ABSTRACT
The intraplate rocks of the Dunedin Volcanic Group (DVG) in New Zealand’s South Island erupted in two discrete areas between 25 and 21 Ma before becoming distributed over > 7,800 km2 until ∼9 Ma. Although most eruptive centres were of small volume and mainly vented alkaline basanite, the largest centre–the 16–11 Ma composite Dunedin Volcano–discharged basanite and basalt through to trachyte and phonolite. DVG components were mainly derived from mantle sources with 87Sr/86Sr = ∼0.7029, 143Nd/144Nd = ∼0.5129, 206Pb/204Pb = ∼20.0, 207Pb/204Pb = ∼15.65, 208Pb/204Pb = 39.5 and εHf = +3.5 to + 10.1 that extended to anomalously light δ26Mg (−0.47). Exceptions are some potassic basalts in NW of the field with elevated 207Pb/204Pb and more radiogenic Sr. The DVG Sr-Nd-Pb isotopes mostly overlap with metasomatised anhydrous mantle peridotite xenoliths but have less radiogenic Hf, meaning that equivalent anhydrous mantle rock-types cannot be the sole magma sources. Although there is debate regarding whether DVG was derived from the lithospheric or asthenospheric mantle, intermittent melting of a middle lithospheric mantle metasomatised by hydrous asthenosphere-derived melts could account for: (1) the widely distributed magmatism for ∼16 Myr during which time Otago lithosphere shifted NW ∼ 870 km over the asthenosphere; (2) the small chemical range of the least evolved magmas; (3) the Sr-Nd-Pb-Hf isotopic range; and (4) an absence of lower lithosphere mantle xenoliths. This process could account for other occurrences of isotopically restricted Zealandia alkaline intraplate volcanism.
Introduction
The 4.9 M km2 Zealandia continent preserves a remarkable record of long-lived and spatially distributed intraplate volcanism. These volcanic fields form the geological foundations to four of New Zealand’s most populous cities (Auckland, Christchurch, Dunedin and Hamilton) but there are many other eruptive centres (; Timm et al. Citation2010; Mortimer and Scott Citation2020). Although some eruptions have occurred near plate boundaries (e.g. Alpine Dike Swarm; Cooper Citation2020), in zones of crustal extension (e.g. Westland Dikes; Waight et al. Citation1998; van der Meer et al. Citation2017), or on the margin of the continental crust (e.g. Auckland Islands; Gamble et al. Citation2018; Scott and Turnbull Citation2019), most do not (). The oldest intraplate eruption took place at 102 Ma (van der Meer et al. Citation2016) and the youngest occurred only 500 years ago (Needham et al. Citation2011), and neither geographical nor temporal distributions are systematic. While the next eruption will likely be in the dormant Auckland Volcanic Field, where the next eruptive field will form is not currently predictable.
Figure 1. Zealandia, which is 94% underwater, is here shown with a selection of its intraplate volcanic fields and eruptions. The inset shows the configuration of the paleo-Pacific Gondwana subduction margin prior to fragmentation of Australia-Antarctica-Zealandia. The diagram is modified from Scott et al. (Citation2019).
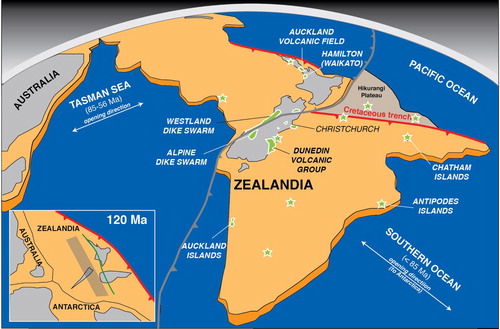
In this contribution, the distribution, age, chemistry and isotopic properties of one of Zealandia’s largest and longest-lived intraplate volcanic fields is reviewed. We present 559 whole rock chemical analyses, 91 K/Ar and 40Ar/39Ar dates, and Sr + Nd, Pb, Hf and Mg isotope data for 87 samples from the Dunedin Volcanic Group (DVG) in the South Island ( and ). Some data are published, some are from unpublished University of Otago student theses, and some are new; all are presented in the Data Supplementary files. DVG rocks have long been known to be part of an alkaline Late Cenozoic volcanic province (Ulrich Citation1891; Marshall Citation1906). Although the last eruption was at ∼ 9 Ma, a prominent seismic reflector near the base of the crust is interpreted to be the signature of recently ponded magma (Godfrey et al. Citation2001), and anomalously high 3He concentrations in gas-rich springs of the Dunedin area may be fed by outgassing of magmas emplaced in the lower crust (Hoke et al. Citation2000). A thorough understanding of the DVG eruptive history would be useful for assessing the significance of magma potentially being sequestered beneath Dunedin today.
Geological Context of East Otago
The DVG () was erupted through 25–30 km thick crust in East Otago in the South Island of New Zealand. This crust is dominated by Otago Schist, which is composed of metamorphosed Gondwana accretionary prism rocks of the dominantly Permo-Triassic Rakaia and Caples terranes (Mortimer Citation1993). The highest Otago Schist metamorphic grade is greenschist facies, with the metamorphic fabrics having been imposed in the Late Jurassic and Early Cretaceous (Mortimer Citation1993; Gray and Foster Citation2004); however, metasedimentary xenoliths in the 33 Ma Kakanui Mineral Breccia component of the Waiareka-Deborah Volcanic Province in North Otago indicate that the middle to lower crust experienced ultra-high-temperature granulite facies conditions at ∼92 Ma (Jacob et al. Citation2017). The lowermost Otago crust has P-wave velocities consistent with a ∼5 km thick zone of mafic underplate or basaltic crust (Godfrey et al. Citation2001).
Figure 2. Map showing the extent of the Dunedin Volcanic Group, with eruptions classified by age. The inset in the upper left corner shows the first derivative geophysical map from the Glass Earth -Otago Regional Council 2007 aeromagnetic survey.
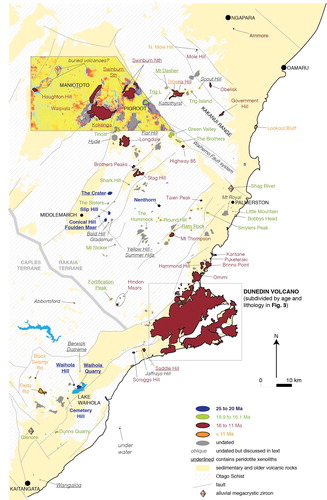
The Otago Schist is overlain by a transgressive and then regressive sedimentary sequence deposited from Late Cretaceous to Miocene time (Bishop Citation1994; Forsyth Citation2001). Deposits commonly form only a thinner veneer that is best-preserved adjacent to faults or underneath DVG lavas (). The peak of marine transgression is marked by an Oligocene carbonate platform that represents near-total continent submergence (Landis et al. Citation2008). Late Oligocene faulting lifted Otago above sea level (Forsyth Citation2001).
Distribution of the DVG
The DVG comprises hundreds of separate lava flows, dikes, sills, tuffs and vent-filling breccias. These are marked as eruptive centres in , of which the most prominent is the partially eroded Dunedin Volcano (). The southernmost known DVG eruption was near Kaitangata and the northernmost occurs 160 km to the north at Arnmore near Ngapara (). The easternmost on-land occurrence is at Lookout Bluff in north Otago but offshore underwater centres are known from seismic data (Gorman et al. Citation2013). The northwestern extent is indicated by Haughton Hill in the Maniototo. The DVG therefore has a minimum eruption area of ∼7,800 km2.
Figure 3. Geological map of the Dunedin Volcano, constructed from Benson (Citation1968), Martin (Citation2000) and Price and Coombs (Citation1975) and personal observations. Data sources for ages are 1, McDougall and Coombs (Citation1973); 2, Hoernle et al. (Citation2006); 3, Coombs et al. (Citation2008); 4, Timm et al. (Citation2010).
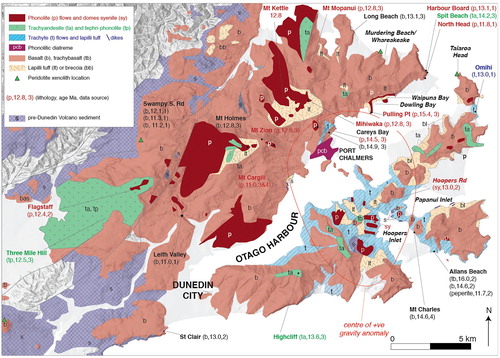
Coombs et al. (Citation1986, Citation2008) formally defined the DVG as comprising two members: the central composite Dunedin Volcano and rocks of the outlying and mainly monogenetic eruptive flows, plugs, dikes and tuffs assigned to the Waipiata Volcanic Formation. However, the distinction between two members is impractical because the ages, geochemical and isotopic (Sr-Nd-Pb-Mg) data overlap, and the boundary between the two members was arbitrarily placed where the stacked flows of the central volcano end (Coombs et al. Citation2008). It is here recommended that Dunedin Volcanic Group (DVG) be used for rocks of the entire volcanic province.
Dunedin Volcano
The oldest known components assigned to the Dunedin Volcano are tuffs within Miocene sandstone and limestone outcropping at Waipuna Bay, Dowling Bay and Blanket Bay on the western side of Otago Harbour () (Coombs et al. Citation1960a, Citation1960b). While these clearly indicate deposition of volcanic ash in those areas, 40Ar/39Ar dating and mapping suggests that submarine eruptions may have occurred slightly earlier in the vicinity of Allans Beach on the Otago Peninsula ( and A) (Martin and White Citation2001; Hoernle et al. Citation2006; Baxter Citation2019). Benson (Citation1941, Citation1942a, Citation1942b, Citation1968) subdivided rocks of the Dunedin Volcano into 4 phases (Initial, First, Second and Third) separated by periods of erosion. Most subsequent workers have struggled to apply these phases; for example, Coombs et al. (Citation1960a) and Allen (Citation1974) found several hundred metres of basaltic and trachytic tuff and basaltic flows beneath the base of the initial phase, and the erosion surfaces inferred by Benson to separate different phases cannot be widely extrapolated (Price and Coombs Citation1975). Regardless of where and precisely when it initiated, the volcano’s first deposits formed in shallow submarine conditions. The volcano was later sub-aerially exposed and built stacks of sub-aerial flows and tuffs ( and A, 4B) that today reach at least as high as the 680 m summit of Mt Cargill (C).
Figure 4. A. View to the southwest over the Dunedin Volcano. B. North Head at Aramoana displays a section through a heterogeneous stack of lavas. C. At 680 m, Mt Cargill is the highest point of the volcano and is capped by phonolitic domes. D. Saddle Hill, Jaffrays Hill and Scroggs Hill are prominent volcanic piles that overlie Cenozoic sequences just south of Dunedin city. E. The Crater near Middlemarch is the oldest dated DVG component and is an eroded diatreme within Otago Schist. A, C, D and E are from the Lloyd Homer GNS collection.
