ABSTRACT
In the following paper, we apply digital mapping techniques and tephrostratigraphy to correlate marker horizons across the Whanganui Basin, culminating in a new 1:25,000 geological map of the central Rangitikei. This is achieved by (1) compilation of existing research, (2) correlation of major element glass shard datasets to known eruptive products and (3) completion of a three-year field survey. We update and refine existing stratigraphic correlations, focusing on two critical units within the basin succession; namely the Makirikiri Tuff (1.6 Ma) and Kaimatira Pumice Sand (1 Ma) formations. Okehu Group is proposed to cover all strata from Pakihikura to Potaka pumice. Rewa Pumice is placed within its own formation bridging the Makirikiri Tuff and Kaimatira Pumice Sand formations. Mapping and analysis facilitate placement of Coutts Creek Horizon within Rewa Formation. Sub-aerial surfaces and influxes of greywacke and volcaniclastic detritus from c. 1.6 Ma onward provide evidence for an alluvial plain developing at the foot of the uplifting, paleo-axial range. We suggest uplift in the paleo-axial range occurred contemporaneously with the onset of rhyolitic activity at Mangakino caldera (c. 1.6 Ma) so that the first influxes of fluvial conglomerate and volcaniclastics occur in close proximity within the eastern basin succession.
Introduction
Tephrochronology and tephrostratigraphy are powerful tools that have been applied since the late nineteenth century to date and correlate New Zealand geological successions (Lowe Citation1990). A focus of central and lower North Island research has been the Taupo Volcanic Zone (TVZ), one of the most frequently active rhyolitic centres on Earth, with eruptions that have dispersed tephra for thousands of kilometres from source (Wilson and Rowland Citation2016). South of the TVZ lies the Late Miocene-Quaternary, Whanganui Basin, providing an important sedimentary archive of volcanic history (). Plio-Pleistocene sedimentation broadly matched subsidence in the Whanganui Basin, leading to the preservation of a multitude of volcanic products related to the initial development and maturation of the TVZ.
Figure 1. Map of the North Island showing the study area in relation to the Whanganui Basin (WB), Auckland Volcanic Zone (AVZ), Coromandel Volcanic Zone (CVZ), Central Volcanic Region (CVR), Taupo Volcanic Zone (TVZ), main axial range, Whanganui City, Makirikiri Valley (MK), Kaimatira Bluff (KB), Foxton township, Palmerston North and major river systems. Red outlines represent the eight major calderas within the TVZ: 1 = Taupo, 2 = Whakamaru, 3 = Ohakuri, 4 = Mangakino, 5 = Reporoa, 6 = Kapenga, 7 = Rotorua, 8 = Okataina (Wilson and Rowland Citation2016). TgVC = Tongariro Volcanic Centre, EgVC = Egmont Volcanic Centre, TRL = Taranaki Ruapehu, NIDFB = North Island Dextral Fault Belt. Black arrows indicate Pliocene shortening and extension (Nicol et al. Citation2007). Black lines indicate faults.
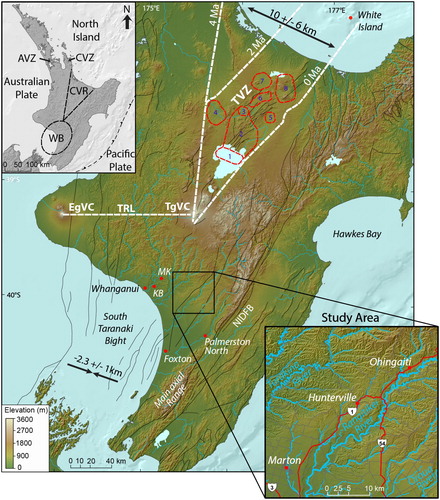
The chronostratigraphic framework of the Whanganui Basin is based on biostratigraphy (Beu Citation2006), tephrostratigraphy (Pillans et al. Citation2005) and magnetostratigraphy (Turner et al. Citation2005). Mapping of the basin fill involves detailed description of key sections and recognition of marker horizons. The most recent maps covering the basin are the Taranaki and Hawkes Bay QMAP sheets at 1:250,000 (Townsend et al. Citation2008; Lee et al. Citation2011), and isolated areas covered at smaller scales (Milne Citation1968; Carter Citation1972; Naish and Kamp Citation1995; Journeaux et al. Citation1996; van der Neut Citation1996; McIntyre and Kamp Citation1998; Rees et al. Citation2018b). Currently, there is a need to update the available maps in the basin. We address this by compiling and formally defining stratigraphic nomenclature in the Whanganui Basin, resolving historic miscorrelations and applying tephrostratigraphy to produce a new map of the central Rangitikei. Sections from the Oroua (Beaconsfield Valley), Rangitikei (Pourewa Stream) and Turakina (Coutts Creek) catchments are presented alongside new geochemical datasets. We investigate spatio-temporal patterns of sedimentation within the basin succession, exploring evidence for the palaeogeographic development of the lower North Island.
Nomenclature
Volcaniclastic units are common in the Whanganui Basin succession and we follow recommendations by Schmid (Citation1981) and Froggatt and Lowe (Citation1990) in our terminology, describing epiclastic sediments dominated by volcanic detritus as ‘tephric’. The original stratigraphic names ‘Potaka Pumice’ and ‘Pakihikura Pumice’, as set out by Te Punga (Citation1952), are retained here in preference to other terms used to encompass a variety of reworked tephric silts, sands and pumice. The name ‘Makirikiri Tuff Formation’ of Fleming (Citation1953) is adopted here to retain historic precedence.
Mapping the Whanganui Basin
Geological mapping of the Whanganui Basin in the mid-twentieth century by geologists of the New Zealand Superior Oil Company and New Zealand Geological Survey quickly made use of conspicuous volcaniclastic marker horizons, tracing their extent across a large portion of the basin. The literature and related nomenclature introduced during this time is still used as the basis for stratigraphic subdivision today, including early recognition of the role of eustatic sea level fluctuations (Fleming Citation1953), identification of important regional structures (Feldmeyer et al. Citation1943; Te Punga Citation1957) and development of a basin history (Te Punga Citation1952; Fleming Citation1953).
Advances in the application of radiometric (Milne Citation1973b; Marden and Neall Citation1990; Pillans et al. Citation1993), palaeomagnetic (Seward and Lienert Citation1986; McGuirre Citation1989; Turner and Kamp Citation1990), fission track (Milne Citation1973a; Seward Citation1974a; Boellstorff and Te Punga Citation1977; Seward Citation1979; Pillans and Kohn Citation1981) and isotopic (Seward Citation1978; Stevens and Vella Citation1981) dating techniques came to the forefront of Whanganui Basin research as technology became more widely available toward the end of the twentieth century. This led to the ongoing development and refinement of a basin wide chronostratigraphic framework, culminating in the correlation of the Whanganui Basin succession to the high resolution, astronomically tuned δ18O record (Naish et al. Citation1998).
Initial work using fission track dating on volcanic glass (Seward Citation1974a, Citation1976; Boellstorff and Te Punga Citation1977) underestimated ages because the method didn’t account for partial track annealing (Naish et al. Citation1996). Alloway et al. (Citation1993) used the isothermal plateau fission track (ITPFT) technique to correct for track annealing thereby synchronising it with revised magnetostratigraphic records (Pillans et al. Citation1994), 40Ar/39Ar dating (Shane et al. Citation1992) and orbitally tuned cyclostratigraphy (Naish et al. Citation1998) to provide refined glass ages in the Whanganui Basin. Studies of recognised tephra-fall and/or volcaniclastic horizons within the Whanganui Basin were presented by Naish et al. (Citation1996), Shane et al. (Citation1996) and Pillans et al. (Citation2005). We further develop this work by incorporating marker horizons into a formal stratigraphic nomenclature and augmenting existing geochemical datasets through the addition of new data from less frequented sections.
Geological setting
Whanganui Basin
The study area is located within the Whanganui Basin c. 200 km behind the Hikurangi subduction zone (). During Miocene to early Pleistocene time, the southern North Island was characterised by a series of marine basins connected by seaways across a shallow basement high in the location of the present day main axial range (Beu Citation1995). Sediments derived from the Central Volcanic Region, Torlesse Composite Terrane of the paleo-axial ranges and South Island granites and schist were transported into peripheral sedimentary basins, where they were deposited throughout climatically forced Quaternary eustatic sea level fluctuations. Up to 4.5 km of basin fill has been preserved within the Whanganui Basin, including coarse-grained transgressive (TST) and fined-grained highstand (HST) deposits separated by sequence bounding unconformities. Consequent uplift along the northern and eastern basin margins has resulted in shore-normal tilting of the Late Miocene–Pleistocene units.
