ABSTRACT
The Waimea-Flaxmore Fault System (W-FFS) comprises NE-striking, SE-dipping reverse faults superposing Eastern Province terranes on Cenozoic sedimentary units of the Moutere Depression. We present a revised geological map and cross sections of the W-FFS in the Nelson-Richmond urban area, aimed at reconstructing the structural evolution during multiple phases of reactivation. Our data and structural interpretations depict the inversion of the W-FFS from an inherited system of Late Cretaceous-Eocene normal faults to multiple reverse fault splays that propagated through the cover sequence over the last 10 Ma. Fault tip propagation with folding of the cover sequence persisted during the Quaternary, with breaching of the Waimea and Flaxmore faults to the surface and propagation of a splay thrust (Tahunanui Fault) still concealed beneath Quaternary deposits. Ongoing crustal shortening associated with the Pacific-Australian plate boundary in the northern South Island creates favourable conditions for reactivation of the long-lived W-FFS in the contemporary stress field.
Introduction
At the southern tip of the Hikurangi margin, the transition from subduction of the Pacific Plate to continental collision along the right-lateral-oblique Alpine-Marlborough faults () controls tectonic deformation and associated seismicity in the north-central South Island (e.g. Eberhart-Phyllips and Reyners Citation1997; Eberhart-Phyllips and Bannister Citation2010; Wallace et al. Citation2012). West of the Alpine Fault, the Australian crust above the Pacific Plate is shortened by sets of NNE-SSW to NE-SW reverse faults and folds that have been active since the inception of the plate boundary in the mid Miocene (Nathan et al. Citation1986; Bishop and Buchanan Citation1995; King Citation2000; Ghisetti et al. Citation2016; Citation2018; Okada et al. Citation2019). Some reverse faults display high angle dips and harpoon-style (McClay Citation1995) folding of Late Cretaceous rocks in their hanging wall, consistent with reverse reactivation of inherited normal faults (Bishop and Buchanan Citation1995; King and Thrasher Citation1996; Ghisetti and Sibson Citation2006). Many of these faults remain active in the contemporary stress field (Ghisetti et al. Citation2014; Citation2016) under horizontal compressional stress trajectories trending c. 110° +/− 15° (Sibson et al. Citation2012; Townend et al. Citation2012). Data on moderate to large historical earthquakes in the north-west of the South Island (e.g. 1929 M7.8 Murchison earthquake; 1968 M7.1 Inangahua earthquake; 1962 M∼6 Westport earthquake; 1991 M∼6 Hawk’s Crag earthquakes; Anderson et al. Citation1993; Citation1994; Doser et al. Citation1999) indicate near-pure reverse slip on NNE-SSW to NE-SW faults.
Figure 1. Regional tectonic setting of the north-central South Island in relation to the Pacific-Australian plate boundary. The right-lateral Marlborough Fault System (including the Wairau, Awatere, Clarence, Kekerengu and Hope faults) provides the kinematic transition from the Hikurangi subduction margin to the Alpine Fault transpressive plate boundary. The Waimea-Flaxmore Fault System (W-FFS) is one of the largest structures west of the plate boundary. The W-FFS continues offshore into the structural belt comprising the Manaia and Taranaki faults. RB-MF is the sub-parallel Ruby Bay-Moutere Fault, continuous with the Surville Fault offshore (see ). Contour depths of the subduction interface after Williams et al. (Citation2013). Red arrows show the relative motion vector and velocity between the Pacific and Australian plates (from Wallace et al. Citation2012). Black arrows show the average direction (c. 110°) of the axis of maximum horizontal compressional stress (σ1) from Sibson et al. (Citation2012) and Townend et al. (Citation2012).
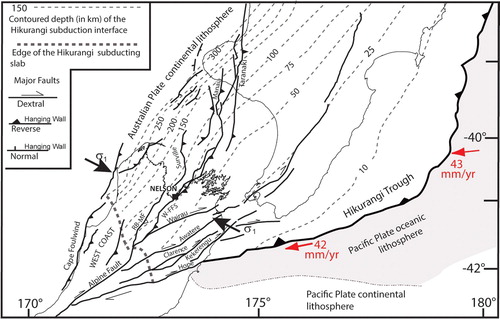
The Waimea-Flaxmore Fault System (W-FFS) is one of the largest, active reverse structures located west of the plate boundary in the northern South Island (). At its southern termination the W-FFS intersects the Alpine Fault south-west of Lake Rotoroa (), close to the area of convergence with the Wairau Fault (Rattenbury et al. Citation2006; Langridge et al. Citation2016). From there, the W-FFS extends northward, and then northeastward, bounding the eastern margin of the Moutere Depression. North-east of Nelson, the W-FFS continues offshore joining the Manaia Fault, which belongs to the Taranaki Fault System at the eastern margin of the Taranaki Basin (King and Thrasher Citation1996; Stagpoole and Nicol Citation2008; Bull et al. Citation2019). Offshore seismic data (Voggenreiter Citation1993; King and Thrasher Citation1996; Reilly et al. Citation2015) have demonstrated the long history of reactivation of the Taranaki and Manaia faults, and geophysical data (Stern and Davey Citation1990; Henrys et al. Citation2013) show that the Taranaki Fault offsets the Moho of the Australian Plate, with reverse separation up to 12–15 km (Stagpoole and Nicol Citation2008). The W-FFS, Manaia and Taranaki faults are interpreted as part of a >600 km long lithospheric back-thrust system exploiting the suture zone between the Median Batholith and Eastern Province terranes (King and Thrasher Citation1996; Mortimer et al. Citation1997; Muir et al. Citation2000; Ghisetti et al. Citation2018).
Figure 2. Tectonic setting of the study area and adjacent Moutere Depression. A, Simplified geological map (redrawn from Rattenbury et al. Citation1998; Citation2006). The black dots show the locations of exploration wells: Ta1, Tapawera-1; RB1, Ruby Bay-1; Tu1, Tuatara-1; Su1, Surville-1 (http://www.nzpam.govt.nz). AF: Alpine Fault; LR: Lake Rotoroa. B, Cross section showing the superposition of basement terranes, the faulting of the Cenozoic cover sequence within the Moutere Depression and the position of the Ruby-Bay Moutere structural high (bounded to the west by the Ruby Bay-Moutere Fault, RB-MF) relative to the adjacent Waimea-Flaxmore Fault System (W-FFS). The subsurface interpretation in the Moutere Depression along this section (see Ghisetti et al. Citation2018 for detail) is based on the MD87-1 seismic line (Seismograph Service Ltd Citation1989). RB1 is the Ruby Bay-1 exploration well projected on the trace of the section. MD87-1 does not extend to the SE across the W-FFS, and there are no available seismic data for interpreting the subsurface geometry of the W-FFS.
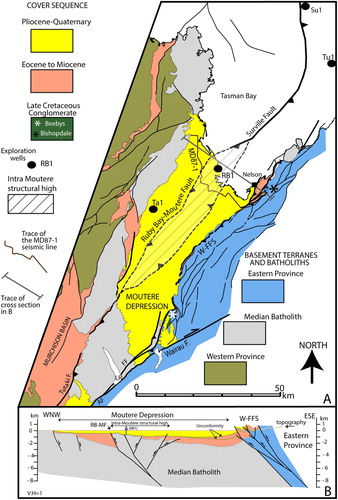
Geometry and structural setting of the onshore part of the W-FFS have been defined by previous studies (Johnston Citation1979; Citation1981; Pettinga and Wise Citation1994), with focus on Quaternary activity and Holocene paleo-seismicity (Fraser Citation2005; Johnston and Nicol Citation2013). However, there has not been so far a comprehensive structural reconstruction of the long-term evolution and deformation mechanisms of the W-FFS in relation to its possible structural inheritance.
In this paper, we use unpublished geological surveys and bore logs, together with LiDAR imagery to reconstruct the structural setting of segments of the northernmost onshore segment of the W-FFS exposed in the Nelson-Richmond urban area. New mapping and cross sections depict the W-FFS and related folding of Cenozoic sedimentary units. Structural reconstructions and forward models show that the W-FFS is a long-lived, basement-penetrating structure that has accommodated increasing amounts of shortening during the Neogene and Quaternary by reverse reactivation, following earlier episodes of normal slip in the Late Cretaceous-Eocene. The W-FFS comprises older fault segments with larger displacement that are exposed at surface, but some fault splays that propagated at a later stage are blind and imprecisely located beneath Quaternary alluvial cover. The largest faults of the W-FFS have similar geometry and structural evolution, suggesting that they can be reactivated in the contemporary stress field, with implications for seismotectonic hazard in the Nelson-Richmond urban area.
Geology of units juxtaposed along the W-FFS in the Nelson-Richmond urban area
In the Nelson-Richmond urban area the W-FFS comprises several NE-striking, SE-dipping reverse faults (from east to west: Eighty-Eight, Waimea, Flaxmore, West Flaxmore, Tahunanui and associated splays) that imbricate Eastern Province terranes above the Median Batholith and the overlying Cenozoic sedimentary cover of the Moutere Depression ( and ). There are no onshore seismic data to constrain the geometry of units and faults at depth, but vintage seismic data in the adjacent Moutere Depression recently re-interpreted by Ghisetti et al. (Citation2018) show the presence of an intra-Moutere structural high of the Median Batholith (). This high is bounded to the west by the steeply SE-dipping Ruby Bay-Moutere Fault (RB-MF), linked to the Surville Fault offshore. The reverse RB-MF is a blind fault sub-parallel to the W-FFS (c. 15 km to the west of it), interpreted to have been active from the Middle Miocene to the Pleistocene as part of the same regional fault set that includes the W-FFS (Ghisetti et al. Citation2018).
The geology of units truncated and juxtaposed along the W-FFS was mapped by Johnston (Citation1979; Citation1981; Citation1982). These maps have provided the basis for subsequent regional compilations (Rattenbury et al. Citation1998), but otherwise they have not been revised to incorporate accumulating stratigraphic and structural data made available by new exposures at building sites and in boreholes and trenches, as well as new geomorphic information from LiDAR surveys undertaken by the Nelson City and Tasman District councils.
Data acquired after publication of Johnston’s (Citation1979; Citation1981; Citation1982) geological maps and disseminated through a number of unpublished documents, together with field revisions carried out by the authors and morpho-tectonic analysis of LiDAR imagery, provide the basis for the revised geological map of and the cross sections of . Our revision focuses on the geology and structural setting of the sedimentary cover in relation to the W-FFS, whilst we refer to Johnston (Citation1979; Citation1981; Citation1982) for detailed structural mapping of the basement units.
Figure 3. Simplified geological map of the Nelson-Richmond urban area showing the W-FFS and associated structures. Location of , A–C and A,B is marked by black squares and relative number. The cross sections are in . See for the chrono-stratigraphy and facies of the formations of the cover sequence.