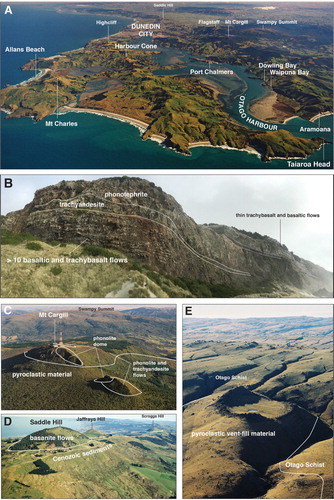
There is no clear correlation of erupted magma compositionFootnote1 versus time, with evolved (phonolite, trachyte) and primitive (basanite) rocks emplaced through the sequence. This eruption heterogeneity is exemplified by the spectacular lava flow stack in the cliffs of North Head at Aramoana (B; see also Marshall Citation1914). The early eruptive basalt to trachyte components to the volcano require a complicated plumbing system involving fractionation (Coombs et al. Citation1960a; Price and Taylor Citation1973; Allen Citation1974; Baxter Citation2019). Capping flows and domes on the top of the volcano appear to mainly be phonolite and trachyandesite (Price and Coombs Citation1975; Martin Citation2000) ( and C).
A significant positive gravity anomaly occurs over the centre of Otago Harbour () (Reilly Citation1972) and is modelled to represent a 13 km diameter by 10 km thick cylinder of unerupted frozen magma. The anomaly may represent the main magma pathway for the Dunedin Volcano and thus the centre of the volcano. A breccia facies with an oblate shape of approximately 1.3 * 2.5 km occurs within the inferred centre of the volcano at Port Chalmers (Benson Citation1968; Allen Citation1974; Price et al. Citation2003). This unit, the Port Chalmers Breccia, is dominated by phonolitic ash and lapilli tuff that encloses clasts up to > 1 m in diameter but more commonly < 20 cm. It is thought to represent the chaotic vent-fill to a large diatreme (Allen Citation1974; Price et al. Citation2003). The volcanic clasts are mainly phonolite but basanite, basaltic-trachyandesite, trachyandesite, syenite, gabbro, pyroxenite, hornblendite and fragments of Otago Schist and Cenozoic sedimentary rocks are also present (Price et al. Citation2003). The breccia was used in the mid to late 1800s as foundation stones for many Dunedin buildings, including the University Clock-tower and Geology buildings, but many stones now show deterioration due to breakdown of hydrothermal carbonate, clay and zeolite (Belton et al. Citation2013).
Outlying DVG centres and rocks
There are estimated to be at least 150 separate flows, dikes, sills, tuffs and vent-filling breccias in the outlying DVG (Coombs et al. Citation2008). These are too many to describe here and many remain to be fully characterised; some of the better-known examples are presented below.
Saddle Hill, Jaffray Hill and Scroggs Hill southwest of the Dunedin Volcano ( and D) and visible from the motorway are volcanic piles made of tuff and basaltic trachyandesite, phonotephrite and basanite lava emplaced through coal-bearing sediments and Cenozoic mudstone (Benson and Turner Citation1940). All three hills contain trachyandesite to phono-tephrite, although basanite is dominant on Saddle Hill. Phonolitic pyroclastic rock underlies lava at Scroggs Hill (Benson and Turner Citation1940) but there are no proximal phonolites and the source is unknown. Benson and Turner (Citation1940) also reported small areas of trachyte SE of these hills but these have not been subsequently documented. Historic underground coal mining is reported to have encountered the feeder pipe at Jaffray Hill (Benson and Turner Citation1940).
Southwest of the Dunedin Volcano, the most comprehensive studies have been on the western side of Lake Waihola () (Benson Citation1942b; Walls Citation1991). Here a stack of basanitic lavas at Waihola Hill and Waihola Quarry probably came from at least three vents (Walls Citation1991). The upper flow, which contains abundant but small fragments of peridotitic material, also encloses a ∼15 m thick sill of differentiated (or perhaps two phase-injection of) olivine theralite (nepheline gabbro) (Benson Citation1942b; Walls Citation1991). The Berwick diatreme is exposed in a disused quarry just north of Waihola (), where deposits are locally bedded and contain clasts of basalt, clinopyroxenite and peridotite, along with fragments of Otago Schist reaching > 1.5 m (Walls Citation1991). Basalts to the south and west of Lake Waihola were mapped by Bishop (Citation1994), who reported that they overlie Oligocene sedimentary rocks.
Rocks on the western and northwestern side of the field near Middlemarch () testify to phreatomagmatic eruptions. For example, the Crater is the spectacular high-level vent diatreme facies of a ∼1 km wide maar excavated through Otago Schist (E) (Coombs et al. Citation1986; Németh and White Citation2003). Other diatremes include: Foulden Maar, which had a ∼1.5 km wide crater lake that filled to > 100 m with a laminated diatomite that preserves a remarkable Miocene fossil and climate record (Lindqvist and Lee Citation2009; Fox et al. Citation2015; Kaulfuss Citation2017); Gladsmuir, in which poorly exposed basanite and pyroclastic rocks may represent the top of a diatreme; and a cluster of 4 buried maars at Hindon reaching up to 1000 m in diameter, aligned in a NE trend and containing up to 8 m of diatomite (Kaulfuss et al. Citation2018). Other eruptive centres in this area, such as Slip Hill, Bald Hill, Abbortsford, Mt Stoker and Fortification Peak, are basanitic lavas.
Some of the better-studied rocks occur at the remote Yellow Hill-Summer Hills area northwest of Dunedin (). Brown (Citation1964) describes this eruptive centre as comprising scoria, tuff and basanite overlying Eocene-Oligocene marine sediments, and inferred it to represent the products of at least one, but probably multiple, vents. To the NE, Mt Thompson and nearby peaks are basanitic lavas, whereas Ram Rock appears to be a ∼400 m diameter eroded basanitic pipe. The radiating columns at Ram Rock quarry contain abundant peridotite (McCoy-West et al. Citation2013; Scott et al. Citation2014b) and pyrometamorphosed Otago Schist xenoliths (Scanlan et al. Citation2020).
The Maniototo area in the NW of the province comprises the Haughton Hill, Kokonga, Swinburn and Waipiata basalts, with the publicly accessible results of the 2007 Glass Earth-Dunedin City Council aeromagnetic programme showing several shallow magnetic highs that may represent buried volcanic rocks (). Swinburn South (south side of the State Highway 85) comprises a pile of basaltic rocks that overlie scoria-fall deposits, which in turn overlie Eocene-Oligocene marine sediments (Giacalone Citation2018). It is unclear whether the remarkably coarse doleritic textures of most of the Swinburn South rocks formed in a sill or one or more lava flows (Giacalone Citation2018). At its southern end, the lavas exceed 100 m thickness and are cut by small diatremes. The basalt in this area contains rare xenoliths of peridotite, schist and now porcellanitised Cenozoic sediment. Swinburn North (mainly north of State Highway 85) comprises thin aphanitic basanitic lavas that overlie Miocene sediments (Youngson et al. Citation1998; Giacalone Citation2018). These lavas, which have subsequently been tilted and now mostly dip to the north and northeast, and a thick elongate belt of bedded pyroclastic rocks, are truncated by the Waihemo fault system (Bishop Citation1974). Basalt at Haughton Hill, which like Swinburn South has portions with doleritic textures, also overlies Miocene sediments. The Waipiata-Kokonga basanitic lava (or lavas?) remain little-studied (Donnelly Citation1996) despite the Waipiata flow apparently being one of the largest single flows outside of the Dunedin Volcano.
Flat Hill and Trig L in the Pigroot area () contain variably evolved rocks. Flat Hill comprises at least three vents with infills of scoria, tuff and basanite to phonotephrite; the vents cut Eocene-Oligocene marine sediments (Tenny Citation1977). The Trig L lavas also overlie marine sediments (Németh and White Citation2003) and include perhaps the most remarkable rock of the entire DVG: a mantle peridotite- and crustal gabbro xenolith-bearing phonolite that caps Trig L. This magma has been inferred to have fractionated at high-P before entraining the xenoliths (Wright Citation1966; Price and Green Citation1972; Irving and Price Citation1981), although this assumption is now less certain as experiments have shown that phonolite can be generated as a primary magma by melting of metasomatised spinel peridotite (Laporte et al. Citation2014).
There are several remote vents in the northwestern area of the Kakanui Range and north of the Waihemo Fault System (). Siberia Hill is the main eruptive centre and it comprises a stack of tuffs and lavas resting upon a thin veneer (∼40 m) of Eocene to Oligocene sediments atop the Otago Schist (Brown Citation1955; Rae Citation1990). The lowermost lavas are trachybasalt and the edifice appears to be capped by basanite (Rae Citation1990). Kattothyrst, 1 km to the east, is a columnar-jointed basanitic plug containing mantle xenoliths (Rae Citation1990; Hodgkinson Citation2013) whereas Mt Dasher, 1.5 km to the northwest of Kattothyrst, is a ∼100 m high stack of basanite lavas with an intervening phonotephrite flow reported from the NE side (Rae Citation1990). The Obelisk is reported to be trachybasalt at the southern end but basalt at the northern end (Coombs et al. Citation2008).
Lavas at Omimi to Karitane () on the coast north of the Dunedin Volcano range from basanite to phonolite (Coombs and Wilkinson Citation1969; McLeod and White Citation2018). Volcanic rocks are relatively abundant around Omimi, but form discrete units through the sedimentary pile from Brinns Point to Karitane (McLeod and White Citation2018).
DVG plutonic rocks
In-situ plutonic rocks across the entire DVG are restricted to syenites in the proximity of Hoopers Inlet on the Otago Peninsula (). Here, a nepheline syenite has been mapped beneath dolerite in a mine shaft on the eastern flank of Harbour Cone (Boult Citation1905). The syenite was once considered to be a source of gold on the peninsula (Boult Citation1905) but a re-investigation by Fleming (Citation2015) failed to find any gold. Nepheline syenite dikes occur on the Portobello-Hoopers Inlet road (Hoernle et al. Citation2006) but the best studied nepheline syenites are the clasts in the Port Chalmers Breccia (). In some cases, these clasts contain sodalite and eucolite (Allen Citation1974; Price et al. Citation2003). Clasts of gabbro also occur in the Port Chalmers Breccia (Price et al. Citation2003), Trig L phonolite in the Pigroot (Price and Wallace Citation1976; Beinlich et al. Citation2006), basanite at Yellow Hill-Summer Hills (Brown Citation1964) and Murdering Beach/Whareakeake. While these could represent deep-seated DVG components, zircon extracted from one Pigroot gabbro clast yielded a Late Cretaceous U-Pb age (Beinlich et al. Citation2006).
