situ weathering of clasts. It most likely relates to movement on the Wakamarama Fault slowing, causing the rate of sediment erosion to decrease and sediment residence time to increase.
Sandstones from the Otimataura Conglomerate are litharenites and sublitharenites, with lithic compositions reflecting the local basement. The North Cape Formation and Farewell Formation sandstones are feldsarenites and lithic feldsarenites with metasedimentary, volcanic, schist, and plutonic lithics. The composition does not vary greatly through the stratigraphy, with the exception of the top of the Farewell Formation which is more quartzose and depleted in labile components such as feldspar, plutonic lithics, and volcanic lithics. This trend is consistent with that seen across the Taranaki Basin through the Paleocene deposits.
The provenance results here support the environmental interpretation for the North Cape Formation of fan deltas draining the uplifted footwall of the Wakamarama Fault. Previous workers have suggested that the Farewell Formation was fed by axial drainage from the southwest along the Pakawau Sub-basin. However, our provenance data indicate that sediment was derived from areas uplifted by the Wakamarama Fault from the east and southeast through the Paleocene.
Supplementary data
Download MS Excel (72.4 KB)Acknowledgements
Field work by SLS was funded by the Mason Trust Fund and by an Institute of Geological and Nuclear Sciences field work grant. The research by SLS was partially funded by an Orion Master’s Energy Scholarship. Funding to GHB was received from MBIE through a Strategic Science and Investment Fund (SSIF) grant to GNS Science and the Sedimentary Basin Research Programme. We thank the Wylie, Kerr, Thompson, Ferguson, and Riley families for land access and sampling permission, and the Department of Conservation Takaka Office for sampling permission within the Kahurangi National Park, farm parks and marine reserves of the area. Technical support was provided by Rob Spiers, Chris Grimshaw, Stephen Brown, and Craig Fraser. Rebecca Joyce and Sophie Hill are thanked for their assistance in the field, and Victoria University of Wellington for the use of the Onekaka field station. The geochemistry interpretations were much improved by consultation with Andy Tulloch and Matt Sagar of GNS Science, Karen Higgs of GNS Science is thanked for her helpful feedback on the unpublished thesis, and Stuart Munday and Chemostrat Ltd. for their support of this project. We thank Dominic Strogen and an anonymous reviewer for their thoughtful feedback on the manuscript.
Disclosure statement
No potential conflict of interest was reported by the authors.
Data availability statement
The data that support the findings of this study are openly available in the Petlab database at http://pet.gns.cri.nz/, collection numbers UC21170–UC21400
ORCID
Sarah L. Smithies http://orcid.org/0000-0002-7734-2952
Greg H. Browne http://orcid.org/0000-0003-0865-6146
Alexander R. L. Nichols http://orcid.org/0000-0002-8298-2882
References
- Adams CJ. 2004. Rb-Sr age and strontium isotope characteristics of the Greenland Group, Buller Terrane, New Zealand, and correlations at the East Gondwanaland margin. New Zealand Journal of Geology and Geophysics. 47:189–200. doi: 10.1080/00288306.2004.9515047
- Adams CJ, Campbell HJ, Mortimer N, Griffin WL. 2017. Perspectives on Cretaceous Gondwana break-up from detrital zircon provenance of southern Zealandia sandstones. Geological Magazine. 154:661–682. doi: 10.1017/S0016756816000285
- Adams CJ, Pankhurst RJ, Maas R, Millar IL. 2005. Nd and Sr isotopic signatures of metasedimentary rocks around the South Pacific margin and implications for their provenance. Geological Society Special Publication. 246:113–141. doi: 10.1144/GSL.SP.2005.246.01.04
- Allibone AH, Tulloch AJ. 2004. Geology of the plutonic basement rocks of Stewart Island, New Zealand. New Zealand Journal of Geology and Geophysics. 47:233–256. doi: 10.1080/00288306.2004.9515051
- Baker JA, Gamble JA, Graham IJ. 1994. The age, geology, and geochemistry of the Tapuaenuku Igneous Complex, Marlborough, New Zealand. New Zealand Journal of Geology and Geophysics. 37:249–268. doi: 10.1080/00288306.1994.9514620
- Bal AA. 1994. Cessation of Tasman Sea spreading recorded as a sequence boundary. In: van der Lingen GJ, Swanson KM, Muir RJ, editor. Evolution of the Tasman Sea Basin. Rotterdam, Netherlands: A. A. Balkema; p. 105–117.