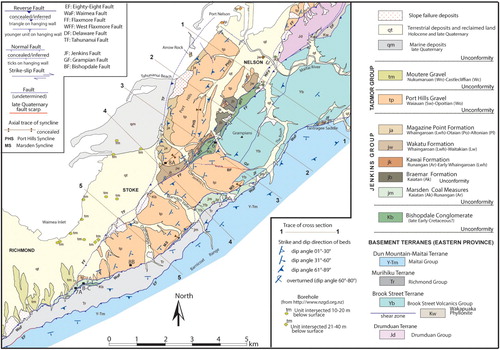
Figure 4. Cross sections illustrating the structure of terranes and sedimentary cover sequence in the Nelson-Richmond urban area. A, Section 1. B, Section 2. C, Section 3. D, Section 4. E, Section 5. Traces in . Units projected above topography are shown in half colour. Refer to and for age of units.
In the following, we describe the major units juxtaposed along different strands of the W-FFS, with emphasis on the stratigraphic and structural relations essential for interpreting the tectonic evolution. The reader is referred to Johnston (Citation1979; Citation1981; Citation1982; Citation1984; Citation1990; Johnston et al. Citation1987; Rattenbury et al. Citation1998; Mortimer et al. Citation1999; Mortimer Citation2004) for more detailed descriptions and comprehensive reference lists.
Basement rocks
The basement rocks in outcrop in the Nelson-Richmond area belong to a stack of thrust sheets that imbricate Eastern Province terranes onto the Median Batholith (Edbrooke Citation2017). The terranes were accreted at the Paleozoic-Early Cretaceous convergent margin of Gondwana prior to Late Cretaceous rifting (110-85 Ma), with opening of the Tasman Sea (Laird and Bradshaw Citation2004; Mortimer et al. Citation2017). The geometry of the basement units and bounding shear zones has been strongly modified by dextral strike-slip of c. 470 km along the Alpine Fault (Wellman Citation1971; Sutherland Citation1999) and associated shortening. The basement units and their boundaries dip steeply, a likely consequence of a long history of superposed deformation, from Late Cretaceous extensional rifting to Neogene-Quaternary rotation, shortening and uplift.
The sedimentary cover within the Moutere Depression and in the Nelson-Richmond urban area masks the contact between the Carboniferous-earliest Cretaceous intrusions of the Median Batholith and the Permian-Mesozoic Eastern Province terranes uplifted in the hanging wall of the W-FFS (). Oil exploration wells in the Moutere Depression and its offshore (Tapawera-1, Ruby Bay-1, Surville-1, Tuatara-1, http://www.nzpam.govt.nz, A) have reached Late Jurassic-Early Cretaceous diorites (Rotoroa Complex) and Early Cretaceous granitoids (Separation Point Suite) belonging to the Median Batholith (Mortimer et al. Citation1997; Muir et al. Citation2000). Late Triassic-Jurassic? volcano-sedimentary assemblages intruded by Early Cretaceous granodiorites of the Median Batholith (Tasman Intrusives) have been recognised as part of the Drumduan Terrane (). This is the structurally lowest unit of the Eastern Province outcropping in the mapped area (Edbrooke Citation2017). The tectonic contact with the overlying units of the Brook Street Terrane (Permian volcanic arc rocks) is controlled by the Flaxmore and Delaware reverse faults (Johnston Citation1981). A belt of highly tectonised and foliated rocks and breccias exposed locally along the Delaware Fault (Wakapuaka Phyllonite, Late Jurassic-Early Cretaceous?) is interposed between the accreted terranes ( and A–C).
The tectonic superposition of the Murihiku Terrane above the Brook Street Terrane is mapped throughout New Zealand (Mortimer Citation2004; Mortimer et al. Citation2014; Edbrooke Citation2017), though extreme lamination of these terranes occurs in the areas of dextral oroclinal bending next to the Alpine Fault (Sutherland Citation1999).
In the Nelson-Richmond urban area, rock units of the Murihiku Terrane (Upper Triassic sandstones, siltstones and conglomerates of the Richmond Group, cf. Johnston Citation1979, with andesitic dykes) are discontinuous and reduced to a narrow tectonic slice in outcrop in the south of the mapped area (). At its base (between Richmond and Jenkins Creek) the slice is thrust above Paleogene-Neogene rocks along the Waimea Fault. At its top, it is overthrust by the Dun Mountain-Maitai Terrane along the Eighty-Eight Fault. In upper Jenkins Creek, the Waimea and Eighty-Eight faults converge into one strand, with lateral closure of the Richmond Group and direct tectonic superposition of the Maitai Group above Brook Street Volcanics (). Thus, in the mapped area there is no contact of the Richmond Group above the Brook Street Volcanics. However, sheared wedges of the Richmond Group between Brook Street and Maitai units are present to the north-east of the mapped area (Johnston Citation1981) and are inferred to lie beneath the Waimea Fault at depth (). The Late Permian to Middle Triassic units of the Maitai Group uplifted in the hanging wall of the Eighty-Eight and Waimea faults belong to the highest imbricate sheet of basement rocks in the mapped area.
Late Mesozoic and Cenozoic sedimentary units
summarises the chrono-stratigraphy of the sedimentary succession in the Nelson-Richmond urban area. We refer to Johnston (Citation1979; Citation1981; Citation1982) for detailed descriptions of lithology, facies and age. The formations have local names, but correlative chrono-stratigraphic units are exposed at the margins of the Moutere Depression and in the Murchison Basin (Johnston Citation1971; Citation1983; Citation1990; King et al. Citation1999; Ghisetti et al. Citation2018), and have been drilled onshore at Tapawera-1 and Ruby Bay-1, and offshore at Tuatara-1 and Surville-1 (http://www.nzpam.govt.nz) (A). The following description employs international ages; the equivalent New Zealand chronology is provided in and used for the legend of the geological map ().
Figure 5. Chrono-stratigraphic column of the formations of the cover sequence in the Nelson-Richmond urban area (). Data from Johnston (Citation1979; Citation1981; Citation1982). Basement terranes below the cover sequence are: Jd, Drumduan; Yb, Brook Street (with the interposed Wakapuaka Phyllonite, Kw); Tr, Murihiku (Richmond Group); Y-Tm, Dun Mountain-Maitai. The Median Batholith (MB) is not in outcrop (see also B). International and New Zealand stratigraphic divisions to the right of the chronological scale provide the key for age of formations on the legend of the geological map ().
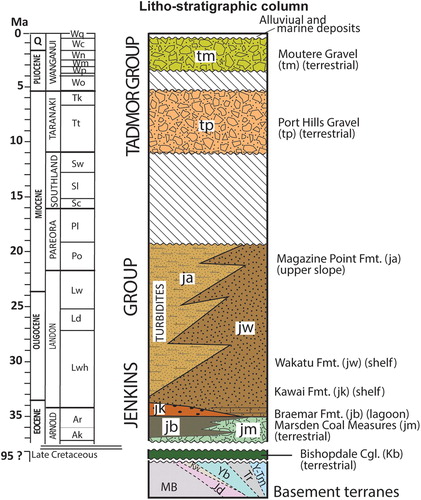
Stratigraphic correlations (Nathan et al. Citation1986; Laird Citation1995; King and Thrasher Citation1996; King et al. Citation1999; Adams et al. Citation2017) and structural retro-deformations (Ghisetti et al. Citation2014; Citation2016) delineate the stratigraphic evolution related to progressive break-up of the Gondwana convergent margin, with separation and drifting of Zealandia, followed by establishment of the Neogene Pacific-Australian plate boundary across New Zealand (King Citation2000; Mortimer et al. Citation2017).
This evolution can be traced in the Nelson-Richmond urban area and its environs with: (1) deposition of terrestrial clastic units within fault-controlled basins during pulses of rifting in late Early Cretaceous (c.105-83 Ma) and Late Cretaceous-Paleocene (c. 80-55 Ma). (2) Unconformable deposition of post-rift Paleogene sequences transitioning from terrestrial coal measures (c. 37-35 Ma) to transgressive marine units (c. 35-25 Ma) within subsiding basins. (3) Dominant silicic-clastic sedimentation at c. 25-22 Ma, with likely tectonic control on the position of turbiditic depocentres relative to adjacent shelves. (4) Sedimentary hiatus at c. 20-10 Ma, coeval with convergent tectonism along the Pacific-Australian plate boundary. (5) Progressive shortening and uplift since the late Miocene, accommodated by reverse faulting and folding, with erosion of the sedimentary cover and subsidence in syn-compressional depocentres infilled with regressive terrestrial sequences. A variety of depositional environments with local and far-field source areas, ongoing tectonism and the strong climatic control during the latest Pliocene to late Quaternary glacial events (Suggate Citation1965; Alloway et al. Citation2007) are all factors controlling large variations in facies, particularly composition and clast size, of the late Neogene and Quaternary terrestrial deposits.
The most significant elements that constrain the syn-tectonic control on the sedimentary cover and its subsequent deformation are outlined below.
Bishopdale Conglomerate (late Early Cretaceous?)
The inferred oldest formation of the sedimentary cover sequence in the Nelson-Richmond urban area is the Bishopdale Conglomerate ( and ). It comprises sub-vertical, sheared conglomerate and breccia embedded in a rusty-red, shaly matrix (). The clasts include diorites, volcaniclastic sandstones, mafic lavas, andesites and ignimbrite, all suggesting local provenance from the Median Batholith, Brook Street and Drumduan rocks.
Figure 6. Bishopdale Conglomerate in the Bishopdale subdivision, Nelson City. A, Steeply SE-dipping slivers of tectonically stretched clasts in red shaly matrix. B, Fabric of tectonically stretched clasts truncated by shear zones. C, Detail (see hammer for scale) of small-scale shear zones and gouges within the deformed unit. Sites of A, B, C are in close proximity, near location NZTM2000 1622230E 5427880N (shown by the black square labelled 6 in Figure 3). These outcrops have now been obliterated by urban development.
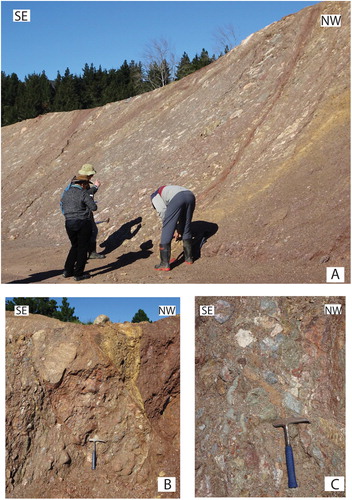
Discontinuous outcrops of the Bishopdale Conglomerate (together with a dissimilar conglomerate that we now attribute to the Kawai Formation, see below), were originally mapped by Johnston (Citation1979) as part of the Late Eocene-Early Oligocene Bishopdale Formation. The Bishopdale Conglomerate was subsequently shown (Johnston Citation1981) to be much more extensive. Johnston (Citation1984) re-appraised the formation as being of likely late Early Cretaceous age and correlative of the Beebys Conglomerate (Johnston Citation1983; Citation1990). The Beebys Conglomerate (youngest unit of the Momotu Super Group, Adams et al. Citation2017) comprises interbedded sandstone, siltstone and conglomerate with carbonaceous lenses. These deposits unconformably overlie Brook Street rocks in the hanging wall of the Flaxmore Fault at the southern end of the Moutere Depression. Plant fossils date the formation as late Early Cretaceous (c. 100-90 Ma, Johnston Citation1990), a date also consistent with a recent study of detrital zircon ages (Adams et al. Citation2017).