DVG mantle xenoliths and xenocrysts
There were at least 24 eruptions in the DVG that brought up spinel facies mantle peridotite xenoliths ( and ). These mainly are in the outlying rocks, with the only known Dunedin Volcano-hosted xenoliths being in tuff and lava at Taiaroa Head, the tephri-phonolite Murdering Beach/Whareakeake flow (McIntosh Citation1989) and in the lowermost exposed basanite flow on Swampy Summit (McDougall and Coombs Citation1973) (). The mineral and whole-rock geochemistry and isotopic compositions of DVG xenoliths have revealed the East Otago mantle lithosphere to comprise fertile (mainly lherzolitic) residues that experienced low to moderate degrees of melt extraction from at least Proterozoic and Mesozoic times (McCoy-West et al. Citation2013; Scott et al. Citation2014a, Citation2014b), followed by variable degrees of overprinting by carbonatitic fluids (Scott et al. Citation2014a, Citation2014b; McCoy-West et al. Citation2015, Citation2016; Scott et al. Citation2016a, Citation2016b; Dalton et al. Citation2017). Otago mantle metasomatism predates entrainment of the xenoliths (Dalton et al. Citation2017) and probably occurred in the Cretaceous at the time that long-lived regional subduction along the Zealandia portion of the Gondwana margin ceased (Scott et al. Citation2014b; McCoy-West et al. Citation2016; Scott et al. Citation2016a; van der Meer et al. Citation2017).
The best-studied mantle-derived xenocrysts are alluvial zircon grains in the Shag River in the north of the field and at Glenore in the south (), although in both cases their specific sources remain unknown. These grains reach 6 mm in diameter and have U-Pb dates of 12–20 Ma that overlap with the K/Ar and 40Ar/39Ar ages of DVG rocks (Sutherland and Meffre Citation2009; van der Meer et al. Citation2019). Trace element compositions and Hf isotopic ratios have been interpreted to indicate derivation from carbonatite-metasomatized mantle (van der Meer et al. Citation2019). Some 2–3 mm diameter blue corundum grains have been found at the same site as the Shag River zircons but have not been comprehensively studied and their source also remains unknown. Xenocrysts of kaersutite, anorthoclase, clinopyroxene and Ti-magnetite have been reported from the Murdering Beach/Whareakeake tephri-phonolite (McIntosh Citation1989) and basanites at Siberia Hill and Kattothyrst (Rae Citation1990; Hodgkinson Citation2013). Anorthoclase occurs as a xenocryst in the Port Chalmers Breccia (Price et al. Citation2003).
Age
A compilation of 91 dates, comprising 69 obtained by K/Ar and 22 by 40Ar/39Ar methods, indicates the DVG eruptions started in the Late Oligocene (∼25 Ma) and extended for 16 Myr until the middle Miocene (; Data Supplementary 1). It is difficult to assess the reliability of individual published ages without detailed field constraints or multiple dates from the same locations. Excess or loss of Ar is likely to be a widespread problem. For example, basalt from Haughton Hill gave a K/Ar date of 21 Ma (Adams Citation1981) but subsequent 40Ar/39Ar analysis has revealed high excess 40Ar and a feldspar separate yielded a crystallisation age of 10.9 ± 0.3 Ma (D. Lee, pers. comm 2019; ). There are unresolved age questions for at least three further locations. First, at the Siberia Hill eruptive centre (), the basal flow has a K/Ar age of 15.5 ± 0.3 Ma (Rae Citation1990) that is within error of a nearby 16.2 ± 1 Ma (K/Ar) flow at Mt Dasher 1.5 km away (Coombs et al. Citation2008). However, the top Siberia Hill flow has yielded a K/Ar age of 9.8 ± 0.2 Ma (Coombs et al. Citation2008). With little evidence for erosion between flows, the low 40Ar (24%) for the top flow suggests that its age may be an underestimate. Second, the oldest dated rock in the Dunedin Volcano is a trachybasalt dike (40Ar/39Ar; 16.0 ± 0.4 Ma) that intrudes peperite-bearing phonolite at Allans Beach (Hoernle et al. Citation2006). However, a glass separate reported as from the peperite yielded an 40Ar/39Ar date of 11.7 ± 0.1 Ma (Hoernle et al. Citation2006); either one of the dates is incorrect, the peperite may be from some location other than Allans Beach, or peperite formed at multiple times in this area. Third, the oldest dated rock at North Head near Aramoana is the trachyandesite ‘kaiwekite’ (Marshall Citation1914), which has a K/Ar age of 14.2 ± 0.3 Ma (Coombs et al. Citation2008). However, this flow is underlain by basalts (B) that are in turn underlain by trachyte at the old Harbour Board Quarry that has a K/Ar age of 13.1 ± 0.1 Ma (McDougall and Coombs Citation1973); either the trachyandesite has excess 40Ar or the trachyte has lost Ar. Nonetheless, there are examples where DVG K/Ar and 40Ar/39Ar dates agree. For example, three dates of a single phonolite sample from the summit of Mt Cargill (C) converge at 11 Ma (McDougall and Coombs Citation1973; Coombs et al. Citation2008; Timm et al. Citation2010). Similarly, K/Ar- and 40Ar/39Ar-dated basalts from the Waihola and Middlemarch areas are all > 20 Ma (Hoernle et al. Citation2006; Coombs et al. Citation2008), although the slightly older K/Ar ages for the same flows imply the presence of small amounts of excess 40Ar.
Figure 5. Summary of Dunedin Volcanic Group K/Ar and 40Ar/39Ar dates. Colours on this diagram correspond to . Data sources are 1, McDougall and Coombs (Citation1973), 2, Rae (Citation1990), 3, Youngson et al. (Citation1998), 4, Hoernle et al. (Citation2006), 5, Coombs et al. (Citation2008), 6, Timm et al. (Citation2010), 7, Fox et al. (Citation2015), 8, Kaulfuss et al. (Citation2018), 9, D Lee (pers comm), and are summarised in the Data Supplementary.
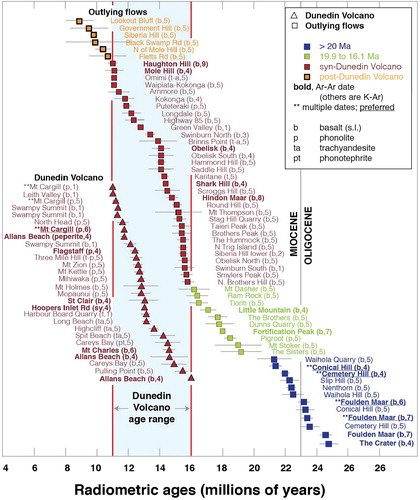
Although further 40Ar/39Ar dating will almost certainly refine DVG eruption ages, several broad patterns to magmatism can be stated with confidence. Firstly, volcanism appears to have begun in the Middlemarch and Lake Waihola areas at between 25 and 21 Ma ( and ) with the oldest rocks so far established being from The Crater (40Ar/39Ar = 24.8 ± 0.6 Ma; Hoernle et al. Citation2006). While there is an apparent age gap at 21–20 Ma, more precise dating is required to confirm this. Secondly, between 20 and 16 Ma, volcanism occurred as distributed small volume eruptions across the field ( and ). Thirdly, the Dunedin Volcano was active from 16 to 11 Ma, concurrent with volcanism elsewhere in the field (). The oldest Dunedin Volcano date is a 40Ar/39Ar age of 16.0 ± 0.4 Ma (Hoernle et al. Citation2006). This age is broadly consistent with the ∼15 Ma lavas in the Careys Bay-Pulling Point area (), which overlie basal ash-bearing sediments and hence the base of this portion of the volcano (Coombs et al. Citation1960a, Citation2008) ( and ). Although the youngest age for the Dunedin Volcano is often quoted as 10 Ma, the sample that yielded this K/Ar age has been established by 40Ar/39Ar methods to be 11 Ma, and so the 10 Ma age is not shown in to avoid confusion. Fourthly, the final phases of DVG magmatism (rocks dated as < 11 Ma in the Kakanui Range, west of Lake Waihola and at Lookout Bluff) should be considered provisional because the four youngest-dated rocks all yielded very little 40Ar (8–24%; Coombs et al. Citation2008).
Geochemical Characterisation
With a database comprising 559 samples compiled from University of Otago student theses, journal articles and our own new data, the total alkalis versus silica (TAS) classification diagram and the sodic/potassic variants definition of Le Maitre (Citation2002) provide a method for broadly describing DVG eruptive centres (A). Analytical methods for the new data are presented in Data Supplementary 2, and the reader is referred to the original sources for the methods related to the remaining data. The DVG analyses plot as a field of dominantly alkaline and variably fractionated rocks (A). The largest eruption centre, the 16–11 Ma Dunedin Volcano, extends from sodic and potassic basanite-basalt through to sodic phonolite and nepheline syenite and potassic trachyte (A). CIPW normative calculations following Le Maitre (Citation2002) indicate that most DVG rocks are undersaturated with respect to SiO2 (). Notable outliers are a peridotite xenolith-bearing tephri-phonolite flow at Murdering Beach/Whareakeake, which is strongly alkaline compared to most DVG rocks of equivalent SiO2 (A) and Mg# (), and the trachytes, which extend to high SiO2 (∼69 wt%) and are quartz-normative (). The range of silica saturation at high Mg# is thought to have controlled multiple fractionation lineages in the Dunedin Volcano (Coombs and Wilkinson Citation1969; Price and Taylor Citation1973; Price and Chappell Citation1975).
Figure 6. Total alkalis versus SiO2 (anhydrous, normalised to 100%) for 559 Dunedin Volcano Group samples. Note that basanite and tephrite are collectively classified here as basanite since it is not practical to identify the amount of modal olivine in most samples. B shows outlying flows more distal to the Dunedin Volcano compared to those shown in C.
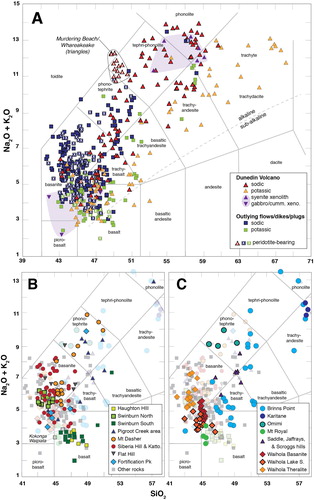
Figure 7. CIPW normative quartz (qz) and nepheline (ne) components versus whole rock Mg#. The diagram reveals how most DVG rocks are nepheline normative. Samples with bold black outlines plotting on the grey vertical line are hypersthene normative.