- Bal AA, Lewis DW. 1994. A Cretaceous - early Tertiary macrotidal estuarine-fluvial succession: Puponga coal Measures in Whanganui Inlet, onshore Pakawau Sub-basin, northwest Nelson, New Zealand. New Zealand Journal of Geology and Geophysics. 37:287–307. doi: 10.1080/00288306.1994.9514622
- Basu A, Young SW, Suttner LJ, James WC, Mack GH. 1975. Re-evaluation of the use of undulatory extinction and polycrystallinity in detrital quartz for provenance interpretation. Journal of Sedimentary Research. 45:873–882.
- Bishop DG. 1971. Geological map of New Zealand sheet S1, S3 & pt.S4: Farewell-Collingwood, scale 1:63,360. Wellington: Department of Scientific and Industrial Research.
- Bishop DJ. 1992. Extensional tectonism and magmatism during the middle Cretaceous to Paleocene, North Westland, New Zealand. New Zealand Journal of Geology and Geophysics. 35:81–91. doi: 10.1080/00288306.1992.9514502
- Bradshaw JY. 1986. Basement geology, northern Franklin Mountains, Northern Fiordland, with emphasis on the origin and evolution of Fiordland granulites [Unpublished PhD thesis]. Dunedin, New Zealand: University of Otago.
- Brathwaite RL, Kamo SL, Faure K. 2004. U-Pb geochronology and geochemistry of molybdenum-bearing granodiorite porphyry at Copperstain Creek, west Nelson, New Zealand. New Zealand Journal of Geology and Geophysics. 47:219–225. doi: 10.1080/00288306.2004.9515049
- Browne GH, Kennedy EM, Constable RM, Raine JI, Crouch EM, Sykes R. 2008. An outcrop-based study of the economically significant Late Cretaceous Rakopi Formation, northwest Nelson, Taranaki Basin, New Zealand. New Zealand Journal of Geology and Geophysics. 51:295–315. doi: 10.1080/00288300809509867
- Burgess AJ. 2004. Emplacement mechanism and geochemical zoning of the Separation Point Batholith, northwest Nelson, New Zealand [Unpublished MSc thesis]. Christchurch, New Zealand: University of Canterbury; 173 p.
- Burgess AW. 1978. Gold mineralization at the Golden Blocks gold field [Unpublished MSc thesis]. Christchurch, New Zealand: University of Canterbury; 150 p.
- Bussell MR. 1985. Report following geological mapping of the Pakawau Group in Westhaven Inlet, northwest Nelson. Ministry of Economic Development New Zealand Unpublished Coal Report CR1571.
- Challis GA. 1971. Chemical analyses of New Zealand rocks and minerals: with C.I.P.W. norms and petrographic descriptions, 1917–57. New Zealand Geological Survey Bulletin. 84: 178 p.
- Chappell BW, White AJR. 1992. I- and S-type granites in the Lachlan Fold Belt. Transactions of the Royal Society of Edinburgh: Earth Sciences. 83:1–26. doi: 10.1017/S0263593300007720
- Cody E-NO. 2015. Sedimentology and hydrocarbon potential of the Paparoa Coal Measures lacustrine mudstones [Unpublished MSc thesis]. Christchurch, New Zealand: University of Canterbury; 217 p.
- Cooper RA. 1979. Lower Palaeozoic rocks of New Zealand. Journal of the Royal Society of New Zealand. 9:29–84. doi: 10.1080/03036758.1979.10418157
- Cooper RA. 1989. Early Paleozoic terranes of New Zealand. Journal of the Royal Society of New Zealand. 19:73–112. doi: 10.1080/03036758.1989.10426457
- Cooper RA, Tulloch AJ. 1992. Early Palaeozoic terranes in New Zealand and their relationship to the Lachlan Fold Belt. Tectonophysics. 214:129–144. doi: 10.1016/0040-1951(92)90193-A
- CRL Energy Ltd. 2017. SpectraChem quality assurance. [accessed 2018 Apr 10]. https://www.crl.co.nz/spectrachem-quality-assurance.
