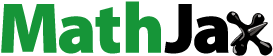
ABSTRACT
Volcaniclastic and coherent volcanic rocks of the Permian island arc Brook Street Terrane crop out along the Southland coast, New Zealand, near Riverton. Extensive along-strike exposures reveal lateral variations in these submarine deposits and provide a rare opportunity to examine deposits of an arc environment within a thin slice of time. We describe pillow lavas, dikes, hydroclastic breccias, tuffs, lapilli tuffs and argillites, all hydrothermally altered and metamorphosed to prehnite-pumpellyite facies. Trace element geochemical data indicate multiple primitive island arc to MORB-like magma sources. Two separate volcanic centres apparently tapped different mantle domains in a source region transitional between the arc and back-arc. The centres’ proximity and inferred small sizes suggest they were satellite vents of a larger arc volcano. Tuff and lapilli tuff were formed as hyaloclastite during eruption of pillow lava, and redeposited by subaqueous mass flows. Peperitic contacts indicate that the lavas intruded unconsolidated volcaniclastic deposits. We infer that hydroclastic fragmentation associated with pillow emplacement produced debris that was then intruded by later pillows, with local peperite formation, as eruption persisted. The hydroclastic debris from these satellite vents was resedimented in turbidite fans with channels, and periodic turbidity currents delivered fine-grained material from the volcano’s main vent.
Introduction
Submarine volcanoes are abundant in the modern ocean, their coherent and volcaniclastic deposits are abundant globally, and they host many significant economic deposits (e.g. White et al. Citation2003 and references therein). Despite their significance, much remains to be learned about their emplacement, particularly in the context of different volcanic settings on the seafloor. Modern submarine sites are difficult to access (White et al. Citation2003; Manville et al. Citation2009; Cas and Giordano Citation2014), so those that are accessible are especially pertinent. The Riverton section on the south coast of New Zealand () holds information about processes of eruption and sedimentation in a now-accreted oceanic island arc. Its extensive along-strike exposures reveal that laterally equivalent environments existed during a thin slice of time during development of the Brook Street Terrane. They offer a view of the Permian arc seafloor that is highly complementary to that extracted by Houghton and Landis (Citation1989) from ridge-crest exposures of subvertically dipping strata in the Takitimu Mountains, which allows inference of evolution through time but not resolution of lateral relationships.
Figure 1. Location map with Riverton section field area boxed. Also shown are intrusive rocks of the Median Batholith, and the largely volcanic Brook Street Terrane (the western contact of the terrane is not exposed on this coast). Basement geology based on Turnbull and Allibone (Citation2003).
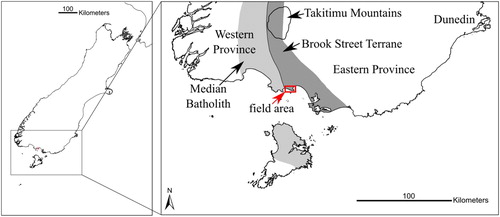
This paper characterises lateral, and to a lesser degree vertical, changes through the well-exposed Riverton section of submarine-emplaced island arc rocks. We address the dominant eruption processes, how lavas and primary volcaniclastic debris were emplaced, and the role of longer-distance transport of volcaniclastic sediment in producing this succession. Whether the succession represents a single eruption, or even a single volcano, is also evaluated.
The studied section, from Riverton to Colac Bay extends for 8 km, approximately along strike, in a section 220 m thick that reveals lateral variations in deposits of an oceanic island arc (). Mapping of lithofacies and geochemical analysis of crystals in lavas and volcaniclastic rocks underpins an interpretation of the relationships between them and the processes by which they formed.
Figure 2. Lithofacies map and generalised vertical successions (GVS). Outcrop along the coast is almost continuous; inland it is sparse. Numbered circles mark field localities. Bold line on inset map shows the location of the cross-section (A) relative to mapped geology.
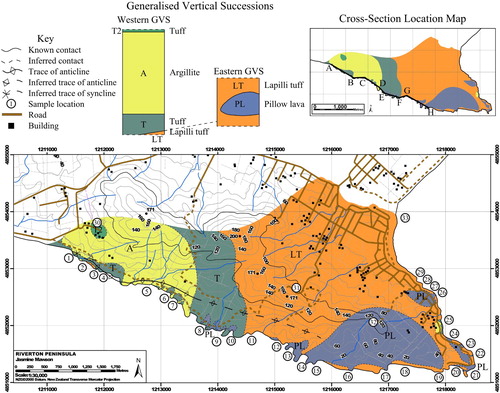
The Brook Street Terrane
The Brook Street Terrane is part of the Eastern Province of New Zealand, and comprises rocks formed in a Permian ocean island volcanic arc that was accreted to the Gondwana margin at 230–245 Ma (Mortimer et al. Citation1999). Oxygen isotopic and palaeomagnetic data indicate that it formed at a low palaeolatitude and was allochthonous to the Gondwana margin (Haston et al. Citation1989; Blattner and Williams Citation1990). Price et al. (Citation2006) and Spandler et al. (Citation2003, Citation2005) classify the Brook Street Terrane as a primitive oceanic arc, with tholeiitic magmas and no continental input (Frost and Coombs Citation1989; Houghton and Landis Citation1989; Mortimer et al. Citation1999; Spandler et al. Citation2005). Spandler et al. (Citation2005) also posit that the Brook Street volcanics were part of an extensive island arc in Permian times, which also included the Australian Gympie Terrane and the Tembera Terrane of New Caledonia. Conversely, according to Haston et al. (Citation1989) and Price et al. (Citation2006) the terrane comprises parts of several fragmented Permian arcs of subtly different ages and compositions, all accreted to the Gondwana margin. The polarity of the subduction zone is not established, although Bradshaw (Citation1994) suggested that terrane structure implies subduction eastwards away from the Gondwana margin. Published ages from Ar-Ar and U-Pb dating range from 292–249 Ma (Mortimer et al. Citation1999).
The Brook Street Terrane includes intrusive and extrusive rocks, mostly basaltic to andesitic in composition, volcaniclastic material, and hemipelagic sedimentary units of the associated back-arc basin (Haston et al. Citation1989; Houghton and Landis Citation1989; Spandler et al. Citation2005). The environments of volcanism ranged from deep submarine to marginally emergent, and volcanism included effusive and explosive eruptions (Houghton and Landis Citation1989). Further from the arc, turbidite sedimentation predominated and many of the volcaniclastic sediments show evidence of having been deposited or redeposited in this way; steep and unstable near-vent slopes are prone to collapse and triggering of mass flows (Houghton and Landis Citation1989).
The Brook Street rocks are hydrothermally altered, and regionally metamorphosed to prehnite-pumpellyite facies, which indicates metamorphism at up to ∼280°C and ∼2–10 km depth (Spandler et al. Citation2003). Any glass in the rocks has been altered, and original matrix cemented and weakly metamorphosed, but major volcanic textures are preserved. The deposit mineralogy has been altered significantly, but the movement of elements is generally on a local scale and has caused little change in the geochemistry of whole-rock samples (Sivell and Rankin Citation1983). Strata are also folded into a series of open anticlines and synclines (Mortimer et al. Citation1999). There has been significant regional deformation; the studied section dips only shallowly, but cannot be assumed to represent original dip.