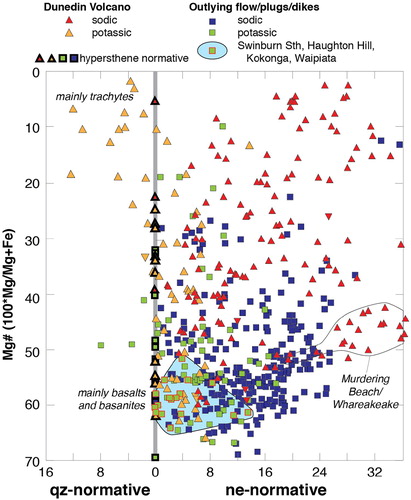
In contrast to rocks of the Dunedin Volcano, the outlying lavas are dominantly basanitic and sodic (A). These basanitic lavas also appear to be, on average, slightly more alkaline at equivalent SiO2 than those of the Dunedin Volcano (B)–as was first noted by Reay et al. (Citation1991). The TAS discrimination plots also reveal spatial geochemical differences between some of the outlying eruption centres (B, C). In the Maniototo area in the northwest of the field, for example, rocks at Haughton Hill, Kokonga, Swinburn South and Waipiata () are potassic basalts with distinctly lower alkali contents than nearby basanites at Swinburn North, Pigroot Creek, Siberia Hill, Kattothyrst or Mt Dasher (B and ). Some outlying eruptive centres have rocks that are slightly to moderately evolved; for example, basanites and phono-tephrites occur at Siberia Hill, Kattothyrst and Mt Dasher, all within 1.5 km of each other (B). The volcanic rocks around Waihola are mainly Si-deficient basanites whereas the nearby (but younger; ) Saddle Hill, Jaffray Hill and Scroggs Hill lavas have more Si-rich compositions (C). The Omimi-Brinns Point-Karitane rocks, described in detail by McLeod and White (Citation2018), represent a fractionated suite that extends to phonolite. Karitane and Trig L in the Pigroot are the only known localities where phonolitic rocks occur outside the Dunedin Volcano.
Of the 559 whole rock samples, comprehensive suites of trace element analyses have been determined for only 52 samples, of which 32 are from the Dunedin Volcano and 20 are from the outlying rocks (A, B; Supplementary File 2). The normalised DVG trace element patterns for mafic rocks closely resemble those of ocean island basalt (OIB). The negative K anomalies could represent hydrous K-bearing phases such as amphibole or phlogopite in their sources. Dunedin Volcano phonolites, their syenitic plutonic equivalents and tephri-phonolitic rocks contain higher concentrations of large iron lithophile and rare earth elements (REE) but larger P and Ti anomalies compared to the basanites at this eruptive centre (A). The prominent P and Ti anomalies are likely the result of depletion of the magma by earlier precipitation of apatite and Fe-Ti oxides, respectively. This same mafic versus felsic trace element pattern can broadly be seen in the outlying lavas, except that two phono-tephrites from Trig L contain lower heavy REE and higher Sm and Eu compared to Dunedin Volcano phonolites (B). The distinct difference in the HREE budgets may mean that the Trig L phonolites underwent fractionation at higher P than the Dunedin Volcano phonolites, with residual garnet, clinopyroxene or amphibole having affected the melts (Price and Green Citation1972). Among the outlying rocks, the potassic flows from Haughton Hill, Kokonga, Swinburn South and Waipiata are characterised by lower trace element abundances when compared to the rest of the suite, although the data are incomplete for Kokonga, Haughton Hill and Waipiata.
Figure 8. Primitive mantle normalised diagram of samples from the Dunedin Volcano (A) and the outlying flows (B). Normalising values and OIB composition are from Sun and McDonough (Citation1989).
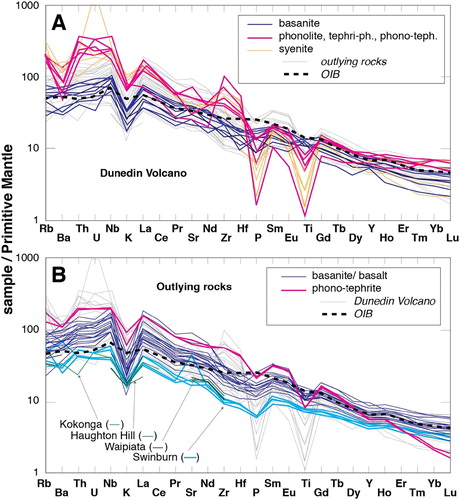
Sr-Nd-Pb-Hf-Mg-He Isotopic Data
In addition to the Sr isotope data of Price and Compston (Citation1973) and the Sr-Nd-Pb ± Hf datasets of Price et al. (Citation2003), Hoernle et al. (Citation2006), Sprung et al. (Citation2007), Timm et al. (Citation2010) and Scanlan et al. (Citation2020), Sr-Nd-Pb-Mg isotopes are here presented for 29 DVG rocks (Data Supplementary 3). The new data have mostly been obtained from locations known to have peridotite xenoliths since these magmas and their xenolith cargo should have rapidly ascended from the mantle with minimal crustal interaction. The possible role of crustal contamination is assessed through new Nd and Pb isotope data for 4 Caples Terrane and 3 Rakaia Terrane Otago Schist rocks, complemented by the Sr isotope data already reported by Scanlan et al. (Citation2018). With the exception of the volcanic rocks at Waihola, which were erupted through the Caples Terrane, most of the DVG magmas for which isotope data are available were emplaced through the Rakaia Terrane (). For this reason, our assimilation-fractionation-crystallisation models adopt average end-member isotopic and element abundances for the Rakaia Terrane and for the peridotite-bearing suite of rocks, excluding the fractionated Trig L and Murdering Beach flows. When the data are coupled with the recently acquired Sr-Nd ± Pb ± Hf ± Mg peridotite xenolith datasets (Scott et al. Citation2014a, Citation2014b; McCoy-West et al. Citation2016; Scott et al. Citation2016a; Dalton et al. Citation2017; Wang et al. Citation2016; this study), potential mantle reservoirs can be evaluated. Given the uncertainty in the quality of the eruption ages, Sr, Nd and Hf data are mostly age-corrected to 15 Ma for the outlying flows. Changing the eruption age by 5 million years makes essentially no difference to most of these analyses because of their low Rb/Sr, Sm/Nd and Lu/Hf. On the other hand, Dunedin Volcano rocks are corrected to between 15 and 11 Ma, depending on their K/Ar or 40Ar-39Ar age because the evolved components have Rb/Sr up to 94, making them very sensitive to the age chosen. There is insufficient quality U, Th and Pb data to age-correct Pb isotopes, and so only measured values are presented.
The outlying rocks of DVG and the mafic ones of the Dunedin Volcano mainly plot in fairly restricted Sr-Nd isotope space, clustering at 87Sr/86Sr(i) = 0.7029–0.7030 and 143Nd/144Nd(i) = 0.51285–0.51292 (A). This field can be seen to overlap the field for metasomatised mantle peridotite. An exception to the main DVG field is the Kokonga, Haughton Hill, Swinburn South and Waipiata cluster (), which extends to more radiogenic Sr. The Swinburn South sample with the most radiogenic Sr (0.70548) corresponds to an anomalous whole-rock Sr abundance (B); it also has carbonate present may therefore have experienced some post-magmatic crustal Sr addition. However, the other flows display no such anomalies and share very similar trace element abundances (B). Therefore, these rocks are distinguished from others in the DVG by their potassic basaltic compositions ( and ), their low trace element abundances () and by their Sr isotope ratios. Many Dunedin Volcano phonolitic and trachytic rocks have high 87Rb/86Sr and measured 87Sr/86Sr (up to 0.71872; Price and Compston Citation1973) that when age-corrected yield values slightly higher (∼ 0.7040) than most of the more mafic components (A). Although the more radiogenic Sr could be due to imprecise dates and therefore an incorrect age correction, the 0.70428 value from a low Rb/Sr anorthoclase from one trachyte means that some of the evolved magmas experienced crustal contamination or were derived from a slightly more radiogenic Sr source than most studied components. While assimilation of crustal components has occurred in some cases (e.g. Scanlan et al. Citation2020), the elevated DVG Sr and Nd concentrations compared to the Otago Schist make evidence for crustal assimilation extremely difficult to see in 87Sr/86Sr and 143Nd/144Nd space (A inset).
Figure 9. Summary of Dunedin Volcanic Group Sr-Nd-Pb-Hf-Mg isotopic data. The DVG data are from Price and Compston (Citation1973), Price et al. (Citation2003), Hoernle et al. (Citation2006), Timm et al. (Citation2009), Scanlan et al. (Citation2020) and this study; Otago Schist data are from this study and Scanlan et al. (Citation2018); peridotite data are from Scott et al. (Citation2014a, Citation2014b), McCoy-West et al. (Citation2016), Dalton et al. (Citation2017) and this study; megacrystic zircon are from van der Meer et al. (Citation2019); Mg isotope data are from Wang et al. (Citation2016) and this study. The AFC trends were calculated using average basanitet and Otago Schist compositions, partition coefficients for Sr, Nd and Pb from Adam and Green (Citation2006) and r values of 0.2.
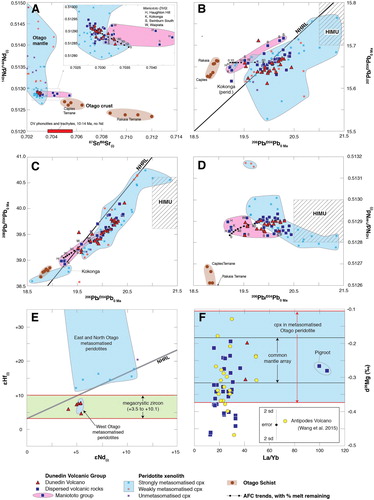
Pb isotopes appear to show subtle source variations to DVG components. On a 207Pb/204Pb versus 206Pb/204Pb plot, most data fall between 206Pb/204Pb = 19.5 and 20.4 and overlap with the main metasomatised peridotite field (B). Clear exceptions to this are the Kokonga, Haughton Hill, Swinburn South and Waipiata potassic basalts, which outline an array of elevated 207Pb/204Pb for a given 206Pb/204Pb (B). Samples from these four locations also extend to less radiogenic 208Pb/204Pb and 206Pb/204Pb values than the main DVG population (C). The less radiogenic portion of this suite does fall close to the AFC trends for crustal contamination, but (1) three clinopyroxene separates from a metasomatised peridotite xenolith from Kokonga shows that less radiogenic Pb components do exist in the underlying mantle; and (2) these Pb isotopic values would require unrealistically large amounts of assimilation (B, C, D). In Cretaceous Zealandia volcanic rocks where the elevated 207Pb/204Pb has been discussed, the cause is inferred to be derivation from an ancient source embedded in the mantle (van der Meer et al. Citation2017). The DVG suite plots along a positive trajectory towards the HIMU field of Stracke et al. (Citation2005) but none of the rocks have the extreme radiogenic 206Pb/204Pb and 207Pb/204Pb ratios of that mantle reservoir (B, C, D).