The revised stratigraphic nomenclature of the Whanganui Basin and its relationship to previous work is summarised in . The basin succession varies from east to west, characterised by changes in the style of deposition and extent of preservation. The best outcrop occurs at with coastal exposures NW of Whanganui () and down NE-SW oriented river valleys draining the central North Island. Each major river valley is characterised by locally specific members and formations. The recognition of key marker horizons facilitates basin wide correlations and the definition of groups. At the Whanganui type section, a prominent 450 ka unconformity occurs at the base of the Butlers Shell Conglomerate, Okehu Group. East of Whanganui the unconformity is not present, at least to the same extent, replaced by 150 + m of marginal to non-marine beds of the Makirikiri Tuff Formation (). The Okehu Group, east of Whanganui is difficult to interpret in terms of sequence stratigraphy due to its marginal to non-marine cycle motifs (Naish et al. Citation2005), including preservation of lowstand systems tracts (LST), conglomerates and sub-aerial surfaces, such as paleosols and lignite.
Table 1. Proposed stratigraphic nomenclature used in this study (far right) and correlation to other proposed nomenclatures of the eastern and western Whanganui Basin.
The Central Volcanic Region and Taupo Volcanic Zone
The Central Volcanic Region (CRV) () is characterised by andesite volcanoes, rhyolitic calderas and negative gravity anomalies resulting from the production of c. 12,000 km3 of Plio-Pleistocene siliceous volcanics (Gravley et al. Citation2016). Active rhyolitic volcanism within the CVR began in the Coromandel Volcanic Zone (CVZ) from 12 to 14 Ma (Carter et al. Citation2003). By c. 2 Ma volcanism had migrated southeast to the younger TVZ, reflecting the evolution of an arc-related magmatic province (Wilson and Rowland Citation2016). Onland records of late Cenozoic volcanism are incomplete due to tectonism, erosion and burial, especially within the TVZ where widespread Whakamaru ignimbrite (c. 350 ka) often obscures evidence for older activity (Downs et al. Citation2014b). Mangakino caldera () is the westernmost and oldest rhyolitic caldera in the TVZ, active during two periods of caldera forming activity from 1.68–1.53 and 1.21–0.95 Ma, respectively (Krippner et al. Citation1998). Voluminous widespread welded and non-welded ignimbrites and fall deposits originating from the Mangakino Volcanic Centre provided an important source of sediment to the Whanganui Basin during Castlecliffian time ().
Table 2. Selected tephra-fall and/or volcaniclastic deposits and their respective stratigraphic units from the Rangitikei. Ages derived from Alloway et al. (Citation2004), Pillans et al. (Citation1994), Naish et al. (Citation1996), Shane et al. (Citation1996), Seward and Kohn (Citation1997), Naish et al. (Citation1998), McIntyre (Citation2002), Pillans et al. (Citation2005) and Grant et al. (Citation2018).
Correlation between Whanganui Basin volcaniclastic horizons and their corresponding proximal source deposits is problematic due to limited exposure, partial welding and extensive vapour-phase alteration of these ignimbrite sheets. Pakihikura Pumice is chronologically equivalent to Ngaroma Ignimbrite and ignimbrite Unit-B from the Mangakino Volcanic Centre, erupted during an early phase of rhyolitic activity within the TVZ (Wilson et al. Citation2009). Potaka Pumice (c. 1 Ma), base of the Kaimatira Pumice Sand Formation (Fleming Citation1953), is correlated to the Rocky Hill and Kidnappers ignimbrites (Cooper et al. Citation2017), also originating from the Mangakino Volcanic Centre.
Methods
In this study, we compiled a 1 : 25,000 geological map of the central Rangitikei (). Ortho-rectified aerial photograph datasets from LINZ and digital elevation data acquired from Horizons Regional Council and Columbus et al. (Citation2011) were used to aid mapping within a Geographic Information System (GIS) environment. Database design was based on the structure established by the GNS Science QMAP (Rattenbury and Heron Citation1997). We placed particular emphasis on linking existing works (Feldmeyer et al. Citation1943; Te Punga Citation1952; Fleming Citation1953; Bussell Citation1984; Potter Citation1984; Naish and Kamp Citation1995; van der Neut Citation1996) into a single nomenclature under the umbrella of stratigraphic groups laid down by Fleming (Citation1953), with type sections near Whanganui (; ). Mapping was assisted by the detailed stratigraphic studies of Seward (Citation1974b), Abbott (Citation1994), Naish and Kamp (Citation1995) and Pillans et al. (Citation2005). Key marker horizons were recognised in the field and traced laterally across the landscape. Prominent horizons in the landscape and/or section were selected as formation and group boundaries within the proposed stratigraphic framework (). We logged and sampled sections within the tributaries and interfluves of the Turakina, Rangitikei and Oroua catchments (Supplementary Data File A). An effort was made to visit type localities of important marker units and trace them laterally over interfluves into adjoining tributaries, in order to gain an understanding of facies changes and variability across the basin through time.
Figure 2. Geological map of the study area showing lithological groups, regional structures and the location of the Potaka Pumice and Pakihikura Pumice; two widespread regional marker horizons used to define the base of the Kai Iwi and Okehu Groups respectively. Sample points are shown from Pillans et al. (Citation2005) and this study, state highways are shown in grey. This work draws from the geological maps of Feldmeyer et al. (Citation1943), Te Punga (Citation1952), Fleming (Citation1953), Milne (Citation1973b), Naish and Kamp (Citation1995), van der Neut (Citation1996), Townsend et al. (Citation2008), Lee et al. (Citation2011).
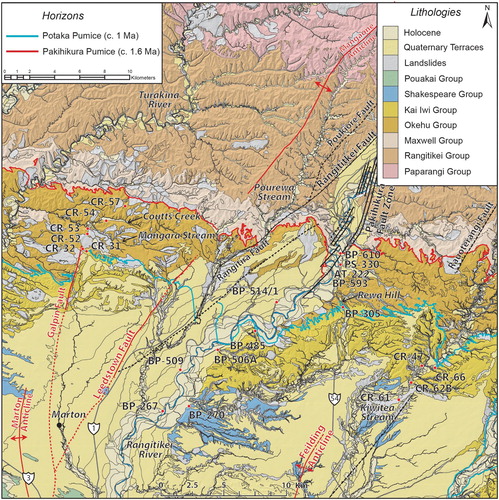
Volcaniclastic and primary tephra-fall horizons were sampled at sections, targeting beds composed entirely of glass or alternatively picking pumice clasts from volcaniclastic deposits. Pumice clasts were cleaned and gently crushed during sample preparation. The glass was washed through a 63 μm sieve, discarding silt and clay sized particles. After drying samples were sieved; the 125–250 μm fraction was collected and set in epoxy plugs, ground and finally polished using 1 μm diamond paste. Glass-shard major elements were determined using a JEOL JXA-8230 Electron Probe Microanalyser (EPMA) at Victoria University of Wellington. Analyses were determined using an 8 nA beam current, 10 μm beam diameter. Matrix-matched glass standards ATHO-G from the MPI-DING collection (Jochum et al. Citation2006) and VG568 (Jarosewich et al. Citation1980) were used and run every 10 samples to monitor instrumental drift. Accuracy of the analyses was within 3.5% of published values, apart from Ti and Cl that had offsets of 26.94% and 68.88%, respectively. Large offsets for Ti and Cl are attributed to the low wt% (<0.35 wt%) within the chosen standards. Ti and Cl were not used for the basis of tephrostratigraphic correlation. All analyses are presented as weight percent, recalculated to 100% on a water-free basis (, Supplementary Data File B). Total Fe (FeOt) was calculated as FeO while the analytical sum prior to recalculation on an anhydrous basis is the difference from 100%. Bivariate plots were constructed to aid tephra correlation with existing published datasets ().
Figure 3. Composition of glass shards from six tephra-fall and/or volcaniclastic deposits in the central Rangitikei and correlation to the published datasets of Pillans et al. (Citation2005). A, Mean CaO versus FeOt (wt%), B, Mean K2O versus SiO2 (wt%), C, Mean K2O versus CaO (wt%), D, CaO versus FeOt (wt%) composition of glass shards from this study including mean analyses with error bars representing one standard deviation, E, Mean K2O versus CaO (wt%) for Coutts Creek horizon (CR-54) and correlation to the datasets of Pillans et al. (Citation2005). Note some overlap in major element, glass shard geochemistry occurs between the different deposits. Correlations are based on stratigraphic position, EPMA data and geological mapping.