Earthworks, particularly in the Bishopdale area (crossed by section line 3, ), have confirmed that the Bishopdale Conglomerate is continuous over a length of c. 5 km, but many outcrops have since been obliterated by ongoing urban development. In central Nelson, the formation is largely concealed beneath alluvial deposits, but is exposed close to the Flaxmore Fault at the northern end of the Grampians, and has been intersected by a borehole at depth of 19 m on the north-east side of the lower Maitai River (). Similar deposits are also exposed on the south bank of Marsden Valley and Orphanage Creek, in Stoke. The formation is tectonically interposed between the sub-parallel strands of the West Flaxmore and Flaxmore faults, with no preserved stratigraphic relations with the Brook Street rocks in the hanging wall and the Eocene-Paleogene formations in the footwall.
Outcrops of the Bishopdale Conglomerate have also been exposed in trenches (Wopereis Citation2011) and recent excavations along the Waimea Fault near Richmond ( and ), where the unit is tectonically interposed between the Richmond Group and Eocene coal measures.
Figure 7. Bishopdale Conglomerate along the Waimea Fault and associated splays in the Barnicoat Range. A, Waimea Fault exposed in a 0.8 m deep trench (Wopereis Citation2011). The fault is steeply dipping to the south and superposes units of the Richmond Group (Tr) above Bishopdale Conglomerate (Kb). A c. 25 cm thick belt of dark carbonaceous shales marks the fault zone. B, Waimea Fault (strike c. 090˚ dip 50˚S) exposed in a recent excavation. The main fault superposes units of the Richmond Group (Tr) above the Bishopdale Conglomerate (Kb). The lower splay superposes the Bishopdale Conglomerate (Kb) above Marsden Coal Measures (jm). C, Detail of the Waimea Fault exposed within the square in B. White carbonaceous claystone marks the contact. The outcrops have now been obliterated by ongoing development. Positions of A (around location NZTM2000 1617410E 5422680 N), B and C (around location NZTM2000 1617650E 5422810N) are shown by black squares respectively labelled A–C in .
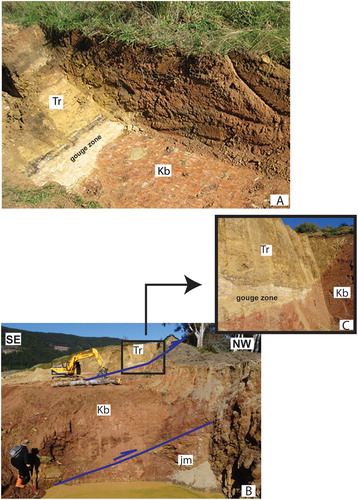
Eocene Marsden Coal Measures (basal terrestrial formation of the Jenkins Group)
The Eocene Marsden Coal Measures are the oldest formation of the Jenkins Group (), consisting of terrestrial, poorly-bedded quartz-rich sandstones and mudstones with interlayered coal seams. The formation is tectonically bounded by the Waimea Fault and sub-parallel fault splays in its footwall, with sheared, steeply-dipping beds between basement (Richmond and Maitai groups) and Bishopdale Conglomerate in the hanging wall and Late Miocene-Pliocene Port Hills Gravel and Moutere Gravel in the footwall. Small outcrops of Marsden Coal Measures are also overthrust by Bishopdale Conglomerate along the West Flaxmore Fault in Bishopdale (north of section line 3, ).
The base of the Marsden Coal Measures above Brook Street units is exposed in small outcrops in the hanging wall of a lower splay of the Waimea Fault between Jenkins Creek and Marsden Valley (along and close to the trace of Section 4 in ). At the top, the stratigraphic contact with the overlying Braemar Formation was temporarily exposed south of Braemar Hospital during urbanisation (Johnston Citation1979).
To the north-east of Jenkins Creek, along the upper course of The Brook, and at Tantragee Saddle (between the Brook and Maitai valleys, ), the Marsden Coal Measures are overthrust by Maitai Group along the Waimea Fault and downfaulted against Brook Street Volcanics along the steep, SE-dipping Jenkins Fault.
Paleogene to early Neogene marine formations of the Jenkins Group
The marine formations of the Jenkins Group include (from base to top) the Braemar (late Eocene), Kawai (Late Eocene to Early Oligocene), Wakatu (late Oligocene) and Magazine Point (Late Oligocene to Early Miocene) formations (). The newly named Kawai Formation introduced here comprises a conglomeratic unit that was previously included in the Bishopdale Formation (now Bishopdale Conglomerate). The Kawai Formation was well exposed in the 1970s adjacent to Kawai Street and in a temporary cutting in nearby Motueka Street. It is now poorly exposed in one small outcrop in the courtyard of Nelson Hospital.
The facies and chrono-stratigraphy of the Jenkins Group track the Eocene to Early Oligocene evolution of depositional environments (Johnston Citation1979) from lagoonal-deltaic and shallow marine to calcareous-rich shelf (Wakatu Formation). The onset of terrigenous input is recorded by the proximal siliciclastic turbidites and debris flows of the Magazine Point Formation (). The flows contain fossiliferous, andesitic and intrusive clasts (e.g. at Arrow Rock, ). The substantial age overlap of the Wakatu and Magazine Point formations (Johnston Citation1979) can be interpreted ( and ) in terms of a lateral facies transition between shelf and upper slope environments that persisted throughout the late Paleogene-early Neogene (Ghisetti et al. Citation2018). However, the subsurface relationships between Wakatu and Magazine Point formations are unknown, and only inferred in .
The formations of the Jenkins Group are exposed mainly on the flanks of the Port Hills Syncline. The oldest units are along the vertical to overturned south-eastern flank, in the footwall of the West Flaxmore Fault, whilst the youngest (Magazine Point Formation) is on the north-western, steeply SE-dipping flank, and well exposed along the coastline and at Tahunanui (). The basal contact of the Magazine Point Formation presumably underlies the Port Hills Gravel in the core of the syncline. The formations of the Jenkins Group exposed in Nelson are likely to be buried underneath the thick succession of late Miocene to Quaternary gravels within the Moutere Depression (B). The sedimentary succession is eroded in the hanging wall of the Flaxmore and Waimea faults, but a pocket of Wakatu Formation is preserved in the down-faulted wedge between the Waimea and Jenkins faults at Tantragee Saddle ().
Late Miocene and Plio-Quaternary terrestrial formations of the Tadmor Group
Following a c. 10 Myrs-long hiatus, the terrestrial Port Hills Gravel was deposited unconformably above the Jenkins Group and basement rocks in the late Miocene-earliest Pliocene ( and ).
In the mapped area, the Port Hills Gravel is exposed in the core of the Port Hills Syncline (in the footwall of the West Flaxmore Fault) and in a dominantly SE-dipping panel in the hanging wall of the Flaxmore Fault and footwall of the Waimea Fault. The Port Hills Gravel contains rounded clasts with dimensions ≤ 900 mm across. The gravel is locally bedded, matrix-supported and interlayered with claystone, mudstone and siltstone. Microflora within sparse carbonaceous lenses dates the formation to the Late Miocene-Early Pliocene (Johnston Citation1979). Locally the gravel is clast-supported and coarsely bedded, with poorly-sorted pebbles and boulders. These variations likely result from distinct depositional environments (braided rivers, fluvial channels, swamps, alluvial fans, debris flows).
The lower part of the formation is dominated by clasts sourced from the Median Batholith. Recently exposed outcrops at the southern end of the Port Hills Syncline, close to the base of the formation, contain relatively abundant granite and granodiorite boulders of likely derivation from the Separation Point and Rotoroa Complex, with rarer sandstones of the Richmond Group (A). While the igneous parent rocks are now only exposed west of the Moutere Depression, at the time of deposition the intra-Moutere structural high (Ghisetti et al. Citation2018) might also have been a source (). Contributions from basement units now exposed in the hanging wall of the Flaxmore and Waimea faults (Richmond and Maitai sandstones and siltstones, Brook Street Volcanics and, rarely, Dun Mountain Ophiolite) increase in the upper part of the formation. In the immediate footwall of the Waimea Fault the exposed top of the formation contains pebbles dominantly derived from the Richmond and Maitai groups (B).
Figure 8. Detail of Port Hills Gravel. A, Exposure close to the base of the formation. Coarse gravel cut by a minor fault. Clasts are dominantly granite and granodiorite of likely derivation from the Separation Point and Rotoroa Complex, with rarer sandstones of the Richmond Group. B, Exposure near the top of the formation. Bedded gravel with rounded clasts in silty matrix. Clasts are mainly from Maitai and Richmond groups, some have outer surface with Mn oxidation. Locations of A (NZTM2000 1620680E 5427190N) and B (NZTM2000 1619500E 5423570N) shown by black squares respectively labelled 8A and 8B in .
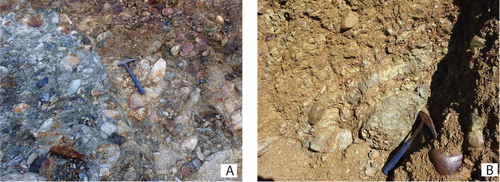
Following another hiatus, terrestrial sedimentation resumed with deposition of the predominantly greywacke-derived Moutere Gravel ( and ). The formation is exposed in narrow north-trending ridges eroded by the Holocene river network south-east of Richmond, where it is overthrust by the Waimea Fault. To the north, subsurface continuity of the Moutere Gravel beneath late Quaternary deposits is testified by several boreholes (http://www.nzgd.org.nz) ().
Within the Moutere Depression () the formation consists of weathered clasts of Torlesse-derived sandstones (Rakaia Terrane) and rarer quartz and chert and, in the south-west, semi-schists, embedded in a yellow-brown silty-clay matrix. Coarser sandstone clasts of local provenance from the Maitai and Richmond groups are very rare. Bedding is generally absent, though locally revealed by sandy interlayers. The Torlesse clasts point to a provenance from the Spenser Mountains, south of the Alpine Fault, with transport in alluvial fans. These deposits are only preserved within the Moutere Depression, but they probably covered a much larger area prior to the development of the depression (Ghisetti et al. Citation2018).