Methods
Major-element geochemistry
Major element geochemical analysis was undertaken to support the mineralogy identified in thin section, and determine more precisely the extent of alteration. Energy-Dispersive x-ray Spectroscopy (EDS) analyses used a Zeiss Sigma VP variable-pressure field-emission scanning electron microscope at the Otago Centre for Electron Microscopy. Analyses were performed in EDS mode with an AsB (Angle-selective Backscatter) detector. We operated with a beam current of 15 kV, aperture size of 60 μm and 1024 × 768, using a working distance of 8.5 mm. In addition to point measurements, linescans and maps were obtained. Analysis used Oxford Instruments’ pre-installed factory (virtual) standards with normalisation of beam current through a Co metal measurement.
Trace-element geochemistry
The trace element compositions of clinopyroxenes were analysed at the Centre for Trace Element Analysis at the University of Otago by laser ablation inductively coupled plasma mass spectrometry (LA-ICP-MS), using a ResoneticsRESOlution M-50-LR excimer (193 nm) laser ablation system coupled to an Agilent 7500 cs/ce Quadrupole ICP mass spectrometer. These analyses were carried out to compare these rocks with others from the Brook Street terrane, and to evaluate their magma sources in the context of the island arc.
Eight polished thin sections were analysed, including two pillow lavas (samples 20 and 21a), two dikes (samples 21b and 29a), two basalt clasts from within lapilli tuff (samples 28 and 29b), one sample from the matrix of lapilli tuff (sample 17), and one sample of tuff (sample 5). For all samples except the pillow lavas, five of the largest, freshest augite phenocrysts were selected and laser ablation tracks with 400 μm transects and 50 μm spot sizes were made along the rim and through the centre of each. The pillow lavas analysed lack phenocrysts so ten groundmass augites were analysed for each of them using a smaller spot size of 33 μm and transect of 200 μm. Ablation lasts for 40 s at 10 Hz with a fluence of 2.50 J/cm2. All runs used a mixture of Ar and He as a carrier gas. Standard synthetic reference glass 610 was measured at the beginning and end of each thin section to correct for instrument drift. A rapid pre-analysis ablation was performed before each transect to clean the analysed surface. Data were normalised to the Si concentration of augite grains.
Whole-rock trace element concentrations were estimated using clinopyroxene-liquid partition coefficients from Bédard (Citation2014) in order to compare this data with whole-rock data from the Brook Street Terrane. Calculated whole-rock data from clinopyroxene compositions introduce some uncertainties, but given the petrographic evidence of pervasive mineralogic alteration it may yield results closer to the original composition of the liquid than would a bulk rock measurement. In order to perform these calculations, the distribution coefficients were taken from the supplemental data of Bédard (Citation2014), and an average of these values were used. The model whole-rock data were calculated for the averages of trace element concentrations from the cores of augite grains (see supplemental material), using the equation where
is the concentration of a given element within clinopyroxene,
is the concentration in the liquid, and
is the distribution coefficient between clinopyroxene and liquid.
Lithofacies
Field description, mapping and thin section analysis were carried out to ascertain the nature of the lithofacies, the relationships between them, and to understand the processes by which they formed. Lithofacies were distinguished in the field on the basis of effusive forms, bedforms, crystallinity and grainsize. Detailed mapping was possible along the coast from Riverton into Colac Bay, with a few exposures inland used to constrain relationships with rocks not exposed ().
The rocks at Riverton comprise five lithofacies (nomenclature from White and Houghton Citation2006). Major characteristics of the lithofacies are summarised in . A geological map (), a cross-section along the coast (A), and a logged stratigraphic section (B) display the relations among lithofacies. A large pillow lava pile (about 1.7 km across, at least 120 m high) dominates the southeastern part of the Riverton Peninsula. This and smaller pillow lava piles intrude into lapilli tuff and tuff. Lapilli tuff underlies and fills channels cut in tuff (, B). Tuff underlies and is interbedded with argillite. The whole section is cut by dikes. Westwards from the large pillow lava pile is a shallowly dipping sequence of lapilli tuff, tuff and argillite, while to the east lapilli tuff dominates and the sequence is less clear.
Figure 3. A, Cross-section along the coast, showing lateral relationships among lithofacies. This section is vertically exaggerated (×2) so relationships can be better seen. The strips of lapilli tuff in section E–F are a schematic representation of lapilli tuff filling channels in tuff (see text). Argillite and tuff are in part interbedded (not illustrated). B, Logged stratigraphic section of the western side of the sequence (that represented in the Western GVS of ).
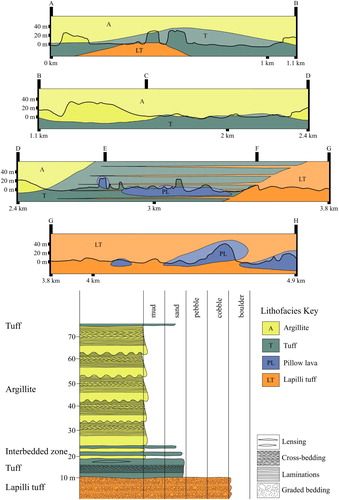
Figure 4. Features of these rocks include: A, Reverse-graded beds in lapilli tuff, with black arrows showing the direction of grading. B, Lapilli tuff filling channels within tuff; C, Peperitic contact between pillow lava below and tuff above. D, Argillite showing Bouma sequences (dotted lines denote boundaries between successive sequences; notebook for scale has 20 cm spine). E, Clasts in lapilli tuff at site 29, showing the distinction between aphyric clasts and those with abundant amygdales, with quartz cement in the matrix. F, Examples of clasts within the tuff, including those bearing chlorite-filled amygdales, and those with albite laths. G, Amygdale in pillow lava showing pumpellyite rim and mixed centre: regions with different crystal sizes are also visible. H, Dike, showing fresh clinopyroxene and altered plagioclase phenocrysts and chlorite pseudomorphing crystal shapes. Thin section images in plane polarised light.
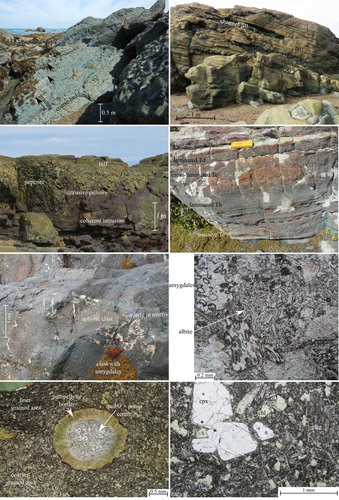
Table 1. Summary of lithofacies features.
All rocks in the Riverton section have undergone hydrothermal alteration and regional metamorphism, to varying degrees. All plagioclase is albitised, and some also bears pumpellyite and clay alteration. The abundance of chlorite, some of which pseudomorphs crystal shapes (H), and absence of olivine suggests that all olivine has been chloritised. Calcite and/or quartz form secondary cement in argillite, lapilli tuff, and the recrystallised inter-pillow matrix (, E). Pillow rims are either not preserved or have lost their glassy texture, and only devitrified glass is discernible in thin section. Some clasts in tuff and lapilli tuff are deformed to form pseudomatrix, and original matrix is not discernible. Throughout the section vesicles are infilled by prehnite, pumpellyite, chlorite, quartz and albite, forming amygdales (G).
Pillow lava displays the prominent pillow lava morphology of sack-like forms filling into depressions between underlying pillows (); in a few localities the outcrop is sufficiently three-dimensional to reveal the elongate lateral sides of the pillows. Glassy pillow rinds are commonly absent, but in a few places they are represented by a 1–2 cm band of darker coloured, sometimes reddish, rock with cracks between it and the body of the pillow.