Hf isotopes have been measured for only 6 lavas from the Dunedin Volcano and 11 alluvial megacrystic zircons from the Shag River. The lavas cluster at εHfi = +6 to + 7.7 (Sprung et al. Citation2007; Timm et al. Citation2010) whereas the zircons overlap but have a wider range (εHfi = +3.5 to + 10.1) (E) (van der Meer et al. Citation2019). Unlike the Sr-Nd-Pb data, the Hf isotopes do not overlap with the clinopyroxene (εHf > +14) from variably metasomatised peridotite xenoliths (Scott et al. Citation2014b); however, the DVG basalts and zircons do overlap with compositions of strongly metasomatised peridotites in Oligocene-Miocene alkaline lamprophyres in West Otago (εHf = +5 to + 9; Scott et al. Citation2016a) (E).
Magnesium isotopes for DVG rocks have been measured only from the peridotite-bearing basalts (this study). The data have a relatively wide range (δ26Mg = −0.20 to −0.47) that extends to values lighter than the common mantle field (δ26Mg = −0.25 ± 0.07) (F). There is no correlation with La/Yb, SiO2 content or Sr-Nd-Pb-Hf isotope ratio. The intraplate Antipodes Islands on the eastern side of Zealandia (F) (Scott et al. Citation2013) also have an isotopically light source, and Zealandia peridotite xenolith δ26Mg data also extent to light values but are not as extreme as the basalts (Wang et al. Citation2016).
3He/4He ratios of olivine phenocrysts from DVG components have an average Ra = 7.7 (Hoke et al. Citation2000) that falls within the range of MORB mantle (Ra = 8 ± 1.5). That study also found that gas emissions from a spring in the Taieri Basin have anomalously high CO2 of 83–88% and interpreted these values to represent outgassing from mantle-derived magma added to the lower crust.
Discussion
Origin of the DVG
The data presented above have illustrated the spatial, temporal and chemical ranges of DVG rocks. However, an outstanding question is: what were the mantle sources and what caused them to melt? The lack of a systematic age progression across the province, despite the Otago lithosphere having moved ∼ 870 km northwest through the 16 Myr period of eruption, as calculated using the rotation poles and moving hotspot reference frame of Seton et al. (Citation2012) (Data Supplementary 4), means that a fixed plume driving volcanism is not a viable mechanism. The main models for Zealandia intraplate volcanism instead involve either decompression melting of asthenosphere (Hoernle et al. Citation2006), melting of a veined mantle lithosphere (Panter et al. Citation2006) or a combination of both (Sprung et al. Citation2007). Lateral lithosphere edge convection may possibly have induced mantle melting to feed some Zealandia intraplate volcanoes (Gamble et al. Citation2018) but DVG rocks were not erupted close to the edge of the continent and there is little evidence for a variation in Otago lithosphere thickness (e.g. as indicated by surface topographic variation) in the Oligocene-Miocene.
In the decompression model, the main source of the DVG magmas is heterogeneous asthenosphere deep under Zealandia (Hoernle et al. Citation2006; Timm et al. Citation2009, Citation2010). The detachment of garnet-bearing peridotite lithosphere blocks at the lithosphere-asthenosphere boundary as a result of gravitational instabilities (e.g. Conrad and Molnar Citation1997) is predicted to have first caused carbonated ancient (>1 Ga) pyroxenite residing deep in the asthenosphere to decompress and melt, followed by lower P melting of decompressing carbonated peridotite (Hoernle et al. Citation2006; Timm et al. Citation2009, Citation2010). Mixing of these melts is interpreted to account for the isotopic, major and trace element geochemistry of the DVG rocks (Hoernle et al. Citation2006; Timm et al. Citation2010), since pyroxenite by itself is insufficient to generate the major element abundances of most intraplate basalts (Pilet Citation2015). While the 207Pb/204Pb of some Zealandia basalts proximal to the Hikurangi Plateau could point to an ancient source, the majority of the Zealandia basalts lack this Pb isotope signature and the radiogenic 206Pb/204Pb and 208Pb/204Pb values could have been generated by rapid ingrowth in Cretaceous-Cenozoic metasomatised mantle (McCoy-West et al. Citation2016; van der Meer et al. Citation2017). Either way, the amount of magma generated in the decompression model relies on the amount and geometry of material delaminated because the volume of partial melting will be governed by the amount of asthenosphere decompressed across peridotite and pyroxenite solidi. Large volumes of volcanic rock formed by low degrees of melting, such as the Dunedin Volcano, would require very large amounts of lithosphere detachment. A large lithosphere thickness change could potentially yield significant crustal uplift in response to isostatic re-equilibration. Given the distance travelled by the Zealandia lithosphere during the time of DVG eruption (∼870 km), the decompression model would require a laterally vast asthenosphere source with little chemical and isotopic variation stretching over thousands of km.
The alternative to the decompression model is the veined lithosphere model. The large negative anomalies in K, and, to a lesser degree, Ba, Pb and Ti anomalies in primitive magmas should represent residual amphibole ± phlogopite in the magma sources (e.g. Foley Citation1992; Class and Goldstein Citation1997; Finn et al. Citation2005; Panter et al. Citation2006). High temperature experiments conducted at 1.5 GPa on amphibole surrounded by peridotite–simulating melting of hydrous veins or ‘metasomes’ in the mantle–can generate alkaline basaltic melts with OIB-like trace elements patterns (Pilet et al. Citation2008). Since the commonly documented mantle amphibole, F-free pargasite, is stable at ∼ < 1100°C (e.g. Green Citation2015) and phlogopite is restricted to ∼ < 1200°C (e.g. Luth Citation2014), such magmas must be derived from the lithospheric mantle. The occurrence of such metasomes would require widespread enrichment of the mantle lithosphere, which Finn et al. (Citation2005), Panter et al. (Citation2006) and van der Meer et al. (Citation2017) suggest occurred in the Cretaceous as a result of termination of subduction along the Gondwana margin. The unusually light δ26Mg of the DVG is consistent with a source that has interacted with, or was derived from, subducted carbonate (Wang et al. Citation2016), although these isotopes provide no insight into whether that carbonate was young or old. In the veined lithosphere model, the process of intermittent and dispersed intraplate melting of the metasomes would need to be triggered by conductive heating from the asthenosphere as a result of delamination or lithosphere thinning, or by injection of batches of hotter magma into adjacent lithosphere (e.g. Pilet Citation2015). The extent of the thermal perturbation controls the volume of magma generated.
The asthenosphere decompression versus veined lithosphere models are difficult to test because both rely on interpretations drawn from the products of melting as potential source regions are far too deep to physically examine. While any model for the origin of DVG rocks must be consistent with the spatial and temporal distribution of magmatism ( and ) and with the dominantly alkaline geochemistry (), it now must also explain the similarity in Sr-Nd-Pb but not Hf isotopes to East Otago carbonatite-metasomatised lithospheric mantle (). The length-scales of element diffusion in pyroxene in the mantle xenoliths that were affected by carbonatitic fluids (Scott et al. Citation2014a, Citation2014b; McCoy-West et al. Citation2015, Citation2016; Scott et al. Citation2016a, Citation2016b) require enrichment to have occurred millions of years before xenolith entrainment (Dalton et al. Citation2017). The radiogenic Hf isotopes in the metasomatised East Otago mantle xenolith suite may indicate that the carbonatitic fluids were low in Hf and unable to completely overprint pre-existing peridotite Hf isotopes (e.g. Bizimis et al. Citation2003; Shaw et al. Citation2007; Scott et al. Citation2014b). In contrast, metasomatism of the more refractory West Otago mantle (with less pre-existing Hf), which yielded εHf + 5 to + 8 (Scott et al. Citation2016a), probably more accurately records the carbonatitic fluid Hf isotopic composition; the West Otago metasomatised mantle xenoliths overlap with the ratios in DVG rocks and megacrystic zircons (F). The carbonatitic fluids that enriched large areas of the Zealandia lithospheric mantle therefore had a very similar, if not identical, Sr-Nd-Pb and Hf isotope composition to the intraplate basalts.
Carbonatitic metasomatised mantle could melt to yield a Zealandia intraplate alkaline basalt with the appropriate trace element composition (e.g. Scott et al. Citation2016a) because carbonatitic magmas can precipitate amphibole and phlogopite during mantle metasomatism (Yaxley et al. Citation1998). Since the formation of hydrous minerals requires a high fluid to wall rock ratio, these metasomatically grown minerals should have an isotopic signature the same or very similar to the metasomatic fluid. A geothermal gradient of ∼ 75 mW m−2 for the Otago lithosphere (Scott et al. Citation2014a, Citation2014b) means that the seismic Moho would have been at ∼ 30 km depth and ∼700°C, the base of the lithosphere (where the geotherm intersects a mid-ocean ridge adiabat (∼1350°C)) was at ∼66 km, and the thermal maxima for pargasite and phlogopite would be attained in the middle lithosphere at ∼55 km and ∼63 km depth, respectively (). Destabilisation of these putative middle-lithosphere hydrous phases would occur via a thermal perturbation, with the resulting melt rising as an intraplate mafic magma with a geochemical and isotopic composition comparable to the DVG rocks. The reason why no DVG basalt has the radiogenic Nd and Hf isotopic composition of weakly metasomatized or unmetasomatised mantle peridotite () could be because these anhydrous domains did not pass their solidi. Furthermore, if the DVG melts were sourced from the middle lithosphere, then the absence of xenoliths of lower lithosphere garnet peridotite or carbonate-bearing metasomatized mantle, which should both be present in the Zealandia lower lithosphere (), can be accounted for by being beneath the melt sources. A key issue yet to be resolved is that while the majority of inspected peridotite xenoliths from the East Otago mantle are carbonatite-metasomatised, the occurrence of amphibole and/or phlogopite is restricted to only a very few. However, some xenoliths in the Alpine Dike Swarm in West Otago do contain veins comprising amphibole-clinopyroxene-phlogopite-apatite-ilmenite that could be exhumed metasomes (Brodie and Cooper Citation1989; Cooper Citation2020; Scott et al. Citation2014a).
Figure 10. Summary of the geotherm and possible lithosphere configuration underlying the Dunedin Volcanic Group. The peridotite + carbonate solidus is from Green and Wallace (Citation1988), the spinel-garnet transition in lherzolite is from Klemme and O'Neill (Citation2000) (and would be displaced down in pressure if the mantle composition was more depleted than lherzolite), the lherzolite dry solidus is from Katz et al. (Citation2003), the geotherm is from Hasterok and Chapman (Citation2011), phlogopite is from Luth (Citation2014) and the pargasite stability is from Green (Citation2015).