The base of the Moutere Gravel is not exposed in the Nelson-Richmond urban area, but in the adjacent Moutere Depression the formation conformably overlies the gravels of the Glenhope Formation (Johnston Citation1971; Ruby Bay-1; Tasman Petroleum Corporation Ltd Citation1966) of inferred Late Miocene (?)-Early Pliocene age (Mildenhall and Suggate Citation1981). In the Moutere Depression the Glenhope Formation and Moutere Gravel unconformably overlie both basement and Cenozoic units. In the mapped area, the Moutere Gravel is either tectonically overlain by the Port Hills Gravel or buried beneath late Quaternary deposits (). However, at the southern edge of the Moutere Depression the Moutere Gravel is unconformably overlain by glacial deposits of the Late Pliocene-Early Pleistocene (2.2-2.1 Ma) Porika Formation. Together with palynologic sampling (Mildenhall and Suggate Citation1981), these relationships indicate a probable Late Pliocene-Early Pleistocene age for the Moutere Gravel.
Principal faults of the W-FFS and associated structures
In the Nelson-Richmond urban area the principal faults of the W-FFS () have NE-SW strike and dip at moderate to steep angles to the SE. There are no seismic data that can be used to infer any change in fault dip with depth, and the cross sections () tentatively depict steep to moderately-dipping faults in the basement down to depths of c. 5 km.
In the following, we describe the principal structural features of individual faults of the W-FFS (from the structurally highest to the lowest) and associated folding.
Eighty-eight and Waimea faults and associated splays
The sub-parallel to obliquely intersecting Eighty-Eight and Waimea reverse faults (together with a number of unnamed splays in the footwall of the Waimea Fault) imbricate basement rocks and the oldest formations of the sedimentary succession (Bishopdale Conglomerate and Eocene Coal Measures) above the formations of the Tadmor Group in the Nelson-Richmond urban area. The faults strike NE-SW and are generally steeply dipping (c. 65°–70°) to the SE, though some fault splays dip at shallower angles (c. 30°–45°, B,C). Reverse separation of the top basement unconformity along the Waimea Fault is c. 3 km in Nelson city, decreasing to 2 km c. 25 km south-west of the mapped area (Ghisetti et al. Citation2018). Reverse slip appears to be dominant, but dextral offset of Quaternary terrace risers of c. 40 m has been reported by Johnston (Citation1983) for segments of the Waimea Fault c. 20 km south-west of the mapped area (between the Motueka River and Quail Valley).
The Eighty-Eight Fault (B) is the uppermost fault splay, superposing Maitai Group over Richmond Group. The Waimea Fault imbricates the Richmond Group over Marsden Coal Measures and, together with its footwall splays, superposes basement rocks and Marsden Coal Measures above the Port Hills and Moutere gravels ( and ). New exposures in the foothills of the Barnicoat Range have revealed a tectonic wedge of Bishopdale Conglomerate interposed between the Richmond Group and the Marsden Coal Measures in the footwall of the Waimea Fault ( and ).
To the north-east of upper Jenkins Creek the Eighty-Eight and Waimea faults converge into one single strand which superposes the Maitai Group units over Marsden Coal Measures. The largest stratigraphic separation is documented along the foothills of the Barnicoat Range, where the Richmond Group overthrusts the Moutere Gravel or, in the north, the Port Hills Gravel.
An unusual tectonic setting is shown in the down-faulted wedge along the upper course of The Brook and at Tantragee Saddle (), where Marsden Coal Measures are interposed between the normal Jenkins Fault to the north-west and the reverse Waimea Fault to the south-east. The two faults are sub-parallel and, at a maximum, only few hundred metres apart, but the Waimea Fault truncates the Jenkins Fault, with superposition of Maitai Group units above Brook Street Volcanics and the Marsden Coal Measures (A–C).
Holocene-active traces along preserved fault scarps of the Waimea Fault have been identified c. 6 km along-strike south-east of the mapped area, in the Wairoa Gorge. A 1–2 m high late Quaternary scarp along the Eighty-Eight Fault () has also been identified in the Barnicoat Range (Johnston and Nicol Citation2013), but the geomorphic escarpment indicates normal (up-slope down), rather than reverse slip displacement. Paleo-earthquake investigations (Fraser Citation2005; Johnston and Nicol Citation2013) have exposed ruptures related to three major events in the last 20 kyrs, with cumulative fault scarp height of c. 3.5 m, and average slip rate of c. 0.2 mm/yr. The youngest rupture is dated c. 5 kyrs BP.
Flaxmore Fault
In Nelson city, the Flaxmore Fault comprises a zone of imbrication with two major NE-striking SE-dipping fault splays between Jenkins Creek and The Brook. North of the Maitai River, the sub-parallel intra-basement Delaware Fault (with the associated shear zone of the Wakapuaka Phyllonite) is c. 1 km to the south-east, and has been mapped to converge southwestwards to almost the Flaxmore Fault at the mouth of the Maitai valley (). Multiple fault splays along the Flaxmore Fault are also recognised at the southern end of the Moutere Depression (Johnston Citation1990; Rattenbury et al. Citation2006), where distinct fault strands mapped 5–6 km west of the Waimea Fault converge into the Alpine Fault.
The Flaxmore Fault has the largest reverse separation north of the Maitai River (), where Drumduan basement is thrust above the Port Hills Gravel. From north of the Maitai River to the intersection with the sub-orthogonal Grampian Fault the Flaxmore Fault superposes Drumduan or Brook Street basement rocks above Bishopdale Conglomerate. An abrupt along-strike change in separation occurs south of the Grampian Fault, with younger Port Hills Gravel in the hanging wall juxtaposed against older units (Bishopdale Conglomerate and Jenkins Group) in the footwall. The contact of younger over older along the SE-dipping fault is mapped from Jenkins Creek southward to Marsden Valley and Orphanage Creek, consistent with normal slip components along this strand of the Flaxmore Fault ( and ). However, net reverse separation is again apparent south of Orphanage Creek, with superposition of outcropping Port Hills Gravel onto Moutere Gravel and overlying Quaternary gravels. A distinct 1–2 m high escarpment several hundred metres in length can be traced across the Quaternary gravel by LiDAR imagery (Supplemental file S1) and is here interpreted as the southernmost trace of the Flaxmore Fault in the mapped area ().
The major footwall splay of the Flaxmore Fault (West Flaxmore Fault) is mapped between the Maitai River and Jenkins Creek, where Bishopdale Conglomerate is thrust above vertical to overturned units of the Jenkins Group along the south-eastern limb of the Port Hills Syncline ( and A–C). The West Flaxmore Fault joins the main strand of the Flaxmore Fault south of Jenkins Creek.
Persistent activity of the Flaxmore Fault in the Quaternary is compatible with the prominent morphological scarp (A) and aligned springs (Johnston Citation1979). Between Bishopdale and Jenkins Creek the fault dextrally offsets ridge crests by 25–30 m (Johnston Citation1981; Citation1983). Largely continuous Holocene-active strands are documented along the southernmost part of the W-FFS (Rattenbury et al. Citation2006), commencing about at the Wairoa River (3 km south-west of the mapped area). Paleoseismic trenches at Bishopdale indicate a last surface-rupturing event dated at c. 5600 yrs BP, i.e. largely coeval with the youngest event identified for the Waimea Fault (Fraser Citation2005).
The wedge bounded by the Waimea and Flaxmore faults is intersected by two faults with E-W to WNW-ESE strikes (Bishopdale and Grampian faults). The Bishopdale Fault offsets the Port Hills Gravel with both dip-slip (south side down) and dextral strike-slip movements (c. 30 m of ridge crest separation) with paleoseismic evidence of Holocene activity (Bruce Citation1962; Fraser Citation2005; Johnston and Nicol Citation2013). The Grampian Fault down-throws the Port Hills Gravel relative to the Brook Street Terrane. Neither fault appears to truncate the traces of the NE-SW Waimea and Flaxmore Faults.
Tahunanui Fault
The Tahunanui Fault is a newly named NE-SW-striking reverse fault of the W-FFS. The fault truncates and uplifts the north-western flank of the Port Hills Syncline (), but is concealed beneath late Quaternary marine and terrestrial deposits of the coastal plain between Tahunanui and Stoke.
The presence of the fault is required by the geometric superposition of steeply SE-dipping beds of the Magazine Point Formation above Moutere Gravel encountered beneath 20–40 m of late Quaternary gravels by a number of boreholes (http://www.nzgd.org.nz, ). There is no evidence of a Quaternary surface escarpment, but the topographic erosional surface above the hanging wall units is c. 200 m higher than in the footwall.
The location of the available boreholes loosely constrains the fault trace projected to the surface, and its inferred convergence with the Flaxmore Fault south of Stoke () is uncertain.
Folds in the cover sequence
The units of the Jenkins Group, together with the overlying Port Hills Gravel and their basal unconformity are folded into the tight Port Hills Syncline, with a NE-SW axial trace. The north-western limb of the syncline dips at moderate to steep angle to the SE in the hanging wall of the Tahunanui Fault ( and ). The south-eastern limb dips steeply (55°–75°) to the NW, and is locally overturned (dipping c. 50°–80° SE) beneath the West Flaxmore Fault.
Close to the mouth of the Maitai River the Port Hills Gravel and the axial trace of the Port Hills Syncline disappear beneath Quaternary alluvium, with possible truncation by a WNW-ESE fault (), inferred from a topographic scarp. The Port Hills Gravel exposed north of the Maitai River dips gently to the SE, suggesting that the fold axial trace and south-eastern limb are shifted to the north-east and overthrust by Drumduan rocks along the Flaxmore Fault. The SW closure of the core of the Port Hills Syncline with exposure of the older beds of the Jenkins Group is compatible with northward plunge and truncation of the axial trace in the panel bounded by the Tahunanui and West Flaxmore faults.
South of the Grampian Fault, the Port Hills Gravel dips to the SE and is morphologically uplifted relative to the south-eastern flank of the Port Hills Syncline in the hanging wall of the Flaxmore Fault ( and B). The gravel is overthrust by the Marsden Coal Measures and Richmond Group in the hanging wall of the Waimea Fault. In Johnston (Citation1979) the Port Hills Gravel within this panel was mapped as folded within the symmetrical Marsden Syncline. However, the SE dip of beds shows that the outcropping Port Hills Gravel belongs to a wide north-western flank of the syncline, whose axial trace lies further east, very close to – and truncated by – the Waimea Fault. Thus, the NW-dipping limb of the Marsden Syncline is almost entirely truncated and only exposed () north of Jenkins Creek (as inferred from the basal contact of the Port Hills Gravel above the Brook Street Volcanics) and between Marsden Valley and Orphanage streams (as inferred from the change in bed dip to the NW).