In lapilli tuff, most of the basalt clasts (2–50 cm) are porphyritic, with phenocrysts of plagioclase and clinopyroxene. Larger blocks (>64 cm) comprise less than ∼20% of the rock (by visual estimate). The majority of basaltic clasts have vesicles infilled with quartz, chlorite, or prehnite and are interpreted as pillow lava clasts (E). Their amygdales are smaller on average than those of intact pillow lavas. A few clasts lack these amygdales; some of these are aphyric (E), and many display flow banding; these are interpreted as dike fragments due to their close similarity to the dikes observed. Lapilli tuff beds are 0.5–2 m thick and weakly defined by grainsize variations (, A). Many beds are reverse graded, with framework-supported upper parts. At some sites, most beds are reverse graded and coarsen upwards, with sequences of 2–4 fine to medium reverse-graded lapilli tuff beds followed by a bed with very large clasts (A). Ungraded beds with similar coarsening upward bedding sequences, and alternations of normal- and reverse-graded beds, are also locally present. Between sites 8 and 10, weakly bedded medium-grained tuff hosts 0.2–1 m deep and 1–6 m wide channel-filling bodies of coarse lapilli tuff with apparently chaotic internal structure (B). The channel deposits thin westwards, towards the area where tuff predominates.
Most vesicles within basalt ash grains are small and filled solely with chlorite (F), and the groundmass of some grains is inferred to have originally been glass (homogeneous in plane light, lacks crystalline textures) (F). Vesicles at the edges of these small clasts are filled with the small crystals matching those in the surrounding finer-grained matrix. Within lapilli, plagioclase commonly and clinopyroxene rarely, occur in glomerocrysts.
Coherent basalt is intrusive, with peperitic contacts against both tuff and lapilli tuff (, C). Here, pillows have formed through contact with water-saturated sediment (Garrison Citation1972; McPhie et al. Citation1993; Portner et al. Citation2010; White et al. Citation2015). Peperitic contacts are gradational over several metres, with pillows becoming increasingly dispersed within inter-pillow matrix of remobilised volcaniclasts, until they occur as isolated dense basalt clasts with subspherical shapes. In most places peperite grades into the host lapilli tuff, as their clast textures, sizes, and compositions are very similar. In the tuff, peperite extends 1–2 m away from the pillow lava, beyond which the weak bedding of the tuff is preserved (C). Intrusive pillows are distinguished from peperite on the basis of size and continuity, with pillows generally being at least ∼10 cm (Walker Citation1992) and connected with coherent basalt or a more continuous pillow lava pile (Skilling et al. Citation2002), and peperite clasts being smaller, detached, or more angular. The peperite is mostly globular in texture, with a few more angular clasts (e.g. Busby-Spera and White Citation1987; Doyle Citation2000).
Argillite has prominent 0.4–1 m beds, which are consistently laminated, and locally cross-laminated. Trough cross-laminae indicate northward flow. The argillite is entirely fine-grained (very fine sand at its coarsest), and displays Bouma Tb-e to Td-e sequences, implying deposition by turbidity currents. Where argillite and tuff are interbedded, they form successions which grow finer and thicker upward, and lack Bouma sequences. Above these, argillite dominates.
Most dikes are porphyritic with phenocrysts of pyroxene and plagioclase (H), though some are aphyric. Most have chilled margins, and several display flow banding. Some have margins which are undulating on a decimetre scale. Some have multiple generations of chilled margins, and some have bands of varying vesicularity. Where dikes appear in lapilli tuff, several of them display apophyses into the surrounding lapilli tuff, and in places have engulfed domains or clasts of the lapilli tuff. One dike at site 19 terminates in the lapilli tuff with a bulbous, blobby form, with clasts that resemble the dike surrounding it in the lapilli tuff.
Major element geochemistry
Major element geochemistry confirms the mineralogy identified in thin section (). Representative samples of the data used to construct the following figures are available in the supplemental material. The majority of clinopyroxenes are compositionally classified as augite or diopside, although one analysed is hedenbergite (). Most feldspars are albite (), suggesting metamorphic albitizaton of originally more anorthite-rich feldspars (Spandler et al. Citation2003). Less common K-feldspar is secondary and occurs as small crystals in the matrix of the clastic rocks. Pillow lavas lack K-feldspar, whereas in dikes both of the feldspars occur in phenocrysts zoned from plagioclase to K-feldspar (probably secondary).
Trace element geochemistry
These data display a wide range of absolute concentrations of trace elements. We consider analyses of the crystal cores to be more reliable than rim analyses, several of which may be contaminated by the groundmass. displays the averages of each set of core analyses, with a few outliers removed (complete data tables available in supplemental material). Rims generally display slightly higher concentrations than cores, and most exhibit the same patterns. Clinopyroxenes in coherent basalts have significantly higher concentrations of trace elements than do those in volcaniclastic rocks, and the unusual sample 28 (a large block from within the lapilli tuff) has by far the lowest concentrations. In addition to having lower concentrations, sample 28 shows the widest spread of concentrations among the clinopyroxenes analysed.
Figure 7. A, Chondrite-normalised rare earth element (REE) and B, Primitive mantle-normalised trace element profiles for the averages of the cores of augite crystals for each sample. Normalising values from Sun and McDonough (Citation1989).
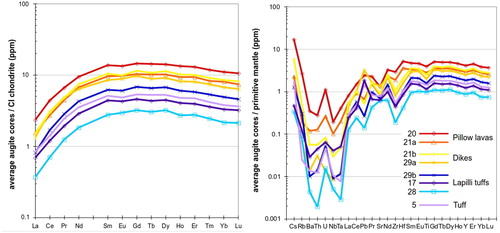
The trace element compositions of the clinopyroxene crystals display patterns typical of island arc tholeiites (). A positive Pb anomaly (as seen in samples 5, 17, 20, and 29a) is characteristic of island arc rocks (Faure Citation2001; Spandler et al. Citation2005; Rossel et al. Citation2013; Schmidt and Jagoutz Citation2017). Other samples (21a, 21b, 28, and 29b) display a negative Pb anomaly, which is more commonly associated with mid-ocean ridge or back-arc basalts. With the exception of sample 28 (which is an unusual sample in every context in which it is examined), the samples with a negative Pb anomaly all have higher concentrations of Th than Ba, higher Ta than Nb, and a pronounced negative Sr anomaly. The samples with positive Pb anomalies are more variable, without any other traits distinguishing them as a group.
Whole-rock compositions calculated from clinopyroxene data were compared with published whole-rock data from the Brook Street Terrane (Spandler et al. Citation2005) (). The two profiles from Spandler et al. (Citation2005) shown in are from sites within the field area, and generally match quite well with our results, confirming the island arc signature of the Brook Street Terrane as reported by other authors. Spandler et al. (Citation2005) acknowledge that alteration affected their data, particularly for Cs, Rb, Ba, Pb, Sr and U, so for these elements in particular our clinopyroxene data are probably more robust.
Figure 8. Calculated model whole-rock A, Chondrite-normalised rare earth element and B, Primitive mantle-normalised trace element profiles, compared to whole-rock data from Spandler et al. (Citation2005), showing similar patterns with a few notable differences (see text, Supplement). Normalising values from Sun and McDonough (Citation1989).