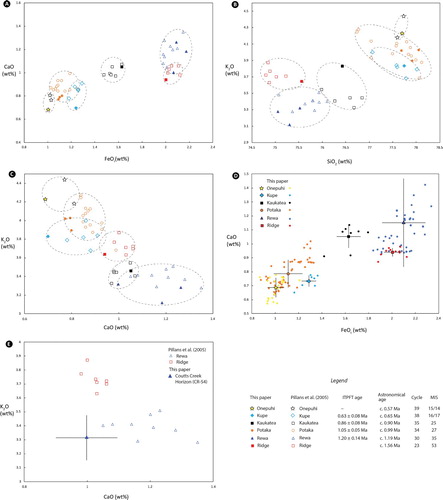
Table 3. Electron Probe Microanalyser data for glass shards from the study area, Whanganui Basin.
Results
Geological map of the central Rangitikei
The geology of the study area has been mapped at 1:25,000 (, Supplementary Data File C). This survey resulted in the amalgamation of several formal and informal stratigraphic nomenclatures introduced in the literature over the last 75 years (). Pakihikura Pumice (, and ) marks the first widespread influx of volcaniclastic detritus into the basin at c. 1.6 Ma. Potaka Pumice represents the second major influx of volcaniclastic detritus into the basin at c. 1 Ma. Both units are prominent in section (A, D and E) and represent important marker horizons within the basin succession.
Figure 4. A, Pakihikura Pumice exposed at a cutting on Turakina Valley Road (BL33 0135 7932). B, Toms conglomerate in the Pourewa Stream section (BL34 0997 6438). C, Waitapu Shell Conglomerate, a typical cross-bedded shell conglomerate within the Rangitikei succession (BL34 1595 6589). D, Potaka Pumice exposed on farm track, western side of Rangitikei Valley (BL34 1586 6860). E, Potaka Pumice exposed in Kiwitea Stream (BL35 3296 6451). F, Mangapipi Tephra enclosed by lignite near Mangapipi Stream on the eastern side of the Rangitikei River (BL34 2539 7275).
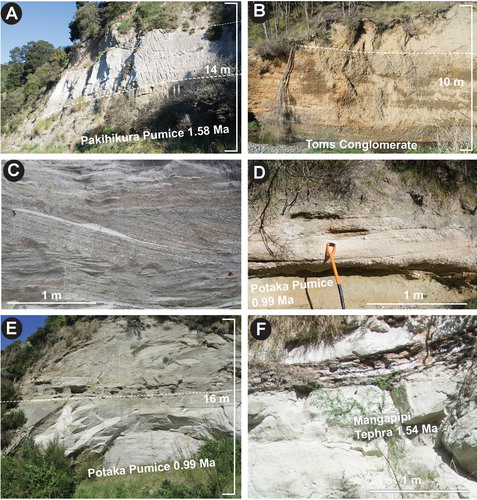
Geological structures have been identified, including the Poukiore Fault () and a southern extension of the Mangaone Anticline (Feldmeyer et al. Citation1943). Major regional reverse faults, the Rangitikei and Rauoterangi faults with maximum-recorded throws of 182 and 293 m, upthrown west, respectively, drive deformation of the Plio-Pleistocene succession, associated with zones of antithetic, normal faulting (Pakihikura and Poukiore faults). In the south of the mapping area, the actively growing Marton and Feilding anticlines are related to faulting at depth (Melhuish et al. Citation1996). Surface features (scarps), stratigraphy and structure contours have been used to map fault locations during the survey. Both sense and throw vary considerably along the length of these faults. They are commonly en echelon, associated with scissor movement and minor antithetic normal faulting. Folds are dome shaped, plunge southward and are often observed to change laterally. Where the underlying faults break to the surface, they offset rather than fold strata. Conversely, faults are also observed to pass laterally into fold structures. No major offsets were noted across the southern end of the Rauoterangi Fault, although a change in dip direction from SW to SE in this area suggests folding of the strata has occurred. Deformation is likely related to the Rauoterangi Fault as it plunges south beneath the Pleistocene strata, with offset accommodated as folding.
The distribution of the Pouakai to Paparangi groups (c. 3–0.3 Ma) is related to the depositional history, stratigraphic architecture and tectonic regime of the Whanganui Basin through the Plio-Pleistocene. The general east–west distribution of geological units reflects the gentle shore-normal tilt (2–11°) of the succession toward the basin depocentre, located offshore. Quaternary river terraces represent climatically controlled aggradational episodes preserved by ongoing regional uplift over the last c. 370 ka (Pillans Citation1994). The terraces fan out in the lower Rangitikei Valley where the river emerges from its confined middle reaches onto the coastal plain. Holocene valley fill is restricted to the main valleys where the rivers have incised into the Plio-Pleistocene marine succession.
Marine terraces are present in the southern portion of the study area, occurring broadly parallel to and c. 30 km inland from the modern coastline. Pillans (Citation1983) mapped and named 12 terraces along the southern Taranaki coastline northwest of Whanganui (), developing a terrace chronology and model of terrace deformation. Each marine terrace comprises a wave cut surface with a fossil sea cliff at its inner margin, representing the paleo-shoreline at maximum transgression during past warm climate episodes. Marine sediments directly overlie the wave cut surface, followed by a range of non-marine coverbed deposits typically comprising loess, dune sand and tephra (Palmer and Pillans Citation1996). Terrace chronology relies on fission track dating of the c. 340 ka Rangitawa Tephra (Alloway et al. Citation1993). Loess stratigraphy of coverbed deposits facilitates dating and correlation of landforms. Loess is associated with aggrading river flood plains and the production of abundant fine sediment during Late Quaternary stadial and glacial periods. Interstadial and interglacial periods are associated with landscape stabilisation and soil development, represented by paleosols within the coverbed succession.
Stratigraphy
The Pleistocene succession of the study area () was deposited toward the eastern basin margin as the sedimentary depocentre migrated from Turakina Valley to its present position offshore of Foxton (). Alternations between coarse-grained (TST) and fine-grained (HST) sedimentary units represent climatically forced changes in water depth of approximately 120 m (Nicol Citation2011). The identification of these textural couplets allows for cycle matching between key sections (Abbott Citation1994), whereby packages of sediment are grouped and ordered into cycles based on sequence stratigraphic concepts. The number of cycles above or below a known marker horizon can then be used to position oneself within the succession at outcrop.
Figure 5. Pleistocene stratigraphic correlations in the Rangitikei. Rangitikei composite data from Pillans et al. (Citation2005), Beaconsfield Valley composite, Pourewa Stream composite and Coutts Creek composite data from this study.
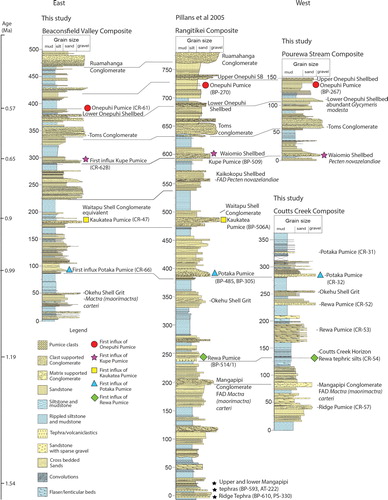
The Whanganui Basin Pleistocene succession consists of cross-bedded pebbly shell conglomerates (Type A shellbeds) (C), heterolithic, often pumiceous and pebbly transgressive units and massive to laminated highstand siltstones with sparse, in situ fossils (Type B shellbeds) (Abbott Citation1994). Overall, the succession coarsens upwards, becoming increasingly dominated by sandstone and conglomerate units. Shellbeds generally form a minor component of the dominantly siliciclastic succession.
Tephrostratigraphy in the Whanganui Basin is based on the first influx of a new glass chemistry within the succession. These changes in provenance have been coupled with biostratigraphic datums to facilitate correlation across the mapping area (). Here, we observe some dramatic changes in sedimentation over relatively short distances (<10 km) within the basin. Particularly pronounced, is the influx of abundant greywacke gravels within the sedimentary record, occurring earlier within the succession and at increasing frequency toward the east. In contrast, the relative concentration of bioclastic material within the succession systematically reduces eastward. For instance, the entire Kai Iwi Group (Potaka Pumice to Toms Conglomerate) is devoid of shell material within Beaconsfield Valley (Oroua Catchment), where we have relied upon tephrostratigraphy and lithostratigraphy to identify tie points and equivalent units.
Conglomerate deposits () occur shortly after deposition of Kaukatea Pumice (0.9 Ma) within Beaconsfield Valley (Rees et al. Citation2019). These conglomerates are clast supported, contain sub-angular to sub-rounded, pebble to cobbled sized clasts of greywacke with intercalated siltstone and sandstone lenses. Low angle cross-bedding with associated channel cut and fill is present together with reverse graded beds. Abundant rip up clasts of siltstone up to 500 mm in diameter occur, associated with scouring and erosion of underlying beds. The first occurrence of a similar conglomerate within the adjacent Rangitikei section c. 10 km west is Toms Conglomerate (B), occurring between the Kupe (0.65 Ma) and Onepuhi (0.57 Ma) pumices. These conglomerates are completely devoid of shell material and contain large clasts (up to 500 mm), being similar in nature to the Late Quaternary terrace deposits of the Rangitikei and Manawatu districts (Milne Citation1973b) although distinguishable by their stratigraphic position, dip angle and degree of weathering ().