Discussion
Structural setting and deformation chronology
The cross sections () provide our interpretation of the geometry of units deformed by the W-FFS. Interpretation is hindered by the lack of subsurface data, eroded sedimentary cover in the uplifted panels, limited exposures of Jenkins Group formations, and the blanket of late Quaternary deposits in central Nelson and from Tahunanui to Hope. However, the surface geology provides important elements for reconstructing the progressive deformation history, as discussed below.
Inherited fault systems
The major faults of the W-FFS juxtapose different basement terranes against one another ( and ), suggesting that they exploit lithological and mechanical contrasts along terrane boundaries. Discrete pulses of extension led to the disruption of the Gondwana convergent margin during late Early Cretaceous and early Late Cretaceous times (from c. 105 to 83 Ma), with establishment of intra-continental rift systems (Adams et al. Citation2017). Continental break-up and seafloor spreading in the Tasman Sea occurred during the Late Cretaceous-Paleocene (c. 80-55 Ma), with development of NE-SW to N-S en échelon grabens infilled by terrestrial to proximal marine sequences (e.g. Paparoa Coal Measures in Westland, Rakopi and North Cape formations of the Pakawau Group in NW Nelson, North Cape Formation in the Manaia sub-basin, cf. Nathan et al. Citation1986; King and Thrasher Citation1996).
Geometry and kinematics of faults along the structural belt comprising the Taranaki, Manaia and Waimea-Flaxmore faults is poorly defined for these stages. The Taranaki Fault is interpreted as exploiting mechanical discontinuities at the western boundary of the Eastern Province (Muir et al. Citation2000; Stagpoole and Nicol Citation2008), but location and geometry of a normal (or oblique-slip) proto Taranaki Fault in the Late Cretaceous remains ambiguous (cf. interpretations of Kamp and Liddell Citation2000; Hill et al. Citation2004; Stagpoole and Nicol Citation2008). In contrast, the thick Late Cretaceous deposits within the Manaia sub-basin provide a strong argument for late-rift normal slip on the Manaia Fault (King and Thrasher Citation1996).
Extensional reactivation of intra-basement weakness zones between the Brook Street and Drumduan terranes (with the interposed Wakapuaka Phyllonite along the Delaware Fault, Johnston Citation1981) may have initiated normal slip along a proto Flaxmore Fault. The 100-90 Ma old Beebys Conglomerate (Momotu Super Group, Adams et al. Citation2017) mapped in the hanging wall of the Flaxmore Fault at the southern end of the Moutere Depression () has been interpreted as evidence for normal slip activity during the late Early Cretaceous (Johnston Citation1990). We speculate that the Bishopdale Conglomerate wedged between the West Flaxmore and Flaxmore faults in Nelson was deposited during extensional fault movements, but the age of the conglomerate remains unknown. The lithology of the Bishopdale Conglomerate and the local provenance of its clasts lead us to consider it a late Early Cretaceous syn-rift deposit (correlative of the Beebys Conglomerate) infilling an isolated terrestrial basin bounded by normal fault escarpments. However, correlation is also possible with younger (80-70 Ma) terrestrial units of the Paparoa and Pakawau groups in Westland, NW Nelson and south Taranaki, deposited along NNE-SSW normal fault escarpments (e.g. Cape Foulwind, Wakamarama faults) that have the same orientation as the Flaxmore Fault.
The Eighty-Eight and Waimea faults are also intra-basement structures bounding different terranes, but their activity as syn– to late-rift normal faults is unclear. The only outcrop of Bishopdale Conglomerate along the Waimea Fault lies within an imbricated thrust wedge (). The Jenkins Fault is perhaps the only preserved branch of a late-rift, proto Waimea normal fault active during deposition of the Eocene Marsden Coal Measures and lately truncated by newly propagating reverse faults in the Cenozoic. Note also that both the Flaxmore and Waimea faults are sub-parallel and dip at moderate to steep angles, consistent with inheritance from sets of earlier normal faults (cf. Sibson Citation1990; Citation2009; Sibson and Ghisetti Citation2018).
Reverse reactivation of inherited normal faults during contractional inversion
Many syn- to late-rift normal faults located within Australian crust were further reactivated as high angle reverse faults since c. 25 Ma, accommodating increased rates of shortening and uplift along the Alpine Fault, with distributed contractional deformation west of the plate boundary (Nathan et al. Citation1986; King and Thrasher Citation1996; Ghisetti et al. Citation2016). Examples documented by surface geology, drilling and seismic profiles include the N-S to NNE-SSW inverted structural highs in the Paparoa and Golden Bay areas (Laird Citation1968; Bishop and Buchanan Citation1995; King and Thrasher Citation1996; Ghisetti and Sibson Citation2006; Ghisetti et al. Citation2014; Citation2016; Reilly et al. Citation2015; Barnes and Ghisetti Citation2016). At the eastern margin of the Taranaki Basin, seismic images across the Manaia Fault and drilling of the inverted Late Cretaceous-Paleocene depocentres in its hanging wall anticlines (Kapuni, Toru, Kupe) provide the best examples of contractional reactivation of normal faults (Voggenreiter Citation1993; King and Thrasher Citation1996) within the structural belt that includes the Taranaki and Manaia faults and the W-FFS (Bull et al. Citation2019).
As discussed above, we favour the interpretation that the faults of the W-FFS are inherited basement structures that were preferentially reactivated with dominant reverse slip since the mid Miocene. There is sparse evidence for small components of dextral horizontal movement for some segments of the W-FFS (Johnston Citation1981; Citation1983). However, analysis of small-scale structural assemblages (Pettinga and Wise Citation1994) does not support significant components of dextral-oblique slip along the fault system.
In the Nelson-Richmond urban area and adjacent Moutere Depression (Ghisetti et al. Citation2018) reverse faulting and folding started after deposition of the Jenkins Group. Shortening and uplift are testified by a sedimentary hiatus at c. 20-10 Ma before onset of terrestrial sedimentation (). Reverse faults may have not precisely followed the same trajectory of the pre-existing normal faults (cf. the cross-cutting relations between the Waimea and Jenkins faults in ), with new reverse faults propagating at shallower, and more favourably oriented, dip angle (Bonini et al. Citation2012; Sibson and Ghisetti Citation2018).
Mechanisms of progressive deformation
Shortening of the Paleogene-Neogene cover sequence exposed in the Nelson-Richmond urban area has occurred by combined folding and reverse faulting. The largest folds are the Port Hills Syncline (footwall of the West Flaxmore Fault) and the partially preserved Marsden Syncline (footwall of the Waimea Fault). The steep to overturned limb of SE-dipping Port Hills Gravel in the Port Hills Syncline and the adjacent SE-dipping limb of the Marsden Syncline must have been connected by a tight anticline, now eroded above the tip of Flaxmore Fault and its splays (A–C). The sub-parallel tectonic fabric between reverse faults and folds, added to the truncation of steeply dipping fold limbs by the reverse faults indicate that folding has accommodated components of crustal shortening within the cover sequence prior to fault propagation.
These relationships are consistent with mechanisms of trishear fault-propagation folding (Erslev Citation1991; Allmendinger Citation1998; Allmendinger and Shaw Citation2000). During trishear fault propagation, slip above the fault tip is transferred into a triangular zone of distributed shear and folding. The amplitude, shape and asymmetry of the fold are primarily controlled by fault dip, trishear angle and propagation-to-slip ratio (P/S). The P/S defines the length of the new fault segment propagating upwards in relation to its accrued incremental slip. Variations in this parameter dictate different fold shapes and are controlled by multiple factors, including mechanical properties of the deformed rock sequence, reactivation of pre-existing faults versus rupture of intact rocks and strain accumulation in the faulted volume (Allmendinger and Shaw Citation2000; Bonanno et al. Citation2017). Low P/S causes localised zones of high strain folding above the pinned fault tip, whereas high P/S leads to unfolded horizons offset by the rapidly upward-propagating fault. The syn-depositional signature of trishear folding above a reverse fault is recorded by sediment thickening in the footwall syncline versus thinning and erosional onlap in the hanging wall anticline, as well as the decreasing amount of displacement from older to younger units.
Reverse reactivation of the inherited, proto Flaxmore normal fault (hosting the syn-rift Bishopdale Conglomerate in its hanging wall), with trishear propagation of the West Flaxmore Fault splay, is consistent with the geometry of the Port Hills Syncline, provided fault propagation across the Jenkins Group occurred with low P/S. Fault propagation continued during deposition of the Port Hills Gravel, resulting in the folding of the mid Miocene unconformity and the localised thick depocentre of Port Hills Gravel in the core of the Port Hills Syncline. We interpret the Flaxmore Fault as another splay of the proto Flaxmore normal fault (), reactivated with reverse slip. However, an earlier history of normal slip on the Flaxmore Fault cannot be proven, because the Bishopdale Conglomerate is not present in its hanging wall and interpreted as either eroded (A,B) or buried beneath younger units (C–E).
The present-day geometry of the West Flaxmore and Flaxmore faults shows that the largest reverse separation occurs north of the Maitai River (), where the two splays merge into one single strand. In contrast, in central Nelson-Stoke there has been a partitioning of slip between the two fault splays, with a likely later propagation of the Flaxmore Fault truncating the West Flaxmore Fault. South of the Grampian Fault, stratigraphic separation along the Flaxmore Fault abruptly changes from reverse to normal (cf. A,B and C–E). The juxtaposition of Port Hills Gravel against Bishopdale Conglomerate along this segment of the Flaxmore Fault can perhaps be explained (Supplemental file S2) by: (i) an original down-to-the-south draping of Port Hills Gravel above the Bishopdale Conglomerate and underlying basement (perhaps above inherited fault escarpments) and, (ii) a decrease in reverse offset along the Flaxmore Fault segment between the Grampian Fault and Orphanage Creek. However, it is also feasible that the superposition of younger over older units results from late gravitative collapse of the south-eastern limb of the anticline uplifted in the hanging wall of the West Flaxmore Fault.
Deformation mechanisms for the Waimea Fault are difficult to assess. The elision of the Marsden Syncline in the footwall and the large components of reverse separation testified by the exposed basement units in the hanging wall are also compatible with trishear growth and propagation, but the resulting geometry requires higher P/S than that of the West Flaxmore Fault. We cannot speculate on the mechanical conditions eventually controlling different P/S for the Waimea and West Flaxmore Faults.
Geometry of the fault system at depth, fault interconnections and seismotectonic implications
The total stripping of cover sequence from the hanging wall of the Eighty-Eight and Waimea faults, the preserved Bishopdale Conglomerate in the hanging wall of the West Flaxmore Fault, and the concealment of the Tahunanui Fault beneath the late Quaternary clastic alluvium may be interpreted in terms of larger amounts of vertical separation along the Waimea Fault (and associated splays) relative to the Flaxmore, West Flaxmore and Tahunanui faults, together with progressive propagation of fault splays towards the NW.