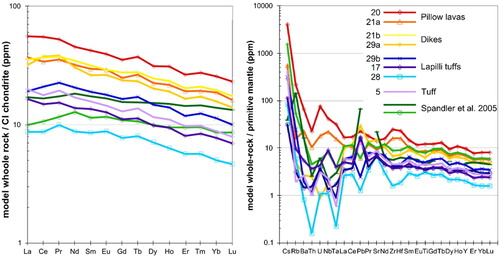
High field strength elements (HFSE), large ion lithophile elements (LILE) and light rare earth elements (LREE) may be used in plots of M/Yb vs. Nb/Yb (where M is the element in question) to evaluate mantle and slab contributions to magma (Pearce and Peate Citation1995; Maurice et al. Citation2012). HFSE behave conservatively during subduction, so their concentrations reflect the composition of the mantle wedge, whereas LILE and LREE are non-conservative and thus related to the contribution from the subducted slab. Maurice et al. (Citation2012) use whole-rock compositions, so calculated whole-rock data is used to compare to theirs. On the HFSE Y/Yb (A), Zr/Yb, and Sm/Yb vs Nb/Yb diagrams (other diagrams in supplemental material), our samples plot within the mantle array, indicating derivation from the mantle, with a wide spread that suggests a source variably enriched or depleted. Plots using the LREE La and the LILE Ba (B), Th, and U (supplemental material) in the same way yield results scattered within, above and below the mantle array; positioning above the mantle array would indicate derivation from the subducted slab. According to Pearce and Stern (Citation2006), compositions that plot between the mantle array and the volcanic arc array on the Ba/Yb vs. Nb/Yb plot are characteristic of back-arc basin basalts, which are transitional between island-arc tholeiites and MORB. The presence of data which plot within the mantle array on the Ba/Yb vs. Nb/Yb diagram indicates some involvement of a back-arc spreading ridge (Pearce and Stern Citation2006). With the exception of B, these plots show very little slab component; thus, while a significant Ba component is contributed from the slab, these other elements are not. This is consistent with the assertions of Houghton and Landis (Citation1989) and Spandler et al. (Citation2005) that the Brook Street Terrane is immature and contains little continental input. According to Maurice et al. (Citation2012), low ratios of Th/Yb such as these should indicate a lack of input from melted sediments. The general pattern of enrichment in these plots is broadly consistent with the variation in absolute concentrations of incompatible elements. Pillow lavas form a group, which is generally more enriched than the other samples. The other samples are not clustered in groups like this. Samples plot in varying positions relative to N-MORB and E-MORB; most cluster around N-MORB, but the spread is significant.
Figure 9. Plots of A, Y/Yb vs. Nb/Yb after Maurice et al. (Citation2012) and B, Ba/Yb vs. Nb/Yb after Pearce and Stern (Citation2006). Key in bottom right applies to both plots.
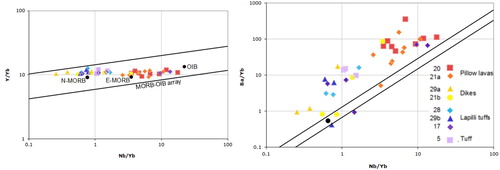
Discussion
The structure of a submarine stratovolcano is highly heterogeneous, with changes between lithofacies known to occur at small spatial scales (Houghton and Landis Citation1989; Kralj Citation2012; De Silva and Lindsay Citation2015; Morley Citation2018). The lateral changes in the Riverton section provide an indication of Permian volcanic processes that occurred contemporaneously, and reveal the geometric relations among their products. To overcome effects of alteration on major-element compositions, we use trace-element geochemical data to identify changes through time of the erupted magmas and to distinguish sources for different surface deposits.
Tectonic setting of magmatism
Trace element analyses of clinopyroxenes show these rocks to be island arc tholeiites, as expected for the Brook Street Terrane (e.g. Spandler et al. Citation2005). Trace element signatures in pillow lavas, dikes and volcaniclastic rocks reveal two distinct geochemical signatures, one with a positive Pb anomaly, and the other with a negative Pb anomaly. Each characterises a pillow lava body and associated volcaniclastic rocks, from which we infer that there were two small volcanic centres (). The dike with a signature different from its host may extend from the other volcanic centre, and has peperitic margins; this suggests that the two vents were probably active penecontemporaneously (McPhie et al. Citation1993; Skilling et al. Citation2002).
Figure 10. Map showing inferred volcanic depositional systems and positions of two small volcanic centres. Positions of depositional fan lobes and branching channel indicated by features in outcrop. Argillite is derived from the main volcano’s central vent(s); tuff and lapilli tuff from satellite vents form the marked lobes. Trace element sample numbers are marked on the map; positive Pb anomaly = oval symbols, negative Pb anomaly = rectangles.
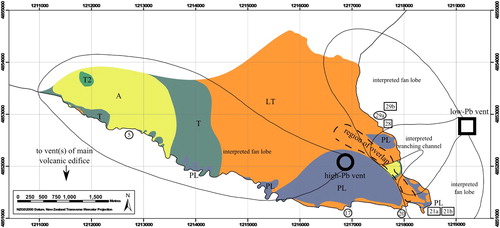
Clinopyroxene crystals from pyroclastic rocks all have lower overall concentrations of trace elements than do those from the pillow lavas and dikes, indicating differences probably linked to fractional crystallisation. The angular block found in the lapilli tuff at site 28 has trace-element patterns different from those of its host deposit. This suggests a distinct source, implying a change in source characteristics through time for the eruption centre that produced the block and its host material. It was probably plucked from the vent or conduit walls during emplacement of the lapilli tuff.
A positive Pb anomaly is characteristic of island arc rocks, whereas a negative one is associated with mid-ocean ridge basalt or back-arc volcanic rocks (Faure Citation2001; Spandler et al. Citation2005; Rossel et al. Citation2013; Schmidt and Jagoutz Citation2017). Many back-arc basin basalts are transitional in composition between arc and MORB, and carry the imprint of heterogeneous mantle sources (Pearce and Stern Citation2006). For example, in the Tonga-Kermadec arc and the Izu-Bonin arc, compositions vary across the arcs, related to the varying input of subduction-related and back-arc spreading-related magma (Ewart et al. Citation1998; Hochstaedter et al. Citation2000). Arc settings may display geochemical gradients between arc and back-arc, sometimes over small distances (Ewart et al. Citation1998). Orton (Citation1996) asserts that the arc and back-arc volcanoes can be as little as 5 km apart, and that the distinction between the two can be ill-defined. The Riverton rocks have island arc tholeiitic trace element patterns, yet occupy back-arc positions on element ratio plots; together these characteristics suggest a transitional character, probably behind the arc but close to it. Thus, it is plausible that the two vents of this Riverton section tapped different mantle domains within a transitional arc-back-arc zone.
Major island arc volcanoes are usually tens of kilometres in diameter at the base and tens to hundreds of kilometres apart (Cas Citation1992; Kano et al. Citation1993). Thus, the small centres of the Riverton section may be satellite vents of a larger volcano, or early products of a developing volcanic system. Satellite vents are commonly fed by small batches of magma which are separate from the main magma source and may vary widely in composition (Davidson and De Silva Citation2000).