- Crouch EM, Brinkhuis H. 2005. Environmental change across the Paleocene–Eocene transition from eastern New Zealand: A marine palynological approach. Marine Micropaleontology. 56:138–160. doi: 10.1016/j.marmicro.2005.05.002
- Dickinson WR, Beard LS, Brakenridge GR, Erjavec JL, Ferguson RC, Inman KF, Knepp RA, Lindberg FA, Ryberg PT. 1983. Provenance of North American Phanerozoic sandstones in relation to tectonic setting. Geological Society of America Bulletin. 94:222–235. doi: 10.1130/0016-7606(1983)94<222:PONAPS>2.0.CO;2
- Folk RL, Andrews PB, Lewis DW. 1970. Detrital sedimentary rock classification and nomenclature for use in New Zealand. New Zealand Journal of Geology and Geophysics. 13:937–968. doi: 10.1080/00288306.1970.10418211
- Funk T. 1996. Geologische und geochemische Untersuchungen an kambrischen Vulkaniten Neuseelands [Unpublished thesis]. Gottingen, Germany: Georg August Universitat; 134 p.
- Grapes RH, Lamb SH, Adams CJ. 1992. K-Ar ages of basanitic dikes, Awatere Valley, Marlborough, New Zealand. New Zealand Journal of Geology and Geophysics. 35:415–419. doi: 10.1080/00288306.1992.9514536
- Grapes RH, Palmer K. 1996. (Ruby—Sapphire)—Chromian Mica—Tourmaline Rocks from Westland, New Zealand. Journal of Petrology. 37:293–315. doi: 10.1093/petrology/37.2.293
- Grindley GW. 1980. Geological map of New Zealand 1:63 360, sheet S23 Cobb, New Zealand Geological Survey. Department of Scientific and Industrial Research. 46 p.
- Gutjahr M. 2002. Provenance of igneous and sandstone clasts in the Cambrian Lockett and Dead Goat conglomerates, northwest Nelson [Unpublished MSc thesis]. Christchurch, New Zealand: University of Canterbury; 218 p.
- Handley L, Crouch EM, Pancost RD. 2011. A New Zealand record of sea level rise and environmental change during the Paleocene–Eocene Thermal Maximum. Palaeogeography, Palaeoclimatology, Palaeoecology. 305:185–200. doi: 10.1016/j.palaeo.2011.03.001
- Harrison IS. 1993. The structure of the Buller Terrane west of the Anatoki Thrust, upper Cobb Valley, northwest Nelson, New Zealand [Unpublished MSc thesis]. Christchurch, New Zealand: University of Canterbury; 97 p.
- Higgs KE, Arnot MJ, Browne GH, Kennedy EM. 2010. Reservoir potential of Late Cretaceous terrestrial to shallow marine sandstones, Taranaki Basin, New Zealand. Marine and Petroleum Geology. 27:1849–1871. doi: 10.1016/j.marpetgeo.2010.08.002
- Higgs KE, King PR. 2018. Sandstone provenance and sediment dispersal in a complex tectonic setting: Taranaki Basin, New Zealand. Sedimentary Geology. 372:112–139. doi: 10.1016/j.sedgeo.2018.05.004
- Hollis CJ, Dickens GR, Field BD, Jones CM, Percy Strong C. 2005. The Paleocene–Eocene transition at Mead Stream, New Zealand: a southern Pacific record of early Cenozoic global change. Palaeogeography, Palaeoclimatology, Palaeoecology. 215:313–343. doi: 10.1016/j.palaeo.2004.09.011
- Hutton PR. 1995. A structural study of the Separation Point Batholith: emplacement mechanisms and the tectonic regime [Unpublished MSc thesis]. Christchurch, New Zealand: University of Canterbury; 96 p.
- International Organization for Standardization. 2017. ISO/IEC 17025:2017(en) General requirements for the competence of testing and calibration laboratories. [accessed 2018 Apr 20]. https://www.iso.org/obp/ui/#iso:std:iso-iec:17025:ed-3:v1:en.
- Jongens R. 1997. The Anatoki Fault and structure of the adjacent Buller and Takaka Terrane rocks, northwest Nelson, New Zealand [Unpublished PhD thesis]. Christchurch, New Zealand: University of Canterbury; 368 p.
- Joyce R. 2018. Assessment of paleo-depositional environments and reservoir potential of the Late Cretaceous North Cape Formation, Nelson, New Zealand [Unpublished MSc thesis]. Christchurch, New Zealand: University of Canterbury; 110 p.
- Kennedy EM. 2003. Late Cretaceous and Paleocene terrestrial climates of New Zealand: leaf fossil evidence from South Island assemblages. New Zealand Journal of Geology and Geophysics. 46:295–306. doi: 10.1080/00288306.2003.9515010
- Killops SD, Woolhouse AD, Weston RJ, Cook RA. 1994. A geochemical appraisal of oil generation in the Taranaki Basin, New Zealand. AAPG Bulletin. 78:1560–1585.