The sub-parallel strike of the faults of the W-FFS, their close spacing, and the presence of obliquely cross-cutting splays suggests that all faults branch from a common basement fault and propagated across the Cenozoic cover sequence during reverse reactivation. Contemporaneous activity of splays rooted into a common, deeper fault is also suggested by the presence of the E-W Bishopdale Fault (Bruce Citation1962; Johnston Citation1979) between the Flaxmore and Waimea faults (). The sub-orthogonal orientation of the Bishopdale Fault relative to the dominant NE-SW structural fabric and its strike-slip components are consistent with its role as a lateral ramp (cf. Fraser Citation2005). The Grampian Fault has perhaps a similar role, but we have no evidence for any strike-slip component along it. Both faults can be interpreted as inherited basement structures, reactivated to accommodate differential shortening of the sedimentary cover during activity of the Waimea and Flaxmore faults at different slip rates.
Reactivation of several interconnected fault splays and eventual late propagation of basement faults beneath the present-day coastal plain has relevant implications for seismotectonic hazard in Nelson and Richmond (). In the urban area, late Quaternary displacements have been documented for the Eighty-Eight, Waimea, Flaxmore and Bishopdale faults, some of which have been connected to earthquake ruptures (Fraser Citation2005; Fraser et al. Citation2006; Johnston and Nicol Citation2013). The morphological scarp interpreted as part of the Flaxmore Fault between Stoke and Richmond (Supplemental file S1) requires further assessment to validate its interpretation as a fault scarp. Late Quaternary activity for the E-W Bishopdale Fault (Johnston and Nicol Citation2013) may be related to contemporaneous reactivation of the Waimea and Flaxmore Fault, as documented by the coeval age of the most recent ruptures for both faults (Fraser Citation2005).
The magnitude of the largest earthquakes on the W-FFS has been derived from estimates of the rupture area (Fraser Citation2005; Fraser et al. Citation2006; Johnston and Nicol Citation2013) and evaluated to be in the range M 6.5-7.4. In the current National Seismic Hazard Model of New Zealand (Stirling et al. Citation2012) the W-FFS is considered to be able to generate M7 earthquakes with average recurrence intervals of c. 6 kyrs.
The rupture length for earthquakes of M∼7 along a reverse fault system should be of several km (Wells and Coppersmith Citation1994; Wesnouski Citation2008). In the Nelson-Richmond area the longest late Quaternary fault scarp preserved along a NE-SW fault has been recognised for a length <3 km along the Eighty-Eight Fault (Johnston and Nicol Citation2013), with a normal (up-slope down) sense of slip. Complex arrays of coseismic surface ruptures have been documented for reverse fault earthquakes (e.g. Philip and Megharoui Citation1983) and the lack of preserved reverse fault scarps in the mapped area can be ascribed to poor exposure and slope instability of the hanging wall. However, it is also feasible that activity along the W-FFS increases southwards, i.e. in proximity of the Alpine Fault ().
Figure 9. Main faults of the Nelson-Richmond urban area. A, Nelson City in relation to the fault-controlled morphological scarps of the Waimea and Flaxmore faults and the projected position of the Tahunanui Fault. B, Richmond in relation to the main strands of the Eighty-Eight, Waimea and Flaxmore faults (triangles on the hanging wall) on the foothills of the Barnicoat Range. The Flaxmore Fault continues to the SW but is concealed beneath alluvial cover in the plain (see ).
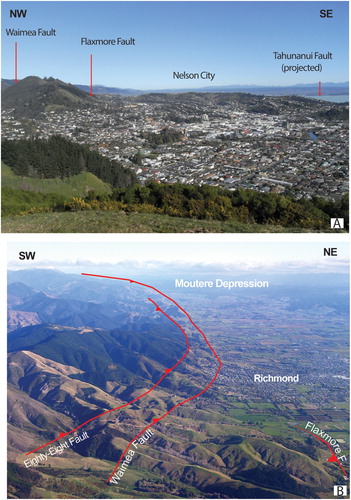
The Waimea and Flaxmore faults display similar orientation and kinematics and – possibly – a similar structural evolution. However, the largest total separation accrued on the Waimea Fault testifies to its preferential reactivation since the Miocene, though not necessarily excluding coeval late stages of slip on the Flaxmore Fault, as well as on the sub-parallel Tahunanui Fault. If this is the case, many splays of the W-FFS sharing similar geometry and kinematics can be reactivated in the present-day stress field, and the lack of known surface ruptures does not necessarily imply inactivity, as was the case of the previously undetected Greendale Fault that ruptured to the surface during the 2010 Darfield earthquake (Quigley et al. Citation2012).
Validation of the interpretation through forward structural models
The interpretation of progressive deformation history outlined in the previous paragraph and the geometric and kinematic consistency of the proposed deformation mechanisms have been tested through forward structural models. shows snapshots of significant deformation stages of the model and the full animated reconstruction with details on the model construction is provided in Supplemental file S3. The model reconstructs accumulation of reverse slip on the Waimea and West Flaxmore faults, considered to be active with different slip rates since the mid Miocene and during sedimentation of the Tadmor Group. The Tahunanui Fault is depicted as a high-angle basement fault (sub-parallel to the major NE-SW faults of the W-FFS) that propagated upwards across the cover sequence post 10 Ma. Age of deposition, relative vertical thickness of the sedimentary succession and chronology of the unconformities are calibrated from surface geology. However, for a clearer depiction of the deformation induced by each fault, the horizontal distance between faults has been exaggerated (see approximate scale in H).
Figure 10. Significant stages of progressive deformation extracted from the forward model (see Supplemental file 3 for the animated forward model and its description). Approximate scale is defined in H, horizontal exaggeration is used to better portray the deformation associated with each fault. A. Structural setting at c. 35 Ma. B, Structural setting at c. 20 Ma. C, D, Progressive stages of contractional inversion of the inherited Waimea and West Flaxmore normal faults between 19-15 Ma. The propagation to slip ratio (P/S) used for the model (see text for details) is indicated by the distance between the triangular dashed lines in the trishear zone (TZ), representing the position of the fault tip before and after propagation. Units eroded in the hanging wall panels of the faults are left blank, with their marker boundaries dashed. E, Structural setting at c. 10 Ma. F, Structural setting at c. 7 Ma. The reverse Tahunanui Fault is inferred to propagate across the cover sequence at this stage. G, Structural setting at c. 3 Ma. H, Structural setting at c. 1 Ma. PHS, Port Hills Syncline; MS, Marsden Syncline. Horizontal shortening (visualised by the hatched rectangles in stages D-H) amounts to c. 17% along the transect.
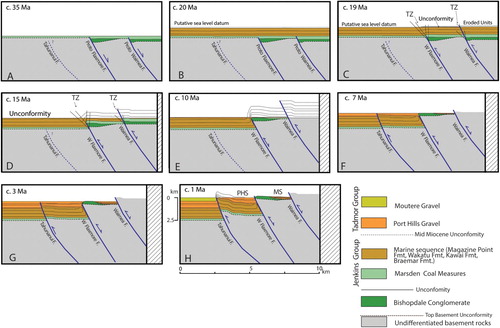
The principal stages of the model are: (1) Initial configuration with an inherited set of late Early Cretaceous normal faults (proto Waimea and West Flaxmore faults) hosting the Bishopdale Conglomerate in their hanging wall, with waning of extensional activity after deposition of the Eocene Marsden Coal Measures at c. 35 Ma (A). (2) Sedimentation of the marine units of the Jenkins Group from c. 34 to 20 Ma. The units are simply depicted with layer-cake configuration, with no deformation (B). (3) Contractional inversion with accumulation of reverse slip on the Waimea and West Flaxmore faults in the time interval c. 19-10 Ma (C–E), associated with uplift and development of an unconformity (mid Miocene hiatus). Fault propagation is modelled using trishear, with high P/S for the Waimea Fault (resulting in no significant folding in the footwall) and low P/S for the West Flaxmore Fault (with the development of the asymmetrical syncline in its footwall). (4) Upward propagation of the Tahunanui Fault during deposition of the Port Hills Gravel coeval with reactivation of the West Flaxmore and Waimea faults in the interval 10-3 Ma (F–G), associated with uplift and truncation of the top of the gravels by an erosional unconformity. The resulting geometry (G) is the gravel depocentre within the Port Hills Syncline and the tilted back-limb of gravels above the anticlinal hinge that exposes the Bishopdale Conglomerate in the hanging wall of the West Flaxmore Fault. (6) Late stage configuration at 1 Ma (H), with all faults modelled as having accrued displacement after deposition of the Moutere Gravel.
Although the model is simplified, it reproduces many features of the cross sections (), with, in particular, the decreasing structural elevation of the hanging wall panels of the Waimea, West Flaxmore and Tahunanui faults, the preserved wedge of Bishopdale Conglomerate in the hanging wall of the West Flaxmore Fault, the vertical to overturned south-easternlimb of the Port Hills Syncline, and the growth accumulation of Port Hills Gravel in its core relative to the uplifted limb of Port Hills Gravel on the north-western flank of the Marsden Syncline.
The present-day structural setting can be replicated with a good fit by the forward structural model. However, as is always the case for modelling, the validation only implies that the chosen initial configuration and proposed kinematic evolution are admissible, but does not necessarily prove their correctness for each deformation step.
Conclusions
The geological map depicting the Waimea-Flaxmore Fault System (W-FFS) in the Nelson-Richmond urban area () updates the maps of Johnston (Citation1979; Citation1981; Citation1982) by incorporating data from new (often ephemeral) outcrops, field revisions, shallow boreholes and trenches, and interpretation of LiDAR imagery.
The geological and structural setting revealed by the new data shows the presence of a continuous strip of Bishopdale Conglomerate in the hanging wall of the West Flaxmore Fault from central Nelson to Jenkins Creek, a disrupted, asymmetric Marsden Syncline in the footwall of the Waimea Fault; a complex system of reverse fault splays along the Flaxmore and Waimea faults, and the concealed Tahunanui Fault, underneath late Quaternary alluvial cover.
Though we can only rely on surface (or near surface) data, the mapped geology and the preservation of the cover sequence in the faulted panels allow the construction of cross sections () that show our interpretation, providing the major geometric and chronological constraints for unravelling the progressive deformation history of the W-FFS.
The major elements of our proposed interpretation are: (1) inherited proto Flaxmore (and, perhaps, Waimea) normal faults hosting depocentres of Bishopdale Conglomerate (late Early Cretaceous?) in the hanging wall; (2) reverse-slip reactivation of the inherited normal faults with mechanisms of trishear fault propagation since 20 Ma (i.e. during regional mid-Miocene hiatus and unconformity), leading to asymmetric folding of the cover sequence; (3) growth faulting of the Waimea and Flaxmore faults and late upward propagation of the Tahunanui Fault during deposition of the Tadmor Group, with accumulation of thicker sequences in the core of the Port Hills Syncline relative to the panel uplifted in the hanging wall of the Flaxmore Fault; (4) larger accumulation of reverse slip on the Waimea Fault relative to the Flaxmore and Tahunanui faults, consistent with the decrease in structural relief from the exposed Waimea and Flaxmore faults to the concealed Tahunanui Fault.