Volcanic setting and eruption processes
Pillow lavas indicate that the studied volcanic section formed subaqueously, while abundant turbidites and the absence of any wave-formed sedimentary structures suggest that the deposits formed below wave-base (McPhie et al. Citation1993; Doucet et al. Citation1994). Abundant argillite suggests a relatively deep-water setting. Thus, this succession corresponds to the initial, deep-water stage of island arc volcanism identified by Houghton and Landis (Citation1989) in the nearby Takitimu Mountains. Some intrusions have pillowed margins extending into peperite (C, B; White et al. Citation2015), but the large pillow lava pile was probably not wholly intrusive as it comprises a continuous edifice of pillow lava thicker than the lapilli tuff around it. The surrounding volcaniclastics were emplaced first, so basalt is inferred to have initially intruded them at shallow levels, with subsequent basalt entering and building out a growing pillow mound (Chadwick et al. Citation2013) that eventually built up above the surrounding seafloor (e.g. Garrison Citation1972). Inter-pillow matrix grains match those of adjacent volcaniclastic rock, and no inter-pillow matrix has textures consistent with geopetal infilling (Garrison Citation1972). We infer that most pillow lava in the Riverton section was intrusively emplaced into wet volcaniclastic sediment, as seen at other submarine eruptive centres (e.g. Snyder and Fraser Citation1963; Hanson and Hargrove Citation1999). Many of the dikes display apophyses, undulating margins, and engulfed domains of lapilli tuff, indicating that they also intruded unconsolidated material. Multiple layers of vesicles within both the dikes and the pillows indicate that multiple injections of magma occurred (Andrews Citation2003).
The lapilli tuff is dominated by clasts with finely crystalline groundmass and abundant amygdales, interpreted as pillow-fragment clasts. Fragments of dikes, identified by their characteristic flow banded textures, are also present in the lapilli tuff. Clasts are angular, with curviplanar margins; these shapes suggest non-explosive (mechanical) quench-fragmentation processes (; A,E; McPhie et al. Citation1993). There are no scoriaceous clasts, or bubble-wall glass shards in the matrix (McPhie et al. Citation1993), and no moulded or fluidal shapes that might suggest hot pyroclasts (Cousineau and Bédard Citation2000). We interpret the lapilli tuff as resedimented hyaloclastite/hydroclastic debris. Pillow lavas do not fragment when their outsides are quenched (White et al. Citation2003), so this suggests that while some lapilli tuff is derived from pillow lava, perhaps by slumping down the lava front, most was formed as lapilli and ash fragments directly, from the same magma producing the pillow lava, perhaps by mechanical stress or hydromagmatic explosions (Cas Citation1992; Nemeth et al. Citation2008). The peperitic nature of contacts between the pillow lavas and the lapilli tuff (C), the feeder dike which comes to a bulbous end with fragments of dike rock surrounding it, and the pillow-fragment clasts in lapilli tuff underlying pillow lavas, indicate that this debris contains abundant pillow lava and dike fragments formed from a previous eruption. These must underlie the pillow lava observed and are not (with the exception of the single feeder dike noted) seen in the section (). The vesicles in clasts of the lapilli tuff are smaller and less abundant, which could indicate that the magma from which they are derived (the same as that of the unseen pillow lava) had a lesser volatile content or formed at a greater depth than the observed pillow lavas.
Clasts from hyaloclastite lapilli tuff formed by in-place (quench/spall) fragmentation of lava are inferred to have been transported and redeposited in mass flows to form laterally extensive deposits in this section (Yamagishi Citation1987, Citation1991; McPhie et al. Citation1993; Soriano et al. Citation2014). Such deposits are common in submarine stratovolcanoes (Kralj Citation2012), and result in an apron of volcaniclastic material surrounding the volcano (Allen et al. Citation2007; Manville et al. Citation2009). Bedding characteristics are consistent with the lapilli tuff here having formed by granular flows that produced normal-graded, reverse-graded or ungraded, centimetre- to decimetre-scale beds (McPhie et al. Citation1993). Mass flows may have been triggered by volcanic processes (Houghton and Landis Citation1989), including shallow intrusions into unstable primary deposits (Yamagishi Citation1987).
Fine tuff is interpreted as deposits of turbidity currents () with partial Ta-c Bouma sequences, and includes cross-laminated beds that consistently indicate westward flow at the depositional sites. Argillite contains silt grains comprising albite and augite, which are consistent with a volcanic origin (Houghton and Landis Citation1989). Argillite shows Bouma Tb-e sequences (D), consistent with deposition from turbidity currents distal from the source (McPhie et al. Citation1993; Allen et al. Citation2007). Unidirectional ripple cross-lamination shows that these currents flowed northwards, transverse to the flow of turbidity currents which deposited the tuff.
The tuff and argillite are interbedded towards the top of the tuff, and the argillite interbeds thicken upwards until the section passes entirely into argillite. The two lithofacies both appear to have formed from turbidity currents, delivered from different directions and coming from different source sites. If the volcanic centres of the Riverton section were submarine satellite vents of a larger stratovolcano, the origin of the extremely fine ash that formed the argillite could have been subaerial eruptions from the main volcano. Alternatively, Gill et al. (Citation2018) assert that explosive submarine volcanism can produce turbidity currents of fine ash that may spread several kilometres from the vent. In either case, flow directions suggest that the main volcano sloped northwards from a high that lay south of the Riverton section.
Little ash is typically produced during emplacement of pillow lavas or associated hyaloclastite (White et al. Citation2003). Le Masurier (Citation2002), however, describes a thick hyaloclastite sequence with up to 33% fine ash, in a subglacial setting where similar magma/cold water fragmentation processes would have occurred. If the Riverton tuff comprises only fine-ash generated during emplacement of hyaloclastite, it would imply a ‘source’ hyaloclastite sequence much larger than what is exposed in the described section (Cas Citation1992). This is plausible, given that there are nearby isolated inland outcrops of hyaloclastite lapilli tuff. Alternatively, the tuff could represent explosive processes instead. There is no other evidence of explosive eruptions at these vents, but the ash was deposited, or redeposited, by turbidity currents and then hydrothermally altered, so diagnostic evidence has been lost.
Volcano depositional systems
The long lateral sequence from Riverton along the coast of Colac Bay allows inferences to be made about the depositional systems fed by submarine volcanism. Submarine volcanic environments, with steep slopes and a high supply of clastic material, commonly form sedimentary features such as submarine fans, ramps and canyons (e.g. Orton Citation1996; Gamberi Citation2001; Weiß et al. Citation2016). Houghton and Landis (Citation1989) identified volcaniclastic turbidite deposits with lateral facies variations consistent with submarine fan deposits in the Takitimu Mountains. The laterally extensive exposuresin the Riverton section allow more-detailed exploration of relationships within the submarine fan. In this section, the lapilli tuff has beds formed by granular flows. Tuff forms a series of turbidites, and cross-laminations in the western part of the section indicate that turbidity currents flowed westward. Lapilli tuff locally appears in channels within the tuff succession; the long axes of these channels also trend east–west, consistent with a fan developed west of vent source(s).
Growth of an individual fan lobe forms a progradational succession (Yamagishi Citation1987; Nichols Citation2009), which is consistent with the bedding patterns of the lapilli tuff in the Riverton section (A). The tuff turbidites may be the more distal deposit of this fan lobe. Submarine debris flows may have turbiditic tails that produce beds grading to fine-grained tops (Brown et al. Citation2009). The channels in the Riverton section could have been incised by the erosive heads of debris flows or high-concentration turbidity currents and then filled by deposition from later parts of the same flows as velocity waned (Lowe Citation1982; Vallance and Pierson Citation2015). Processes may have been more complex here, however, because the coarse-grained channel deposits are not obviously identical, in terms of particle types, to the lapilli tuff. There are also differences between the tuff to the west and the tuff within the zone where channelised deposits are prominent.