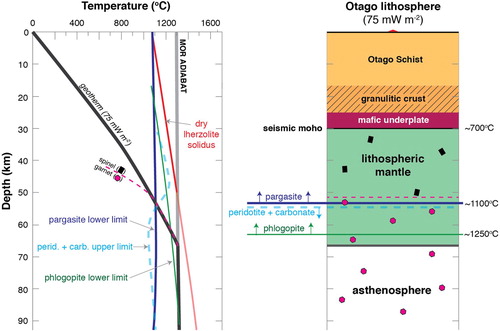
The DVG rock compositions and isotopes are not unique. In Zealandia, geochemically and isotopically similar alkaline volcanic rocks span 100 million years, from the < 0.5 Myr in the Auckland Volcanic Field (Needham et al. Citation2011) and Antipodes Volcano (Scott et al. Citation2013), though the Cenozoic (Timm et al. Citation2010), to the 100 Ma alkaline parts of the Westland Dike Swarm (van der Meer et al. Citation2017). Furthermore, volcanic rocks with a similar age range and composition have erupted though the once contiguous West Antarctica (e.g. Panter et al. Citation2018) and Australian (e.g. Zhang et al. Citation2001; Price et al. Citation2014) continental crust. These correlations mean that the process of continental intraplate magma generation in the SW Pacific is likely one that has been repeated (e.g. Finn et al. Citation2005). Since all three continental areas rapidly separated yet none of the volcanic provinces display age or geochemical trends, and because most of the intervening ocean basin seamounts and oceanic crust have MORB-like isotopic compositions (e.g. Mortimer et al. Citation2012; Park et al. Citation2019), it seems most likely that the continental intraplate mantle source has migrated with the migrating lithosphere blocks. One exception may be the Marie Byrd Seamounts just off the West Antarctic margin, which Kipf et al. (Citation2014) assign to having been derived from lithospheric mantle translated from the continental margin into the adjacent oceanic lithosphere and then melted. In our view, the available geochemical and isotopic evidence from volcanic rocks and their mantle cargoes currently lends more support to a lithospheric mantle than to an asthenospheric source for Zealandia’s alkaline intraplate magmas.
Conclusions
Rocks of the Dunedin Volcanic Group (DVG) represent one of the largest (>7,800 km2) and longest-lived (16 million years) of the many intraplate volcanic provinces in Zealandia. The DVG rocks are mainly alkaline, with the majority of components being silica-undersaturated basanites. Fractionation to phonolite has occurred in several of the centres, including the large 16–11 Ma Dunedin Volcano. The DVG mantle reservoirs show slight isotopic variations but tend to have unradiogenic Sr and radiogenic Nd, Hf, and Pb isotopes. Although there is little consensus in the literature on asthenosphere versus lithospheric mantle sources to the DVG magmas, the compiled major element, trace element and isotopic data could reflect a two-stage process in which the Zealandia mantle lithosphere was first enriched by asthenosphere-derived fluids with the resulting hydrous lithospheric mantle components subsequently destabilised to generate OIB-like alkaline magmas. A lithospheric mantle source would account for why the DVG shows no spatial age or geochemical progression despite erupting while the Otago lithosphere moved ∼870 km northwest relative to the asthenosphere in the late Cenozoic, as well as why there is a distinct lack of lower lithosphere mantle xenoliths.
DS4 - plate motion
Download MS Excel (2.5 MB)DS3 - isotopes
Download MS Excel (59.6 KB)DS2 - whole rock
Download MS Excel (156.6 KB)DS1 - dates
Download MS Word (108.7 KB)Acknowledgments
This work benefitted massively from the vast amounts of work done by previous staff and students at University of Otago, particularly: N Benson, D Coombs, K Donnelly, P McIntosh, U Martin, R Price, A Rae, A Reay and D Walls. Haughton Hill unpublished age data were provided by D Lee (U. Otago), F-Z Teng (U. Washington) provided Mg isotope data, and the Geology Department funded acquisition of new isotope and whole rock analyses. A Cooper and F-Z Teng commented on drafts, N. Mortimer calculated the late Cenozoic lithosphere movement, and J Gamble, Q van der Meer and T Waight critically reviewed the manuscript.
Disclosure statement
No potential conflict of interest was reported by the authors.
Notes
1 Some volcanic rock names described in this paper differ from those in original texts due to adoption of TAS nomenclature of Le Maitre (Citation2002).
References
- Adam J, Green T. 2006. Trace element partitioning between mica-and amphibole-bearing garnet lherzolite and hydrous basanitic melt: 1. Experimental results and the investigation of controls on partitioning behaviour. Contributions to Mineralogy and Petrology. 152(1):1–17. doi: 10.1007/s00410-006-0085-4
- Adams CJ. 1981. Migration of late Cenozoic volcanism in the South Island of New Zealand and the Campbell Plateau. Nature. 294:153–155. doi: 10.1038/294153a0
- Allen JM. 1974. Port Chalmers Breccia and adjacent early flows of the Dunedin volcanic complex at Port Chalmers. New Zealand Journal of Geology and Geophysics. 17:209–223. doi: 10.1080/00288306.1974.10428484
- Baxter RJM. 2019. Petrogenesis, eruption and emplacement of the volcanic rocks at Otapahi, Otago Peninsula [MSc thesis]. University of Otago.
- Beinlich A, Palin JM, Cooper AF. 2006. Accessory mineral U-Pb Ti-Zr thermochronology of the deep crust of Zealandia: rift, break-up and drift from 90–20 Ma. American Geophysical Union meeting 2006AGUFM.V31B0581B.
- Belton AD, Scott JM, Lee DE. 2013. Deterioration of Port Chalmers Breccia Building Stone in Otago. Report for the New Zealand Historic Places Trust: 42p.
- Benson WN. 1941. The basic igneous rocks of eastern Otago and their tectonic environment, Part 1. Transactions of the Royal Society of New Zealand. 71:208–222.
- Benson WN. 1942a. The basic igneous rocks of eastern Otago and their tectonic environment, Part II. Transactions of the Royal Society of New Zealand. 72:85–118.
- Benson WN. 1942b. The basic igneous rocks of eastern Otago and their tectonic environment, Part III. The olivine theralite of Waihola, East Otago, a gravitationally differentiated sill, with notes on related rocks. Transactions of the Royal Society of New Zealand. 72:160–185.
- Benson WN. 1968. Dunedin District. 1:50,000. NZ Geological Survey Miscellaneous Series Map 1. Wellington, New Zealand Department of Scientific and Industrial Research.
- Benson WN, Turner FJ. 1940. Mugearites in the Dunedin district. Transactions of the Royal Society of New Zealand. 70:188–199.
- Bishop DG. 1974. Tertiary and early Quaternary geology of the Naseby and Kyeburn areas, Central Otago. New Zealand Journal of Geology and Geophysics. 17:19–39. doi: 10.1080/00288306.1974.10428474
- Bishop DG. 1994. Geology of the Milton area: Sheets H45BD & Part I45, Scale 1: 50000. Institute of Geological & Nuclear Sciences.
- Bizimis M, Salters VJ, Dawson JB. 2003. The brevity of carbonatite sources in the mantle: evidence from Hf isotopes. Contributions to Mineralogy and Petrology. 145:281–300. doi: 10.1007/s00410-003-0452-3
- Boult CN. 1905. The occurrence of gold at Harbour Cone. Transactions of the Royal Society of New Zealand. 38:425–446.
- Brodie CG, Cooper AF. 1989. Nodule associations from ouachitite and camptonite lamprophyres, western Otago and South Westland, New Zealand. Special Publication of the Geological Society of Australia. 14:545–559.
- Brown DA. 1955. The geology of Siberia Hill and Mount Dasher, North Otago. Transactions of the Royal Society of New Zealand. 83:347–372.
- Brown EH. 1964. The geology of the Mt Stoker area, Eastern Otago: Part 2—post-metamorphic geology and volcanic petrology. New Zealand Journal of Geology and Geophysics. 7:192–204. doi: 10.1080/00288306.1964.10420170
- Class C, Goldstein SL. 1997. Plume-lithosphere interactions in the ocean basins: constraints from the source mineralogy. Earth and Planetary Science Letters. 150:245–260. doi: 10.1016/S0012-821X(97)00089-7
- Conrad CP, Molnar P. 1997. The growth of Rayleigh—taylor-type instabilities in the lithosphere for various rheological and density structures. Geophysical Journal International. 129:95–112. doi: 10.1111/j.1365-246X.1997.tb00939.x
- Coombs DS, Adams CJ, Roser BP, Reay A. 2008. Geochronology and geochemistry of the Dunedin volcanic group, eastern Otago, New Zealand. New Zealand Journal of Geology and Geophysics. 51:195–218. doi: 10.1080/00288300809509860
- Coombs DS, Cas RA, Kawachi Y, Landis CA, McDonough WF, Reay A. 1986. Cenozoic volcanism in North, East and Central Otago. Royal Society of New Zealand Bulletin. 23:278–312.
- Coombs DS, White AJR, Hamilton D. 1960a. Age relations of the Dunedin volcanic complex and some paleogeographic implications—part I. New Zealand Journal of Geology and Geophysics. 3:325–336. doi: 10.1080/00288306.1960.10423605
- Coombs DS, White AJR, Hamilton D, Couper RA. 1960b. Age relations of the Dunedin Volcanic Complex and some paleogeographic implications—part II. New Zealand Journal of Geology and Geophysics. 3:572–579. doi: 10.1080/00288306.1960.10420145
- Coombs DS, Wilkinson JFG. 1969. Lineages and fractionation trends in undersaturated volcanic rocks from the East Otago volcanic province (New Zealand) and related rocks. Journal of Petrology. 10:440–501. doi: 10.1093/petrology/10.3.440
- Cooper AF. 2020. Petrology and petrogenesis of an intraplate alkaline lamprophyre-phonolite-carbonatite association in the Alpine Dyke Swarm, New Zealand. New Zealand Journal of Geology and Geophysics. in press.
- Dalton HB, Scott JM, Liu J, Waight TE, Pearson DG, Brenna M, le Roux PJ, Palin JM. 2017. Diffusion-zoned pyroxenes in an isotopically heterogeneous mantle lithosphere beneath the Dunedin Volcanic Group, New Zealand, and their implications for intraplate alkaline magma sources. Lithosphere. 9:463–475. doi: 10.1130/L631.1
- Donnelly KE. 1996. The Waipiata volcanic formation [MSc thesis]. University of Otago.
- Finn CA, Müller RD, Panter KS. 2005. A Cenozoic diffuse alkaline magmatic province (DAMP) in the southwest Pacific without rift or plume origin. Geochemistry, Geophysics, Geosystems. 6:26. doi: 10.1029/2004GC000723
- Fleming JA. 2015. Characterisation of gold bearing lithologies, Battery Creek, Otago Peninsula, New Zealand [BSc Honours thesis]. University of Otago. 87p.
- Foley S. 1992. Vein-plus-wall-rock melting mechanisms in the lithosphere and the origin of potassic alkaline magmas. Lithos. 28:435–453. doi: 10.1016/0024-4937(92)90018-T
- Forsyth PJ. 2001. Geology of the Waitaki area. Institute of Geological & Nuclear Sciences 1:250 000 Geological Map 19. 1 sheet + 64 p. Lower Hutt, New Zealand, Institute of Geological & Nuclear Sciences Ltd.