Our inferred structural evolution is admissible and the main tectonic features of the present-day setting can be reproduced by the proposed forward structural model.
The accumulated reverse displacement, similar geometry and close spacing of the Waimea, Flaxmore and Tahunanui faults and their history of coeval activity during and post deposition of the Tadmor Group are all elements consistent with faults of the W-FFS splaying from a deeper, common major fault along terrane boundaries. Holocene activity and earthquake rupture documented for the Eighty-Eight, Waimea and Flaxmore faults show that the W-FFS can be reactivated with dominant reverse mechanisms in the present-day stress field, with possible induced reactivation of transfer structures, like the Bishopdale Fault.
Our reconstruction of the long-term evolution of the W-FFS in the Nelson-Richmond area helps to define modes and timing of progressive deformation in crustal domains west of the Alpine Fault plate boundary, where relatively low strain rate accumulation may result in elusive signature of tectonically active faults.
Acknowledgements
We acknowledge the use of LiDAR data made available by the Nelson City Council and the Tasman District Council. We thank R.H. Sibson for discussions in the field, advice on structural evolution and comments on an early draft of the manuscript. Stephen Read (formerly at the Nelson City Council and now at University of Otago) and Glenn Stevens (Tasman District Council) are acknowledged for discussions on the geologic and geomorphic interpretation. We also thank Nick Mortimer for discussions on the clast provenance in Nelson conglomerates. We acknowledge the careful editorial revisions and constructive comments provided by Tim Little, Dougal Townsend and David Barrell.
Disclosure statement
No potential conflict of interest was reported by the authors.
Additional information
Funding
References
- Adams CJ, Campbell HJ, Mortimer N, Griffin WL. 2017. Perspectives on Cretaceous Gondwana break-up from detrital zircon provenance of southern Zealandia sandstones. Geological Magazine. 154:661–682. doi: 10.1017/S0016756816000285
- Allmendinger RW. 1998. Inverse and forward numerical modelling of trishear fault-propagation folds. Tectonics. 17:640–656. doi: 10.1029/98TC01907
- Allmendinger RW, Shaw JH. 2000. Estimation of fold propagation distance from fold shape: implications for earthquake hazard assessment. Geology. 28:1099–1102. doi: 10.1130/0091-7613(2000)28<1099:EOFPDF>2.0.CO;2
- Alloway BV, Lowe DJ, Barrell DJA, Newnham RM, Almond PC, Augustinus PC, Bertler NAN, Carter L, Litchfield NJ, McGlone MS, et al. 2007. Towards a climate event stratigraphy for New Zealand over the past 30000 years (NZ-INTIMATE project). Journal of Quaternary Science. 22:9–35. doi: 10.1002/jqs.1079
- Anderson H, Beanland S, Blick G, Darby D, Downes G, Haines J, Jackson J, Robinson R, Webb T. 1994. The 1968 May 23 Inangahua, New Zealand earthquake: an integrated geological, geodetic and seismological source model. New Zealand Journal of Geology and Geophysics. 37:59–86. doi: 10.1080/00288306.1994.9514601
- Anderson H, Webb T, Jackson J. 1993. Focal mechanisms of large earthquakes in the south Island of New Zealand: implications for the accommodation of Pacific-Australia plate motion. Geophysical Journal International. 115:1032–1054. doi: 10.1111/j.1365-246X.1993.tb01508.x
- Barnes PM, Ghisetti FC. 2016. Structure, late Quaternary slip rate and earthquake potential of marine reverse faults along the north Westland deformation front, New Zealand. . New Zealand Journal of Geology and Geophysics. 59:157–175. doi: 10.1080/00288306.2015.1112816
- Bishop DJ, Buchanan PG. 1995. Development of structurally inverted basins: a case study from the West Coast, South Island, New Zealand. In: Buchanan JG, Buchanan PG, editors. Basin inversion. London: Geological Society of London Special Publication 88; p. 549–585.
- Bonanno E, Bonini L, Basili R, Toscani G, Seno S. 2017. How do horizontal, frictional discontinuities affect reverse fault-propagation folding? Tectonophysics. 102:147–167.
- Bonini M, Sani F, Antonielli B. 2012. Basin inversion and contractional reactivation of inherited normal faults: A review based on previous and new experimental models. Tectonophysics. 522-523:55–88. doi: 10.1016/j.tecto.2011.11.014
- Bruce JG. 1962. The geology of Nelson City area. Transactions of the Royal Society of New Zealand. Geology. 1:157–181.
- Bull S, Nicol A, Strogen D, Kroeger KF, Seebeck HS. 2019. Tectonic controls on Miocene sedimentation in the Southern Taranaki Basin and implications for New Zealand plate boundary deformation. Basin Research. 31:253–273. doi: 10.1111/bre.12319
- Doser DI, Webb T, Maunder DE. 1999. Source parameters of large historical (1918-1962) earthquakes, south Island, New Zealand. Geophysical Journal International. 139:769–794. doi: 10.1046/j.1365-246x.1999.00986.x
- Eberhart-Phyllips D, Bannister S. 2010. 3-D imaging of Marlborough, New Zealand, subducted plate and strike-slip fault systems. Geophysical Journal International. 182:73–96.
- Eberhart-Phyllips D, Reyners M. 1997. Continental subduction and three-dimensional crustal structure: the northern South Island, New Zealand. Journal of Geophysical Research. 102:11843–11861. doi: 10.1029/96JB03555
- Edbrooke SW. 2017. The geological map of New Zealand: to accompany geological map of New Zealand 1:1,000,000. GNS science geological map 2. 183 p + 2 sheets. Lower Hutt: GNS Science.
- Erslev E. 1991. Trishear fault-propagation folding. Geology. 19:617–620. doi: 10.1130/0091-7613(1991)019<0617:TFPF>2.3.CO;2
- Fraser JG. 2005. Paleoseismic investigation of the Waimea-Flaxmore fault system, Nelson region. [Unpublished M.Sc Thesis]. University of Canterbury, p. 153.
- Fraser JG, Nicol A, Pettinga JR, Johnston MR. 2006. Paleoearthquake investigation of the Waimea-Flaxmore fault system, Nelson, New Zealand. Earthquakes and urban development: NewZealand Geotechnical Society 2006 Symposium; Nelson, New Zealand; February 2006, 31. p. 59–67.
- Ghisetti FC, Barnes P, Sibson R. 2014. Deformation of the top basement unconformity west of the Alpine Fault (south Island, New Zealand): seismotectonic implications. New Zealand Journal of Geology and Geophysics. 57:271–294. doi: 10.1080/00288306.2013.876433
- Ghisetti FC, Johnston MR, Wopereis P, Sibson RH. 2018. Structural and morpho-tectonic evidence of quaternary faulting within the moutere depression, South Island, New Zealand. New Zealand Journal of Geology and Geophysics. 61:461–479. doi: 10.1080/00288306.2018.1502673
- Ghisetti FC, Sibson RH. 2006. Accommodation of compressional inversion in north-western South Island (New Zealand): old faults versus new? Journal of Structural Geology. 28:1994–2010. doi: 10.1016/j.jsg.2006.06.010
- Ghisetti F, Sibson RH, Hamling I. 2016. Deformed Neogene basins, active faulting and topography in Westland: distributed crustal mobility west of the Alpine Fault transpressive plate boundary (South Island New Zealand). Tectonophysics. 693:340–362. doi: 10.1016/j.tecto.2016.03.024
- Henrys S, Wech A, Sutherland R, Stern T. 2013. SAHKE geophysical transect reveals crustal and subduction zone structure at the southern Hikurangi margin, New Zealand. Geochemistry, Geophysics. Geosystems. 14:2063–2083. doi: 10.1002/ggge.20136
- Hill KC, Hoffman N, Channon G, Courteney S, Kendrick RD, Keetley JT. 2004. Structural styles and hydrocarbon traps in the onshore Taranaki Fold Belt, New Zealand. PESA Eastern Australasian Basin Symposium II. Adelaide 19–22 September 2004, 2. p.181–196.
- Johnston MR. 1971. Pre-Hawera geology of the Kaka District, North-West Nelson. New Zealand Journal of Geology and Geophysics. 14:82–102. doi: 10.1080/00288306.1971.10422462
- Johnston MR. 1979. Geology of the Nelson urban area 1:25,000 (1st ed.), New Zealand geological Survey. Urban Series Map 1, Map 1 sheet, notes 52 p. Wellington: Department of Scientific and Industrial Research.
- Johnston MR. 1981. Sheet 027AC. Dun Mountain (1st edition). Geological Map of New Zealand 1:50,000. Wellington: Department of Scientific and Industrial Research.
- Johnston MR. 1982. Sheet N27 (part) Richmond (1st edition). Geological Map of New Zealand 1:50,000. Wellington: Department of Scientific and Industrial Research.
- Johnston MR. 1983. Sheet N28 AC- Motupiko. Geological Map of New Zealand 1:50,000, Map 1 sheet, notes 40 p. Wellington: Department of Scientific and Industrial Research.
- Johnston MR. 1984. Probable upper Mesozoic conglomerate in Nelson city. New Zealand Geological Survey Record. 3:4–7.
- Johnston MR. 1990. Geology of the St Arnaud district, southeast Nelson (Sheet N29AC). New Zealand Geological Survey Bulletin. 99: 119 p.
- Johnston MR, Nicol A. 2013. Assessment of the location and paleoearthquake history of the Waimea-Flaxmore fault system in the Nelson-Richmond area with recommendations to mitigate the hazard arising from fault rupture of the ground surface. GNS Science Consultancy Report. 2013/186. 28 p.
- Johnston MR, Raine JI, Watters WA. 1987. Drumduan Group of East Nelson, plant-bearing Jurassic arc rocks metamorphosed during terrane interaction. Journal of the Royal Society of New Zealand. 17:275–301. doi: 10.1080/03036758.1987.10418162
- Kamp PJJ, Liddell IJ. 2000. Thermochronology of northern Murihiku Terrane, New Zealand, derived from apatite FT analysis. Journal of the Geological Society, London. 157:345–354. doi: 10.1144/jgs.157.2.345
- King PR. 2000. Tectonic reconstructions of New Zealand, 40 Ma to the present. New Zealand Journal of Geology and Geophysics. 43:611–638. doi: 10.1080/00288306.2000.9514913
- King PR, Naish TR, Browne GH, Field BD, Edbrooke SW. 1999. Cretaceous to recent sedimentary patterns in New Zealand. Lower Hutt: Institute of Geological & Nuclear Sciences folio series 1. Institute of Geological & Nuclear Sciences Limited.