Another possibility is that the lapilli tuff is a large-scale channel deposit (B), with tuff turbidites forming levees at its sides (Morris and Busby-Spera Citation1990; Gamberi Citation2001; Nichols Citation2009). Channel deposits typically comprise coarse sands and gravels such as these, and may form graded beds (Nichols Citation2009). Partial Bouma sequences typically dominate levee turbidites (Nichols Citation2009). The channels into the tuff could then be distributaries from a main channel as on many submarine fans (Gamberi Citation2001). The flow direction of currents that deposited the tuff, away from the lapilli tuff, is consistent with this, but the scale of the conglomerate-filled channel is much smaller than modern submarine-fan features (Morris and Busby-Spera Citation1990; Gamberi Citation2001).
We infer that the western lapilli tuff-tuff sequence was deposited as a single submarine fan lobe (A, ). It is possible that the other areas of lapilli tuff around the western vent (those to the south of the pillow lava pile and the isolated outcrops further inland) represent marginal deposits of other fan lobes, possibly from other fans ().
Figure 11. depositional system scenarios for the Riverton section (not to scale); A, Single lobe of a submarine fan, with lapilli tuff-filled channels. B, Large-scale lapilli tuff-filled channel with tuff turbidites forming levees.
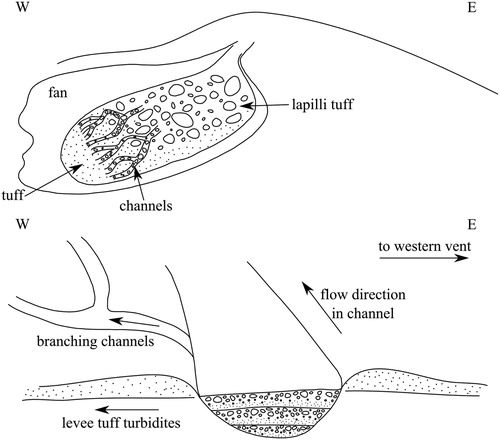
Figure 12. interpretative cross-section of the Riverton section along the Colac Bay coast, showing a proposed structure and sequence of events for deposits of the western vent (not to scale): A, Initial generation of pillow lava is accompanied by generation of hyaloclastite which is redeposited in a submarine fan lobe by a series of channel-forming mass flows. B, A second (observed) generation of pillow lava intrudes these deposits to form peperite.
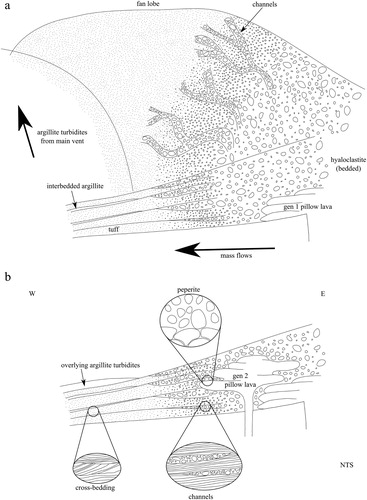
In volcanic flank systems such as this, early-emplaced coherent volcanic units control the distribution of other facies (Gamberi Citation2001). If the approximate placement of vents suggested in is correct, the resedimented volcaniclastic deposits in the area from Howells Point northwards along the Riverton Peninsula were probably emplaced by flows from the eastern vent area towards the western vent area. Their path would thus have been influenced by the piles of pillow lava plus hyaloclastite forming at both vents. The precise location of the eastern vent is unknown, but it must have been sufficiently nearby for pillow lavas from it to reach the observed section (White et al. Citation2015).
Gamberi (Citation2001) describes a process where a single turbulent gravity flow (turbidity current) separates downstream, resulting in channels with longitudinal banks between them. Such banks are characterised by fine-grained sediment; it is plausible that this arrangement could account for the relationships observed. Channelised deposits may be massive or have thick and/or indistinct bedding (Nichols Citation2009), whereas fan lobes are more commonly well-bedded. We infer that the well-bedded deposits of this part of the coastline represent fan lobes from the eastern volcano; the poorly bedded lapilli tuff and bedded tuff between them comprise a branching channel system ().
Conclusions
The kilometres-long lateral ‘Riverton section’, from Riverton to Colac Bay, shows that a single large oceanic arc edifice/volcano hosted multiple non-central submarine vents, fed from different magma sources, and represented by distinct, but interfingering, depositional successions that were each host to significant shallow-level basaltic intrusions. Lithofacies and geochemical evidence suggest that the centres tapped different mantle domains within a transitional zone between the arc and back-arc. Geochemical characteristics indicate that the magmas also underwent fractional crystallisation in two separate magma storage zones before erupting.
Lapilli tuff is a major lithofacies comprising redeposited hyaloclastite, emplaced by a series of granular flows produced simultaneously with pillow lava effusion, and like fine tuff and argillite, was emplaced by a series of density currents. Detritus forming argillite was derived from the main volcano and played the same ‘background’ role as terrestrial hemipelagic sediment in other settings. Tuff turbidites were shed from the satellite vents.
The overall sequence of events was as follows. Feeder dikes and pillow lava piles formed from two small vents on the relatively deep-water flanks of a submarine island arc stratovolcano. These vents also produced hyaloclastite, which was resedimented in systems of fans and channels across an area that was also accumulating argillite fed by turbidity currents from the volcano’s main vent. Dikes and intrusive pillow lavas were emplaced into all of these deposits while they were still unconsolidated, and commonly produced peperites.
Supplemental material
Download MS Word (693.4 KB)Acknowledgements
This paper is based on a Master’s of Science study at the University of Otago. We acknowledge the support of the University of Otago, by means of the University of Otago Postgraduate Publishing Bursary (Master’s). Scanning Electron Microscope analyses were performed at the Otago Centre for Electron Microscopy, and LA-ICP-MS at the Centre for Trace Element Analysis. We thank Professor Karoly Nemeth for his helpful comments on this paper.
Data availability statement
The data that support the findings of this study are openly available in Pangaea https://doi.pangaea.de/10.1594/PANGAEA.917577, reference number PDI-23688.
Disclosure statement
No potential conflict of interest was reported by the author(s).
References
- Allen SR, Hayward BW, Mathews E. 2007. A facies model for a submarine volcaniclastic apron: the Miocene Manukau Subgroup, New Zealand. Geological Society of America Bulletin. 119:725–742. doi: 10.1130/B26066.1
- Andrews B. 2003. Eruptive and depositional mechanisms of an Eocene shallow submarine volcano, Moeraki Peninsula, New Zealand. In: White JDL, Smellie JL, Clague DA, editors. Explosive subaqueous volcanism. Washington (DC): American Geophysical Union; p. 179–188.