Figure 6. A, 25 m thick, clast supported conglomerate. Clasts are sub-rounded pebbles to cobbles of dominantly greywacke. Common channel cut and fill, rip up clasts and interbedded lenses of siltstone. Occurs between Kaukatea (0.9 Ma) and Kupe (0.65) pumice in Beaconsfield Valley (BL35 3194 6425). B, Sketch to illustrate structures within the conglomerate.
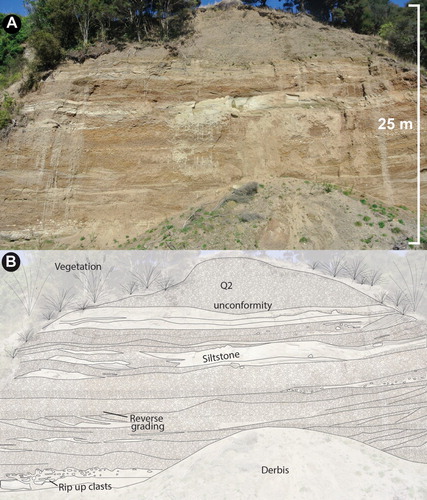
Tephrostratigraphy
The primary tephra-fall and reworked volcaniclastic deposits sampled and analysed during this study are presented in and Supplementary Data file B. Glass shards were analysed for nine major elements by electron probe microanalysis (EPMA). Major oxide chemistry demonstrates ranges of SiO2 of between 75 and 78 wt%, placing the samples within the rhyolite classification of Le Maitre (Citation1984). The samples have been correlated to geochemical datasets of known eruptive products collected from the Whanganui Basin (Pillans et al. Citation2005) by distinctive changes in major element oxide composition of glass shards, in particular, CaO, K2O and FeOt ().
Discussion
Kaimatira Pumice Sand Formation – Potaka Pumice
Fleming (Citation1953) defined the Kaimatira Pumice Sand Formation, mapping it eastward from its designated type section at Kaimatira Bluff in Whanganui to the Turakina catchment (). Fleming correlated the Kaimatira Pumice Sand Formation to the Coutts Creek Horizon of Feldmeyer et al. (Citation1943), noting it outcropping c. 90 m above the Makirikiri Tuff Formation on the southern ridge of Makirikiri Valley (). Fleming (Citation1953) notes the thickness of the formation increases toward the east and that distinguishing individual formations east of the Whangehu Valley is inhibited by extensive landslides and lateral facies variation.
Te Punga (Citation1952) defined the Potaka Pumice in his geological survey of the Rangitikei District. Describing a strongly current-bedded 18 m thick pumice unit with clasts between 50 and 180 mm in diameter, clearly exposed in the cliffs of the Rangitikei, north of the confluence with Waitapu Stream (: BP 485).
Seward (Citation1976) correlated the Potaka Pumice of Te Punga (Citation1952) to the Kaimatira Pumice Sand Formation. Documenting similar ferromagnesian mineral assemblages and chemistry between samples at the type section of Potaka Pumice on Rewa Hill () and the type section of the Kaimatira Pumice Sand Formation at Kaimatira Bluff (). Originally, both Rewa Pumice and Potaka Pumice were included within the Kaimatira Pumice Sand Formation (Seward Citation1976). However, following additional geochemical analysis of the Kaimatira Bluff and Rewa Hill type sections (Pillans et al. Citation2005) it was found that Rewa Pumice is absent from the Kaimatira Pumice Sand Formation type section in Whanganui, resulting in a necessary subdivision. The Kaimatira Pumice Sand Formation is now defined by the first influx of Potaka Pumice into the Whanganui Basin succession ().
We sampled Potaka Pumice at the Coutts Creek and Beaconsifield Valley sections ( and ), mapping the horizon between the Turakina (Deepdem Conglomerate of van der Neut Citation1996), Rangitikei and Oroua sections (). Considerable effort was placed on finding the first influx of Potaka glass chemistry at the Coutts Creek locality to establish group and formation boundaries within this area. The localities on Taurimu Road (: CR-32) described by Hellstrom (Citation1993) and van der Neut (Citation1996) are supported by our work as containing the first influx of Potaka Pumice. Substantial landslides off the scarp slope above Mangara Stream () where the terrace country suddenly gives way to broken hill country need to be treated with caution. Rock rotational slides of Kaimatira Pumice Sand frequent the base of this scarp slope. However, logging and sampling of insitu material along Ngaruru Road (: CR-52, CR-53) indicates the area is underlain by Rewa Formation (, Coutts Creek Composite).
Shane (Citation1991) and Rees et al. (Citation2018b) have documented Potaka Pumice in the Manawatu and Pohangina valleys, enabling correlation of local stratigraphic units to the Kaimatira Pumice Sand Formation of Fleming (Citation1953). Potaka Pumice has also been found cropping out across the Pohangina-Oroua interfluve (Brackley Citation1999), where it is able to be traced as a mappable unit across the Pohangina Anticline westward to the Oroua River. This horizon correlates to the Kimbolton Ash horizon mapped by Feldmeyer et al. (Citation1943). Here we adopt Seward’s (Citation1976) correlation of the Kimbolton Ash to Potaka Pumice without emendation. We note substantial lateral facies variation within the Potaka Pumice moving east from the Turakina toward the Oroua Valley, including a marked increase in the concentration of glass, thickness of the basal unit (up to 10 m) and the prominence it attains in the landscape (E).
Although Seward’s (Citation1976) initial ages for the pumiceous sands mapped by Rich (Citation1959) south of the Manawatu Gorge () were substantially younger than the c. 1 Ma Potaka Pumice, we suggest here a strong likelihood that the band of Castlecliffian volcaniclastics mapped along the Pohangina Monocline (Rees et al. Citation2018b) continue to the south, east of Palmerston North (Rich Citation1959). This correlation is solely based on stratigraphic position and mapping conducted farther north.
Coutts Creek Horizon – Rewa Formation
Feldmeyer et al. (Citation1943) mapped the Coutts Creek Horizon west from Hunterville into the Makirikiri Valley, 10 km NE of Whanganui (). They described 45 m of very fine, interbedded tephric sand and silt that is hard and well consolidated, resulting in good exposure (). The succession thins from Rangitikei west toward Whanganui, with the stratigraphic distance between the Coutts Creek Horizon and the underlying Pakihikura Pumice reducing from c. 180 to 90 m, respectively. West of Whanganui, the horizon becomes unmappable, merging laterally into siliciclastic sediments of the upper Okehu Siltstone, western Whanganui Basin.
Figure 7. A, Coutts Creek Horizon cropping out along the southern scarp slope of Coutts Creek (BL34 2539 7275). B, Close up of Coutts Creek Horizon at the Coutts Creek locality. C, Coutts Creek Horizon forming a conspicuous ledge in the landscape D, An example of the landslides on the southern dip slopes in the Rangitikei District (BL34 0862 7724).
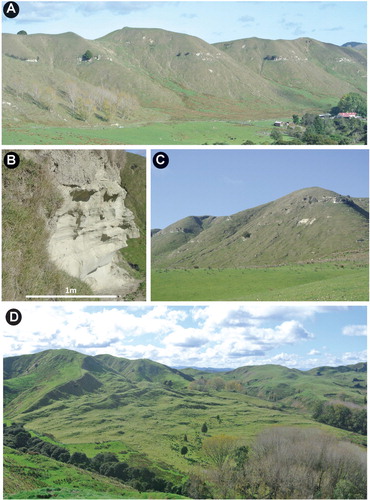
In this study, we logged Coutts Creek and adjacent sections forming a composite column () and correlating samples to the published geochemical datasets of Pillans et al. (Citation2005) (). The Coutts Creek Horizon is particularly well exposed, especially considering the nature of the heavily eroded country it crosses (D). This horizon typically outcrops on the steep northern scarp slopes, where the strata are dipping south, into the slope. The southern dip slopes in comparison are characterised by extensive landslides, related to bedding plane failures within the gently dipping strata.
Coutts Creek horizon was initially incorporated into the Kaimatira Pumice Sand Formation by Fleming (Citation1953) and Seward (Citation1976). However, the designated type section of the Kaimatira Pumice Sand near Whanganui is defined by the first influx of Potaka glass chemistry into the Whanganui Basin (Hellstrom Citation1993; Pillans et al. Citation2005; Rees et al. Citation2018b). The first influx of Potaka Pumice occurs c. 120 m above the Coutts Creek Horizon in the Coutts Creek succession. EPMA analysis of tephric silts from Coutts Creek are correlated to Rewa glass chemistry (E). The first occurrence of Mactra (Maorimactra) carteri occurs 30 m below the Coutts Creek horizon. Therefore, we suggest Coutts Creek Horizon falls within the Rewa Formation, Okehu Group ().