- King PR, Thrasher GP. 1996. Cretaceous-Cenozoic geology and petroleum systems of the Taranaki Basin, New Zealand. Lower Hutt, New Zealand: Institute of Geological & Nuclear Sciences Limited. 243 p.
- Ladley EB. 1999. The Pre-Cenozoic geology of Lake Hauroko, Southeast Fiordland, New Zealand [Unpublished MSc thesis]. Dunedin, New Zealand: University of Otago.
- Laird MG. 1972. Sedimentology of the Greenland Group in the Paparoa Range, West Coast, South Island. New Zealand Journal of Geology and Geophysics. 15:372–393. doi: 10.1080/00288306.1972.10422338
- Laird MG, Bradshaw JD. 2004. The break-up of a long-term relationship: the Cretaceous separation of New Zealand from Gondwana. Gondwana Research. 7:273–286. doi: 10.1016/S1342-937X(05)70325-7
- Lamb S, Mortimer N, Smith E, Turner G. 2016. Focusing of relative plate motion at a continental transform fault: Cenozoic dextral displacement >700 km on New Zealand’s Alpine Fault, reversing >225 km of Late Cretaceous sinistral motion. Geochemistry, Geophysics, Geosystems. 17:1197–1213. doi: 10.1002/2015GC006225
- Le Maitre RW. 2004. Igneous Rocks: a classification and glossary of terms: recommendations of the International Union of Geological Sciences, Subcommission on the Systematics of Igneous rocks, 2nd ed. Cambridge: Cambridge University Press.
- Lewis DW, McConchie D. 1994. Analytical sedimentology. New York: Chapman & Hall.
- Maclean DR. 1994. The geology and geochemistry of the Cambrian Devil River volcanics, Anatoki Range, northwest Nelson [Unpublished MSc thesis]. Christchurch, New Zealand: University of Canterbury; 114 p.
- Mason B. 1961. Some analysed rocks from Westland, New Zealand. New Zealand Journal of Geology and Geophysics. 4:347–351. doi: 10.1080/00288306.1961.10420122
- Mercadier J, Richard A, Boiron M-C, Cathelineau M, Cuney M. 2010. Migration of brines in the basement rocks of the Athabasca Basin through microfracture networks (P-Patch U deposit, Canada). Lithos. 115:121–136. doi: 10.1016/j.lithos.2009.11.010
- McCoy-West AJ, Baker JA, Faure K, Wysoczanski R. 2010. Petrogenesis and origins of mid-Cretaceous continental intraplate volcanism in Marlborough, New Zealand: Implications for the long-lived HIMU magmatic mega-province of the SW Pacific. Journal of Petrology. 51:2003–2045. doi: 10.1093/petrology/egq046
- McCulloch MT, Bradshaw JY, Taylor SR. 1987. Sm-Nd and Rb-Sr isotopic and geochemical systematics in Phanerozoic granulites from Fiordland, southwest New Zealand. Contributions to Mineralogy and Petrology. 97:183–195. doi: 10.1007/BF00371238
- Milton D. 1983. Geology of the Riwaka Valley, with emphasis on the origin and nature of amphibolites [Unpublished BSc Hons thesis]. Wellington, New Zealand: Victoria University of Wellington.
- Morgan PG. 1908. The geology of the Mikonui subdivision, north Westland. New Zealand Geological Survey Bulletin. 6:175 p.