- Bédard JH. 2014. Parameterizations of calcic clinopyroxene – melt trace element partition coefficients. Geochemistry Geophysics Geosystems. 15(2):303–336. doi: 10.1002/2013GC005112
- Blattner P, Williams JG. 1990. The Largs high-altitude oxygen isotope anomaly (New Zealand) and climatic controls of oxygen isotopes in magma. Earth and Planetary Science Letters. 103:270–284. doi: 10.1016/0012-821X(91)90166-F
- Bradshaw JD. 1994. Brook Street and Murihiku terranes of New Zealand in the context of a mobile South Pacific Gondwana margin. Journal of South American Earth Sciences. 7(3):325–332. doi: 10.1016/0895-9811(94)90018-3
- Brown DJ, Holohan EP, Bell BR. 2009. Sedimentary and volcano-tectonic processes in the British Paleocene Igneous Province: a review. Geological Magazine. 146(3):326–352. doi: 10.1017/S0016756809006232
- Busby-Spera CJ, White JDL. 1987. Variation in peperite textures associated with differing host-sediment properties. Bulletin of Volcanology. 49:765–775. doi: 10.1007/BF01079827
- Cas RAF. 1992. Submarine volcanism eruption styles, products, and relevance to understanding the host-rock successions to volcanic-hosted massive sulfide deposits. Economic Geology. 87:511–541. doi: 10.2113/gsecongeo.87.3.511
- Cas RAF, Giordano G. 2014. Submarine volcanism: a review of the constraints, processes and products, and relevance to the Cabo de Gata volcanic succession. Italian Journal of Geosciences. 133(3):362–377. doi: 10.3301/IJG.2014.46
- Chadwick WW, Clague DA, Embley RW, Perfit MR, Butterfield DA, Caress DW, Paduan JB, Martin JF, Sasnett P, Merle SG, Bobbitt AM. 2013. The 1998 eruption of axial seamount: new insights on submarine lava flow emplacement from high-resolution mapping. Geochemistry, Geophysics, Geosystems. 14(10):3939–3968. doi: 10.1002/ggge.20202
- Cousineau PA, Bédard JH. 2000. Sedimentation in a subaqueous arc/back-arc setting: the Bobby Cove Formation, Snooks Arms Group, Newfoundland. Precambrian Research. 101:111–134. doi: 10.1016/S0301-9268(99)00097-2
- Davidson J, De Silva S. 2000. Composite volcanoes. In: Sigurdursson H, Houghton B, Rymer H, Stix J, McNutt S, editors. Encyclopedia of volcanoes. 1st ed. London: Academic Press; p. 663–681.
- De Silva SL, Lindsay JM. 2015. Primary volcanic landforms. In: Sigurdsson H, Houghton BF, Rymer H, Stix J, McNutt S, editors. Encyclopedia of volcanoes. 2nd ed. New York (NY): Academic Press; p. 274–295.
- Doucet P, Mueller W, Chartrand F. 1994. Archean, deepwater, volcanic eruptive products associated with the Coniagas massive deposit, Quebec, Canada. Canadian Journal of Earth Sciences. 31:1569–1584. doi: 10.1139/e94-139
- Doyle MG. 2000. Clast shape and textural associations in peperite as a guide to hydromagmatic interactions: Upper Permian basaltic and basaltic andesite examples from Kiama, Australia. Australian Journal of Earth Sciences. 47:167–177. doi: 10.1046/j.1440-0952.2000.00773.x
- Ewart A, Collerson KD, Regelous M, Wendt JI, Niu Y. 1998. Geochemical evolution within the Tonga–Kermadec–Lau arc–back-arc systems: the role of varying mantle wedge composition in space and time. Journal of Petrology. 39(3):331–368. doi: 10.1093/petroj/39.3.331
- Faure G. 2001. Origin of igneous rocks: the isotopic evidence. Berlin: Springer-Verlag.
- Frost CD, Coombs DS. 1989. Nd isotope character of New Zealand sediments: implications for terrane concepts and crustal evolution. American Journal of Science. 289:744–770. doi: 10.2475/ajs.289.6.744
- Gamberi F. 2001. Volcanic facies associations in a modern volcaniclastic apron (Lipari and Vulcano offshore, Aeolian Island Arc). Bulletin of Volcanology. 63:264–273. doi: 10.1007/s004450100143
- Garrison RE. 1972. Inter- and intrapillow limestones of the Olympic Peninsula, Washington. The Journal of Geology. 80(3):310–322. doi: 10.1086/627734
- Gill JB, Bongiolo EM, Miyazaki T, Hamelin C, Jutzeler M, DeBari S, Jonas A-S, Vaglarov BS, Nascimento LS, Yakavonis M. 2018. Tuffaceous mud is a volumetrically important volcaniclastic facies of submarine arc volcanism and record of climate change. Geochemistry, Geophysics, Geosystems. 19:1217–1243. doi: 10.1002/2017GC007300
- Hanson RE, Hargrove US. 1999. Processes of magma/wet sediment interaction in a large-scale Jurassic andesitic peperite complex, northern Sierra Nevada, California. Bulletin of Volcanology. 60:610–626. doi: 10.1007/s004450050255
- Haston RB, Luyendyk BP, Landis CA, Coombs DS. 1989. Paleomagnetism and question of original location of the Permian Brook Street Terrane, New Zealand. Tectonics. 8:791–801. doi: 10.1029/TC008i004p00791
- Hochstaedter AG, Gill JB, Taylor B, Ishizuka O, Yuasa M, Morita S. 2000. Across-arc geochemical trends in the Izu-Bonin arc: constraints on source composition and mantle melting. Journal of Geophysical Research. 105(B1):495–512. doi: 10.1029/1999JB900125
- Houghton BF, Landis CA. 1989. Sedimentation and volcanism in a Permian arc-related basin, southern New Zealand. Bulletin of Volcanology. 51:433–450. doi: 10.1007/BF01078810
- Kano K, Yamamoto T, Takeuchi K. 1993. A Miocene island-arc volcanic seamount: the Takashibiyama Formation, Shimane Peninsula, SW Japan. Journal of Volcanology and Geothermal Research. 59:101–119. doi: 10.1016/0377-0273(93)90080-B
- Kralj P. 2012. Facies architecture of the Upper Oligocene submarine Smrekovec stratovolcano, Northern Slovenia. Journal of Volcanology and Geothermal Research. 247–248:122–138. doi: 10.1016/j.jvolgeores.2012.07.016
- Le Masurier WE. 2002. Architecture and evolution of hydrovolcanic deltas in Marie Byrd Land, Antarctica. In: Smellie JL, Chapman MG, editors. Volcano-ice interaction on Earth and Mars. London: Geological Society; p. 115–148. Special Publications, 202.
- Lowe DR. 1982. Sediment gravity flows: II. Depositional models with special reference to the deposits of high-density turbidity currents. Journal of Sedimentary Petrololgy. 52:279–297.
- Manville V, Németh K, Kano K. 2009. Source to sink: a review of three decades of progress in the understanding of volcaniclastic processes, deposits, and hazards. Sedimentary Geology. 220(3–4):136–161. doi: 10.1016/j.sedgeo.2009.04.022
- Maurice AE, Basta FF, Khiamy AA. 2012. Neoproterozoic nascent island arc volcanism from the Nubian Shield of Egypt: magma genesis and generation of continental crust in intra-oceanic arcs. Lithos. 132–133:1–20. doi: 10.1016/j.lithos.2011.11.013
- McPhie J, Doyle M, Allen R. 1993. Volcanic textures: a guide to the interpretation of textures in volcanic rocks. Hobart: CODES Key Centre, University of Tasmania.