- Fox BR, Wartho J, Wilson GS, Lee DE, Nelson FE, Kaulfuss U. 2015. Long-term evolution of an Oligocene/Miocene maar lake from Otago, New Zealand. Geochemistry, Geophysics, Geosystems. 16:59–76. doi: 10.1002/2014GC005534
- Gamble JA, Adams CJ, Morris PA, Wysoczanski RJ, Handler M, Timm C. 2018. The geochemistry and petrogenesis of Carnley Volcano, Auckland Islands, SW Pacific. New Zealand Journal of Geology and Geophysics. 61:480–497. doi: 10.1080/00288306.2018.1505642
- Giacalone E. 2018. Swinburn volcanic Complex, East Otago. New Zealand [PhD thesis]. University of Otago. 220p.
- Godfrey NJ, Davey F, Stern TA, Okaya D. 2001. Crustal structure and thermal anomalies of the Dunedin region, South Island, New Zealand. Journal of Geophysical Research: Solid Earth. 106:30835–30848. doi: 10.1029/2000JB000006
- Gorman AR, Hill MG, Orpin AR, Koons PO, Norris RJ, Landis CA, Allan TMH, Johnstone T, Gray FL, Wilson D, Osterberg EC. 2013. Quaternary shelf structures SE of the South Island, imaged by high-resolution seismic profiling. New Zealand Journal of Geology and Geophysics. 56:68–82. doi: 10.1080/00288306.2013.772906
- Gray DR, Foster DA. 2004. 40Ar/39Ar thermochronologic constraints on deformation, metamorphism and cooling/exhumation of a Mesozoic accretionary wedge, Otago Schist, New Zealand. Tectonophysics. 385:181–210. doi: 10.1016/j.tecto.2004.05.001
- Green DH. 2015. Experimental petrology of peridotites, including effects of water and carbon on melting in the Earth’s upper mantle. Physics and Chemistry of Minerals. 42:95–122. doi: 10.1007/s00269-014-0729-2
- Green DH, Wallace ME. 1988. Mantle metasomatism by ephemeral carbonatite melts. Nature. 336:459–462. doi: 10.1038/336459a0
- Hasterok D, Chapman DS. 2011. Heat production and geotherms for the continental lithosphere. Earth and Planetary Science Letters. 307:59–70. doi: 10.1016/j.epsl.2011.04.034
- Hodgkinson A. 2013. Mantle xenoliths and metasomatism at Kattothyrst, north Otago [BSc Honours thesis]. University of Otago. 118p.
- Hoernle K, White JDL, van den Bogaard P, Hauff F, Coombs DS, Werner R, Timm C, Garbe-Schönberg D, Reay A, Cooper AF. 2006. Cenozoic intraplate volcanism on New Zealand: Upwelling induced by lithospheric removal. Earth and Planetary Science Letters. 248:350–367. doi: 10.1016/j.epsl.2006.06.001
- Hoke L, Poreda R, Reay A, Weaver SD. 2000. The subcontinental mantle beneath southern New Zealand, characterised by helium isotopes in intraplate basalts and gas-rich springs. Geochimica et Cosmochimica Acta. 64:2489–2507. doi: 10.1016/S0016-7037(00)00346-X
- Irving AJ, Price RC. 1981. Geochemistry and evolution of Iherzolite-bearing phonolitic lavas from Nigeria, Australia, East Germany and New Zealand. Geochimica et Cosmochimica Acta. 45:1309–1320. doi: 10.1016/0016-7037(81)90224-6
- Jacob JB, Scott JM, Turnbull RE, Tarling MS, Sagar MW. 2017. High-to ultrahigh-temperature metamorphism in the lower crust: An example resulting from Hikurangi Plateau collision and slab rollback in New Zealand. Journal of Metamorphic Geology. 35:831–853. doi: 10.1111/jmg.12257
- Katz RF, Spiegelman M, Langmuir CH. 2003. A new parameterization of hydrous mantle melting. Geochemistry, Geophysics, Geosystems. 4:1–19. doi: 10.1029/2001GC000209
- Kaulfuss U. 2017. Crater stratigraphy and the post-eruptive evolution of Foulden maar, southern New Zealand. New Zealand Journal of Geology and Geophysics. 60:410–432. doi: 10.1080/00288306.2017.1365733
- Kaulfuss U, Lee DE, Wartho J, Bowie E, Lindqvist JK, Conran JG, Bannister JM, Mildenhall DC, Kennedy EM, Gorman AR. 2018. Geology and palaeontology of the Hindon maar Complex: A Miocene terrestrial fossil Lagerstätte in southern New Zealand. Palaeogeography, Palaeoclimatology, Palaeoecology. 500:52–68. doi: 10.1016/j.palaeo.2018.03.022
- Kipf A, Hauff F, Werner R, Gohl K, van den Bogaard P, Hoernle K, Maicher D, Klügel A. 2014. Seamounts off the West Antarctic margin: A case for non-hotspot driven intraplate volcanism. Gondwana Research. 25:1660–1679. doi: 10.1016/j.gr.2013.06.013
- Klemme S, O'Neill HS. 2000. The near-solidus transition from garnet lherzolite to spinel lherzolite. Contributions to Mineralogy and Petrology. 138:237–248. doi: 10.1007/s004100050560
- Landis CA, Campbell HJ, Begg JG, Mildenhall DC, Paterson AM, Trewick SA. 2008. The Waipounamu erosion surface: questioning the antiquity of the New Zealand land surface and terrestrial fauna and flora. Geological Magazine. 145:173–197. doi: 10.1017/S0016756807004268
- Laporte D, Lambart S, Schiano P, Ottolini L. 2014. Experimental derivation of nepheline syenite and phonolite liquids by partial melting of upper mantle peridotites. Earth and Planetary Science Letters. 404:319–331. doi: 10.1016/j.epsl.2014.08.002
- Le Maitre RW. 2002. Igneous rocks: a classification and glossary of terms: recommendations of the IUGS, Subcommission on the Systematics of Igneous rocks. University Press.
- Lindqvist JK, Lee DE. 2009. High-frequency paleoclimate signals from Foulden Maar, Waipiata Volcanic Field, southern New Zealand: an early Miocene varved lacustrine diatomite deposit. Sedimentary Geology. 222:98–110. doi: 10.1016/j.sedgeo.2009.07.009
- Luth RW. 2014. Volatiles in Earth’s mantle. Treatise in geochemistry, 2nd ed. doi:10.1016/B978-0-08-095975-7.00207-2.
- Marshall P. 1906. The Geology of Dunedin, (New Zealand). Quarterly Journal of the Geological Society. 62:381–424. doi: 10.1144/GSL.JGS.1906.062.01-04.17
- Marshall P. 1914. The sequence of lavas at the North Head, Otago Harbour, Dunedin (New Zealand). Quarterly Journal of the Geological Society. 70:382–408. doi: 10.1144/GSL.JGS.1914.070.01-04.23
- Martin U. 2000. Eruptions and deposition of volcaniclastic rocks in the Dunedin volcanic Complex, Otago Peninsula, New Zealand [PhD thesis]. University of Otago. 434p.
- Martin U, White JDL. 2001. Depositional and eruptive mechanisms of density current deposits from a submarine vent at the Otago Peninsula, New Zealand. Particulate Gravity Currents. 114:245–259. doi: 10.1002/9781444304275.ch18
- McCoy-West AJ, Bennett VC, Amelin Y. 2016. Rapid Cenozoic ingrowth of isotopic signatures simulating “HIMU” in ancient lithospheric mantle: distinguishing source from process. Geochimica et Cosmochimica Acta. 187:79–101. doi: 10.1016/j.gca.2016.05.013
- McCoy-West AJ, Bennett VC, O’Neill HSC, Hermann J, Puchtel IS. 2015. The interplay between melting, refertilization and carbonatite metasomatism in off-cratonic lithospheric mantle under Zealandia: an integrated major, trace and platinum group element study. Journal of Petrology. 56:563–604. doi: 10.1093/petrology/egv011
- McCoy-West AJ, Bennett VC, Puchtel IS, Walker RJ. 2013. Extreme persistence of cratonic lithosphere in the southwest Pacific: Paleoproterozoic Os isotopic signatures in Zealandia. Geology. 41:231–234. doi: 10.1130/G33626.1
- McDougall I, Coombs DS. 1973. Potassium-argon ages for the Dunedin volcano and outlying volcanics. New Zealand Journal of Geology and Geophysics. 16:179–188. doi: 10.1080/00288306.1973.10431451
- McIntosh PE. 1989. Geochemistry of the Murdering Beach flow East Otago, New Zealand [MSc thesis]. University of Otago. 201p.
- McLeod OE, White JDL. 2018. Petrogenetic links between the Dunedin Volcano and peripheral volcanics of the Karitane suite. New Zealand Journal of Geology and Geophysics. 61:543–561. doi: 10.1080/00288306.2018.1518248
- Mortimer N. 1993. Jurassic tectonic history of the Otago schist, New Zealand. Tectonics. 12:237–244. doi: 10.1029/92TC01563
- Mortimer N, Gans PB, Hauff F, Barker DHN. 2012. Paleocene MORB and OIB from the Resolution Ridge, Tasman Sea. Australian Journal of Earth Sciences. 59:953–964. doi: 10.1080/08120099.2012.676569
- Mortimer N, Scott JM. 2020. Zealandia’s Volcanos. New Zealand Journal of Geology and Geophysics. in press.
- Needham AJ, Lindsay JM, Smith IEM, Augustinus P, Shane PA. 2011. Sequential eruption of alkaline and sub-alkaline magmas from a small monogenetic volcano in the Auckland volcanic field, New Zealand. Journal of Volcanology and Geothermal Research. 201:126–142. doi: 10.1016/j.jvolgeores.2010.07.017
- Németh K, White JD. 2003. Reconstructing eruption processes of a Miocene monogenetic volcanic field from vent remnants: Waipiata Volcanic Field, South Island, New Zealand. Journal of Volcanology and Geothermal Research. 124:1–21. doi: 10.1016/S0377-0273(03)00042-8
- Panter KS, Blusztajn J, Hart SR, Kyle PR, Esser R, McIntosh WC. 2006. The origin of HIMU in the SW Pacific: evidence from intraplate volcanism in southern New Zealand and subantarctic islands. Journal of Petrology. 47:1673–1704. doi: 10.1093/petrology/egl024
- Panter KS, Castillo P, Krans S, Deering C, McIntosh W, Valley JW, Kitajima K, Kyle P, Hart S, Blusztajn J. 2018. Melt origin across a rifted continental margin: a case for subduction-related metasomatic agents in the lithospheric source of alkaline basalt, NW Ross Sea, Antarctica. Journal of Petrology. 59:517–558. doi: 10.1093/petrology/egy036
- Park SH, Langmuir CH, Sims KW, Blichert-Toft J, Kim SS, Scott SR, Lin J, Choi H, Yang YS, Michael PJ. 2019. An isotopically distinct Zealandia–antarctic mantle domain in the Southern Ocean. Nature Geoscience. 12:206–214. doi: 10.1038/s41561-018-0292-4
- Pilet S. 2015. Generation of low-silica alkaline lavas: Petrological constraints, models, and thermal implications. The Interdisciplinary Earth: A Volume in Honor of Don L Anderson: Geological Society of America Special Paper. 514:281–304. doi: 10.1130/2015.2514(17)
- Pilet S, Baker MB, Stolper EM. 2008. Metasomatized lithosphere and the origin of alkaline lavas. Science. 320:916–919. doi: 10.1126/science.1156563
- Price RC, Chappell BW. 1975. Fractional crystallisation and the petrology of Dunedin Volcano. Contributions to Mineralogy and Petrology. 53:157–182. doi: 10.1007/BF00372602
- Price RC, Compston W. 1973. The geochemistry of the Dunedin Volcano: strontium isotope chemistry. Contributions to Mineralogy and Petrology. 42:55–61. doi: 10.1007/BF00521647
- Price RC, Coombs DS. 1975. Phonolitic lava domes and other features of the Dunedin Volcano, East Otago. Journal of the Royal Society of New Zealand. 5:133–152. doi: 10.1080/03036758.1975.10419368
- Price RC, Cooper AF, Woodhead JD, Cartwright I. 2003. Phonolitic diatremes within the Dunedin Volcano, South Island, New Zealand. Journal of Petrology. 44:2053–2080. doi: 10.1093/petrology/egg070
- Price RC, Green DH. 1972. Lherzolite nodules in a “mafic phonolite” from north-east Otago, New Zealand. Nature. 235:133.