- Mortimer N, Rattenbury MS, King PR, Bland KJ, Barrell DJA, Bache F, Begg JG, Campbell HJ, Cox SC, Crampton JS, et al. 2014. High-level stratigraphic scheme for New Zealand rocks. New Zealand Journal of Geology and Geophysics. 57:402–419. doi: 10.1080/00288306.2014.946062
- Mortimer N, Tulloch AJ, Ireland TR. 1997. Basement geology of Taranaki and Wanganui Basins, New Zealand. New Zealand Journal of Geology and Geophysics. 40:223–236. doi: 10.1080/00288306.1997.9514754
- Mortimer N, Tulloch AJ, Spark RN, Walker NW, Ladley E, Allibone A, Kimbrough DL. 1999. Overview of the Median Batholith, New Zealand: a new interpretation of the geology of the Median Tectonic Zone and adjacent rocks. Journal of African Earth Sciences. 29:257–268. doi: 10.1016/S0899-5362(99)00095-0
- Muir RJ, Ireland TR, Weaver SD, Bradshaw JD, Evans JA, Eby GN, Shelley D. 1998. Geochronology and geochemistry of a Mesozoic magmatic arc system, Fiordland, New Zealand. Journal of the Geological Society. 155:1037–1053. doi: 10.1144/gsjgs.155.6.1037
- Muir RJ, Weaver SD, Bradshaw JD, Eby GN, Evans JA. 1995. The Cretaceous Separation Point batholith, New Zealand: granitoid magmas formed by melting of mafic lithosphere. Journal of the Geological Society, London. 152:689–701. doi: 10.1144/gsjgs.152.4.0689
- Muir RJ, Weaver SD, Bradshaw JD, Eby GN, Evans JA, Ireland TR. 1996. Geochemistry of the Karamea Batholith, New Zealand and comparisons with the Lachlan Fold Belt granites of SE Australia. Lithos. 39:1–20. doi: 10.1016/S0024-4937(96)00017-5
- Münker C. 1993. Geology and geochemistry of metavolcanic rocks west of Cobb reservoir, northwest-Nelson, New Zealand [Unpublished thesis]. Göttingen, Germany: Georg August Universität; 185 p.
- Münker C. 1997. Geochemical and isotopic systematics of the Cambrian Devil River volcanics in the Takaka Terrane, New Zealand [Unpublished PhD thesis]. Göttingen, Germany: Georg August Universität; 166 p.
- Münker C. 2000. The isotope and trace element budget of the Cambrian Devil River arc system, New Zealand: Identification of four source components. Journal of Petrology. 41:759–788. doi: 10.1093/petrology/41.6.759
- Münker C, Cooper RA. 1999. The Cambrian arc complex of the Takaka Terrane, New Zealand: An integrated stratigraphical, paleontological and geochemical approach. New Zealand Journal of Geology and Geophysics. 42:415–445. doi: 10.1080/00288306.1999.9514854
- Nathan S. 1976. Geochemistry of the Greenland Group (early Ordovician), New Zealand. New Zealand Journal of Geology and Geophysics. 19:683–706. doi: 10.1080/00288306.1976.10426314
- Nathan S. 1999. Petrographic notes on samples from the Glasgow and Victoria Ranges. Immediate report. GNS Science.
- Nebel-Jacobsen Y, Münker C, Nebel O, Mezger K. 2011. Precambrian sources of Early Paleozoic SE Gondwana sediments as deduced from combined Lu–Hf and U–Pb systematics of detrital zircons, Takaka and Buller terrane, South Island, New Zealand. Gondwana Research. 20:427–442. doi: 10.1016/j.gr.2010.11.014
- Neilson MJ, Brockman GF. 1977. The error associated with point-counting. American Mineralogist. 62:1238–1244.
- Nicholson KN, Black PM. 2004. Cretaceous to early Tertiary basaltic volcanism in the Far North of New Zealand: geochemical associations and their tectonic significance. New Zealand Journal of Geology and Geophysics. 47:437–446. doi: 10.1080/00288306.2004.9515069
- Palmer K. 1995. Chemical and petrographic analyses of Paleozoic-Mesozoic igneous and metasedimentary rocks from various parts of New Zealand. Wellington, New Zealand: Institute of Geological and Nuclear Sciences 95/16. 37 p.
- Pancost RD, Taylor KWR, Inglis GN, Kennedy EM, Handley L, Hollis CJ, Crouch EM, Pross J, Huber M, Schouten S, et al. 2013. Early Paleogene evolution of terrestrial climate in the SW Pacific, Southern New Zealand. Geochemistry, Geophysics, Geosystems. 14:5413–5429. doi: 10.1002/2013GC004935
- Pearce JA, Harris NBW, Tindle AG. 1984. Trace element discrimination diagrams for the tectonic interpretation of granitic rocks. Journal of Petrology. 25:956–983. doi: 10.1093/petrology/25.4.956
- Pickett DA, Wasserburg GJ. 1989. Neodymium and strontium isotopic characteristics of New Zealand granitoids and related rocks. Contributions to Mineralogy and Petrology. 103:131–142. doi: 10.1007/BF00378499
- Pound KS, Cooper RA, Grapes RH, Stedman H. 1993. Northwest Nelson - basement geology. Wellington, New Zealand. Geologial Society of New Zealand Miscellaneous Publication. 79B:75–146.