- Morley CK. 2018. 3-D seismic imaging of the plumbing system of the Kora Volcano, Taranaki Basin, New Zealand: the influence of syn-rift structure on shallow igneous intrusion architecture. Geosphere. 14(6):2533–2584. doi: 10.1130/GES01645.1
- Morris W, Busby-Spera C. 1990. A submarine-fan valley-levee complex in the Upper Cretaceous Rosario Formation: Implication for turbidite facies models. Geological Society of America Bulletin. 102(7):900–914. doi: 10.1130/0016-7606(1990)102<0900:ASFVLC>2.3.CO;2
- Mortimer N, Gans P, Calvert A, Walker N. 1999. Geology and thermochronometry of the east edge of the Median Batholith (Median Tectonic Zone): a new perspective on Permian to Cretaceous growth of New Zealand. The Island Arc. 8:404–425. doi: 10.1046/j.1440-1738.1999.00249.x
- Nemeth K, Pecskay Z, Martin U, Gmeling K, Molnar F, Cronin SJ. 2008. Hyaloclastites, peperites and soft-sediment deformation textures of a shallow subaqueous Miocene rhyolitic dome-cryptodome complex, Palhaza, Hungary. Geological Society Special Publications. 302:63–86. doi: 10.1144/SP302.5
- Nichols G. 2009. Sedimentology and stratigraphy. Chichester: Wiley-Blackwell.
- Orton GJ. 1996. Volcanic environments. In: Reading HG, editor. Sedimentary environments: processes, facies, and Stratigraphy. 3rd ed. Oxford: Blackwell Science; p. 485–566.
- Pearce JA, Peate DW. 1995. Tectonic implications of the composition of volcanic arc magmas. Annual Reviews in Earth and Planetary Sciences. 23:251–285. doi: 10.1146/annurev.ea.23.050195.001343
- Pearce JA, Stern RJ. 2006. Origin of back-arc basin magmas: trace element and isotope perspectives. Back-arc spreading systems: geological, biological, chemical, and physical interactions. Geophysical Monograph Series. 166:63–86.
- Portner RA, Daczo NR, Dickinson JA. 2010. Vitriclastic lithofacies from Macquarie Island (Southern Ocean): compositional influence on abyssal eruption explosivity in a dying Miocene spreading ridge. Bulletin of Volcanology. 72:165–183. doi: 10.1007/s00445-009-0312-8
- Price RC, Ireland TR, Maas R, Arculus RJ. 2006. SHRIMP ion probe zircon geochronology and Sr and Nd isotope geochemistry for southern Longwood Range and Bluff Peninsula intrusive rocks of Southland, New Zealand. New Zealand Journal of Geology and Geophysics. 49(3):291–303. doi: 10.1080/00288306.2006.9515168
- Rossel P, Oliveros V, Ducea M, Charrier R, Scaillet S, Retamal L, Figueroa O. 2013. The Early Andean subduction system as an analogue to island arcs: evidence from across-arc geochemical variations in northern Chile. Lithos. 179(2):211–230. doi: 10.1016/j.lithos.2013.08.014
- Schmidt MW, Jagoutz O. 2017. The global systematics of primitive arc melts. Geochemistry, Geophysics, Geosystems. 18:2817–2854. doi: 10.1002/2016GC006699
- Sivell W, Rankin P. 1983. Arc-tholeiite and ultramafic cumulates, Brook Street Volcanics, west D’Urville Island, New Zealand. New Zealand Journal of Geology and Geophysics. 26:239–257. doi: 10.1080/00288306.1983.10422238
- Skilling IP, White JDL, McPhie J. 2002. Peperite: a review of magma-sediment mingling. Journal of Volcanology and Geothermal Research. 114:1–17. doi: 10.1016/S0377-0273(01)00278-5
- Snyder GL, Fraser GD. 1963. Pillow lavas, 1: intrusive layered lava pods and pillow lavas, Unalaska Island, Alaska. United States Geological Survey Professional Paper. 454-B:1–23.
- Soriano CC, Giordano G, Riggs NR, Porreca M, Bonamico A, Iosimi D, Cifelli F, Mattei M, De Benedetti A, Guarnieri L, Marchionni S. 2014. Geologic map, volcanic stratigraphy and structure of the Cabo de Gata volcanic zone, Betic-Rif orogen, SE Spain. Italian Journal of Geosciences. 133(3):325–340. doi: 10.3301/IJG.2014.45
- Spandler CJ, Arculus RJ, Eggins SM, Mavrogenes JA, Price RC, Reay AJ. 2003. Petrogenesis of the Green Hills Complex, Southland, New Zealand: magmatic differentiation and cumulate formation at the roots of a Permian island arc volcano. Contributions to Mineralogy and Petrology. 144:703–721. doi: 10.1007/s00410-002-0424-z
- Spandler C, Worden K, Arculus R, Eggins S. 2005. Igneous rocks of the Brook Street Terrane, New Zealand: Implications for Permian tectonics of eastern Gondwana and magma genesis in modern intra-oceanic volcanic arcs. New Zealand Journal of Geology and Geophysics. 48(1):167–183. doi: 10.1080/00288306.2005.9515107
- Sun S-S, McDonough WF. 1989. Chemical and isotopic systematics of oceanic basalts: implications for mantle composition and processes. In: Saunders AD, Norry MJ, editors. Magmatism in ocean basins. London: Geological Society Special Publication; p. 313–345.
- Turnbull IM, Allibone AH. (compilers). 2003. Geology of the Murihiku area. Institute of Geological & Nuclear Sciences 1:250 000 geological map 20. 1 sheet and 74 p. Lower Hutt, New Zealand. Institute of Geological & Nuclear Sciences Limited.
- Vallance JW, Pierson T. 2015. Lahars and their deposits. In: Sigurdursson H, Houghton B, Rymer H, Stix J, McNutt S, editors. Encyclopedia of volcanoes. 2nd ed. New York (NY): Academic Press; p. 663–681.
- Walker GPL. 1992. Morphometric study of pillow-size spectrum among pillow lavas. Bulletin of Volcanology. 54(6):459–474. doi: 10.1007/BF00301392
- Weiß BJ, Hübscher C, Lüdmann T, Serra N. 2016. Submarine sedimentation processes in the southeastern Terceira Rift/São Miguel region (Azores). Marine Geology. 374:42–58. doi: 10.1016/j.margeo.2016.02.004
- White JDL, Houghton BF. 2006. Primary volcaniclastic rocks. Geological Society of America Bulletin. 34:677–680.
- White JDL, McPhie J, Soule SA. 2015. Submarine lavas and hyaloclastite. In: Sigurdsson H, Houghton B, Rymer H, Stix J, McNutt S, editors. Encyclopedia of volcanoes. 2nd ed. New York (NY): Academic Press; p. 363–375.
- White JDL, Smellie JL, Clague DA. 2003. Introduction: a deductive outline and topical overview of subaqueous explosive volcanism. In: White JDL, Smellie JL, Clague DA, editors. Explosive subaqueous volcanism. Washington (DC): American Geophysical Union; p. 1–20.
- Yamagishi H. 1987. Studies on the Neogene subaqueous lavas and hyaloclastites in Southwest Hokkaido. Report of the Geological Survey of Hokkaido No. 59. 55–117.
- Yamagishi H. 1991. Morphological and sedimentological characteristics of the Neogene submarine coherent lavas and hyaloclastites in Southwest Hokkaido, Japan. Sedimentary Geology. 74:5–23. doi: 10.1016/0037-0738(91)90032-9