Makirikiri Tuff Formation – Pakihikura Pumice
Fleming (Citation1953) originally proposed Makirikiri Tuff Formation for the basal Castlecliffian ash of Feldmeyer et al. (Citation1943), reasoning that the use of ‘Castlecliff’ for a deposit that does not crop out at Castlecliff would be undesirable. Fleming (Citation1953) also suggested the use of the term ‘tuff’ would be more suitable than ‘ash’, a sentiment reflected in later papers by Schmid (Citation1981) and Froggatt and Lowe (Citation1990). The formation was described as white, fine to medium-grained tephric sands interfingering with lenses of coarse, pebbly sand and conglomerate. Fleming (Citation1953) described the Makirikiri Tuff Formation as the eastern correlative of the Butlers Shell Conglomerate. These two units interfinger with one another in the Okehu Stream area before the shell conglomerate is completely replaced by Makirikiri Tuff Formation in the Whanganui River Valley near Makirikiri Valley (). The base of the Makirikiri Tuff Formation was noted to be unconformable, similar to the Butlers Shell Conglomerate. The Makirikiri Tuff Formation thickens to the east, reflecting the location of the paleo-depocentre.
Difficulties have arisen from the application of stratigraphic nomenclature based on type sections near Whanganui to a succession that thickens and becomes somewhat more complete to the east. The presence of a long-lived 450 ka unconformity at the base of the Okehu Group, Whanganui type section, not present to the same extent in eastern Whanganui Basin, requires acknowledgment and suitable provision for regional mapping. We suggest here all groups introduced by Fleming (Citation1953) are retained, with a simple redefinition of group boundaries within the central and eastern Whanganui Basin to incorporate new stratigraphic data gathered over the past 65 years. We adopt Pakihikura Pumice (base of Makirikiri Tuff Formation) as the base of the Okehu Group as originally suggested by Fleming (Citation1953) ().
This redefinition results in Okehu Group encompassing a considerably thicker succession in the east, covering all strata from Pakihikura Pumice to Potaka Pumice. This allows Seward’s (Citation1976) correlation of Pakihikura Pumice, Ridge and Mangapipi tephras to Makirikiri Tuff Formation to be retained, however, necessitates an amendment of Seward’s (Citation1976) definition of Kaimatira Pumice Sand Formation from including Rewa Pumice to being defined by the first influx of Potaka Pumice. Rewa Pumice is then suggested to be placed within its own formation bridging the Makirikiri Tuff Formation and Kaimatira Pumice Sand Formation ().
Palaeogeographic interpretation
The first influx of fluvial conglomerate within the Whanganui Basin succession occurs progressively earlier toward the east. The conglomerates become increasingly frequent, thicker (10–200 m) and are associated with heterolithic intervals devoid of any marine fossils, including the common occurrence of lignite, wood and siltstone beds dominated entirely by small, stunted Austrovenus stutchburyi. This pattern within the succession is known to continue and become more dramatic within the upper Oroua catchment, where Feldmeyer et al. (Citation1943), Milne (Citation1968), Seward (Citation1974b) and Shane et al. (Citation1996) note the occurrence of conglomerate above and below the Pakihikura Pumice (). Feldmeyer et al. (Citation1943) and Milne (Citation1968) who mapped this upper portion of the Oroua Valley in detail suggest the conglomerates become increasingly prominent toward the Ruahine Range reaching thicknesses of c. 200 m. The conglomerates described in this area have an erosive lower contact cut into the underling sediments, large clasts (up to 230 mm) and grade upward into strongly current-bedded pebbly sandstones with rare interbedded lignite (Milne Citation1968).
Figure 8. Regional correlation of the Pakihikura Pumice toward the main axial range. Data, including the geochemical data from CJR-4, PS-137 and BP-609 are sourced from Milne (Citation1968), Carter (Citation1972), Shane et al. (Citation1996), Pillans et al. (Citation2005), Rees et al. (Citation2018b) and this study.
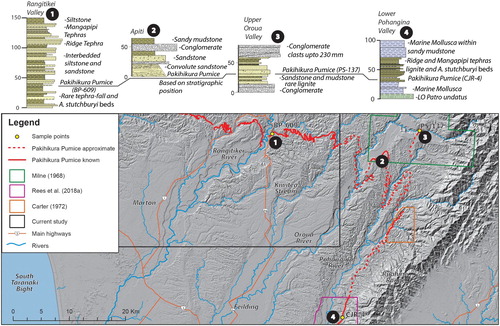
We suggest the conglomerates recognised in the upper Oroua Valley are part of a shared facies to those we document in the Rangitikei and Beaconsfield Valley successions (B and ), interfingering with the dominantly marine Whanganui Basin succession from a northeast direction. We interpret the common intercalated siltstone and lignite, channel cut and fill and total absence of marine fossils within these conglomerates to be typical of terrestrial deposits, characteristic of a braided alluvial plain system.
The first influx of fluvial conglomerate within the Whanganui Basin between the Whanganui and Rangitikei rivers does not occur until Toms Conglomerate, Shakespeare Group (c. 0.6 Ma); whilst in the Oroua Valley, we have evidence for influxes as early as c. 1.6 Ma (). This trend is confirmed in the Pohangina Valley, where a change to non-marine sedimentation occurs at c. 1.6 Ma. We suggest progressive influxes of coarse greywacke detritus were intermittently prograding southwestwards into the Whanganui Basin during sea level low stands, likely forming an alluvial plain about the emergent base of the paleo-axial ranges. Low stand periods being characterised by a lowering of the tree line in the ranges, increased mechanical breakdown and erosion, overloading of river headwaters with detritus and fluvial aggradation (Palmer and Pillans Citation1996). Incision of the Whanganui Basin fill occurred during marine transgression, as the climate warmed, erosion rates lowered in headwater catchments and paleo-rivers formed localised valleys that retreated landward as sea level continued to rise. Substantial erosion and reworking is evidenced by common rip up clasts, channel cut and fill and erosive contacts (). Progressive flooding of the eastern basin margin during marine transgression and high stand infilled local valley systems, such that they were transient, short-lived features on a shallow gradient coastline. Heterolithic deposition occurred within coastal plain to marginal margin environments, including common back swamp environments where preservation of organic matter and tephra-fall beds occurred (F).
A partially emergent, alluvial and coastal plain along the Whanganui Basin’s eastern margin during Castlecliffian time () is consistent with strata observed in the lower Pohangina Valley (Rees et al. Citation2018a, Citation2018b), Ruahine Range (MacDonald-Creevey Citation2011) and Kuripapango (Browne Citation2004) suggesting uplift of the North Island axial range began as early as Late Miocene time. Uplift along the Whanganui Basin’s eastern margin has resulted in pulses of greywacke detritus prograding into local eastern depocentres (Rees et al. Citation2018a). We suggest uplift in the axial range occurred contemporaneously with commencement of volcanic activity at the Mangakino Volcanic Centre c. 1.6 Ma. Such that the first influxes of fluvial conglomerate and volcaniclastics occur in close proximity within the eastern Whanganui Basin succession. The intercalation of siliciclastic and volcaniclastic sediment along the modern range front provides important constraint on the timing of uplift within the paleo-axial range.
Figure 9. A, Cartoon schematic of lower North Island at c. 1.6 Ma, during an early phase of large-scale rhyolitic eruptions from Mangakino Caldera (MA). Uplift along the paleo-axial range to the east and the western margin of the Whanganui Basin occurs during this time resulting in interbedded conglomeratic and volcaniclastic units being deposited within eastern Whanganui Basin and formation of a long-lived 450 ka unconformity in the western Whanganui Basin. B, Cartoon schematic of lower North Island at c. 1 Ma, during emplacement of Rocky Hill and Cape Kidnappers ignimbrites from Mangakino Caldera (MA). Sources of information include (Seward Citation1974b; Beu Citation1995; King and Thrasher Citation1996; Field et al. Citation1997; King Citation2000; Kamp et al. Citation2004; Kamp and Furlong Citation2006; Bunce et al. Citation2009; Cooper et al. Citation2012; Trewick and Bland Citation2012; Downs et al. Citation2014a; Gravley et al. Citation2016). Cartoons depict sea level highstands.