- Price RC, Mortimer N, Smith IEM, Maas R. 2015. Whole-rock geochemical reference data for Torlesse and Waipapa terranes, North Island, New Zealand. New Zealand Journal of Geology and Geophysics. 58:213–228. doi: 10.1080/00288306.2015.1026832
- Rabone SDC. 1977. Molybdenum-base metal-bismuth mineralisation at Eliot Creek, Karamea Bend, and Taipo Spur, northwest Nelson, New Zealand [Unpublished PhD thesis]. Auckland, New Zealand: University of Auckland.
- Rad F. 2015. PEP 38451 Romney-1 well completion report. NZP&M, Ministry of business, innovation & employment (MBIE). New Zealand Unpublished Petroleum Report PR4951.
- Raine JI, Kennedy EM, Griffin AG, Clowes CD. 2016. New Zealand mid-Cretaceous coal abundance, flora and climate. GNS Science Report 2016/68. GNS Science, Lower Hutt. 78 p.
- Randall SB. 2004. Granite intrusions & Greenland Group metasediments from the Haast region, South Westland [Unpublished BSc Hons thesis]. Dunedin, New Zealand: University of Otago.
- Rattenbury MS, Cooper RA, Johnston MR, Forsyth PJ. 1998. Geology of the Nelson area. Institute of Geological & Nuclear Sciences 1:250 000 geological map 9. 1 sheet +67 p. Lower Hutt, New Zealand: Institute of Geological & Nuclear Sciences Limited.
- Reed JJ. 1958. Regional metamorphism in south-east Nelson. New Zealand Geological Survey bulletin. 60:64 p.
- Reilly C, Nicol A, Walsh JJ, Seebeck H. 2015. Evolution of faulting and plate boundary deformation in the Southern Taranaki Basin, New Zealand. Tectonophysics. 651–652:1–18. doi: 10.1016/j.tecto.2015.02.009
- Richard A, Banks DA, Mercadier J, Boiron M-C, Cuney M, Cathelineau M. 2011. An evaporated seawater origin for the ore-forming brines in unconformity-related uranium deposits (Athabasca Basin, Canada): Cl/Br and δ37Cl analysis of fluid inclusions. Geochimica et Cosmochimica Acta. 75:2792–2810. doi: 10.1016/j.gca.2011.02.026
- Roser BP, Cooper RA, Nathan S, Tulloch AJ. 1996. Reconnaissance sandstone geochemistry, provenance, and tectonic setting of the lower Paleozoic terranes of the West Coast and Nelson, New Zealand. New Zealand Journal of Geology and Geophysics. 39:1–16. doi: 10.1080/00288306.1996.9514690
- Roser BP, Korsch RJ. 1988. Provenance signatures of sandstone-mudstone suites determined using discriminant function analysis of major-element data. Chemical Geology. 67:119–139. doi: 10.1016/0009-2541(88)90010-1
- Ryland CB. 2011. The geology and structural evolution of the Bald Hill-Maori Saddle region, South Westland, New Zealand [Unpublished MSc thesis]. Dunedin, New Zealand: University of Otago; 237 p.
- Sagar MW. 2014. Geology, petrology and thermochronology of the Glenroy Complex and associated granitoids, southeast Nelson, New Zealand [Unpublished PhD thesis]. Dunedin, New Zealand: University of Otago.
- Sagar MW, Palin JM. 2011. Emplacement, metamorphism, deformation and affiliation of mid-Cretaceous orthogneiss from the Paparoa Metamorphic Core Complex lower-plate, Charleston, New Zealand. New Zealand Journal of Geology and Geophysics. 54:273–289. doi: 10.1080/00288306.2011.562904
- Sahoo TR, King PR, Bland KJ, Strogen DP, Sykes R, Bache F. 2014. Tectono-sedimentary evolution and source rock distribution of the mid to Late Cretaceous succession in great South Basin, New Zealand. APPEA Journal. 54:259–274. doi: 10.1071/AJ13026
- Sarll O. 1996. Rameka Creek geology, Paleozoic plutonism and structure of the Takaka Terrane [Unpublished BSc Hons thesis]. Dunedin, New Zealand: University of Otago.
- Saunders JK. 1997. The petrology, geochemistry and petrogenesis of the Riwaka Complex, South Island, New Zealand [Unpublished thesis]. Newfoundland, Canada: Memorial University of Newfoundland; 147 p.