- King PR, Thrasher GP. 1996. Cretaceous-Cenozoic geology and petroleum systems of the Taranaki basin, New Zealand. Lower Hutt: Institute of Geological & Nuclear Sciences Monograph 13. 243 p, 6 enclosures. Institute of Geological & Nuclear Sciences Limited.
- Laird MG. 1968. The Paparoa tectonic zone. New Zealand Journal of Geology and Geophysics. 11:435–454. doi: 10.1080/00288306.1968.10423661
- Laird MG. 1995. Coarse-grained lacustrine fan-delta deposits (Pororari Group) of the northwestern South Island, New Zealand: evidence for Mid-Cretaceous rifting. In: A Guy Plint, editor. Sedimentary facies analysis: a tribute to research and teaching of Harold G. Reading: International Association of Sedimentologists. Special Publication; p. 22: 197–217.
- Laird MG, Bradshaw JD. 2004. The break-up of a long-term relationship the Cretaceous separation of New Zealand from Gondwana. Gondwana Research. 7:273–286. doi: 10.1016/S1342-937X(05)70325-7
- Langridge RM, Ries WF, Litchfield NJ, Villamor P, Van Dissen RJ, Barrell DJA, Rattenbury MS, Heron DW, Townsend DB, Lee JA, et al. 2016. The New Zealand active faults database. NZAFD250. New Zealand Journal of Geology and Geophysics. 59:86–96. doi: 10.1080/00288306.2015.1112818
- McClay KR. 1995. The geometries and kinematics of inverted fault systems: a review of analogue model studies. In: Buchanan JG, Buchanan PG, editors. Basin inversion. London: Geological Society of London Special Publication 88; p. 97–118.
- Mildenhall DC, Suggate RP. 1981. Palynology and age of the Tadmor Group (Late Miocene-Pliocene) and Porika formation (early Pleistocene), South Island, New Zealand. New Zealand Journal of Geology and Geophysics. 24:515–528. doi: 10.1080/00288306.1981.10422742
- Mortimer N. 2004. New Zealand’s geological foundations. Gondwana Research. 7:261–272. doi: 10.1016/S1342-937X(05)70324-5
- Mortimer N, Campbell HJ, Tulloch AJ, King PR, Stagpoole VM, Wood RA, Rattenbury MS, Sutherland R, Adams CJ, Collot J, Seton M. 2017. Zealandia: Earth’s hidden continent. GSA Today. 27:27–35. doi: 10.1130/GSATG321A.1
- Mortimer N, Rattenbury MS, King PR, Bland K, Barrell J, Bache DJA, Begg JG, Campbell HJ, Cox SC, Crampton JS, et al. 2014. High-level stratigraphic scheme for New Zealand rocks. New Zealand Journal of Geology and Geophysics. 57:402–419. doi: 10.1080/00288306.2014.946062
- Mortimer N, Tulloch AJ, Ireland TR. 1997. Basement geology of Taranaki and Wanganui basins, New Zealand. New Zealand Journal of Geology and Geophysics. 40:223–236. doi: 10.1080/00288306.1997.9514754
- Mortimer N, Tulloch AJ, Spark RN, Walker NW, Ladley E, Allibone A, Kimbrough DL. 1999. Overview of the Median Batholith, New Zealand: a new interpretation of the geology of the Median tectonic zone and adjacent rocks. Journal of African Earth Sciences. 29:257–268. doi: 10.1016/S0899-5362(99)00095-0
- Muir RJ, Bradshaw JD, Weaver SD, Laird MG. 2000. The influence of basement structure on the evolution of the Taranaki Basin, New Zealand. Journal of the Geological Society. 157:1179–1185. doi: 10.1144/jgs.157.6.1179
- Nathan S, Anderson HJ, Cook RA, Herzer RH, Hoskins RH, Raine JI, Smale D. 1986. Cretaceous and Cenozoic sedimentary basins of the West Coast region, South Island, New Zealand. New Zealand Geological Survey Basin Studies. 1:89.
- Okada T, Iio Y, Matsumoto S, Bannister S, Ohmi S, Horiuchi S, Sato T, Miura T, Pettinga J, Ghisetti F, Sibson RH. 2019. Comparative tomography of reverse-slip and strike-slip seismotectonic provinces in the northern South Island, New Zealand. Tectonophysics. 765:172–186. doi: 10.1016/j.tecto.2019.03.016
- Pettinga JR, Wise DU. 1994. Paleostress adjacent to the Alpine Fault: broader implications from fault analysis near Nelson, South Island, New Zealand. Journal of Geophysical Research: Solid Earth. 99:2727–2736. doi: 10.1029/93JB02900
- Philip H, Megharoui M. 1983. Structural analysis and interpretation of the surface deformations of the El Asnam earthquake of October 10, 1980. Tectonics. 2:17–49. doi: 10.1029/TC002i001p00017
- Quigley M, Van Dissen R, Litchfield N, Villamor P, Duffy B, Barrell D, Furlong K, Stahl T, Bilderback E, Noble D. 2012. Surface rupture during the 2010 Mw 7.1 Darfield (Canterbury) earthquake; implications for fault rupture dynamics and seismic-hazard analysis. Geology. 40:55–58. doi: 10.1130/G32528.1
- Rattenbury MS, Cooper RA, Johnston MR. 1998. Geology of the Nelson area. Institute of geological & nuclear sciences 1:250,000 geological map 9. Lower Hutt: Institute of Geological & Nuclear Sciences. 1 sheet + 67 p.
- Rattenbury MS, Townsend DB, Johnston MR. 2006. Geology of the Kaikoura area. Institute of geological & nuclear sciences 1:250,000 geological map 13. Lower Hutt: Institute of Geological & Nuclear Sciences. 1 sheet + 70 p.
- Reilly C, Nicol A, Walsh JJ, Seebeck H. 2015. Evolution of faulting and plate boundary deformation in the southern Taranaki basin, New Zealand. Tectonophysics. 651–652:1–18. doi: 10.1016/j.tecto.2015.02.009
- Seismograph Service Ltd. 1989. Field area report on a seismic reflection survey conducted in Nelson area, PL 38500 (MD87 lines). Petroleum Corporation of NZ Exploration Ltd. Ministry of Economic Development Crown Minerals, Petroleum Report Series. PR 1488. http://www.nzpam.govt.nz.
- Sibson RH. 1990. Conditions for fault-valve behaviour. In: Knipe RJ, Rutter EH, editors. Deformation mechanisms, Rheology and Tectonics. London: Geological Society of London Special Publication 54; p. 15–28.
- Sibson RH. 2009. Rupturing in overpressured crust during compressional inversion – the case from NE Honshu, Japan. Tectonophysics. 473:404–416. doi: 10.1016/j.tecto.2009.03.016
- Sibson RH, Ghisetti FC. 2018. Factors affecting the assessment of earthquake hazard from compressional inversion structure. Bulletin of the Seismological Society of America. 108:1819–1836. doi: 10.1785/0120170375
- Sibson RH, Ghisetti FC, Crookbain RA. 2012. Andersonian wrench faulting in a regional stress field during the 2010-2011 Canterbury, New Zealand, earthquake sequence. In: Healy D, Butler RWH, Shipton ZK, Sibson RH, editors. Faulting Fracturing and igneous Intrusion in the Earth’s crust. London: Geological Society of London Special Publication 367; p. 7–18.
- Stagpoole V, Nicol A. 2008. Regional structure and kinematic history of a large subduction back thrust: Taranaki Fault, New Zealand. Journal of Geophysical Research. Solid Earth. 113:B01403. doi:10.1029/2007JB005170.
- Stern TA, Davey FJ. 1990. Deep seismic expression of a foreland basin: Taranaki Basin, New Zealand. Geology. 18:979–982. doi: 10.1130/0091-7613(1990)018<0979:DSEOAF>2.3.CO;2
- Stirling M, McVerry G, Gerstenberger M, Litchfield N, Van Dissen R, Berryman K, Barnes P, Wallace L, Villamor P, Langridge R, et al. 2012. National seismic hazard model for New Zealand: 2010 update. Bulletin of the Seismological Society of America. 102:1514–1542. doi: 10.1785/0120110170
- Suggate RP. 1965. Late Pleistocene geology of the northern part of the south Island. New Zealand Geological Survey Bulletin. 77:91p.
- Sutherland R. 1999. Basement geology and tectonic development of the greater New Zealand region: an interpretation from regional magnetic data. Tectonophysics. 308:341–362. doi: 10.1016/S0040-1951(99)00108-0
- Tasman Petroleum Corporation Ltd. 1966. Ruby Bay. Ministry of Economic Development Crown Minerals, Petroleum Report Series. PR 599. http://www.nzpam.govt.nz.
- Townend J, Sherburn S, Arnold R, Boese C, Woods L. 2012. Three-dimensional variations in present-day tectonic stress along the Australia-Pacific plate boundary in New Zealand. Earth and Planetary Science Letters. 353-354:47–59. doi: 10.1016/j.epsl.2012.08.003
- Voggenreiter WR. 1993. Structure and evolution of the Kapuni Anticline, Taranaki Basin, New Zealand: evidence from the Kapuni 3D seismic survey. New Zealand Journal of Geology and Geophysics. 36:77–94. doi: 10.1080/00288306.1993.9514556
- Wallace LM, Barnes P, Beavan J, Van Dissen R, Litchfield N, Mountjoy J, Langridge G, Lamarche G, Pondard N. 2012. The kinematics of a transition from subduction to strike-slip: an example from the central New Zealand plate boundary. Journal of Geophysical Research. 117:B02405. doi: 10.1029/2011JB008640.
- Wellman HW. 1971. Age of the Alpine Fault, New Zealand. Proceedings of the 22nd International Geological Congress (India 1965). 4. p. 148–162.
- Wells DL, Coppersmith KJ. 1994. New empirical relationships among magnitude, rupture length, rupture width, rupture area and surface displacement. Bulletin of the Seismological Society of America. 84:974–1002.
- Wesnouski SG. 2008. Displacement and geometric characteristics of earthquake surface ruptures: issues and implications for seismic-hazard analysis and the process of earthquake rupture. Bulletin of the Seismological Society of America. 98:1609–1632. doi: 10.1785/0120070111
- Williams CA, Eberhart-Phillips D, Bannister S, Barker DHN, Henrys S, Reyners M, Sutherland R. 2013. Revised interface geometry for the Hikurangi subduction zone, New Zealand. Seismological Research Letters. 84:1066–1073. doi: 10.1785/0220130035
- Wopereis P. 2011. Waimea-Flaxmore Fault system and geohazards in Nelson. In: JM Lee, editor. Field Trip Guides. Geosciences 2011 Conference. Nelson: Geoscience Society of New Zealand Miscellaneous publication 130B. p. 19.