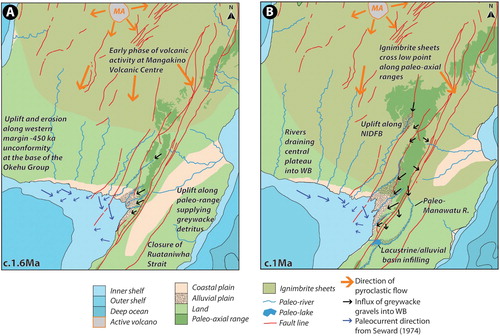
Conclusion
The eastern Whanganui Basin contains a Pleistocene record of sedimentation along the emerging margin of the Whanganui Basin and paleo-axial range. Tephra-fall and volcaniclastic deposits provide useful chronostratigraphic marker horizons, enabling correlation between sections within the Whanganui Basin.
Lateral changes in sedimentary preservation across the Whanganui Basin have led to difficulties with correlation, resulting in nomenclatural issues. We present a revised version of the Whanganui Basin stratigraphic nomenclature, enabling historic precedence to be maintained, whilst incorporating modern techniques and new datasets. We correlate Coutts Creek Horizon to Rewa Formation and formally propose Okehu Group to cover all strata from Pakihikura to Potaka Pumice. We recommend Rewa Pumice is placed within its own formation bridging the Makirikiri Tuff Formation and Kaimatira Pumice Sand Formation.
Influxes of conglomeratic and carbonaceous rich heterolithic facies within the basin succession are interpreted to be a feature of an alluvial and coastal plain adjacent to the paleo-axial range. The first influx of fluvial conglomerate occurs shortly before the Pakihikura Pumice (c. 1.6 Ma) within the upper Oroua catchment, whilst within the Rangitikei, there is a delay until around Toms Conglomerate (c. 0.6 Ma). We suggest gravels derived from uplifting basement in the position of the main axial range were entering the Whanganui Basin from the northeast. Sea level low stands being characterised by aggradation and progradation of broad fans of greywacke gravel forming an apron of sediment about the paleo-axial range front. Marine transgression is characterised by a warmer climate, reduction of erosion in headwater catchments and fluvial incision into and reworking of LST deposits. Landward migration of the coastal plain and fluvial distributary systems during rising sea level resulted in sedimentary infilling of channels and valleys. We suggest uplift in the main axial range occurred contemporaneously with the onset of volcanic activity at Mangakino caldera so that the first influxes of fluvial conglomerate and volcaniclastics occur in close proximity within the eastern Whanganui Basin succession.
Supplementary Data File C R1
Download PDF (1.6 MB)Supplementary Data File B R1
Download MS Excel (48.2 KB)Supplementary Data File A R1
Download PDF (1.9 MB)Acknowledgements
We thank the residents in the Rangitikei and Manawatu districts for granting land access. This work is part of an overarching PhD project on the groundwater of the Pourewa sub-catchment undertaken by CR. Thanks are extended to Dr Ranvir Singh and Dr John Begg for the support and direction given during the course of this research.
Disclosure statement
No potential conflict of interest was reported by the authors.
ORCID
Callum Rees http://orcid.org/0000-0001-5213-9598
Correction Statement
This article has been republished with minor changes. These changes do not impact the academic content of the article.
Additional information
Funding
References
- Abbott ST. 1994. Sequence stratigraphy, sedimentology and paleoecology of Pleistocene cyclothems in the castlecliff section, Wanganui Basin, New Zealand [unpublished PhD thesis]. James Cook University of Northern Queensland, Australia. 297 p.
- Alloway BV, Pillans BJ, Sandhu AS, Westgate JA. 1993. Revision of the marine chronology of the Wanganui Basin, New Zealand, based on the isothermal plateau fission-track dating of tephra horizons. Sedimentary Geology. 82(1–4):299–310. doi: 10.1016/0037-0738(93)90128-R
- Alloway BV, Westgate JA, Pillans B, Pearce N, Newnham R, Byrami M, Aarburg S. 2004. Stratigraphy, age and correlation of middle Pleistocene silicic tephras in the Auckland Region, New Zealand: a prolific distal record of TVZ volcanism. New Zealand Journal of Geology and Geophysics. 47:447–479. doi: 10.1080/00288306.2004.9515070
- Beu AG. 1995. Pliocene limestones and their scallops: lithostratigraphy, pectinid biostratigraphy and paleogeography of eastern North Island late Neogene limestone. Institute of Geological and Nuclear Sciences Monograph. 10(68):1–243.
- Beu AG. 2006. Marine Mollusca of oxygen isotope stages of the last 2 million years in New Zealand. Part 2. Biostratigraphically useful and new Pliocene to recent bivalves. Journal of the Royal Society of New Zealand. 36(4):151–338. doi: 10.1080/03014223.2006.9517808
- Boellstorff JD, Te Punga MT. 1977. Fission track age and correlation of middle and lower Pleistocene sequences from Nebraska and New Zealand. New Zealand Journal of Geology and Geophysics. 20:47–58. doi: 10.1080/00288306.1977.10431591
- Brackley H. 1999. The stratigraphy and environments of deposition of early-mid Pleistocene sediments of the Pohangina Region, Eastern Wanganui Basin, New Zealand [MSc, unpublished MSc thesis]. Palmerston North, New Zealand: Massey University. 113 p.
- Browne GH. 2004. Late Neogene sedimentation adjacent to the tectonically evolving North Island axial ranges: insights from Kuripapango, western Hawke’s Bay. New Zealand Journal of Geology and Geophysics. 47(4):663–674. doi: 10.1080/00288306.2004.9515082
- Bunce M, Worthy TH, Phillips MJ, Holdaway RN, Willerslev E, Haile J, Shapiro B, Scofield RP, Drummond A, Kamp PJJ, et al. 2009. The evolutionary history of the extinct ratite moa and New Zealand Neogene paleogeography. Proceedings of the National Academy of Sciences. 106(49):20646–20651. doi: 10.1073/pnas.0906660106
- Bussell MR. 1984. Geology and paleobotany of the Rangitawa Stream area, southeast Wanganui Basin, Unpublished BSc (Hons) [Thesis]. Wellington, New Zealand: Victoria University of Wellington, 181 p.
- Carter L, Shane PAR, Alloway BV, Hall IR, Harris SE, Westgate JA. 2003. Demise of one volcanic zone and birth of another – A 12 m.y. Marine record of major Rhyolitic eruptions from New Zealand. Geology. 31(6):493–496. doi: 10.1130/0091-7613(2003)031<0493:DOOVZA>2.0.CO;2
- Carter RM. 1972. Wanganui strata of Komako District, Pohangina Valley, Ruahine range, Manawatu. Journal of the Royal Society of New Zealand. 2(3):293–324. doi: 10.1080/03036758.1972.10421819
- Columbus J, Sirguey P, Tenzer R. 2011. A free fully assessed 15 m digital elevation model for New Zealand. Survey Quarterly. 300(66):16–19.
- Cooper GF, Morgan DJ, Wilson CJN. 2017. Rapid assembly and rejuvenation of a large silicic magmatic system: insights from mineral diffusive profiles in the Kidnappers and Rocky Hill deposits, New Zealand. Earth and Planetary Science Letters. 473:1–13. doi: 10.1016/j.epsl.2017.05.036
- Cooper GF, Wilson CJN, Millet M, Baker JA, Smith EGC. 2012. Systematic tapping of independent magma chambers during the 1 Ma Kidnappers supereruption. Earth and Planetary Science Letters. 313:23–33. doi: 10.1016/j.epsl.2011.11.006
- Downs DT, Rowland JV, Wilson CJN, Rosenberg MD, Leonard GS, Calvert AT. 2014a. Evolution of the intra-arc Taupo-Reporoa Basin within the Taupo Volcanic Zone of New Zealand. Geosphere. 10(1):185–206. doi: 10.1130/GES00965.1
- Downs DT, Wilson CJN, Cole JW, Rowland JV, Calvert AT, Leonard GS, Keall JM. 2014b. Age and eruptive center of the Paeroa Subgroup ignimbrites (Whakamaru Group) within the Taupo Volcanic Zone of New Zealand. Geological Society of America Bulletin. 126(9/10):1131–1144. doi: 10.1130/B30891.1
- Feldmeyer AE, Jones BC, Firth CW, Knight J. 1943. The geology of the Palmerston – Wanganui Basin, ‘West side’, North Island, New Zealand. Unpublished Report of the Superior Oil Company of New Zealand, Petroleum Report Series 171, Ministry of Economic Development, Wellington, New Zealand. 46 p.
- Field BD, Uruski CI, Beu AG, Browne G, Crampton J, Funnell R, Killops S, Laird M, Mazengarb C, Morgans H, et al. 1997. Cretaceous-Cenozoic geology and petroleum systems of the east coast region, New Zealand: lower Hutt, New Zealand. Institue of Geological and Nuclear Sciences Monograph. 19:301.
- Fleming CA 1953. The geology of Wanganui subdivision. New Zealand Geological Survey Bulletin 52. New Zealand Department of Scientific and Industrial Research, Wellington, New Zealand, 362 p.