- Scott JM. 2008. Tectonic evolution of the Eastern Fiordland Gondwana margin [Unpublished PhD thesis]. Dunedin, New Zealand: University of Otago.
- Scott JM, Palin JM. 2008. LA-ICP-MS U-Pb zircon ages from Mesozoic plutonic rocks in eastern Fiordland, New Zealand. New Zealand Journal of Geology and Geophysics. 51:105–113. doi: 10.1080/00288300809509853
- Smith BA. 1993. Eastern Fiordland geology [Unpublished MSc thesis]. Dunedin, New Zealand: University of Otago.
- Smithies SL. 2018. Provenance analysis of the Late Cretaceous to Paleocene Rakopi, North Cape, and Farewell formations, northwest Nelson, New Zealand [Unpublished MSc thesis]. Christchurch, New Zealand: University of Canterbury; 210 p.
- Stallard AR. 1994. An investigation of the geology and tectonics of the Bay Schist in the context of the Buller Terrane-Takaka Terrane boundary [Unpublished MSc thesis]. Christchurch, New Zealand: University of Canterbury; 124 p.
- Stark CJ. 1996. Interpretation of some Paleocene fluvial sediments from the upper Pakawau and Kapuni groups, Pakawau Sub-Basin, north-west Nelson [Unpublished MSc thesis]. Christchurch, New Zealand: University of Canterbury; 220 p.
- Steadman RD. 2017. Provenance and porosity analysis of the Greymouth Basin, New Zealand [Unpublished MSc thesis]. Christchurch, New Zealand: University of Canterbury; 202 p.
- Stewart M. 1988. The geology of the Cobb reservoir area, North West Nelson [Unpublished MSc thesis]. Christchuch, New Zealand: University of Canterbury; 106 p.
- Strogen DP, Seebeck H, Nicol A, King PR. 2017. Two-phase Cretaceous – Paleocene rifting in the Taranaki Basin region, New Zealand; implications for Gondwana break-up. Journal of the Geological Society. 174:929–946. doi: 10.1144/jgs2016-160
- Strong DT, Turnbull RE, Haubrock SN, Mortimer N. 2016. Petlab: New Zealand’s national rock catalogue and geoanalytical database. New Zealand Journal of Geology and Geophysics. 59:475–481. doi: 10.1080/00288306.2016.1157086
- Suggate RP. 1956. Puponga Coalfield. New Zealand Journal of Science and Technology. 37:539–559.
- Sun S, McDonough WF. 1989. Chemical and isotopic systematics of oceanic basalts: implications for mantle composition and processes. Geological Society, London, Special Publications. 42:313–345. doi: 10.1144/GSL.SP.1989.042.01.19
- Taylor SR, McLennan SM 1985. The continental crust: its composition and evolution. Hallam A, editor. Oxford, London, Edinburgh, Boston, Palo Alto, Carlton, Blackwell Scientific Publications. 312 p.
- Thrasher GP. 1990. Tectonics of the Taranaki Rift. 1989 New Zealand petroleum conference proceedings. Wellington, New Zealand: Petroleum and Geothermal Unit, Energy and Resources Division, Ministry of Commerce; p. 124–133
- Thrasher GP. 1992. Late Cretaceous geology of Taranaki Basin, New Zealand [Unpublished PhD thesis]. Wellington, New Zealand: Victoria University of Wellington; 252 p.
- Titheridge DG. 1977. Stratigraphy and sedimentology of the upper Pakawau and lower Westhaven groups (upper Cretaceous - Oligocene), northwest Nelson [Unpublished MSc thesis]. Christchurch, New Zealand: University of Canterbury; 146 p.
- Tulloch AJ. 1979. Plutonic and metamorphic rocks in the Victoria Range segment of the Karamea Batholith, Southwest Nelson, New Zealand [Unpublished MSc thesis]. Dunedin, New Zealand: University of Otago; 301 p.
- Tulloch AJ. 1983. Granitoid rocks of New Zealand - A brief review. Memoir of the Geological Society of America: Circum-Pacific plate tectonics. 159:5–20. doi: 10.1130/MEM159-p5
- Tulloch AJ, Challis GA. 2000. Emplacement depths of Paleozoic-Mesozoic plutons from western New Zealand estimated by hornblende-Al geobarometry. New Zealand Journal of Geology and Geophysics. 43:555–567. doi: 10.1080/00288306.2000.9514908
- Tulloch AJ, Kimbrough DL. 1989. The Paparoa Metamorphic Core Complex, New Zealand: Cretaceous extension associated with fragmentation of the Pacific margin of Gondwana. Tectonics. 8:1217–1234. doi: 10.1029/TC008i006p01217
- Tulloch AJ, Kimbrough DL. 2003. Paired plutonic belts in convergent margins and the development of high Sr/Y magmatism: Peninsular ranges batholith of Baja-California and Median Batholith of New Zealand. Geological Society of America Special Paper. 374:275–295.