- Price RC, Nicholls IA, Day A. 2014. Lithospheric influences on magma compositions of late Mesozoic and Cenozoic intraplate basalts (the older Volcanics) of Victoria, south-eastern Australia. Lithos. 206-207:179–200. doi: 10.1016/j.lithos.2014.07.027
- Price RC, Taylor SR. 1973. The geochemistry of the Dunedin volcano, east Otago, New Zealand: rare earth elements. Contributions to Mineralogy and Petrology. 40:195–205. doi: 10.1007/BF00373784
- Price RC, Wallace RC. 1976. The significance of corona textured inclusions from a high pressure fractionated alkalic lava: North Otago, New Zealand. Lithos. 9:319–329. doi: 10.1016/0024-4937(76)90022-0
- Rae AJ. 1990. Geochemistry of the Siberia Hill volcanics and the ultramafic inclusions, Siberia Hill, East Otago, New Zealand [MSc thesis]. University of Otago. 210p.
- Reay A, McIntosh PE, Gibson IL. 1991. Lherzolite xenolith bearing flows from the east Otago province: crystal fractionation of upper mantle magmas. New Zealand Journal of Geology and Geophysics. 34:317–327. doi: 10.1080/00288306.1991.9514469
- Reilly WI. 1972. Gravitational expression of the Dunedin Volcano. New Zealand Journal of Geology and Geophysics. 15:16–21. doi: 10.1080/00288306.1972.10423943
- Scanlan EJ, Scott JM, le Roux PJ. 2020. Pyrometamorphosed Otago Schist xenoliths reveal domainal equilibration and limited chemical contamination of host Dunedin Volcanic Group basanite. NZJGG.
- Scanlan EJ, Scott JM, Wilson VJ, Stirling CH, Reid MR, le Roux PJ. 2018. In situ 87Sr/86Sr of scheelite and calcite reveals proximal and distal fluid-rock interaction during orogenic W-Au mineralization, Otago Schist, New Zealand. Economic Geology. 113:1571–1586. doi: 10.5382/econgeo.2018.4603
- Scott JM, Brenna M, Crase JA, Waight TE, van der Meer QHA, Cooper AF, Palin JM, le Roux PJ, Münker C. 2016a. Peridotitic lithosphere metasomatized by volatile-bearing melts, and its association with intraplate alkaline HIMU-like magmatism. Journal of Petrology. 57:2053–2078. doi: 10.1093/petrology/egw069
- Scott JM, Hodgkinson A, Palin JM, Waight TE, van der Meer QHA, Cooper AF. 2014a. Ancient melt depletion overprinted by young carbonatitic metasomatism in the New Zealand lithospheric mantle. Contributions to Mineralogy and Petrology. 167:963. doi: 10.1007/s00410-014-0963-0
- Scott JM, Liu J, Pearson DG, Harris GA, Czertowicz TA, Woodland SJ, Riches AJV, Luth RW. 2019. Continent stabilisation by lateral accretion of subduction zone-processed depleted mantle residues; insights from Zealandia. Earth and Planetary Science Letters. 507:175–186. doi: 10.1016/j.epsl.2018.11.039
- Scott JM, Liu J, Pearson DG, Waight TE. 2016b. Mantle depletion and metasomatism recorded in orthopyroxene in highly depleted peridotites. Chemical Geology. 441:280–291. doi: 10.1016/j.chemgeo.2016.08.024
- Scott JM, Turnbull IM. 2019. Geology of New Zealand’s Sub-Antarctic Islands. New Zealand Journal of Geology and Geophysics. In press.
- Scott JM, Turnbull IM, Auer A, Palin JM. 2013. The sub-Antarctic Antipodes Volcano: a < 0.5 Ma HIMU-like Surtseyan volcanic outpost on the edge of the Campbell Plateau, New Zealand. New Zealand Journal of Geology and Geophysics. 56:134–153. doi: 10.1080/00288306.2013.802246
- Scott JM, Waight TE, van der Meer QHA, Palin JM, Cooper AF, Münker C. 2014b. Metasomatized ancient lithospheric mantle beneath the young Zealandia microcontinent and its role in HIMU-like intraplate magmatism. Geochemistry, Geophysics, Geosystems. 15:3477–3501. doi: 10.1002/2014GC005300
- Seton M, Müller RD, Zahirovic S, Gaina C, Torsvik T, Shephard G, Talsma A, Gurnis M, Turner M, Maus S, et al. 2012. Global continental and ocean basin reconstructions since 200 Ma. Earth-Science Reviews. 113(3–4):212–270. doi: 10.1016/j.earscirev.2012.03.002
- Shaw JE, Baker JA, Kent A JR, Ibrahim KM, Menzies MA. 2007. The geochemistry of the Arabian lithospheric mantle—a source for intraplate volcanism? Journal of Petrology. 48:1495–1512. doi: 10.1093/petrology/egm027
- Sprung P, Schuth S, Münker C, Hoke L. 2007. Intraplate volcanism in New Zealand: the role of fossil plume material and variable lithospheric properties. Contributions to Mineralogy and Petrology. 153:669–687. doi: 10.1007/s00410-006-0169-1
- Stracke A, Hofmann AW, Hart SR. 2005. FOZO, HIMU, and the rest of the mantle zoo. Geochemistry, Geophysics, Geosystems. 6:1–20. doi: 10.1029/2004GC000824
- Sun S-S, McDonough WF. 1989. Chemical and isotopic systematics of oceanic basalts: implications for mantle composition and processes. Geological Society, London, Special Publications. 42:313–345. doi: 10.1144/GSL.SP.1989.042.01.19
- Sutherland FL, Meffre S. 2009. Zircon megacryst ages and chemistry, from a placer, Dunedin volcanic area, eastern Otago, New Zealand. New Zealand Journal of Geology and Geophysics. 52:185–194. doi: 10.1080/00288300909509885
- Tenny SM. 1977. The Geology of the Flat Hill area, Otago, New Zealand [MSc thesis]. University of Otago.
- Timm C, Hoernle K, Van Den Bogaard P, Bindeman I, Weaver S. 2009. Geochemical evolution of intraplate volcanism at Banks Peninsula, New Zealand: interaction between asthenospheric and lithospheric melts. Journal of Petrology. 50:989–1023. doi: 10.1093/petrology/egp029
- Timm C, Hoernle K, Werner R, Hauff F, van den Bogaard P, White J, Mortimer N, Garbe-Schönberg D. 2010. Temporal and geochemical evolution of the Cenozoic intraplate volcanism of Zealandia. Earth-Science Reviews. 98:38–64. doi: 10.1016/j.earscirev.2009.10.002
- Ulrich GHF. 1891. On the occurrence of nepheline-bearing rocks at Dunedin, New Zealand. Australasian Association for the Advancement of Science. 3:127–150.
- van der Meer QHA, Scott JM, Serre SH, Whitehouse MJ, Kristoffersen M, le Roux PJ, Pope EC. 2019. Low-δ18O zircon xenocrysts in alkaline basalts; a window into the complex carbonatite-metasomatic history of the Zealandia lithospheric mantle. Geochimica et Cosmochimica Acta. 254:21–39. doi: 10.1016/j.gca.2019.03.029
- van der Meer QHA, Storey M, Scott JM, Waight TE. 2016. Abrupt spatial and geochemical changes in lamprophyre magmatism related to Gondwana fragmentation prior, during and after opening of the Tasman Sea. Gondwana Research. 36:142–156. doi: 10.1016/j.gr.2016.04.004
- van der Meer QHA, Waight TE, Scott JM, Münker C. 2017. Variable sources for Cretaceous to recent HIMU and HIMU-like intraplate magmatism in New Zealand. Earth and Planetary Science Letters. 469:27–41. doi: 10.1016/j.epsl.2017.03.037
- Waight TE, Weaver SD, Maas R, Eby GN. 1998. French Creek granite and Hohonu dyke swarm, South Island, New Zealand: Late Cretaceous alkaline magmatism and the opening of the Tasman Sea. Australian Journal of Earth Sciences. 45:823–835. doi: 10.1080/08120099808728438
- Walls DJ. 1991. West Waihola basanites and associated segregated liquids [MSc thesis]. University of Otago. 239 p.
- Wang SJ, Teng F-Z, Scott JM. 2016. Tracing the origin of continental HIMU-like intraplate volcanism using magnesium isotope systematics. Geochimica et Cosmochimica Acta. 185:78–87. doi: 10.1016/j.gca.2016.01.007
- Wright JB. 1966. Olivine nodules in a phonolite of the East Otago alkaline province, New Zealand. Nature. 5035:519. doi: 10.1038/210519a0
- Yaxley GM, Green DH, Kamenetsky V. 1998. Carbonatite metasomatism in the southeastern Australian lithosphere. Journal of Petrology. 39:1917–1930. doi: 10.1093/petroj/39.11-12.1917
- Youngson JH, Craw D, Landis CA, Schmitt KR. 1998. Redefinition and interpretation of late Miocene-Pleistocene terrestrial stratigraphy, central Otago, New Zealand. New Zealand Journal of Geology and Geophysics. 41:51–68. doi: 10.1080/00288306.1998.9514790
- Zhang M, Stephenson PJ, O’Reilly SY, McCulloch MT, Norman M. 2001. Petrogenesis and geodynamic implications of late Cenozoic basalts in north Queensland, Australia: trace-element and Sr–Nd–Pb isotope evidence. Journal of Petrology. 42:685–719. doi: 10.1093/petrology/42.4.685