- Froggatt PC, Lowe DJ. 1990. A review of late Quaternary silicic and some other tephra formations from New Zealand: their stratigraphy, nomenclature, distribution, volume, and age. New Zealand Journal of Geology and Geophysics. 33:89–109. doi: 10.1080/00288306.1990.10427576
- Grant GR, Sefton JP, Patterson MO, Naish TR, Dunbar GB, Hayward BW, Morgans HEG, Alloway BV, Seward D, Tapia CA, et al. 2018. Mid- to late Pliocene (3.3-2.6 Ma) global sea-level fluctuations recorded on a continental shelf transect, Whanganui Basin, New Zealand. Quaternary Science Reviews. 201:241–260. doi: 10.1016/j.quascirev.2018.09.044
- Gravley DM, Deering CD, Leonard GS, Rowland JV. 2016. Ignimbrite flare-ups and their drivers: a New Zealand perspective. Earth-Science Reviews. 162:65–82. doi: 10.1016/j.earscirev.2016.09.007
- Hellstrom JC 1993. An investigation of the Galpin Fault, Marton, Rangitikei [Unpublished BSc (Hons) Thesis]. Wellington, New Zealand: Victoria University of Wellington, 89 p.
- Jarosewich E, Nelen JA, Norberg JA. 1980. Reference samples for electron microprobe analysis. Geostandards Newsletter. 4(1):43–47. doi: 10.1111/j.1751-908X.1980.tb00273.x
- Jochum KP, Stoll B, Herwig K, Willbold M, Hofmann AW, Amini M, Aarburg S, Abouchami W, Hellebrand E, Mocek B, et al. 2006. MPI-DING reference glasses for in situ microanalysis: new reference values for element concentrations and isotope ratios. Geochemistry, Geophysics, Geosystems. 7(2):1–44. doi: 10.1029/2005GC001060
- Journeaux TD, Kamp PJJ, Naish TR. 1996. Middle Pliocene cyclothems, Mangaweka region, Wanganui Basin, New Zealand: A lithostratigraphic framework. New Zealand Journal of Geology and Geophysics. 39(1):135–149. doi: 10.1080/00288306.1996.9514700
- Kamp PJJ, Furlong KP. 2006. Neogene pate tectonic reconstructions and geodynamics of North Island sedimentary basins: implications for petroleum systems. New Zealand Petroleum Conference. 1:6–10.
- Kamp PJJ, Vonk AJ, Bland KJ, Hansen RJ, Hendy AJW, McIntyre AP, Ngatai M, Cartwright SJ, Hayton S, Nelson CS. 2004. Neogene stratigraphic architecture and tectonic evolution of Wanganui, King country, and eastern Taranaki Basins, New Zealand. New Zealand Journal of Geology and Geophysics. 47(4):625–644. doi: 10.1080/00288306.2004.9515080
- King PR. 2000. Tectonic reconstructions of New Zealand: 40 Ma to the present. New Zealand Journal of Geology and Geophysics. 43(43):611–638. doi: 10.1080/00288306.2000.9514913
- King PR, Thrasher GP. 1996. Cretaceous-Cenozoic geology and petroleum systems of the Taranaki Basin, New Zealand. Institute of Geological and Nuclear Sciences Monograph 13. 6 enclosures, 243 p.
- Krippner SJP, Briggs RM, Wilson CJN, Cole JW. 1998. Petrography and geochemistry of lithic fragments in ignimbrites from the Mangakino Volcanic Centre: implications for the composition of subvolcanic crust in western Taupo Volcanic Zone, New Zealand. New Zealand Journal of Geology and Geophysics. 41(2):187–199. doi: 10.1080/00288306.1998.9514803
- Lee JM, Bland KJ, Townsend DB, Kamp PJJ, (compilers) 2011. Geology of the Hawke’s Bay area. Institute of Geological & Nuclear Sciences 1:250,000 geological map 8. Lower Hutt, New Zealand. GNS Science. 1 sheet + 93 p.
- Le Maitre RW. 1984. A proposal by the IUGS Subcommission on the systematics of igneous rocks for a chemical classification of volcanic rocks based on total Alkali silica (TAS) diagram. Australian Journal of Earth Sciences. 31(2):243–255. doi: 10.1080/08120098408729295
- Lowe DJ. 1990. Tephra studies in New Zealand: an historical review. Journal of the Royal Society of New Zealand. 20(1):119–150. doi: 10.1080/03036758.1990.10426736
- MacDonald-Creevey AM. 2011. Late Holocene environmental record and geological history of the Lake Colenso area, North-western Ruahine Range, New Zealand [Unpublished MSc thesis]. Palmerston North, New Zealand: Massey University. 159 p.
- Marden M, Neall VE. 1990. Dated Ohakean terraces offset by the Wellington Fault, near Woodville. New Zealand. New Zealand Journal of Geology and Geophysics. 33(3):449–453. doi: 10.1080/00288306.1990.10425700
- McGuirre DM. 1989. Paleomagnetic stratigraphy and magnetic properties of Pliocene strata, Turakina River, North Island, New Zealand [unpublished PhD thesis]. 280–280 p.
- McIntyre AP 2002. Geology of Mangapanian (late Pliocene) strata, Wanganui Basin: lithostratigraphy, paleontology and sequence stratigraphy. [PhD thesis]. Hamilton, New Zealand: Waikato University, 283 p.
- McIntyre AP, Kamp PJJ. 1998. Late Pliocene (2.8-2.4 Ma) cyclothemic shelf deposits, Parikino, Wanganui Basin, New Zealand: lithostratigraphy and correlation of cycles. New Zealand Journal of Geology and Geophysics. 41:69–84. doi: 10.1080/00288306.1998.9514791
- Melhuish A, van Dissen R, Berryman KR. 1996. Mount Stewart-Halcombe anticline: a look inside a growing fold in the Manawatu region, New Zealand. New Zealand Journal of Geology and Geophysics. 39:123–133. doi: 10.1080/00288306.1996.9514699
- Milne JDG. 1968. The geology and soils of the Apiti District. [Unpublished MSc thesis]. New Zealand: Victoria Universtiy of Wellington, 122 p.
- Milne JDG. 1973a. Mount Curl tephra, a 230000-year-old implications for quaternary chronology marker bed in New Zealand, and its implications for Quaternary chronology. New Zealand Journal of Geology and Geophysics. 16(3):519–532. doi: 10.1080/00288306.1973.10431375
- Milne JDG. 1973b. Upper Quaternary geology of the Rangitikei drainage basin, North Island, New Zealand. [Unpublished PhD Thesis]. Wellington, New Zealand: Victoria University of Wellington, 472 p.
- Naish TR, Abbott ST, Alloway BV, Beu AG, Carter RM, Edwards AR, Journeaux TD, Kamp PJJ, Pillans BJ, Saul G, et al. 1998. Astronomical calibration of a southern hemisphere Plio-Pleistocene reference section, Wanganui Basin, New Zealand. Quaternary Science Reviews. 17(8):695–710. doi: 10.1016/S0277-3791(97)00075-9
- Naish TR, Field BD, Zhu H, Melhuish A, Carter RM, Abbott ST, Edwards S, Alloway BV, Wilson GS, Niessen F, et al. 2005. Integrated outcrop, drill core, borehole and seismic stratigraphic architecture of a cyclothemic, shallow marine depositional system, Wanganui Basin, New Zealand. Journal of the Royal Society of New Zealand. 35(1–2):91–122. doi: 10.1080/03014223.2005.9517778
- Naish TR, Kamp PJJ. 1995. Pliocene-Pleistocene marine cyclothems, Wanganui Basin, New Zealand: a lithostratigraphic framework. New Zealand Journal of Geology and Geophysics. 38:223–243. doi: 10.1080/00288306.1995.9514651
- Naish TR, Kamp PJJ, Alloway BV, Pillans BJ, Wilson GS, Westgate JA. 1996. Integrated tephrochronology and magnetostratigraphy for cyclothemic marine strata, Wanganui Basin: implications for the Pliocene-Pleistocene boundary in New Zealand. Quaternary International. 34–36(95):29–48. doi: 10.1016/1040-6182(95)00067-4
- Nicol A. 2011. Landscape history of the Marlborough Sounds, New Zealand. New Zealand Journal of Geology and Geophysics. 54(2):195–208. doi: 10.1080/00288306.2010.523079
- Nicol A, Mazengarb C, Chanier F, Rait G, Uruski C, Wallace L. 2007. Tectonic evolution of the active Hikurangi subduction margin, New Zealand, since the Oligocene. Tectonics. 26(4):1–24. doi: 10.1029/2006TC002090
- Palmer AS, Pillans BJ. 1996. Record of climatic fluctuations from ca. 500 Ka loess deposits and paleosols near Wanganui, New Zealand. Quaternary International. 34-36:155–162. doi: 10.1016/1040-6182(95)00080-1
- Pillans B, Kohn B. 1981. Rangitawa Pumice: a widespread (?) Quaternary marker bed in Taranaki-Wanganui. Victoria University of Wellington Geology Department Publication. 20:94–104.