- Tulloch AJ, Mortimer N. 2017. New basement map of Taranaki Basin. 2nd New Zealand Petroleum Geoscience Workshop. Lower Hutt, New Zealand.
- Tulloch AJ, Rabone SDC. 1993. Mo-bearing granodiorite porphyry plutons of the Early Cretaceous Separation Point Suite, west Nelson, New Zealand. New Zealand Journal of Geology and Geophysics. 36:401–408. doi: 10.1080/00288306.1993.9514586
- Tulloch AJ, Ramezani J, Kimbrough DL, Faure K, Allibone AH. 2009. U-Pb geochronology of mid-Paleozoic plutonism in western New Zealand: Implications for S-type granite generation and growth of the east Gondwana margin. GSA Bulletin. 121:1236–1261. doi: 10.1130/B26272.1
- Turnbull RE, Tulloch AJ, Ramezani J. 2013. Zetland Diorite, Karamea Batholith, west Nelson: field relationships, geochemistry and geochronology demonstrate links to the Carboniferous Tobin Suite. New Zealand Journal of Geology and Geophysics. 56:83–99. doi: 10.1080/00288306.2013.775166
- Uruski CI. 2008. Deepwater Taranaki, New Zealand: structural development and petroleum potential. Exploration Geophysics. 39:94–107. doi: 10.1071/EG08013
- van der Plas L, Tobi AC. 1965. A chart for judging the reliability of point counting results. American Journal of Science. 263:87–90. doi: 10.2475/ajs.263.1.87
- Waight TE, Weaver SD, Muir RJ, Maas R, Eby GN. 1998. The Hohonu Batholith of North Westland, New Zealand: granitoid compositions controlled by source H2O contents and generated during tectonic transition. Contributions to Mineralogy and Petrology. 130:225–239. doi: 10.1007/s004100050362
- Weaver SD, Pankhurst RJ. 1991. A precise Rb-Sr age for the Mandamus Igneous Complex, North Canterbury, and regional tectonic implications. New Zealand Journal of Geology and Geophysics. 34:341–345. doi: 10.1080/00288306.1991.9514472
- Whalen JB, Currie KL, Chappell BW. 1987. A-type granites: geochemical characteristics, discrimination and petrogenesis. Contributions to Mineralogy and Petrology. 95:407–419. doi: 10.1007/BF00402202
- Wilding A, Sweetman LAD, BP Shell Aquitaine Todd Petroleum Developments Ltd. 1971. Endeavour-1 well completion report. Ministry of Economic Development New Zealand Unpublished Petroleum Report PR303.
- Windle SJ, Craw D. 1991. Gold mineralisation in a syntectonic granite dike, Sams Creek, northwest Nelson, New Zealand. New Zealand Journal of Geology and Geophysics. 34:429–440. doi: 10.1080/00288306.1991.9514481
- Winter JD. 2014. Principles of igneous and metamorphic petrology, 2nd ed. Harlow, Essex: Pearson Education Limited. 740 p.
- Wizevich MC. 1992. Petrography of sandstones in the Pakawau Basin, northwest Nelson. In: Nobes DC, editor. Geological Society of New Zealand and New Zealand Geophysical Society Joint Annual Conference. Christchurch, New Zealand: University of Canterbury; p. 164–164.
- Wizevich MC. 1994. Sedimentary evolution of the onshore Pakawau Subbasin: rift sediments of the Taranaki Basin deposited during Tasman Sea spreading. In: Van der Lingen GJ, Swanson KM, Muir RJ, editor. Evolution of the Tasman Sea Basin. Rotterdam, Netherlands: A.A. Balkema; p. 83–104.
- Wizevich MC, Thrasher GP, Bussell MR, Wilson GJ, Collen JD. 1992. Evidence for marine deposition in the Late Cretaceous Pakawau Group, northwest Nelson. New Zealand Journal of Geology and Geophysics. 35:363–369. doi: 10.1080/00288306.1992.9514529