- Pillans BJ. 1983. Upper Quaternary marine terrace chronology and deformation, South Taranaki. New Zealand. Geology. 11(5):292–297.
- Pillans BJ. 1994. Direct marine-terrestrial correlations, Wanganui Basin, New Zealand: the last 1 million years. Quaternary Science Reviews. 13(3):189–200. doi: 10.1016/0277-3791(94)90024-8
- Pillans BJ, Alloway B, Naish TR, Westgate J, Abbott S, Palmer AS. 2005. Silicic tephras in Pleistocene shallow-marine sediments of Wanganui Basin, New Zealand. Journal of the Royal Society of New Zealand. 35(1–2):43–90. doi: 10.1080/03014223.2005.9517777
- Pillans BJ, McGlone M, Palmer A, Mildenhall D, Alloway B, Berger G. 1993. The last glacial maximum in central and southern North Island, New Zealand: a paleoenvironmental reconstruction using the Kawakawa tephra Formation as a chronostratigraphic marker. Palaeogeography, Palaeoclimatology, Palaeoecology. 101(3):283–304. doi: 10.1016/0031-0182(93)90020-J
- Pillans BJ, Roberts AP, Wilson GS, Abbott ST, Alloway BV. 1994. Mangnetostratigraphic, lithostratigraphic and tephrostratigraphic constraints on lower and middle Pleistocene sea-level changes, Wanganui Basin, New Zealand. Earth and Planetary Science Letters. 121:81–98. doi: 10.1016/0012-821X(94)90033-7
- Potter WD. 1984. Upper Quaternary geology of part of the lower Rangitikei Valley, Unpublished BSc (hons). [Thesis]. Wellington, New Zealand: Victoria University of Wellington, 84 p.
- Rattenbury MS, Heron DW. 1997. Revised procedures and specifications for the QMAP GIS, Institute of Geological and Nuclear Sciences science report 97/3, Lower Hutt, New Zealand. 52 p.
- Rees CJ, Palmer JA, Palmer AS. 2018a. Gilbert-style Pleistocene fan delta reveals tectonic development of North Island axial ranges, New Zealand. New Zealand Journal of Geology and Geophysics. 61(1):64–78. doi: 10.1080/00288306.2017.1406377
- Rees CJ, Palmer JA, Palmer AS. 2018b. Plio-Pleistocene geology of the lower Pohangina Valley, New Zealand. New Zealand Journal of Geology and Geophysics. 61(1):44–63. doi: 10.1080/00288306.2017.1408023
- Rees CJ, Palmer JA, Palmer AS. 2019. Whanganui Basin, an archive of prehistoric earthquakes? Waitapu shell conglomerate (0.9 Ma), North Island, New Zealand. New Zealand Journal of Geology and Geophysics. 62(2):195–216. doi:10.1080/00288306.2019.1587475.
- Rich CC. 1959. Late Cenozoic geology of the lower Manawatu valley, New Zealand. [PhD thesis]. Cambridge, Massachusetts, USA: Harvard University, 188 p.
- Schmid R. 1981. Descriptive nomenclature and classification of pyroclastic deposits and fragments: recommendations of the IUGS Subcommission on the systematics of igneous rocks. Geology. 9:41–43. doi: 10.1130/0091-7613(1981)9<41:DNACOP>2.0.CO;2
- Seward D. 1974a. Age of New Zealand Pleistocene substages by fission-track dating of glass shards from tephra horizons. Earth and Planetary Science Letters. 24(2):242–248. doi: 10.1016/0012-821X(74)90102-2
- Seward D. 1974b. Some aspects of sedimentology of the Wanganui Basin, North Island, New Zealand. [PhD, Unpublished PhD thesis]. Wellington, New Zealand: Victoria University of Wellington. 285 p.
- Seward D. 1976. Tephrostratigraphy of the marine sediments in the Wanganui Basin, New Zealand. New Zealand Journal of Geology and Geophysics. 19(1):9–20. doi: 10.1080/00288306.1976.10423546
- Seward D. 1978. Palaeosalinities and palaeotemperatures from carbon and oxygen isotopes of carbonate shells in three Quaternary formations, Wanganui Basin, New Zealand. Palaeogeography, Palaeoclimatology, Palaeoecology. 23:47–55. doi: 10.1016/0031-0182(78)90081-0
- Seward D. 1979. Comparison of zircon and glass fission-track ages from tephra horizons. Geology. 7(10):479–482. doi: 10.1130/0091-7613(1979)7<479:COZAGF>2.0.CO;2
- Seward D, Kohn BP. 1997. New zircon fission-track ages from New Zealand Quaternary tephra: an interlaboratory experiment and recommendations for the determination of young ages. Chemical Geology. 141(1–2):127–140. doi: 10.1016/S0009-2541(97)00086-7
- Seward D, Lienert B. 1986. Magnetic polarity stratigraphy of a Plio-Pleistocene marine sequence of North Island, New Zealand. Earth and Planetary Science Letters. 80(3–4):353–360. doi: 10.1016/0012-821X(86)90117-2
- Shane PA, Black TM, Alloway BV, Westgate JA. 1996. Early to middle Pleistocene tephrochronology of North Island, New Zealand: implications for volcanism, tectonism, and paleoenvironments. Geological Society of America Bulletin. 108(8):915–925. doi: 10.1130/0016-7606(1996)108<0915:ETMPTO>2.3.CO;2
- Shane PAR. 1991. Remobilised silicic tuffs in middle Pleistocene fluvial sediments, southern North Island, New Zealand. New Zealand Journal of Geology and Geophysics. 34(4):489–499. doi: 10.1080/00288306.1991.9514485
- Shane PAR, Walter R, Froggatt P. 1992. 40Ar/39Ar ages of Pleistocene silicic tephras in New Zealand: implications for the age of the Jaramillo Subchron. Geological Society of New Zealand Miscellaneous Publication. 63A: 1–139.
- Stevens KF, Vella P. 1981. Paleoclimatic interpretation of stable isotope ratios in molluscan fossils from middle Pleistocene marine strata, Wanganui, New Zealand. Palaeogeography, Palaeoclimatology, Palaeoecology. 34:257–265. doi: 10.1016/0031-0182(81)90067-5
- Te Punga MT. 1952. The geology of Rangitikei Valley. New Zealand Geological Survey Memoir 8, Wellington, New Zealand. 46 p.
- Te Punga MT. 1957. Live anticlines in western Wellington. New Zealand Journal of Science and Technology. 38:433–466.
- Townsend D, Vonk A, Kamp PJJ. 2008. Geology of the Taranaki area: scale 1:250,000. Lower Hutt, GNS Science. Institute of Geological and Nuclear Sciences 1:250,000 geological map 7, Lower Hutt, New Zealand: 1 sheet + 77p.
- Trewick SA, Bland KJ. 2012. Fire and slice: palaeogeography for biogeography at New Zealand’s North Island/south Island juncture. Journal of the Royal Society of New Zealand. 42:153–183. doi: 10.1080/03036758.2010.549493
- Turner GM, Kamp PJJ. 1990. Palaeomagnetic location of the Jaramillo Subchron and the Matuyama-Brunhes transition in the Castlecliffian stratotype section, Wanganui Basin, New Zealand. Earth and Planetary Science Letters. 100(1–3):42–50. doi: 10.1016/0012-821X(90)90174-V
- Turner GM, Kamp PJJ, McIntyre AP, Hayton S, McGuire DM, Wilson GS. 2005. A coherent middle Pliocene magnetostratigraphy, Wanganui Basin, New Zealand. Journal of the Royal Society of New Zealand. 35(1–2):197–227. doi: 10.1080/03014223.2005.9517781
- van der Neut M. 1996. Sequence stratigraphy of Plio-Pleistocene sediments in lower Turakina Valley, Wanganui Basin, New Zealand [Unpublished MSc Thesis]. Palmerston North, New Zealand: Massey Univeristy, 149 p.
- Wilson CJN, Gravley DM, Leonard GS, Rowland JV. 2009. Volcanism in the central Taupo Volcanic Zone, New Zealand: temp, styles and controls. In: Thordarson T, Self S, Larsen G, Rowland SK, Hoskuldsson A, editors. Studies in volcanology: The legacy of George Walker, special publications of IAVCEI. London, UK: Geological Society; p. 225–247.
- Wilson CJN, Rowland JV. 2016. The volcanic, magmatic and tectonic setting of the Taupo Volcanic Zone, New Zealand, reviewed from a geothermal perspective. Geothermics. 59:168–187. doi: 10.1016/j.geothermics.2015.06.013